Abstract
Introduction
Benign prostatic hyperplasia (BPH) is a non-neoplastic proliferative disease of the prostate. Eriochloa villosa (EV) reportedly possesses various pharmacological activities, including anti-lipase activity and modulation of various antioxidative enzymes. In this study, we investigate the therapeutic potential of EV against BPH in a testosterone-induced BPH rat model.
Methods
Rats were subjected to a daily subcutaneous injection of testosterone (3 mg kg−1) for 4 weeks to induce BPH. Along with testosterone, rats in the treatment group were administered finasteride (10 mg kg−1) or EV (150 mg kg−1) via oral gavage. Prostatic cancer (LNCaP) cell line was used to examine the effect of EV.
Results
Finasteride and EV significantly decrease the relative prostate weight, serum levels of dihydrotestosterone and testosterone, and prostate epithelial thickness. Testosterone injection induced prostatic hyperplasia and proliferating cell nuclear antigen expression; however, EV treatment significantly attenuated these effects. Moreover, finasteride- and EV-treated rats exhibit an increase in the number of TUNEL-positive cells and reduced Bcl-2 expression in the prostate tissues compared with the testosterone-treated animals. Furthermore, EV suppresses inflammatory cytokines, including interleukin (IL)-6 and IL-8, in the prostate tissues. Meanwhile, the expression of inflammatory mediator cyclooxygenase-2 is consistently upregulated in testosterone-treated rats, whereas EV treatment significantly reverses this effect. Notably, EV treatment suppresses malondialdehyde (MDA) levels and upregulates testosterone-induced catalase (CAT) expression. In addition, EV suppresses expression of androgen receptor (AR) and prostate-specific antigen (PSA) induced by testosterone in LNCaP cells.
Conclusion
The present study results suggest that EV regulates prostatic proliferation, apoptosis, response to inflammation, and oxidative stress in the BPH rat model, and may, therefore, serve as a useful therapeutic agent for BPH.
Introduction
Benign prostatic hyperplasia (BPH) is one of prevalent diseases in men and its prevalence increases to nearly 70% in men over 80 years.1 BPH is characterized by an enlarged prostate and lower urinary tract symptoms (LUTS), which can significantly impact the quality of life (QOL) and requires medical intervention.Citation1
Although many reports have suggested pathophysiology of BPH, precise molecular mechanisms of BPH are yet to be elucidated. However, androgens, such as testosterone and dihydrotestosterone (DHT), stimulate prostatic growth, while 5α-reductase plays an important role in BPH pathogenesis.Citation1 In addition, an imbalance between the cell cycle and apoptotic cell death can result in an increase in cell number, ultimately resulting in uncontrolled prostatic growth.Citation2 Moreover, considering that chronic inflammation is associated with the histological changes occurring in the prostatic tissue of patients with BPH,Citation3 we hypothesized that inflammation contributes to BPH development. Local inflammatory process may be triggered by infection, which produce cytokines and chemokines involved in the inflammatory response, with consequent growth of epithelial and stromal prostatic cells.Citation3,Citation4
Currently, BPH treatment are aimed to ameliorate LUTS, inhibit disease progression, and reduce complications.Citation5 Currently available drugs for the treatment of BPH include alpha-adrenergic blockers (α1-blockers), 5α-reductase inhibitors (5-ARIs), muscarinic receptor antagonists (MRAs), phosphodiesterase 5 inhibitors (PDE5Is) and β3-adrenoceptor agonists. Furthermore, and herbal extracts, such as saw palmetto extract is an herbal product used in the treatment of symptoms related to benign prostatic hyperplasia. Among these, the most commonly used are α1-blockers, 5-ARIs, and a combination of α1-blockers and 5-ARIs (dutasteride and tamsulosin).Citation5
Eriochloa villosa (EV), also known as woolly cupgrass, is a member of the Poaceae family, which originates from Asia.Citation6 In a previous report, methanol EV extracts exhibited strong (>80%) in vitro anti-lipase activity.Citation7 The shoots and roots of EV also exhibit allelopathic effects for various antioxidative enzymes, such as ascorbate peroxidase, guaiacol peroxidase, and superoxide dismutase (SOD), while also impacting malondialdehyde (MDA) content.Citation6 Among constituents of EV extracts, chlorogenic acid have shown antioxidant, hepatoprotective, cardioprotective, anti-inflammatory, anti-obesity, antiviral, antimicrobial, and lipid metabolism modulation properties and is a dietary constituent.Citation8 Hwang et al demonstrated that chlorogenic acid attenuates LPS-induced cytokine release in RAW 264.7 macrophages, suggesting anti-inflammatory activity.Citation9 Meanwhile, orientin and vitexin have been isolated from various medicinal plants and exhibit multiple biological activities, including anti-inflammatory, antioxidant, antiviral, antibacterial, and cardioprotective properties.Citation10,Citation11 Lastly, dihydroactinidiolide possesses acetylcholinesterase inhibitory, antioxidant, and anti-aggregation activity, and reduces ROS generation in N2A cells.Citation12 However, the protective effects of EV against BPH have not yet been studied; therefore, in the present study, we investigate the protective effects of EV in a testosterone-induced BPH rat model.
