Abstract
The optimal route for clinical delivery of oncolytic viruses is thought to be systemic intravenous injection; however, the immune system is armed with several highly efficient mechanisms to remove pathogens from the circulatory system. To overcome the challenges faced in trying to delivery oncolytic viruses specifically to tumors via the bloodstream, carrier cells have been investigated to determine their suitability as delivery vehicles for systemic administration of oncolytic viruses. Cell carriers protect viruses from neutralization, one of the most limiting aspects of oncolytic virus interaction with the immune system. Cell carriers can also possess inherent tumor tropism, thus directing the delivery of the virus more specifically to a tumor. With preclinical studies already demonstrating the success and feasibility of this approach with multiple oncolytic viruses, clinical evaluation of cell-mediated delivery of viruses is on the horizon. Meanwhile, ongoing preclinical studies are aimed at identifying new cellular vehicles for oncolytic viruses and improving current promising cell carrier platforms.
Introduction
Oncolytic viruses infect and kill tumor cells while leaving normal tissues unharmed. Specificity toward cancer cells can be a natural feature of the virus, as is the case with reovirus, Newcastle disease virus, and mumps virus, or it can be selected for or engineered into the virus through the use of tumor-specific cell surface molecules,Citation1 transcription factors,Citation2 and tissue-specific microRNAs.Citation3 Similarly, vesicular stomatitis virus, herpes simplex virus, and adenovirus have been genetically attenuated by subduing their ability to antagonize antiviral defenses, thus improving tumor specificity. This strategy leads to enhanced replication in tumor cells, which often possess defects in antiviral pathways,Citation4 while sparing normal cells. Oncolytic viruses exert their antitumor activities through both direct and indirect mechanisms. Direct infection of tumor cells leads to virus and immune-mediated cytotoxicity, and in some cases, alerting the immune system to the previously tolerated tumor through the recruitment of natural killer (NK) cells and cluster of differentiation (CD)8+ cytotoxic T cells.Citation5 Infection of tumor vasculature can lead to vascular collapse and compromised blood flow within the tumor, thus choking off its access to nutrients.Citation6 To increase potency, oncolytic viruses have been engineered to express genes that augment virus replication,Citation7 induce cytotoxicity,Citation8 promote bystander cell killing,Citation9 and enhance antitumor immunity.Citation5 Of overarching concern, however, is that these numerous improvements will provide no benefit to antitumor efficacy unless the virus is successfully delivered to the tumor. Although direct intratumoral injection should deliver all virus particles directly to the tumor, there are several advantages to systemic administration. Firstly, not all tumors are amenable to direct intratumoral injection since they may consist of several small nodules spread out over a large area, or they are in an anatomic location that is inaccessible by direct injection. Systemic delivery has a greater chance of reaching disseminated metastases as well as the primary tumor. Although not thoroughly investigated, the ability of some oncolytic viruses to stimulate antitumor immune responses may be greater when administered systemically.Citation10
Despite the need for efficient systemic delivery of oncolytic viruses, there exist several barriers to their efficient systemic delivery (). The immune system has evolved several mechanisms to prevent the systemic spread of microorganisms and does not discriminate between pathogens and therapeutic oncolytic viruses. Intravenous delivery exposes oncolytic viruses to circulating factors such as antibodies, which bind to and neutralize virus directly or mark them for destruction by complement and various immune cells.Citation11 Virus is also neutralized by nonspecific binding to serum proteins and circulating cells present in the bloodstream.Citation11 Organs such as the lung, spleen, and especially the liver, also play a significant role in clearing virus from the bloodstream because these tissues contain resident macrophages, the role of which is to scavenge the blood for circulating pathogens.Citation12 Because these mechanisms are present in virus-naïve individuals as part of the innate immune system, the neutralization of oncolytic viruses is even greater if previous exposure to the virus has occurred. Since adaptive immunity is able to mount a significantly more specific and potent immune response compared with its innate counterpart, this represents an additional and significant hurdle to an efficacious repeat dosing regimen using oncolytic viruses. Indeed, a significant proportion of the human population has already been exposed to and thus has developed immunologic memory to many of the oncolytic viruses currently undergoing clinical development and testing, including reovirus,Citation13 vaccinia virus,Citation14 and measles virus.Citation15 Apart from neutralization of virus in the bloodstream, physical barriers such as tumor extracellular matrix, as well as limited extravasation of oncolytic viruses into the tumor bed due to high interstitial fluid pressure within the tumor, can also result in fewer virions being delivered to tumor cells.Citation16 Despite all these existing barriers to systemic delivery of oncolytic viruses, recent clinical trialsCitation17,Citation18 suggest that intravenously administered virus can reach the tumor and replicate if administered at sufficiently high doses, presumably saturating the neutralizing mechanisms present within the human body. Although this was a milestone achievement in the field, there is still much room for improvement as several sets of preclinicalCitation19–Citation21 and clinicalCitation13,Citation18 data demonstrate that pre-existing, virus-specific neutralizing antibodies dramatically reduce the amount of infectious virus that can be delivered to the tumor.
Figure 1 Virus neutralization during systemic delivery. (A) Circulating antibodies and (B) complement proteins bind to virus and neutralize them, as well as marking them for destruction by immune effector cells. (C) Intravenously administered virus also interacts with circulating blood cells, leading to virus sequestration. (D) Liver macrophages, which are part of the reticulo-endothelial system, filter viruses from the blood. (E) Viruses that do reach the tumor encounter extensive tumor extracellular matrix and high interstitial fluid pressure which limits their extravasation into the tumor.
