Abstract
Purpose
Renal carcinoma (RC) originates in the renal tubular epithelial system, among which renal cell carcinoma (RCC) is the most frequent one. The forkhead activin signal transducer 1 (FAST1) has been shown to interfere with tumor progression as an oncogene, while its role in RC is limited. Therefore, this paper explored the prognostic significance, specific effects, and related mechanisms of FAST1 on RC.
Patients and Methods
Cell colony formation assay, cell counting kit-8 (CCK8) assay, flow cytometry and Transwell assay were used to test cell proliferation, viability, apoptosis, migration and invasion, respectively. Western blot (WB) was employed to determine the protein level of FAST1.
Results
Our study confirmed that FAST1 was up-regulated in RC tissues and cell lines, and its overexpression often represented a poor prognosis of RC patients. Meanwhile, the in vitro experiments showed that overexpressing FAST1 facilitated RC cell viability, proliferation, migration, invasion and epithelial-mesenchymal transition (EMT), and repressed cell apoptosis. In addition, the in vivo experiments illustrated that the up-regulation of FAST1 strengthened tumor growth. On the contrary, knocking down FAST1 had the opposite effects. Mechanistically, The TGF-β/Smad pathway contributed to RC evolvement and was activated by FAST1 both in vitro and in vivo.
Conclusion
This article suggests that FAST1 exerts a carcinogenic role in RC by regulating the TGF-β/Smad signaling.
Introduction
Renal carcinoma (RC) is a highly malignant tumor of the urinary system, with insidious onset and high mortality. Renal cell carcinoma (RCC) is the most typical type of RC. Due to the lack of specific markers and typical clinical manifestations, early RC is not easily detected. Studies have revealed that the five-year survival rate of early RC patients is about 90%, while that of advanced RC patients falls to 10%.Citation1 Worse still, advanced RC patients are not suitable for surgery and are resistant to chemoradiotherapy, with high metastasis and recurrence rate. Meanwhile, they are not sensitive to immunotherapy.Citation2 Therefore, it is crucial to find new biomarkers of RC. This paper aims to study new RC markers to improve the poor prognosis of RC.
Forkhead activin signal transducer 1 (FOXH1), also called FAST1, is encoded by a gene located on 8q24.3. Functionally, FOXH1 acts as a primitive-streak specifier and activin co-effector, thus having a vital role in the development by promoting the generation of human-induced pluripotent stem cells (iPSCs) from somatic cells via cellular signaling transduction.Citation3 Moreover, FOXH1 binds SMAD2 and activates an activin response element by binding the DNA motif TGT(G/T) (T/G) ATT.Citation4 Recently, FAST1 has been found dysregulated in several tumors and contributes to tumor growth and metastasis. Some studies have demonstrated that the FAST1 level is positively related to the poor prognosis of colorectal cancer (CRC). Overexpressing FAST1 enhances cell proliferation, migration, invasion, and pulmonary metastasis of CRC.Citation5 Additionally, Let-7b and let-7f inhibit cell proliferation by blocking the cell cycle of the G1-S transition through directly targeting FOXH1.Citation6 However, the specific effects of FAST1 on RC, especially the interaction between FAST1 and different downstream pathways on RC, need to be further studied.
Transforming growth factor β (TGF-β) is a disulfide-bonded dimer protein containing three ligands, namely TGF-β1, TGF-β2 and TGF-β3. Current studies have found that TGF-β has a dual role in tumors. It inhibits tumors in the early stage while acts as a carcinogen in the advanced stage.Citation7 Oshimori et al have illustrated that TGF-β plays a carcinogenic role in squamous cell carcinoma and that tumor cells that are highly sensitive to TGF-β have a higher invasion and metastasis rate and stronger drug resistance than those are less sensitive to TGF-β.Citation8 Solvated metal atom dispersed (Smad) is one of the classic TGF-β dependent proteins with a length of about 500 amino acids, containing spherical MH1 and MH2 domains and a cohesive variable zone. Smad2, a vital member of the Smad family, often acts as an activator of TGF-β.Citation9 Some scholars have found that the activated TGF-β/Smad pathway mediates the dysfunction of NK cells and induces immune escape in B-acute lymphoblastic leukemia (ALL).Citation10 Similar carcinogenic effects were also observed in renal cancer. For instance, Liu et al revealed that miR-93 targets RUNX3 and regulates the TGF-β/Smad4 signaling pathway to accelerate RCC.Citation11 Interestingly, FAST-1 mediates embryogenesis by transducing TGF-β superfamily signals.Citation12
In this study, we found FAST1 was significantly up-regulated in terms of its expression in RC tissues. Further analysis showed that a higher level of FAST1 was associated with lower survival of RC patients. Combined with the above findings, both FAST1 and the TGF-β/Smad signal can interfere with RC progression. Therefore, we hypothesized that FAST1 influences the progression of RC by regulating the TGF-β/Smad signaling pathway.
Patients and Methods
Clinical Samples
Twenty-eight patients who underwent surgical RCC in Zaozhuang Municipal Hospital from June 2016 to June 2017 were selected, and their RCC tissues and paired normal adjacent tissues were obtained. Tissue samples were immediately frozen in −80°C liquid nitrogen for subsequent experiments. All the sample patients did not receive radiotherapy, chemotherapy and other adjuvant treatment before the surgery. All samples were confirmed by the pathology department of our hospital. The patients and their family members signed informed consent. This study was authorized by the Ethics Committee of Zaozhuang Municipal Hospital. The FAST1 expression was tested by quantitative reverse transcription PCR (qRT-PCR), and the expression level > 2.5 was defined as high.