Materials and Methods
Plants, Chemicals, and Reagents
EV extract (KPM037-022) was purchased from the Korea Plant Extract Bank at the Korea Research Institute of Bioscience and Biotechnology (Daejeon, Korea). The plant was collected from Sunchang-gun, Jeollabuk-do, KOREA in 2009. A voucher specimen (KRIB 0029775) is kept in the herbarium of the Korea Research Institute of Bioscience and Biotechnology. The plant (85 g) dried in the shade and powdered was added to 1L of Methyl alcohol 99.9% (HPLC grade) and extracted through 30 cycles (40KHz, 1500W, 15 min. ultrasonication-120 min. standing per cycle) at room temperature using an ultrasonic extractor (SDN-900H, SD-ULTRASONIC CO., LTD). After filtration (Qualitative Filter No.100, HYUNDAI MICRO CO., LTD) and drying under reduced pressure, EV extract (7.51 g) was obtained. For identification of EV constituents, chlorogenic acid, orientin, vitexin, and dihydroactinidiolide were provided from ChemFaces (Wuhan, China).
Ultra-High Performance Liquid Chromatography-Tandem Mass Spectrometry (UHPLC-MS/MS) Analyses of EV
UHPLC-MS/MS analysis was performed according to previously described methods, with slight modifications to identify the constituents of the EV extract.Citation13,Citation14 The analysis was performed using a Dionex UltiMate 3000 system equipped with a Thermo Q-Exactive mass spectrometer (Thermo Fisher Scientific, Waltham, MA, USA). Chromatographic separation was achieved using an Acquity BEH C18 column (100 × 2.1 mm, 1.7 µm, Waters Corp., Milford, MA, USA) and gradient elution consisting of 0.1% (v v−1) formic acid in water (A) and acetonitrile (B).Citation13 The injection volume and flow rate were 3 μL and 0.25 mL min−1, respectively. MS analysis was conducted using the full-scan MS-ddMS2 method in both positive and negative ionization modes, using an electrospray ionization source. The MS/MS parameters were set as follows: capillary temperature, 320 °C; scan range, 100–1500 m z−1; full-scan MS1 resolution, 70,000; and data-dependent MS2 resolution, 13,500. Data acquisition and processing were carried out using the software Xcalibur v.3.2 and TraceFinder v.4.0 (Thermo Fisher Scientific). The mass spectral patterns of the identified compounds were consistent with those reported previously.Citation14
Animals
Male Sprague–Dawley rats (7-weeks-old) were purchased from Orient Bio (Seongnam-si, Republic of Korea). Animals were maintained in constant environment (22 ± 2 °C; 50 ± 5% humidity and 12 h light/dark cycle) during experimental period. Animal experiments were performed in compliance with the National Institutes of Health Guide for the Care and Use of Laboratory Animals. The experimental animal study procedures were approved by the Animal Experimental Ethics Committee of Chungnam National University (approval number: CNU-00711).
Induction of BPH in Rats and Treatment Design
Rats were 220–250 g at dosing starting day and were randomly separated into four groups (7–8 rats in each group) and subjected to the following dosage regimens: 1) normal control (NC) group, administered a PBS oral gavage and corn oil as a vehicle subcutaneously (SC); 2) BPH group, BPH was induced daily via SC injection of testosterone propionate (Tokyo Chemicals Ins. Co., Tokyo, Japan) at 3 mg kg−1 and administered PBS as a vehicle control via oral gavage; 3) BPH plus finasteride (BPH + F) group (positive control), received finasteride (10 mg kg−1, Sigma) orally and SC injection of testosterone propionate (3 mg kg−1); (4) BPH + EV group, administered EV (150 mg kg−1) via oral gavage and SC injection of testosterone propionate (3 mg kg−1). All treatments were administered daily for 4 weeks, and administration volumes were 5 mL kg−1 for oral doses (PBS, finasteride, and EV) and 3 mL kg−1 for subcutaneous injections. All treatment groups were shown in . The body weights of the experimental animals were recorded once per week during the experiment, as well as on the day of necropsy. After the last injection, the rats were euthanized and whole blood was collected from the vena cava. Isolated sera from whole blood were stored at −70 °C. The prostate tissues were isolated and weighed.