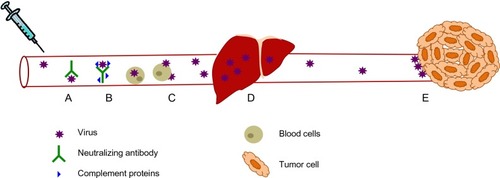
Cell carriers: playing virus “hide and seek”
To overcome some of the challenges presented in delivering oncolytic viruses systemically, several investigators are exploring the use of cells as delivery vehicles for oncolytic viruses. This approach mimics what some viruses have evolved to do in order to spread systemically or gain access to various tissues within their host. For example, the human immunodeficiency virus is known to bind to circulating dendritic cells and macrophages which then naturally migrate to lymph nodes and pass on the virus to its target cell population, ie, CD4+ T cells.Citation22 Some viruses that replicate via cell-to-cell spread are also able to evade neutralization by circulating antibodies,Citation23 a feature that would clearly be of benefit for the systemic delivery of oncolytic viruses. The utility of cells as delivery vehicles for oncolytic viruses has therefore been investigated for several oncolytic viruses using a variety of cell carrier types and different tumor models in numerous preclinical studies. Notably, in recent clinical trials,Citation17,Citation18 intravenously administered virus was found to bind several types of circulating cells, and in the case of reovirus, the cell-bound virus was still infectious and could deliver virus to tumor cells in vitro despite the presence of neutralizing antibodies in patients.Citation18
Based on the preclinical studies completed so far, most of the cell types that have been studied as delivery vehicles for oncolytic viruses fall under one of the following three categories: transformed cells; immune cells; and progenitor cells. Each of these cell types has its unique advantages and potential disadvantages. In theory, the ideal cell carrier would not only protect its viral cargo from neutralization and direct it specifically to the location of the tumor, thus limiting toxicity to normal tissues, but the cell carrier would also have antitumor activity of its own. Additional properties, such as a favorable safety profile, ease of isolation, and/or manufacturing, are important features to consider when deciding what carrier cell type is most suitable for clinical application. In this review, we aim to summarize the promising research that has been accomplished thus far using carrier cells for efficient delivery of viral therapeutics and what lies ahead in terms of bringing this approach to a clinical setting for evaluation in patients.
Virus protection
There are several preclinical studies demonstrating that carrier cells can deliver a variety of oncolytic viruses to tumors. Indeed, successful delivery in vivo has been achieved with several oncolytic virus platforms using a wide range of carrier cell types (). Clearly this approach is not limited to a single virus platform or to a single carrier cell type. In choosing a suitable cell carrier for the delivery of oncolytic viruses, one must consider the susceptibility of the cell to the virus, the kinetics of viral replication and release, as well as the kinetics of trafficking of the cell carrier from the site of injection to the tumor location. All of these parameters must be optimized to ensure that virus remains unseen by the immune system and therefore protected from neutralization. After a virus enters a cell, there is a period of time before progeny virions are released from the infected cell. Ideally, the cell carrier should reach the tumor during this stage of virus replication. For some rapidly replicating viruses, such as vesicular stomatitis virus, this eclipse phase can be as short as an hour,Citation24 whereas for slower replicating viruses, such as vaccinia, this period of time is much longer.Citation25 Therefore, with slower replicating viruses, there is more flexibility in optimizing the timing of infection and delivery of the carrier cell. For example, with vesicular stomatitis virus, we found that successful delivery could be achieved in the presence of virus-specific neutralizing antibodies using tumor cell carriers if the cells were injected after being infected for only 1–2 hours.Citation19 On the other hand, studies performed by Thorne et al demonstrated that cytokine-induced killer (CIK) cells took 72 hours to traffic to tumors and this was compatible with vaccinia virus replication kinetics in CIK cells in which peak virus release also occurred at 72 hours post-infection.Citation26 From these studies and several others, it is clear that proper characterization of virus replication in the cell carrier of choice and subsequent optimization is important in achieving successful delivery to tumors.
Table 1 Cell carriers used to deliver virus vectors
It is important to note that even before progeny virions are released from an infected cell, viral proteins can accumulate at the surface of the infected cell either as membrane-bound proteins, as is the case with vesicular stomatitis virus G protein, or in complexes with host cell surface proteins such as major histocompatibility complex molecules which present intracellular peptides to immune cells. This raises the possibility that extracellular viral antigens present on the cell carrier can make it a target for immune-mediated destruction, thus negatively impacting successful delivery. Because of the premature clearing of infected cell carriers as a result of viral antigens being exposed on the cell surface, some investigators have attempted to regulate virus replication in the carrier cell such that virus replication and release is initiated once the carrier cells localize to the tumor. One such study by Muthana et alCitation27 used an adenovirus/macrophage cell carrier system to selectively target prostate cancer. Macrophages which have a natural propensity to migrate to hypoxic areas of tumor tissue, were infected with a replication-deficient adenovirus carrying a therapeutic transgene controlled by prostate-specific promoters, and then transfected with a hypoxia-driven E1A/B plasmid. Upon migration of these cotransduced macrophages into hypoxic areas of prostate tumors, hypoxic response element-driven expression of E1A/B initiated viral replication and release, thus allowing the adenovirus to infect prostate cancer cells and express the therapeutic transgene. A similar approach used human mesenchymal stem cells (MSCs) to deliver conditionally replicating adenovirus under the control of osteocalcin.Citation28 This delivery system relied on the observation that MSCs normally express low levels of osteocalcin but that its expression can be highly induced by vitamin D3. Thus, it is possible to deliver the carrier cell and wait until it reaches the tumor, at which point virus replication can be induced by intraperitoneal injection of vitamin D3. In both of these approaches, the end result was that virus release occurred only once the carrier cell reached the tumor, thus potentially minimizing neutralization of the virus in the bloodstream and limiting off-target toxicities.