Cell Culture and Transfection
Human normal proximal renal tubular cell lines (OAT1) and RC cell lines (769-P and ACHN) were bought from the American Type Culture Collection (ATCC, Rockville, MD, USA). Cells were cultured in the RPMI-1640 medium (Thermo Fisher Scientific, MA, USA) supplemented with 10% fetal bovine serum (FBS) (Thermo Fisher Scientific, MA, USA) and 1% penicillin/streptomycin (Invitrogen, CA, USA) and incubated at 37 °C with 5% CO2. When the cell abundance reached 70%-80%, FAST1 overexpression plasmids (FAST1), knockdown plasmids (Si-FAST1#1 and Si-FAST1#2), and corresponding control group (NC and Si-NC) were transfected into 769-P and ACHN with the Lipofectamine2000 transfection agent (ThermoFisherScience, Waltham, MA, USA). The cells were collected for subsequent experiments after incubation for 48 hours. The TGF-β inhibitor RepSox was obtained from Abcam (2 µmol/L, ab142139, USA).
qRT-PCR
Total RNA was isolated from 769-P and ACHN cells with the TRIzol reagent (Invitrogen, Carlsbad, CA, USA), and its concentration was measured with a NanoDrop2000 photometer (Thermo Fisher, Waltham, MA, USA). Then, RNA was reverse-transcribed into cDNA using the PrimeScript™ RT Reagent kit (Invitrogen, Shanghai, China) following the manufacturer’s instructions, and qRT-PCR was operated on the Applied Biosystems StepOnePlus System (Foster City, CA, USA). GAPDH was the internal reference of FAST1, and the 2(-ΔΔCt) method was adopted for statistics. Each experiment was done three times. The specific primer sequences include FAST1, forward, 5ʹ-GAGGTACCTGCGACATGACA-3ʹ, reverse, 5ʹ-GTTGTGGCGAATGGAGTCTT-3ʹ. GAPDH, forward, 5ʹ- TGGTTGAGCACAGGGTACTT −3ʹ reverse: 5ʹ- CCAAGGAGTAAGACCCCTGG −3ʹ.
Western Blot (WB)
The cells were lysed in the RIPA lysis buffer and electrically transferred to the polyvinylidene fluoride (PVDF) membranes, which were then blocked with 5% skimmed milk for 1 hour at room temperature (RT) and washed with TBST three times (10 min each time). Afterward, the membranes were incubated with the primary Anti-FAST1 antibody (1:1000, ab189960, Abcam, USA), Anti-E-Cadherin antibody (1:1000, ab231303, Abcam, USA), Anti-Claudin 1 antibody (1:1000, ab211737, Abcam, USA), Anti-N Cadherin antibody (1:1000, ab76057, Abcam, USA), Anti-Snail antibody (1:1000, ab82846, Abcam, USA), Anti-TGF-β (phospho S165) (1:1000, ab183037, Abcam, USA), Anti-TGF-β antibody (1:1000, ab36495, Abcam, USA), Anti-Smad2 (phospho S255) antibody (1:1000, ab188334, Abcam, USA), Anti-Smad2 antibody (1:1000, ab40855, Abcam, USA) and Anti-GAPDH antibody (1:1000, ab181603, Abcam, USA) at 4 °C overnight. After being rinsed with TBST, the membranes were incubated with the horseradish peroxidase (HRP)-labeled Goat Anti-Rabbit IgG (1:3000, ab6721, Abcam, USA) for 1 hour at RT. Then, the membranes were washed with TBST three times (10 min each time). Finally, ECL Western blot special reagents (Invitrogen) were used for color development and imaging, and Image J was applied to analyze each protein’s gray intensity.
Cell Colony Formation Experiment
The stably transfected 769-P and ACHN cells were inoculated in 6-well plates at 1×103/well and treated with overexpressed FAST1 and knocked down FAST1 to testify the colony formation. After the cells were incubated at 37 °C with 5% CO2 for two weeks, they were cleared with PBS and stained with crystal violet solution (0.1% crystal violet) for 15 minutes. The stained colonies were counted with an optical microscope (Olympus, Tokyo, Japan).
Flow Cytometry
Two groups of stably transfected cells were inoculated in 96-well plates at 1×104 cells/well. After 24 hours of culture, the cells were washed twice with PBS, fixed with 75% ethanol, and preserved overnight at 4 °C. The cells were then suspended in PBS washing buffer (1×106 cells/mL). Afterward, the propidium iodide staining solution was added for staining for 30 minutes, and the cells were stained with the Annexin V-fluorescein isothiocyanate/PI kit (cat. no. 556,570, BD Biosciences, New Jersey, USA). Flow cytometry (Beckman Coulter, Fullerton, CA, USA) was employed for cell detection, and FlowJo 10 (FlowJo LLC) was adopted for apoptosis analysis.
Cell Counting Kit-8 (CCK8) Assay
Two groups of cells (2×103 cells in each group) were taken and inoculated into 96-well plates. Cell proliferation was detected with the CCK8 kit (Beyotime Biotechnology, Shanghai, China), and 10 μL of CCK8 solution was added to each well after 24 hours of adherent culture. The culture plates were incubated for 4 hours, and the absorbance value was observed with a microplate reader (Bio-Rad Laboratories, Hercules, CA, USA) at 450 nm. The proliferation changes were monitored at 0, 24, 48, 72, and 96 hours after the transfection or the inhibitor treatment.