Table 1 Group Design for Treatment
Determination of DHT, Testosterone, and MDA Level
Commercially available assay kits were used to measure testosterone (Caymanchem, MI, USA) and DHT (ALPCO Diagnostics, NH, USA) levels in the serum; all procedures were performed according to the manufacturer’s instructions. MDA levels in the prostatic tissue were determined using an enzyme-linked immunosorbent assay (ELISA) kit (Cell Biolabs, Inc., San Diego, USA), according to the manufacturer’s instructions. Values are expressed per milligram of protein for each prostatic tissue and content of protein was determined using protein assay kit (Thermo Scientific, MA, USA).
Histological Examination
Prostatic tissue fixed in 10% buffered formalin was embedded in paraffin wax, cut into 4-μm-thick sections and stained with hematoxylin and eosin (H&E). Epithelial thickness was measured in the ventral lobe of each prostate, as described in a previous study.Citation15
Proliferative Cell Nuclear Antigen (PCNA) Staining
To perform immunohistochemistry of PCNA, the tissue sections were treated with antibodies against PCNA (Abcam, Cambridge, UK) for 2 h, followed by biotinylated secondary antibody for 1 h. Diaminobenzidine (DAB; Vector Laboratories, CA, USA) was used for detection of immunological complexes, and then, the tissue samples were counterstained with hematoxylin. Five ventral lobe sections of the tissues from each rat were randomly analyzed. PCNA-positive cells were calculated as the percentage of total cells.
Terminal Deoxynucleotidyl Transferase dUTP Nick-End Labeling (TUNEL) Assay
Terminal deoxynucleotidyl transferase dUTP nick-end labeling (TUNEL) assay was performed using a commercial kit (Merck Millipore Corporation, MA, USA). TUNEL-positive cells were considered as apoptotic cells and these cells were counted in randomly selected fields. The results were showed as a percentage of the total cells.
Western Blot Analysis
Equal amounts (15 µg) of total protein from rat prostatic tissues were resolved using SDS-PAGE (Bio-Rad, CA, USA). Total protein was determined using protein assay kit (Thermo Scientific, MA, USA). The transferred membranes were incubated overnight with the following primary antibodies: anti-COX-2, anti-catalase, anti-androgen receptor (AR), anti-prostate specific antigen (PSA), anti-β-actin (Cell Signaling Technology, MA, USA), and anti-B-cell lymphoma 2 (Bcl-2; Santa Cruz, CA, USA). The membranes were then incubated with secondary antibodies at room temperature for 2 h, and the signals were detected using a chemiluminescence detection kit and quantified using CSAnalyzer4 (Atto, Tokyo, Japan).
Quantitative Real-Time PCR (RT-qPCR)
Total RNA was extracted from prostate tissue using TRIzol reagent (Sigma, MO, USA) and the absorbance of RNA samples were measured at 260 and 280 nm. Equal amounts of total RNA (1.4 µg) from the prostate tissue of each rat were subjected to reverse transcription (RT) using a commercially available RT kit (Toyobo, Osaka, Japan). Quantitative real-time PCR was performed using SYBR Green Master Mix (Thermo Scientific, MA, USA). The following primer sequences were used: Il6, 5ʹ-TAGTCCTTCCTACCCCAACT −3ʹ (forward) and 5ʹ-TTGGTCCTTAGCCACTCCTT-3ʹ (reverse); Il8, 5ʹ-CATTAATATTTAACGATGTGGATGCGTTTCA-3ʹ (forward) and 5ʹ-GCCTACCATCTTTAAACTGCACAAT-3ʹ (reverse); Gapdh, 5ʹ-ACAGCAACAGGGTGGTGGAC-3ʹ (forward) and 5ʹ-TTTGAGGGTGCAGCGAACTT-3ʹ (reverse). Relative expressions are presented as fold-change in the cDNA level of the target gene relative to that of the endogenous control (GAPDH), determined using the 2−ΔΔCt method, as described previously.Citation16
Cell Culture and Treatment
The Lymph Node Carcinoma of the Prostate (LNCaP) was obtained from the American Type Culture Collection (ATCC, Manassas, VA, USA). The cell line was incubated in RPMI-1640 medium (Gibco, CA, USA) with 10% fetal bovine serum (FBS). The cells were incubated in medium containing testosterone propionate (10 µM) and EV (50 to 200 µg mL−1) for 72h. After incubation, the cells were harvested for protein analysis. Cytotoxicity of EV was evaluated using the 3-(4,5-dimethylthiazol-2-yl)-2,5-diphenyltetrazolium bromide (MTT) assay as previously described.Citation17
Statistical Analysis
Multiple comparisons were performed using one-way analysis of variance (ANOVA), followed by Dunnett’s post-hoc test for comparison between BPH group and the other groups. Statistical significance was set at p < 0.05. Statistical analyses were performed using GraphPad Prism 6 (GraphPad Software Inc., CA, USA). Values are expressed as the mean ± standard deviation (SD).