The use of a cell carrier that supports viral replication is an attractive approach because it has the added advantage of en route dose amplification as the cell carrier produces virus and makes its way to the tumor. Given that for some viruses, the current doses used in clinical trials are at their maximum due to limits in production of the clinical grade virus stocks, a cell carrier with the capacity to produce hundreds of virus particles per cell can dramatically increase the total amount of therapeutic virus that is delivered to the tumor and increase the likeliness of overcoming neutralizing mechanisms. Because oncolytic viruses are selected or engineered to replicate poorly in normal cells, transformed cell carriers are more likely to be permissive to virus replication and produce more virus particles per cell.Citation29 While the use of transformed cells as carriers does not come without safety concerns, it has been demonstrated that irradiation of the cell before administration can halt its ability to grow, and thus its tumorigenic potential, while still maintaining virus infectivity.Citation30,Citation31 Finally, when using a replicating virus in a permissive carrier cell, one must consider the impact of virus replication on carrier cell viability, trafficking, and effector functions because these may be altered as a consequence of infection.
Although considered an advantage, amplification of the virus by the carrier cell is not absolutely necessary for successful protection and delivery to tumors, as exemplified by tumor antigen-specific T cell carriers. It was demonstrated that vesicular stomatitis virus,Citation32 reovirus,Citation20 herpes simplex virus,Citation33 Newcastle disease virus,Citation34 and retrovirusCitation35 particles could “hitchhike” on the surface of T cells and could be delivered to tumor cells through various mechanisms, either passively or involving cellular synapses between the carrier cell and the tumor cell. With retrovirus particles, it was found that the virus was handed off either passively or via intracellular perforin-containing cytotoxic granules released from activated T cells when they engaged their target cells.Citation36 Importantly, surface-adsorbed virus was protected from neutralizing antibodies. With vesicular stomatitis virus-loading of T cells,Citation32 it was observed that at a high multiplicity of infection, the T cells could not deliver virus to tumors in passively immunized mice but that this could be achieved if loaded at a low multiplicity of infection. This suggests that at high multiplicities of infection, surface-bound virus was still accessible to neutralizing antibodies, thus emphasizing the importance of optimizing infection conditions when preparing cell carriers.
T cells are not the only cell type capable of protecting and delivering surface-adhered virus to tumor cells. A study by Ilett et alCitation20 compared delivery of reovirus using either murine T cells, immature dendritic cells, or mature dendritic cells, and found that mature dendritic cells were superior at delivering virus to tumors in immunized mice, a finding that can perhaps be explained in part by the observation that mature dendritic cells trafficked to tumors in greater numbers than T cells. In contrast, immature dendritic cells were not able to deliver reovirus to tumors in immunized mice, leading the authors to speculate that the high level of reovirus replication in this cell type marked it for immune destruction. In support of this hypothesis, in a follow-up studyCitation37 performed in vitro with human T cells and dendritic cells, both immature and mature dendritic cells were able to protect reovirus from immune serum whereas T cells could not. Moreover, in these studies, both mature and immature dendritic cells supported low levels of reovirus replication and thus were not neutralized by immune serum, whereas the T cells failed to internalize the virus, leaving the authors to hypothesize that the virus was exposed and thus vulnerable to neutralization. In summary, future studies aimed at better understanding of the mechanisms by which viruses bind to and enter cells will most certainly shed some light on how we can take advantage of these complex interactions between viruses and carrier cells to further enhance the systemic delivery of oncolytic viruses.
Tumor-specific delivery
Virus protection, as discussed above, is not the only benefit of using carrier cells for the delivery of oncolytic viruses. Indeed, a protected virus is not useful unless it is delivered specifically to the site of the tumor. For this reason, there has been a lot of effort invested in identifying cell types that not only protect viruses during systemic delivery, but also have the ability to traffic to tumors. This section briefly reviews the current knowledge regarding promising tumor-targeted cellular vehicles for oncolytic virus delivery.
Transformed cells
Transformed cells were among the first cell carriers tested for oncolytic virus delivery to tumors.Citation31,Citation38 They are relatively easy to grow to sufficiently large numbers and are more readily infected with oncolytic viruses than normal cells, and are thus capable of delivering large payloads of virus to tumors. However, their ability to home to specific body and tumor locations is rather limited. In fact, we have observed that cancer cells that form solid tumors (HeLa cervical carcinoma, A549 lung carcinoma, MCF-7 breast carcinoma, CT26 colorectal carcinoma, and SF268 glioblastoma) tend to arrest in the small capillary beds of the lungs and fail to recirculate when injected intravenously into miceCitation19 (and unpublished data, Bell, 2007). This is most likely due to their large size and the fact that organs such as the lung and liver act as filters. Although these findings suggest that solid tumor cells may be ideal carriers for targeting oncolytic viruses to lung tumors, as demonstrated by Power et al,Citation19 these studies led us to explore the use of transformed cells of hematologic origin, such as leukemia cells, with the rationale that they would circulate much better than solid tumor cells and be able to bypass the lungs for delivery to tumors in anatomic locations other than the lung. Although the L2120 murine leukemia cells used in these studies did transiently accumulate in the lungs, as determined by bioluminescent imaging, they eventually managed to recirculate and thus were able to deliver virus to subcutaneous tumors.Citation19 In this model, delivery to the subcutaneous tumors was most likely by nonspecific accumulation of infected cells within the tumor as opposed to specific homing of the cell carriers to the tumor location. Tumor cells can, however, have the propensity to traffic, or home, to specific organs, as is seen with metastatic disease. The ability of tumor cells to migrate to specific tissues can be exploited to achieve targeted delivery to metastatic tumor beds. For example, myeloma cells, which have been used as cell carriers for delivery of oncolytic measles virus,Citation39 express high levels of CXC chemokine receptor 4 (CXCR4) and thus often metastasize to the bone marrow.Citation40 Therefore, it has been proposed that myeloma cells themselves can be used to achieve delivery of measles virus to metastatic tumor beds in the bone marrow.Citation30,Citation39 Although an “off the shelf” cell carrier is possible when using transformed cells, use of a patient’s own tumor cells as a carrier for oncolytic virus delivery is also attractive from an immunologic standpoint, since infected cell vaccines have been demonstrated to enhance antitumor immune responses.Citation10,Citation41,Citation42 Finally, even though transformed cells have been used in clinical trials,Citation43 proper safety measures must be considered when using any transformed cell line as a cell carrier.