Transwell Assay
Transwell assay was employed to test cell migration and invasion. For the cell migration experiment, cells were seeded to the upper chamber of the transwell (2×104/well), and 200 μL culture medium containing 20% FBS was added to the lower chamber for culture at 37 °C. After 12 hours, the cells in the upper chamber were removed, fixed with 4% paraformaldehyde, stained with 0.1% crystal violet for 20 minutes, and washed with PBS. In the invasion assay, the transwell’s upper chamber was precoated with the matrigel (Biosciences, Lincoln, NE) before the cells were added, and the remaining steps were the same as that of the migration assay.
Animal Experiment
BALB/c nude mice (6–8 weeks old) were bought from the JunKeBiological Co., Ltd. (Nanjing, China). They were divided into five groups (five mice in each group) and raised in a specified pathogen-free environment. Meanwhile, 769-P cells stably transfected with FAST1 overexpression plasmids were trypsinized to make single-cell suspension (1×107 cells/mL). Then, 0.1 mL of the suspension was subcutaneously injected into both sides of the back of each nude mouse. The tumor size was measured with a caliper every four days from day 12. The volume was equal to the long diameter × the short diameter2/2. In the fourth week, mice were euthanized, and the tumors in nude mice were carefully dissected and weighed. These trials were conducted in strict accordance with the Guidelines for the Care and Use of Laboratory Animals published by the National Institutes of Health (NIH Publication No. 85–23, 2011). The Ethics Committee of Zaozhuang Municipal Hospital approved all experimental procedures.
Bioinformatic Analysis
Through STRING database (https://string-db.org/), the potential biological function of FAST1 was analyzed by gene ontology (GO) enrichment analysis.Citation13 Next, the protein-protein interaction network was also constructed, which showed the functional interactions between proteins thus insighting into the molecular mechanism of cellular processing. The protein-protein interaction (PPI) network of FAST1 with other proteins were constructed by the online website of STRING. The interacting pairs with a combined score >0.7 (high confidence) were selected for the PPI network construction.
Co-Immunoprecipitation
The 769-P cells were cultured in 6-well plates with 5×105 cells each well. 24 hours after the incubation, the cells were transfected or co-transfected with plasmids, as shown in the figures for 24 h, the nuclear protein was extracted for immunoprecipitation. The ANTI-FLAG® M2 Affinity Gel was added into the lysis and incubated with continuing slow rotation at 4 °C overnight. After washing and denatured, the samples were used for Western blot analysis.
Immunohistochemistry
Immunohistochemistry was used to detect FAST1, p-SMAD2 and E-cadherin in the tumor tissues. 4-um thick histological sections of the paraffin-embedded tissues were prepared, dewaxed, deparaffinized in fresh xylene and dehydrated using a graded series of ethanol solutions. Next, the sections received antigen retrieval (in citrate buffer (0.01 M, PH 6.0)) and then incubation with 1% bovine serum albumin. After that, the sections were then incubated with primary antibodies (FOXH1, 1:200, Biorbyt; p-SMAD2, 1:200, abcam; E-cadherin, 1:500, abcam) at 4 °C overnight. At the next day, horseradish peroxidase-labelled anti-rabbit IgG was used to incubate with the sections after being washed with PBS. Finally, the stained images were observed under a light microscope (Olympus, Japan).
Statistical Analysis
GraphPad Prism 6 Software (GraphPad Software, Inc., City, State) was employed for analysis. The data were presented as mean ± standard deviation (±s). The multi-factor comparison was made by one-way ANOVA, while t-test was used for pairwise comparison. P<0.05 indicated statistical significance.
Results
FAST1 Was Up-Regulated in RC
QRT-PCR and WB were carried out to testify the FAST1 level, and it was found that the FAST1 level in RC tissues was much higher than that in adjacent normal tissues (P < 0.05, and ). Additionally, the patients’ survival analysis manifested that patients with low FAST1 expression had a higher survival rate (P=0.043, ). Through GEPIA (http://gepia.cancer-pku.cn/), we verified that the higher level of FAST1 in kidney renal clear cell carcinoma (KIRC) predicts the poorer survival rate (P < 0.05, ). By analyzing the clinical characteristics of the sample patients, it was found that the higher expression of FAST1 was significantly associated with a more advanced stage of RC, indicated by the higher TNM stage and lymphatic metastasis rate (). These results indicated that FAST1 was overexpressed in RC and might be a new diagnostic marker of RC.
Table 1 Association Between Clinicopathological Features and FAST1 Expression in RCC Tissues (n =28)
Figure 1 FAST1 was up-regulated in RC tissues. RC tissues and paired paracancerous tissues were harvested and stored in −80°C liquid nitrogen. (A and B) QRT-PCR and WB were applied to test the FAST1 expression in RC tissues and adjacent normal tissues, ***P < 0.001 (vs Normal group); (C) survival of RCC patients were analyzed via KM Plotter assay. (D) GEPIA (http://gepia.cancer-pku.cn/) was adopted to query the FAST1 expression and survival prognosis in KIRC.
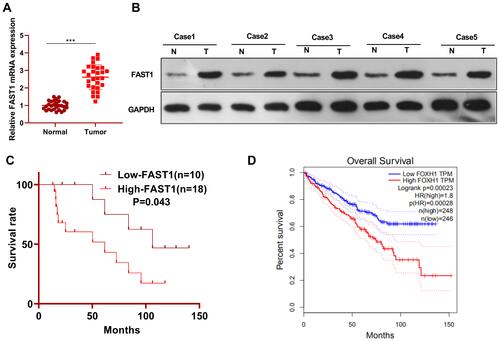
Overexpressing FAST1 Aggravated the Malignant Behaviors of RC Cells
We constructed a FAST1 overexpression model in 769-P cell lines to probe the impact of FAST1 on RC development (P< 0.05, ). Then, the colony formation assay and CCK8 experiment were conducted to test cell proliferation and viability. It was confirmed that overexpressing FAST1 significantly enhanced cell viability and proliferation (P < 0.05, and ). Flow cytometry demonstrated that the number of apoptotic cells was declined after overexpression of FAST1 (P < 0.05, ). Transwell assay testified that cell migration and invasion were strengthened after overexpressing FAST1 (P < 0.05, and ). Moreover, WB was employed to monitor the epithelial-mesenchymal transition (EMT) markers (E-cadherin, Claudin1, N-cadherin, and Snail), and it was found that the expression of E-cadherin and Claudin1 was higher, while the expression of N-cadherin and Snail was lower in the FAST1 group than that of the control group (P < 0.05, ). These results suggested that FAST1 overexpression expedited RC and might be an oncogene of RC.