Results
Analysis of EV
UHPLC-MS/MS analysis was conducted to identify EV constituents. The base peak chromatograms of EV and the extracted ion chromatograms for each phytochemical are shown in . The mass spectral characteristics of all identified compounds in EV are summarized in . In this study, four phytochemicals of EV, namely, chlorogenic acid, orientin, vitexin, and dihydroactinidiolide were identified from EV extract by comparisons with the mass spectra and retention times of reference standards. Most compounds except chlorogenic acid were identified in positive ion mode. The mass spectral patterns of the identified compounds were consistent with those reported previously.Citation14
Table 2 Phytochemicals Identified in Eriochloa villosa Using Ultra-High Performance Liquid Chromatography-Tandem Mass Spectrometry (UHPLC-MS/MS)
Effect of EV Extracts on Prostatic Weight
Compared to the prostates of the animals in the NC group, those of rats administered testosterone exhibited a significant increase in relative weight. However, these increases were significantly alleviated in the finasteride- and EV-treated groups ().
Table 3 Effect of Eriochloa villosa on Prostate Weight and Percentage Inhibition
Effect of EV Extracts on the Level of Testosterone and DHT
Considering that androgen hormones, such as testosterone and DHT, are critical mediators of BPH pathogenesis,Citation18 we examined the effects of EV on androgen hormone levels. The serum DHT levels were higher in the BPH group (6559 ± 1509 pg/mL) than in the NC group (478 ± 116 pg/mL); however, the levels were significantly lower in the finasteride- (4479 ± 285 pg/mL) and EV-treated groups (3414± 1247 pg/mL) compared to those in the BPH group (). Similarly, testosterone was higher in the prostate of the BPH group than in that of the NC group; however, these levels were markedly lower in the finasteride- and EV-treated groups ().
Figure 2 Effect of EV extracts on DHT and testosterone levels in a rat model of testosterone-induced benign prostatic hyperplasia (BPH). The levels of (A) DHT and (B) testosterone in serum (B). NC, corn oil-injected and PBS-treated rats; BPH, testosterone (3 mg kg−1)-injected and PBS-treated rats; BPH + F, testosterone-injected and finasteride (10 mg kg−1)-treated rats; BPH + EV, testosterone (3 mg kg−1)-injected and EV (150 mg kg−1)-treated rats. Data are expressed as the mean ± SD. ##p <0.01 compared with the NC group; *p <0.05 and **p <0.01 compared with the BPH group.
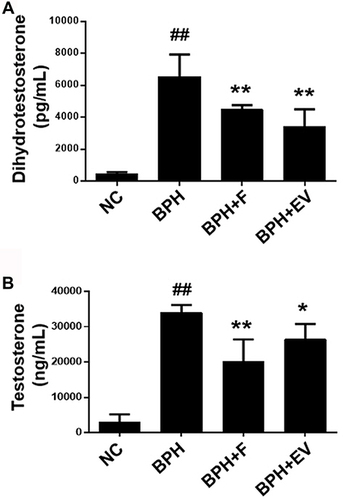
Effects of EV on Histological Changes in Prostate Tissues in a Rat Model of Testosterone-Induced BPH
Within the NC group, the prostate tissue morphology exhibited normal features. In contrast, the tissues of the BPH group showed glandular hyperplasia and increased epithelial thickness with decreased glandular luminal area (). Meanwhile, epithelial thickness was markedly decreased in the finasteride- and EV-treated groups compared to that in the BPH group ().
Figure 3 Effects of EV on histology in prostatic epithelium in a rat model of testosterone-induced benign prostatic hyperplasia (BPH). (A) Representative hematoxylin and eosin-stained prostate tissues (magnification, ×400). (B) The epithelial thickness of prostate tissues. NC, corn oil-injected and PBS-treated rats; BPH, testosterone (3 mg kg−1)-injected and PBS-treated rats; BPH + F, testosterone-injected and finasteride (10 mg kg−1)-treated rats; BPH + EV, testosterone (3 mg kg−1)-injected and EV (150 mg kg−1)-treated rats. Data are expressed as the mean ± SD. ##p <0.01 compared with the NC group; **p <0.01 compared with the BPH group.