Immune cells
Several immune cell types, including T cells,Citation20,Citation32,Citation34,Citation35 CIK cells,Citation26,Citation44 monocytes/macrophages,Citation45,Citation46 and myeloid-derived suppressor cellsCitation47 have been investigated as carrier cells because they can circulate systemically, have the ability to specifically recognize tumors or tumor-associated features, and possess antitumor activity on their own, therefore delivering a one-two punch to the tumor. Adoptive transfer of tumor antigen-specific T cells has been extensively investigated as a cancer immunotherapy in both preclinical and clinical settings. Since this cell type has direct anticancer effector functions and can also protect and deliver oncolytic viruses, it seems like an ideal combination of cancer therapeutics. In preclinical studies using OT-1 T cells specific for ovalbumin,Citation32 it was found that 5%–14% of adoptively transferred T cells migrated to B16-OVA tumors, which is quite remarkable. However, in this tumor model, all tumor cells, and only tumor cells, express the highly immunogenic ovalbumin antigen and this does not mirror what is seen in human patients, where truly tumor-specific and highly immunogenic tumor antigens are rare.
Adoptively transferred T cells that do not migrate to the tumor are often found in the spleen and lymph nodes, thus having the potential to target any metastatic disease in these locations or conversely leading to off-target toxicities. One advantage of combining adoptively transferred T cells loaded with oncolytic viruses is that the highly proinflammatory nature of a virus infection in the tumor milieu can help to prevent T cell silencing and inactivation as a consequence of the immune suppressive microenvironment of the tumor, which is often seen with adoptive T cell therapy.Citation48 Despite the attractiveness of this approach, its clinical application is challenging and expensive, so the overall feasibility of this approach is limited.
CIK cells, which are a population of CD3+, CD56+ NK-like T cells obtained from human peripheral blood or mouse splenocytes after ex vivo expansion with interferon gamma, CD3-specific antibody, and interleukin-2, are another type of cell carrier possessing direct anticancer functions.Citation49,Citation50 However, unlike tumor antigen-specific T cells, they are not restricted to one single antigen. Rather, they recognize NKG2D ligands, which are often upregulated on tumor cells as a result of stress,Citation51 thus increasing the chances of targeting a larger percentage of tumor cells within the tumor mass. Additionally, it has been demonstrated that expression of the NKG2D ligands, MICA (MHC class I polypeptide-related sequence A) and MICB, within tumors can be upregulated by treatment with histone deacetylase inhibitors, thus increasing the trafficking of CIK cells to tumors.Citation52 These cells are also easier to obtain from patients and expand ex vivo as compared with antigen-specific T cells, so clinical application of this strategy may be a simpler alternative to tumor antigen-specific T cells. However, vaccinia virusCitation26 and measles virusCitation44 are the only oncolytic viruses that have been successfully delivered to tumors by CIK cells, so it remains unknown if this cell carrier will be useful for delivery of other oncolytic virus platforms.
Macrophages are another class of immune cells that have been associated with tumors and tumor stroma, and have the ability to either enhance or inhibit tumor growth depending on their cytokine expression profile. Tumor cells often secrete monocyte chemotactic protein-1, macrophage colony-stimulating factor, and vascular endothelial growth factor, which promote migration of monocytes to the tumor, where they differentiate into tumor-associated macrophages and localize to hypoxic regions within the tumor.Citation53 Because of the natural homing of macrophages to tumors and the ease of clinical translation of this approach, the delivery of oncolytic measlesCitation45 and adenovirusCitation46 has been explored in preclinical studies, with promising results. Interestingly, using a similar approach, a relatively recent study has investigated the use of myeloid-derived suppressor cells as cell carriers for delivery of vesicular stomatitis virus.Citation47 Myeloid-derived suppressor cells are a heterogeneous population of immature myeloid cells critical to the development of tumor-induced immune tolerance.Citation54 However, it was found that infection of this cell population with vesicular stomatitis virus promoted their conversion from an M2 immune suppressive phenotype to an M1-like tumor killing phenotype.Citation47 In summary, with their ability to circulate throughout the body, to specifically recognize and migrate to tumors or tumor stroma, and to have natural antitumor activities, future studies using immune cells as carriers may hopefully lead to clinical testing.
Progenitor cells
Progenitor cells that have been utilized to deliver oncolytic viruses include MSCs, neural stem cells, and vascular progenitor cells, with MSCs being the most extensively studied progenitor cell type. MSCs are multipotent stromal cells that can differentiate into a variety of cell types, including adipocytes, chondrocytes, and osteoblasts.Citation55 They are readily obtainable from various tissues including bone marrow, adipose tissue, the umbilical cord, and peripheral blood, and proliferate fairly rapidly in culture, making it possible to expand to sufficiently large numbers for clinical application.Citation55 MSCs naturally home to areas of inflammation, stress, and tissue injury, and thus the tumor microenvironment, which is often viewed as a wound that never heals, also provides the necessary signals to direct MSCs to traffic to their location. Apart from their ability to migrate to tumors, factors released by MSCs are known to have antitumor properties capable of reducing the proliferation of glioma, melanoma, lung cancer, and breast cancer cells.Citation56 Neural stem cells are another progenitor cell type that has been investigated specifically for intracranial delivery of oncolytic viruses to brain tumors.Citation57–Citation59 These cells are characterized by their ability to differentiate into cells of the nervous system (neurons, astrocytes, or oligodendrocytes) and by their capacity to self-renew.Citation60 Their ability to migrate to tumors resembles that of MSCs; however, it is quite difficult to obtain sufficient numbers of these cells for clinical studies. Advancements in the isolation, propagation, or generation of these cells will immensely benefit future studies. Finally, it has been suggested that endothelial progenitor cells, with their ability to contribute to neovascularization of growing tumors, would also allow for tumor-specific delivery of oncolytic viruses.Citation61 These cells are readily obtainable from peripheral blood and can be expanded in vitro, and a study performed by Jevremovic et al demonstrated that these cells could transfer retroviruses to tumors in mice upon systemic administration.Citation62 Therefore, future studies aimed at determining if endothelial progenitor cells can deliver other oncolytic viruses would further support the use of these cells as carriers for systemic delivery of viruses.