Figure 2 Overexpressing FAST1 aggravated the malignant biological behaviors of RC and inhibited apoptosis. The 769-P cell lines were transfected with the FAST1 overexpressing plasmids. (A) The FAST1 expression was verified by qRT-PCR. (B) Cell colony formation assay was used to examine cell viability. (C) CCK8 was used to test cell proliferation. (D) Flow cytometry was employed to determine apoptosis. (E and F) Cell migration and invasion were testified by the Transwell assay. (G) The expression of EMT-related markers (E-cadherin, Claudin1, N-cadherin and Snail) was detected by WB. *P< 0.05, **P < 0.01, ***P < 0.001 (vs NC group). N=5.
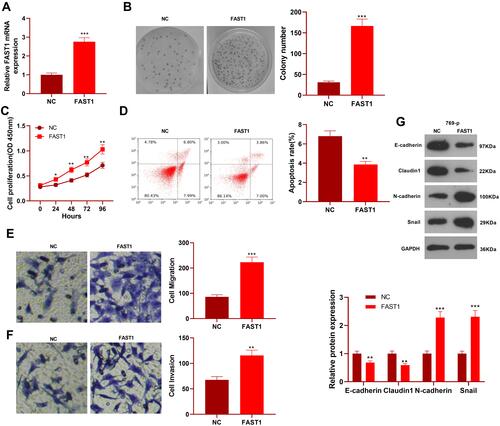
Knockdown of FAST1 Repressed the Malignant Biological Behaviors of RC and Induced Apoptosis
We constructed a FAST1 knockdown model in ACHN cell lines to verify the role of FAST1 in RC (P < 0.05, ). Then, cell proliferation, viability, apoptosis, migration, and invasion were determined by the colony formation experiment, flow cytometry, CCK8 and Transwell assay, respectively. The results confirmed that knocking down FAST1 weakened cell viability and proliferation, promoted apoptosis, and abated cell migration and invasion (P < 0.05, ). Also, WB showed that compared with the control group, E-cadherin and Claudin1 expression was increased, while the N-cadherin and Snail expression was abated in the Si-FAST1 group (P < 0.05, ). These findings illustrated that knocking down FAST1 inhibited the malignant biological behaviors of RC cells and prevented EMT.
Figure 3 Knocking down FAST1 abated the malignant biological behaviors of RC cells. The ACHN cell lines were transfected with sh-FAST1 and negative control (sh-NC). (A) The FAST1 expression was tested by qRT-PCR. (B–F) Cell viability, apoptosis, proliferation, migration and invasion were determined by colony formation assay, CCK8, flow cytometry and Transwell assay, respectively. (G) The levels of E-cadherin, Claudin1, N-cadherin and Snail were monitored by WB. *P < 0.05, **P < 0.01, ***P < 0.001 (vs Si-NC group). N=5.
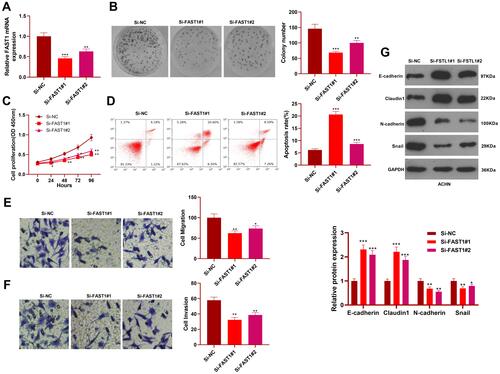
FAST1 Promoted the Growth of RC Cells in vivo
Furthermore, 769-P cells transfected with FAST1 overexpression plasmids were injected into nude mice, and the tumor growth was observed in vivo. The tumor size was determined from day 12 and recorded every four days, and a growth curve was plotted. As a result, the mice’s tumor volume and weight were significantly increased after overexpressing FAST1 compared with the control group (P < 0.05, ). We then performed IHC to detect FAST1 in the tumor tissues. It was found that compared with the NC group, FAST1 was overexpressed in the tumor tissues, mainly located at the cytoplasm and nucleus (). Then, the EMT marker expression in the tumor was tested by IHC and WB. The results confirmed that the levels of E-cadherin and Claudin1 in the FAST1 group were higher than those in the control group, while the profiles of N-cadherin and Snail were completely opposite (P < 0.05, and ). Thus, FAST1 served as an oncogene in vivo, promoting tumor growth and EMT.
Figure 4 FAST1 promoted the growth of RC cells in vivo. The 769-P cell lines stably transfected with FAST1 overexpression plasmids were injected subcutaneously into the experimental nude mice. (A–C) Tumor size was recorded, tumor weight was measured, and the growth curve and weight histogram were plotted. (D and E) IHC was performed to detect FAST1 and E-cadherin expression in the tumor tissues. (F) WB was employed to examine the expression of E-cadherin, Claudin1, N-cadherin and Snail in tumor tissues. *P < 0.05, **P < 0.01, ***P < 0.001 (vs NC group). N=5.
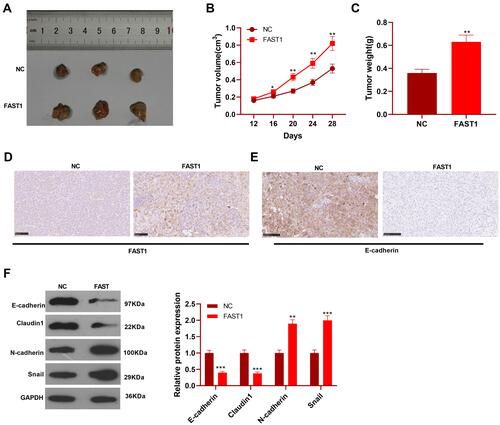
FAST1 Induced the TGF-β/Smad2 Signaling Activation
To clarify the specific mechanism of the carcinogenic function of FAST1 in RC, we investigated its downstream TGF-β/Smad2 pathway. The levels of TGF-β, Smad2 in RC cells were determined by WB, and it was discovered that their expression was higher in the FAST1 overexpression group than that in the control group. In contrast, knocking down FAST1 inhibited TGF-β and p-Smad2 levels (P < 0.05, and ). Interestingly, TGF-β and p-Smad2 levels in the xenograft tumors were also increased with FAST1 up-regulation ( and ). Additionally, we predicted the protein-protein interaction network of FAST1 and the downstream protein pathways through STRING (https://string-db.org/cgi/input.pl). The analysis result showed that FOXH1 functions as a transcriptional activator, recognizes and binds to the DNA sequence 5ʹ-TGT[GT][GT]ATT-3ʹ, is required for induction of the goosecoid (GSC) promoter by TGF-beta or activin signaling. Moreover, FOXH1 forms a transcriptionally active complex containing FOXH1/SMAD2/SMAD4 on a site on the GSC promoter called TARE (TGF-beta/activin response element) (). Moreover, FAST1 has potential function in regulating the TGF-beta signaling pathway (). To confirm the binding relationship between SMAD2 and FAST1, we conducted co-immunoprecipitation, the results showed that FAST1 formed a complex with the Smad2 (), suggesting that there was crosstalk between the FAST1 and Smad2. Overall, these results testified that FAST1 activated TGF-β/Smad2 pathway by binding with SMAD2.
Figure 5 FAST1 activated TGF-β/Smad. The 789-P cell lines stably transfected with overexpressing FAST1, ACHN cell lines stably transfected with knocking down FAST1, and mouse tumors obtained by tumor formation experiment in nude mice were selected. (A–C) WB was employed to examine the expression of TGF-β and p-Smad2/Smad2 in 789-P or ACHN cells or in vivo tumor tissues. (D) IHC was performed to detect p-Smad2 expression in the tumor tissues. (E) STRING (https://string-db.org/cgi/input.pl) showed the protein protein interaction (PPI) network of FAST1. (F) The downstream protein pathways of FAST1 were predicted through STRING (https://string-db.org/cgi/input.pl), TGF-β signaling pathway was potential pathways underlying FAST1. (G) Co-immunoprecipitation was used to confirm the interaction between FAST1 and Smad2 in 789-P cells. **P < 0.01, ***P < 0.001 (vs NC group); *P < 0.05, **P < 0.01, ***P < 0.001 (vs Si-NC group). N=5.
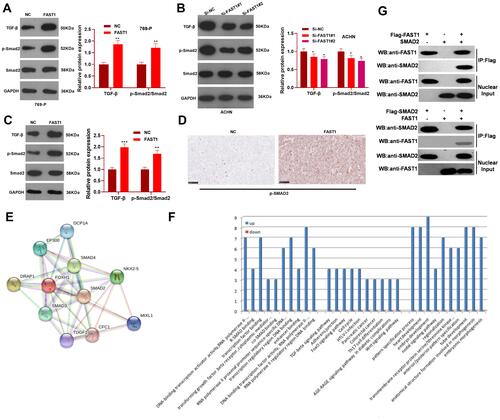
Inhibiting TGF-β Attenuated Malignant Biological Behaviors of RC Induced by Overexpressing FAST1
We further investigated whether TGF-β affected the effect of FAST1 on RC. The TGF-β inhibitor (RepSox) was added on the basis of FAST1 overexpression, and the FAST1 level was monitored by qRT-PCR. Interestingly, the FAST1 expression was higher after overexpressing FAST1 (vs the control group), and its level was lower in the FAST1+RepSox group than that in the FAST1 group (P < 0.05, ). Next, the cell viability, apoptosis, migration and invasion were measured by colony formation, flow cytometry, CCK8 and Transwell assay, respectively. Interestingly, the FAST1+RepSox group had eliminated cell proliferation, migration and invasion, and had enhanced apoptosis compared with FAST1 group (P < 0.05, ). Additionally, WB results manifested that FAST1+RepSox inhibited the EMT induced by overexpressing FAST1, indicated by up-regulated E-cadherin and Claudin1 and down-regulated N-cadherin and Snail (). At the same time, FAST1+RepSox attenuated the activation of FAST1 on the TGF-β/Smad2 signaling pathway. Namely, TGF-β and p-Smad2/Smad2 were down-regulated (P < 0.05, ). Moreover, we treated 769-P cells with TGF-β. The result of Western blot showed that TGF-β markedly enhanced p-Smad2 level, and also promoted FAST1 expression (). The above results proved that inhibiting TGF-β reduced the oncogenic effect of FAST1 in RC.