Clinical trials with carrier cells: are we there yet?
Based on the above summary of what has been accomplished by the use of carrier cells for delivery of oncolytic viruses, it seems that there is no shortage of potentially useful cell carriers for protecting and delivering oncolytic viruses specifically to tumors. The challenge now is to apply what has been learned in these preclinical studies and evaluate the safety, feasibility, and efficacy of this approach in human patients. But how do we decide what is the best cell type that will allow for successful delivery of virus to tumor beds? It is likely that different tumor types may require different cell carriers in order to achieve tumor specific delivery and thus preclinical testing of these strategies remains an important and informative exercise. Apart from the observation that the only infectious virus recovered from the blood of patients treated with reovirus was cell-associated,Citation18 suggesting that intravenous delivery of the virus might naturally occur via a cell carrier, no clinical trial has been conducted looking specifically at carrier cell-mediated delivery of oncolytic viruses. However, in a translational study looking at the viability of this approach, Mader et al assessed the feasibility of obtaining MSCs from adipose tissue of patients with ovarian cancer for the delivery of measles virus to ovarian tumors.Citation63 In this study, it was found that MSCs could be obtained and expanded to sufficient numbers for a clinical dose within 14 days. Furthermore, the MSCs supported measles virus infection and were able to migrate to human ovarian cancers both in vitro and in vivo. Interestingly, the MSCs could be infected and subsequently frozen, and upon thawing, still displayed active viral replication and antitumor activity comparable with that of freshly infected cells. Infection of the MSCs with measles expressing a sodium iodide symporter gene allowed for SPECT-CT (single-photon emission computed tomography/computed tomography) imaging of the cells trafficking to tumors.Citation63 Imaging of the cell carriers is invaluable because it will be informative in understanding the exact trafficking kinetics and ultimate fate of the cell carriers in patients and may guide the optimization of clinical protocols for future studies. Importantly, the MSCs did not form tumors in immune compromised mice nor did they increase the growth of ovarian cancer xenografts. From this study, a clinical protocol is proposed whereby certified MSCs will be thawed, mixed with clinical grade virus on the day of treatment, followed by a 5-minute low speed centrifugation to increase loading efficiency, then incubated for 2 hours at 37°C, and finally infused into the patient by a catheter in the peritoneal cavity. Hopefully, the findings from this trial will not only validate the carrier cell approach for protecting and delivering oncolytic viruses, but will pave the way for future trials involving different cell carrier and oncolytic virus combinations. Equally important, careful analysis of the interactions between oncolytic viruses and cells naturally found in the bloodstream may lead to the discovery of new cell carriers for systemic delivery.
Future of cell carriers: from Trojan horses to stealth fighters
As pointed out by Willmon et al, even with tumor-targeted cell carriers, only 10%, at best, of the administered cell carriers actually find their way to the target tissue.Citation64 Although this is a big improvement from having only 0.001% of systemically administered virions reach the tumor, as estimated for intravenous delivery of “naked” vesicular stomatitis virus,Citation65 there is still the potential for improvement. Given the vast number of biological tools available and our growing understanding in the areas of tumor biology and cell migration, it is possible that cell trafficking can be increased through biological engineering of the carrier cell and/or oncolytic virus. In fact, some investigators are already devising strategies that can perhaps be translated to cell carriers. One strategy is to conjugate or express molecules that bind to exposed tumor antigens or to tumor vasculature. Expression of certain adhesion molecules such as integrins and selectins is known to be upregulated on tumor vasculature as a consequence of the inflammatory environment within tumors.Citation66 Tumor vasculature is also an attractive aspect of tumor biology to target since it is a common feature of most cancer types and some oncolytic viruses are already known to infect the tumor vasculature,Citation6,Citation67,Citation68 thus leading to reduced blood flow to the tumor as a result of vascular collapse.Citation65 There are several published reports of investigators who have exploited this phenomenon to target viruses to the tumor vasculature,Citation69–Citation72 and so perhaps similar targeting strategies could be applied to cell carriers. Indeed, chemical conjugationCitation73 and polymer coatingCitation74 of sialyl Lewis X to the surface of MSCs improves their ability to bind to P-selectin in vitro. Similarly, enzymatic conversion of carbohydrate groups on the glycoprotein CD44, which is expressed by MSCs and subsets of T cells, to the sialyl Lewis X epitope enabled CD44 binding to E-selectin.Citation75 Whether these modifications would result in preferential binding of circulating cell carriers to the tumor vasculature remains to be seen.
Magnetic targeting is another attractive approach that can be applicable to several tumor types, as it does not rely on expression of certain molecules by the carrier cell or the tumor. Although it is more commonly utilized to target smaller agents such as drugsCitation76 or liposomesCitation77 to tumors, it has been demonstrated to be feasible to target a wide range of cell types to several tissues for various applications.Citation78–Citation80 Another potential approach to enhance the trafficking of carrier cells to tumors is to modify the tumor microenvironment itself. As pointed out earlier, treatment of ovarian tumors with histone deacetylase inhibitors or radiation led to an increase in the number of tumor-infiltrating CIK cells due to increased NKG2D ligand expression.Citation44,Citation52 Induction of inflammation within the tumor, either via drug treatment or radiation, can lead to increased expression of adhesion molecules, promote leakiness within the tumor vasculature, and induce the release of cytokines, which may enhance carrier cell homing or extravasation from blood vessels and into the tumor.