Figure 6 Inhibition of TGF-β attenuated the malignant biological behaviors of RC cells induced by overexpressing FAST1. The 789-P cell lines were transfected with FAST1 overexpression plasmids, and the TGF-β inhibitor (RepSox, 2 µmol/L) was added. (A) The FAST1 expression was tested by qRT-PCR. (B–F) Cell colony formation assay, CCK8, flow cytometry and Transwell assay were adopted to monitor the viability, apoptosis, proliferation, migration and invasion of cells in the two groups. **P<0.01 vs vector group, &P<0.05, &&P<0.01 vs FAST1 group. N=3. (G and H) WB was implemented to compare the expression of EMT-related markers and TGF-β/Smad2 pathway. (I) 789-P cells were treated with TGF-β (10 ng/mL) for 24 hours, then WB was conducted to detect Smad2 and FAST1 level. *P<0.05, **P<0.01, ***P< 0.001. N=3.
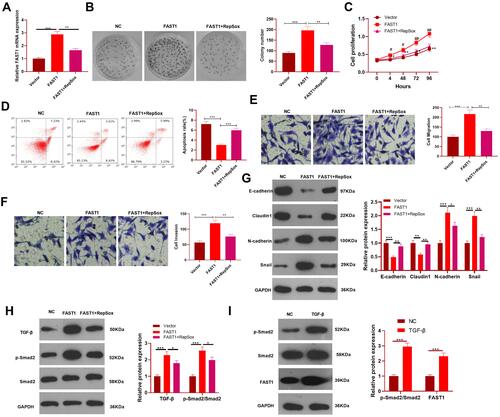
Discussion
RCC is the most common type of RC with a high degree of malignancy. According to statistics, there were about 627,000 new RCC cases in the United States in 2016, and the current incidence is still on the rise.Citation14 Although the popularity of new technologies has improved the diagnostic rate of RC to some extent, the specific markers related to RC are still lacking. In this study, we found the potential of FAST1 as a biomarker for RC diagnosis and prognosis. Namely, FAST1 is highly expressed in RC tissues and is related to patients’ adverse prognosis, and knocking down FAST1 inhibits the RC evolvement.
Earlier studies have revealed that Forkhead box transcription factors can regulate cell proliferation, apoptosis, migration, metabolism and DNA damage response.Citation15 For example, Forkhead Box M1 (FOXM1), which is up-regulated in malignant meningioma tissues, facilitates meningioma cell viability, proliferation, tube formation and inhibits apoptosis by repressing AHR, CYP1A1 and VEGFA.Citation16 Interestingly, several Forkhead box transcription factors such as FOXM1Citation17 and FOXA1Citation18 are found to induce oncogenic effects in RC, while the other Forkhead box transcription factor members, including FOXO3,Citation19 FOXL1Citation20 and FOXO1,Citation21 show potent anti-tumor effects by mitigating the proliferation and invasion of RC cells. As a novel member of the Forkhead box transcription factor family, FOXH1 has been revealed to modulate the malignant behaviors of tumor cells.Citation5,Citation6 Recently, Liu Y et al reported that FOXH1 significantly promotes cell proliferation, invasion and tumorigenesis of breast cancer cells. Benzyl isothiocyanate (BITC), an anti-tumor reagent in breast cancer, can exert a magnified tumor-suppressive effect when FOXH1 is down-regulated.Citation22 In the present study, FAST1 was found significantly up-regulated in RC and associated with poorer survival or RC patients. Therefore, it is reasonable to speculate that FAST1 may serve as an oncogene in RC. Our experimental results confirmed that forced FAST1 overexpression promotes RC cell proliferation, viability, invasion, migration, and inhibits apoptosis rate. Therefore, FAST1 is a potential biological target for RC diagnosis and treatment.
EMT refers to the process that epithelial cells lose the apical-basal polarity and cell–cell adhesion and transit to invasive mesenchymal cells.Citation23 In terms of its functions, EMT plays a vital role in modulating embryonic development, wound healing, cancer cell metastasis, and drug resistance.Citation24–Citation27 It is worth noting that in non-small cell lung cancer (NSCLC), UBE2T enhances the degradation of FOXO1 in a ubiquitination-dependent manner, thus repressing EMT of NSCLC cells.Citation28 Similarly, this study found that FAST1 up-regulation led to up-regulated N-cadherin and Snail but inhibited E-cadherin and Claudin1, indicating FAST1 promoted the EMT of RC cells. Moreover, down-regulation of FAST1 led to the reduced potential of EMT in RC. As a result, we concluded that FAST1 aggravated the migration and invasion by promoting EMT of RC cells.
A previous study has found that FAST1 can interact with the Smad2 pathway, thereby mediating biological processes.Citation29 TGF-β is a highly effective cytokine that contributes to maintaining cell homeostasis, embryonic development, and cell differentiation. It is considered an effective cell growth inhibitor. Above all, tumor cells can antagonize the inhibition of TGF-β in advanced stages, resulting in the conversion of TGF-β into cancer promoters.Citation8 It has been found in the literature that TGF-β functions through its upstream and downstream molecular interactions, and Smad is one of the classic TGF-β dependent proteins. When TGF-β ligand binds to TGF-β1 and TGF-β2 receptors, TGF-β1 and TGF-β2 form a heterologous complex, which directly activates Smad2 and Smad3. While Smad2, 3 and 4 form trimers and transfer to the nucleus, regulating the target gene transcription through synergistic action.Citation30,Citation31 The TGF-β/Smad signal is involved in the development of multiple malignancies. For instance, Zhang et al showed that overexpressed SALT4 promotes the metastasis of gastric cancer by activating the TGF-β/Smad signaling pathway.Citation32 Also, Gao et al claimed that Topoisomerase II (TOP2A) aggravates the progression of advanced ovarian cancer by activating TGF-β/Smad,Citation33 suggesting that inhibiting the TGF-β/Smad signaling pathway may be a novel method for cancer treatment. Some scholars have found that the activation of down-regulated miR-542-3p on the TGF-β/Smad signaling pathway mediates hepatocellular carcinoma progression.Citation34 In addition, Dong et al reported that the MMP-9 inhibitor effectively blocked the activation of TGF-β/Smad, thus inhibiting the invasion of breast cancer.Citation35 Fortunately, TGF-β/Smads activation is demonstrated to play an oncogenic role in RCC.Citation36 In our study, we found that FAST1 positively regulates the TGF-β/SMAD pathway. Importantly, the TGF-β inhibition partly reversed the FAST1-mediated promoting effect in RC. Specifically, FAST1 potentials RC development dependently through the TGF-β pathway.
Overall, FAST1 is up-regulated in RC and also promotes the progression of RC. This pro-oncogenic effect of FAST1 in RC is closely related to the activation of its downstream TGF-β/Smad signaling pathway. Nevertheless, the sample size involved in this paper is small and cannot represent a large range of the population. It is necessary to further expand the sample source and diversity to testify its applicability in clinical practice.
Data Sharing Statement
The data sets used and analyzed during the current study are available from the corresponding author on reasonable request.
Ethics Statement
Our study was approved by the Ethics Review Board of Zaozhuang Municipal Hospital.
Author Contributions
All authors contributed to data analysis, drafting or revising the article, have agreed on the journal to which the article will be submitted, gave final approval of the version to be published, and agree to be accountable for all aspects of the work.
Disclosure
The authors report no conflicts of interest in this work.
Additional information
Funding
References
- Wang AB, Bao Y, Wu ZJ, et al. Long noncoding RNA EGFR-AS1 promotes cell growth and metastasis via affecting HuR mediated mRNA stability of EGFR in renal cancer. Cell Death Dis. 2019;10(3):154. doi:10.1038/s41419-019-1331-9
- Liu XB, Hao YX, Yu W, et al. Long non-coding RNA emergence during renal cell carcinoma tumorigenesis. Cell Physiol Biochem. 2018;47(2):735–746. doi:10.1159/000490026
- Wang L, Su Y, Huang C, et al. FOXH1 is regulated by NANOG and LIN28 for early-stage reprogramming. Sci Rep. 2019;9(1):16443. doi:10.1038/s41598-019-52861-8
- Roessler E, Ouspenskaia MV, Karkera JD, et al. Reduced NODAL signaling strength via mutation of several pathway members, including FOXH1, is linked to human heart defects and holoprosencephaly. Am J Hum Genet. 2008;83(1):18–29. doi:10.1016/j.ajhg.2008.05.012
- Cheng Q, Shi YJ, Li Z, Kang H, Xiang Z, Kong LF. FAST1 promotes the migration and invasion of colorectal cancer cells. Biochem Biophys Res Commun. 2019;509(2):407–413. doi:10.1016/j.bbrc.2018.12.122
- Huang CX, Chen N, Wu XJ, et al. Zebrafish let-7b acts downstream of hypoxia-inducible factor-1α to assist in hypoxia-mediated cell proliferation and cell cycle regulation. Life Sci. 2017;171:21–29. doi:10.1016/j.lfs.2017.01.005
- Connolly EC, Freimuth J, Akhurst RJ. Complexities of TGF-β targeted cancer therapy. Int J Biol Sci. 2012;8(7):964–978. doi:10.7150/ijbs.4564
- Oshimori N, Oristian D, Fuchs E. TGF-β promotes heterogeneity and drug resistance in squamous cell carcinoma. Cell. 2015;160(5):963–976. doi:10.1016/j.cell.2015.01.043
- Massagué J, Seoane J, Wotton D, et al. Smad transcription factors. Genes Dev. 2005;19(23):2783–2810. doi:10.1101/gad.1350705
- Rouce RH, Shaim H, Sekine T, et al. The TGF-β/SMAD pathway is an important mechanism for NK cell immune evasion in childhood B-acute lymphoblastic leukemia. Leukemia. 2016;30(4):800–811. doi:10.1038/leu.2015.327
- Liu LJ, Yu JJ, Xu XL. MicroRNA-93 inhibits apoptosis and promotes proliferation, invasion and migration of renal cell carcinoma ACHN cells via the TGF-β/Smad signaling pathway by targeting RUNX3. Am J Transl Res. 2017;9(7):3499–3513.