In addition to increasing the trafficking of cells to tumors, cell carriers and their viral cargoes can be manipulated in order to increase various aspects of cell-mediated delivery of oncolytic viruses, such as loading capacity, virus production, and delivery to tumor cells. It is possible that the oncolytic virus of choice may not enter or replicate in a useful cell carrier that migrates to tumors. If the cell carrier does not express the entry receptor for the virus, viruses can be engineered or pseudotyped to bind to a different receptor that is expressed by the carrier cell. There are several drugs that have been reported to enhance virus replicationCitation81,Citation82 and thus can be used to increase virus output by the carrier cell. In doing so, it will be important to ensure the drugs do not alter the trafficking patterns of the carrier cell. Cell carriers could also be engineered to express immune modulatory molecules, to either suppress the immune response, therefore priming the tumor for virus infection, or to call on the immune system for a secondary wave of attack on the tumor. As our understanding of cell trafficking, tumor biology, and virology increases, next-generation cell carriers have the potential of being transformed from simple delivery tools into sophisticated, mobile biological factories capable of delivering a coordinated multipronged attack on tumors.
Summary
There is a clear need for improving specificity and enhancing systemic delivery of oncolytic viruses for clinical regimens. Cell carriers are a promising delivery vehicle for the protection and tumor-specific delivery of oncolytic virus and could address both these concerns. As the field waits for the first clinical testing of this approach, the search for better cell carriers continues. Future studies aimed at improving the trafficking of cells to tumors and increasing virus production by cell carriers will only add to the therapeutic gains provided by this approach.
Disclosure
The authors report no conflicts of interest in this work.
References
- CattaneoRMiestTShashkovaEVBarryMAReprogrammed viruses as cancer therapeutics: targeted, armed and shieldedNat Rev Microbiol20086752954018552863
- DorerDENettelbeckDMTargeting cancer by transcriptional control in cancer gene therapy and viral oncolysisAdv Drug Deliv Rev2009617–855457119394376
- KellyEJRussellSJMicroRNAs and the regulation of vector tropismMol Ther200917340941619107117
- NaikSRussellSJEngineering oncolytic viruses to exploit tumor specific defects in innate immune signaling pathwaysExpert Opin Biol Ther2009991163117619637971
- MelcherAParatoKRooneyCMBellJCThunder and lightning: immunotherapy and oncolytic viruses collideMol Ther20111961008101621505424
- BreitbachCJDe SilvaNSFallsTJTargeting tumor vasculature with an oncolytic virusMol Ther201119588689421364541
- Le BoeufFBatenchukCVaha-KoskelaMModel-based rational design of an oncolytic virus with improved therapeutic potentialNat Commun20134197423764612
- DoroninKTothKKuppuswamyMWardPTollefsonAEWoldWSTumor-specific, replication-competent adenovirus vectors overexpressing the adenovirus death proteinJ Virol200074136147615510846098
- FreytagSORogulskiKRPaielliDLGilbertJDKimJHA novel three-pronged approach to kill cancer cells selectively: concomitant viral, double suicide gene, and radiotherapyHum Gene Ther199899132313339650617
- BridleBWStephensonKBBoudreauJEPotentiating cancer immunotherapy using an oncolytic virusMol Ther20101881430143920551919
- FergusonMSLemoineNRWangYSystemic delivery of oncolytic viruses: hopes and hurdlesAdv Virol2012201280562922400027
- FisherKStriking out at disseminated metastases: the systemic delivery of oncolytic virusesCurr Opin Mol Ther20068430131316955693
- WhiteCLTwiggerKRVidalLCharacterization of the adaptive and innate immune response to intravenous oncolytic reovirus (dearing type 3) during a phase I clinical trialGene Ther2008151291192018323793
- HeoJReidTRuoLRandomized dose-finding clinical trial of oncolytic immunotherapeutic vaccinia JX-594 in liver cancerNat Med201319332933623396206
- GalanisEHartmannLCClibyWAPhase I trial of intraperitoneal administration of an oncolytic measles virus strain engineered to express carcinoembryonic antigen for recurrent ovarian cancerCancer Res201070387588220103634
- WojtonJKaurBImpact of tumor microenvironment on oncolytic viral therapyCytokine Growth Factor Rev2010212–312713420399700
- BreitbachCJBurkeJJonkerDIntravenous delivery of a multi-mechanistic cancer-targeted oncolytic poxvirus in humansNature201147773629910221886163
- AdairRARoulstoneVScottKJCell carriage, delivery, and selective replication of an oncolytic virus in tumor in patientsSci Transl Med20124138138ra77
- PowerATWangJFallsTJCarrier cell-based delivery of an oncolytic virus circumvents antiviral immunityMol Ther200715112313017164783
- IlettEJPrestwichRJKottkeTDendritic cells and T cells deliver oncolytic reovirus for tumour killing despite pre-existing anti-viral immunityGene Ther200916568969919282847
- IankovIDBlechaczBLiuCInfected cell carriers: a new strategy for systemic delivery of oncolytic measles viruses in cancer virotherapyMol Ther200715111412217164782
- MartinNSattentauQCell-to-cell HIV-1 spread and its implications for immune evasionCurr Opin HIV AIDS20094214314919339954
- SattentauQAvoiding the void: cell-to-cell spread of human virusesNat Rev Microbiol200861181582618923409
- PittmanDSt JohnRCShechmeisterILLatent period of vesicular stomatitis virus in chick embryo cellsNature1965206990122812314286459