- Weisberg E, Winnier GE, Chen X, Farnsworth CL, Hogan BL, Whitman M. A mouse homologue of FAST-1 transduces TGF beta superfamily signals and is expressed during early embryogenesis. Mech Dev. 1998;79(1–2):17–27. doi:10.1016/S0925-4773(98)00160-9
- Franceschini A, Szklarczyk D, Frankild S, et al. STRING v9.1: protein-protein interaction networks, with increased coverage and integration. Nucleic Acids Res. 2013;41(Databaseissue):D808–D815. doi:10.1093/nar/gks1094
- Siegel RL, Miller KD, Jemal A. Cancer statistics, 2016. CA Cancer J Clin. 2016;66(1):7–30. doi:10.3322/caac.21332
- Zhu H. Forkhead box transcription factors in embryonic heart development and congenital heart disease. Life Sci. 2016;144:194–201. doi:10.1016/j.lfs.2015.12.001
- Wu Q, Zheng J, Dou C, Qi S. Forkhead box M1 promotes the growth and tube formation of human malignant meningioma cells via the aryl hydrocarbon receptor signaling pathway. Folia Neuropathol. 2020;58(3):223–236. doi:10.5114/fn.2020.100065
- Liu F, Li N, Liu Y, Zhang J, Zhang J, Wang Z. Homeodomain interacting protein kinase-2 phosphorylates FOXM1 and promotes FOXM1-mediated tumor growth in renal cell carcinoma. J Cell Biochem. 2019;120(6):10391–10401. doi:10.1002/jcb.28323
- Tong Z, Meng X, Wang J, Wang L. MicroRNA-212 inhibits the proliferation and invasion of human renal cell carcinoma by targeting FOXA1. Mol Med Rep. 2018;17(1):1361–1367. doi:10.3892/mmr.2017.7956
- Nie W, Ni D, Ma X, et al. miR-122 promotes proliferation and invasion of clear cell renal cell carcinoma by suppressing Forkhead box O3 [published correction appears. Int J Oncol. 2019;54(2):559–571. doi:10.3892/ijo.2018.4636
- Yang FQ, Yang FP, Li W, et al. Foxl1 inhibits tumor invasion and predicts outcome in human renal cancer. Int J Clin Exp Pathol. 2013;7(1):110–122.
- Zhou L, Yin B, Liu Y, Hong Y, Zhang C, Fan J. Mechanism and function of decreased FOXO1 in renal cell carcinoma. J Surg Oncol. 2012;105(8):841–847. doi:10.1002/jso.23013
- Liu Y, Zhang L, Meng Y, Huang L. Benzyl isothiocyanate inhibits breast cancer cell tumorigenesis via repression of the FoxH1-mediated Wnt/β-catenin pathway. Int J Clin Exp Med. 2015;8(10):17601–17611.
- Zhao Y, Ye X, Chen R, et al. Sirtuin 7 promotes non-small cell lung cancer progression by facilitating G1/S phase and epithelial-mesenchymal transition and activating AKT and ERK1/2 signaling. Oncol Rep. 2020;44(3):959–972. doi:10.3892/or.2020.7672
- Du B, Shim JS. Targeting epithelial-mesenchymal transition (EMT) to overcome drug resistance in cancer. Molecules. 2016;21(7):965. doi:10.3390/molecules21070965
- Dong J, Zhai B, Sun W, Hu F, Cheng H, Xu J. Activation of phosphatidylinositol 3-kinase/AKT/snail signaling pathway contributes to epithelial-mesenchymal transition-induced multi-drug resistance to sorafenib in hepatocellular carcinoma cells. PLoS One. 2017;12(9):e0185088. doi:10.1371/journal.pone.0185088
- Navas T, Pfister TD, Colantonio S, et al. Novel antibody reagents for characterization of drug- and tumor microenvironment-induced changes in epithelial-mesenchymal transition and cancer stem cells. PLoS One. 2018;13(6):e0199361. doi:10.1371/journal.pone.0199361
- Wang H, Zhang B, Wang X, et al. TRPV4 overexpression promotes metastasis through epithelial-mesenchymal transition in gastric cancer and correlates with poor prognosis. Onco Targets Ther. 2020;13:8383–8394. doi:10.2147/OTT.S256918
- Yin H, Wang X, Zhang X, et al. UBE2T promotes radiation resistance in non-small cell lung cancer via inducing epithelial-mesenchymal transition and the ubiquitination-mediated FOXO1 degradation. Cancer Lett. 2020;494:121–131. doi:10.1016/j.canlet.2020.06.005
- Noda D, Itoh S, Watanabe Y, et al. ELAC2, a putative prostate cancer susceptibility gene product, potentiates TGF-beta/Smad-induced growth arrest of prostate cells. Oncogene. 2006;25(41):5591–5600. doi:10.1038/sj.onc.1209571
- Yoshimatsu Y, Wakabayashi I, Kimuro S, et al. TNF-α enhances TGF-β-induced endothelial-to-mesenchymal transition via TGF-β signal augmentation. Cancer Sci. 2020;111(7):2385–2399. doi:10.1111/cas.14455
- Nakao A, Imamura T, Souchelnytskyi S, et al. TGF-beta receptor-mediated signalling through Smad2, Smad3 and Smad4. EMBO J. 1997;16(17):5353–5362. doi:10.1093/emboj/16.17.5353
- Zhang X, Zhang P, Shao M, et al. SALL4 activates TGF-β/SMAD signaling pathway to induce EMT and promote gastric cancer metastasis. Cancer Manag Res. 2018;10:4459–4470. doi:10.2147/CMAR.S177373
- Gao Y, Zhao HY, Ren M, et al. TOP2A promotes tumorigenesis of high-grade serous ovarian cancer by regulating the TGF-β/Smad pathway. J Cancer. 2020;11(14):4181–4192. doi:10.7150/jca.42736
- Zhang T, Liu W, Meng W, et al. down-regulation of miR-542-3p promotes cancer metastasis through activating TGF-β/Smad signaling in hepatocellular carcinoma. Onco Targets Ther. 2018;11:1929–1939. doi:10.2147/OTT.S154416
- Dong HD, Diao HX, Zhao Y, et al. Overexpression of matrix metalloproteinase-9 in breast cancer cell lines remarkably increases the cell malignancy largely via activation of transforming growth factor-beta/SMAD signalling. Cell Prolif. 2019;52(5):e12633. doi:10.1111/cpr.12633
- Tretbar S, Krausbeck P, Müller A, et al. TGF-β inducible epithelial-to-mesenchymal transition in renal cell carcinoma. Oncotarget. 2019;10(15):1507–1524. doi:10.18632/oncotarget.26682