- FurnessGYoungnerJSOne-step growth curves for vaccinia virus in cultures of monkey kidney cellsVirology1959938639513825718
- ThorneSHNegrinRSContagCHSynergistic antitumor effects of immune cell-viral biotherapyScience200631157681780178416556847
- MuthanaMGiannoudisAScottSDUse of macrophages to target therapeutic adenovirus to human prostate tumorsCancer Res20117151805181521233334
- HsiaoWCSungSYLiaoCHWuHCHsiehCLVitamin D3- inducible mesenchymal stem cell-based delivery of conditionally replicating adenoviruses effectively targets renal cell carcinoma and inhibits tumor growthMol Pharm2012951396140822480282
- StojdlDFLichtyBDtenOeverBRVSV strains with defects in their ability to shutdown innate immunity are potent systemic anticancer agentsCancer Cell20034426327514585354
- MunguiaAOtaTMiestTRussellSJCell carriers to deliver oncolytic viruses to sites of myeloma tumor growthGene Ther2008151079780618356812
- CoukosGMakrigiannakisAKangEHUse of carrier cells to deliver a replication-selective herpes simplex virus-1 mutant for the intraperitoneal therapy of epithelial ovarian cancerClin Cancer Res1999561523153710389942
- QiaoJWangHKottkeTLoading of oncolytic vesicular stomatitis virus onto antigen-specific T cells enhances the efficacy of adoptive T-cell therapy of tumorsGene Ther200815860461618305577
- KanzakiAKasuyaHYamamuraKAntitumor efficacy of oncolytic herpes simplex virus adsorbed onto antigen-specific lymphocytesCancer Gene Ther201219429229822281756
- PfirschkeCSchirrmacherVCross-infection of tumor cells by contact with T lymphocytes loaded with newcastle disease virusInt J Oncol200934495196219287952
- ColeCQiaoJKottkeTTumor-targeted, systemic delivery of therapeutic viral vectors using hitchhiking on antigen-specific T cellsNat Med200511101073108116170322
- KottkeTQiaoJDiazRMThe perforin-dependent immunological synapse allows T-cell activation-dependent tumor targeting by MLV vector particlesGene Ther200613151166117716625245
- IlettEJBarcenaMErrington-MaisFInternalization of oncolytic reovirus by human dendritic cell carriers protects the virus from neutralizationClin Cancer Res20111792767277621389099
- LambrightESCaparrelliDJAbbasAEOncolytic therapy using a mutant type-1 herpes simplex virus and the role of the immune systemAnn Thorac Surg19996851756176010585055
- LiuCRussellSJPengKWSystemic therapy of disseminated myeloma in passively immunized mice using measles virus-infected cell carriersMol Ther20101861155116420234340
- MollerCStrombergTJuremalmMNilssonKNilssonGExpression and function of chemokine receptors in human multiple myelomaLeukemia200317120321012529679
- ConradDPTsangJMacleanMLeukemia cell-rhabdovirus vaccine: personalized immunotherapy for acute lymphoblastic leukemiaClin Cancer Res201319143832384323714728
- LemayCGRintoulJLKusAHarnessing oncolytic virus-mediated antitumor immunity in an infected cell vaccineMol Ther20122091791179922760544
- SimonsJWMikhakBChangJFInduction of immunity to prostate cancer antigens: results of a clinical trial of vaccination with irradiated autologous prostate tumor cells engineered to secrete granulocyte-macrophage colony-stimulating factor using ex vivo gene transferCancer Res199959205160516810537292
- LiuCSuksanpaisanLChenYWRussellSJPengKWEnhancing cytokine-induced killer cell therapy of multiple myelomaExp Hematol201341650851723403007
- PengKWDoganAVranaJTumor-associated macrophages infiltrate plasmacytomas and can serve as cell carriers for oncolytic measles virotherapy of disseminated myelomaAm J Hematol200984740140719507209
- MuthanaMRodriguesSChenYYMacrophage delivery of an oncolytic virus abolishes tumor regrowth and metastasis after chemotherapy or irradiationCancer Res201373249049523172310
- EisensteinSCoakleyBABriley-SaeboKMyeloid derived suppressor cells as a vehicle for tumor-specific oncolytic viral therapyCancer Res201373165003501523536556
- MorganRADudleyMEWunderlichJRCancer regression in patients after transfer of genetically engineered lymphocytesScience2006314579612612916946036
- BakerJVernerisMRItoMShizuruJANegrinRSExpansion of cytolytic CD8(+) natural killer T cells with limited capacity for graft-versus-host disease induction due to interferon gamma productionBlood200197102923293111342413
- LuPHNegrinRSA novel population of expanded human CD3+CD56+ cells derived from T cells with potent in vivo antitumor activity in mice with severe combined immunodeficiencyJ Immunol19941534168716967519209
- VernerisMRKaramiMBakerJJayaswalANegrinRSRole of NKG2D signaling in the cytotoxicity of activated and expanded CD8+ T cellsBlood200410383065307215070686
- HuangBSikorskiRSampathPThorneSHModulation of NKG2D-ligand cell surface expression enhances immune cell therapy of cancerJ Immunother201134328929621389869
- BingleLBrownNJLewisCEThe role of tumour-associated macrophages in tumour progression: Implications for new anticancer therapiesJ Pathol2002196325426511857487
- GabrilovichDINagarajSMyeloid-derived suppressor cells as regulators of the immune systemNat Rev Immunol20099316217419197294
- OreffoROCooperCMasonCClementsMMesenchymal stem cells: lineage, plasticity, and skeletal therapeutic potentialStem Cell Rev20051216917817142852
- HodgkinsonCPGomezJAMirotsouMDzauVJGenetic engineering of mesenchymal stem cells and its application in human disease therapyHum Gene Ther201021111513152620825283
- HerrlingerUWoiciechowskiCSena-EstevesMNeural precursor cells for delivery of replication-conditional HSV-1 vectors to intracerebral gliomasMol Ther20001434735710933953
- TylerMAUlasovIVSonabendAMNeural stem cells target intracranial glioma to deliver an oncolytic adenovirus in vivoGene Ther200916226227819078993
- AhmedAUThaciBAlexiadesNGNeural stem cell-based cell carriers enhance therapeutic efficacy of an oncolytic adenovirus in an orthotopic mouse model of human glioblastomaMol Ther20111991714172621629227
- GageFHMammalian neural stem cellsScience200028754571433143810688783
- DengWJiaJEndothelial progenitor cells as cellular vehicles to deliver oncolytic virus therapies to metastatic tumors: the “Trojan horse” approachMed Hypotheses200870484284417869010
- JevremovicDGulatiRHennigIUse of blood outgrowth endothelial cells as virus-producing vectors for gene delivery to tumorsAm J Physiol Heart Circ Physiol20042872H494H50015277193
- MaderEKButlerGDowdySCOptimizing patient derived mesenchymal stem cells as virus carriers for a phase I clinical trial in ovarian cancerJ Transl Med2013112023347343
- WillmonCHarringtonKKottkeTPrestwichRMelcherAVileRCell carriers for oncolytic viruses: fed ex for cancer therapyMol Ther200917101667167619690519
- BreitbachCJPatersonJMLemayCGTargeted inflammation during oncolytic virus therapy severely compromises tumor blood flowMol Ther20071591686169317579581
- SteegPSTumor metastasis: mechanistic insights and clinical challengesNat Med200612889590416892035
- BreitbachCJArulanandamRDe SilvaNOncolytic vaccinia virus disrupts tumor-associated vasculature in humansCancer Res20137341265127523393196
- BenenciaFCourregesMCConejo-GarciaJROncolytic HSV exerts direct antiangiogenic activity in ovarian carcinomaHum Gene Ther200516676577815960607
- OngHTTrejoTRPhamLDObergALRussellSJPengKWIntravascularly administered RGD-displaying measles viruses bind to and infect neovessel endothelial cells in vivoMol Ther20091761012102119277014
- JingYTongCZhangJTumor and vascular targeting of a novel oncolytic measles virus retargeted against the urokinase receptorCancer Res20096941459146819208845
- HallakLKMerchanJRStorgardCMLoftusJCRussellSJTargeted measles virus vector displaying echistatin infects endothelial cells via alpha(v)beta3 and leads to tumor regressionCancer Res200565125292530015958576
- BachtarziHStevensonMSubrVUlbrichKSeymourLWFisherKDTargeting adenovirus gene delivery to activated tumour-associated vasculature via endothelial selectinsJ Control Release2011150219620320965218
- SarkarDVemulaPKTeoGSChemical engineering of mesenchymal stem cells to induce a cell rolling responseBioconjug Chem200819112105210918973352
- SarkarDSpencerJAPhillipsJAEngineered cell homingBlood201111825e184e19122034631
- SacksteinRMerzabanJSCainDWEx vivo glycan engineering of CD44 programs human multipotent mesenchymal stromal cell trafficking to boneNat Med200814218118718193058
- WahajuddinAroraSSuperparamagnetic iron oxide nanoparticles: magnetic nanoplatforms as drug carriersInt J Nanomedicine201273445347122848170
- FattahiHLaurentSLiuFArsalaniNVander ElstLMullerRNMagnetoliposomes as multimodal contrast agents for molecular imaging and cancer nanotheragnosticsNanomedicine (Lond)20116352954421542690
- MuthanaMScottSDFarrowNA novel magnetic approach to enhance the efficacy of cell-based gene therapiesGene Ther2008151290291018418420
- PolyakBFishbeinIChornyMHigh field gradient targeting of magnetic nanoparticle-loaded endothelial cells to the surfaces of steel stentsProc Natl Acad Sci U S A2008105269870318182491
- ArbabASJordanEKWilsonLBYocumGTLewisBKFrankJAIn vivo trafficking and targeted delivery of magnetically labeled stem cellsHum Gene Ther200415435136015053860
- DialloJSLe BoeufFLaiFA high-throughput pharmacoviral approach identifies novel oncolytic virus sensitizersMol Ther20101861123112920389287
- MacTavishHDialloJSHuangBEnhancement of vaccinia virus based oncolysis with histone deacetylase inhibitorsPLoS One2010512e1446221283510
- HamadaKZhangTDesakiJCarrier cell-mediated cell lysis of squamous cell carcinoma cells by squamous cell carcinoma antigen 1 promoter-driven oncolytic adenovirusJ Gene Med201012654555420527047
- GuoZSParimiVO’MalleyMEThe combination of immunosuppression and carrier cells significantly enhances the efficacy of oncolytic poxvirus in the pre-immunized hostGene Ther201017121465147520703311
- RaykovZBalboniGAprahamianMRommelaereJCarrier cell-mediated delivery of oncolytic parvoviruses for targeting metastasesInt J Cancer2004109574274914999784
- OngHTHasegawaKDietzABRussellSJPengKWEvaluation of T cells as carriers for systemic measles virotherapy in the presence of antiviral antibodiesGene Ther200714432433317051248
- IankovIDMsaouelPAllenCDemonstration of anti-tumor activity of oncolytic measles virus strains in a malignant pleural effusion breast cancer modelBreast Cancer Res Treat2010122374575419894113
- AdairRAScottKJFraserSCytotoxic and immune-mediated killing of human colorectal cancer by reovirus-loaded blood and liver mononuclear cellsInt J Cancer2013132102327233823114986
- KomarovaSKawakamiYStoff-KhaliliMACurielDTPereboevaLMesenchymal progenitor cells as cellular vehicles for delivery of oncolytic adenovirusesMol Cancer Ther20065375576616546991
- MaderEKMaeyamaYLinYMesenchymal stem cell carriers protect oncolytic measles viruses from antibody neutralization in an orthotopic ovarian cancer therapy modelClin Cancer Res200915237246725519934299
- JosiahDTZhuDDreherFOlsonJMcFaddenGCaldasHAdipose-derived stem cells as therapeutic delivery vehicles of an oncolytic virus for glioblastomaMol Ther201018237738519904233