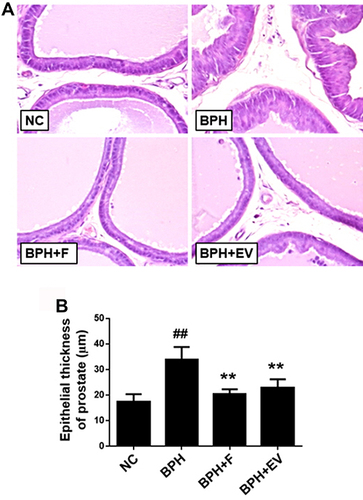
Effects of EV on Prostatic Cell Proliferation in a Rat Model of Testosterone-Induced BPH
PCNA staining was performed to evaluate the effect of EV on cell proliferation in the prostatic tissues. The number of PCNA-positive cells was significantly higher in the BPH group (50.10 ± 10.88%) than in the NC group (13.42 ± 10.45%). Notably, this increase was significantly inhibited in both finasteride (8.80 ± 4.20%) and EV-treated groups (10.22 ± 4.62%) (). The data suggest that EV prevents BPH progression through its antiproliferative activity.
Figure 4 Effects of EV on proliferation in prostatic tissue. (A) Representative prostate tissues immunostained with an anti- proliferative cell nuclear antigen (PCNA) antibody (magnification, ×400). (B) Number of PCNA-positive cells in the prostate. PCNA-positive nuclei were counted in five randomly selected fields (magnification, ×400) from each rat; results are calculated as PCNA-positive nuclei 100 cells−1. NC, corn oil-injected and PBS-treated rats; benign prostatic hyperplasia (BPH), testosterone (3 mg kg−1)-injected and PBS-treated rats; BPH + F, testosterone-injected and finasteride (10 mg kg−1)-treated rats; BPH + EV, testosterone (3 mg kg−1)-injected and EV (150 mg kg−1)-treated rats. Data are expressed as the mean ± SD. ##p <0.01 compared with the NC group; **p <0.01 compared with the BPH group.
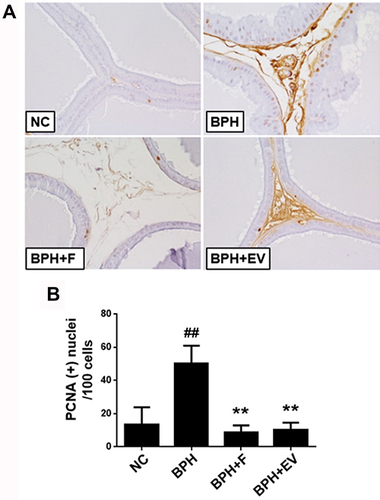
Effects of EV on Prostatic Cell Apoptosis in a Rat Model of Testosterone-Induced BPH
Next, we examined the effect of EV on apoptosis in the prostate using the TUNEL assay and Western blot analysis. Representative TUNEL staining is shown in . A higher number of TUNEL-positive cells was observed in the finasteride (13.82 ± 2.42%) and EV-treated groups (9.37 ± 2.02%) than in the BPH group (2.85 ± 1.77%) (). Protein expression of the anti-apoptotic protein, Bcl-2, was increased in the BPH group; in contrast, the finasteride- and EV-treated groups showed lower Bcl-2 expression (). These findings suggest that EV activates the apoptotic pathway via Bcl-2-dependent signaling.
Figure 5 Effects of EV on apoptotic pathway in prostatic tissue. (A) Representative prostate tissues of terminal deoxynucleotidyl transferase dUTP nick-end labeling (TUNEL) staining (magnification, ×400). (B) Number of TUNEL-positive cells in the prostate. TUNEL-positive nuclei were counted in five randomly selected fields (magnification, ×400) from each rat; results are calculated as TUNEL-positive nuclei 100 cells−1. (C) The expression levels of Bcl-2 using Western blotting; β-actin was used as a normalized control. NC, corn oil-injected and PBS-treated rats; benign prostatic hyperplasia (BPH), testosterone (3 mg kg−1)-injected and PBS-treated rats; BPH + F, testosterone-injected and finasteride (10 mg kg−1)-treated rats; BPH + EV, testosterone (3 mg kg−1)-injected and EV (150 mg kg−1)-treated rats. Data are expressed as the mean ± SD. ##p <0.01 compared with the NC group; **p <0.01 compared with the BPH group.
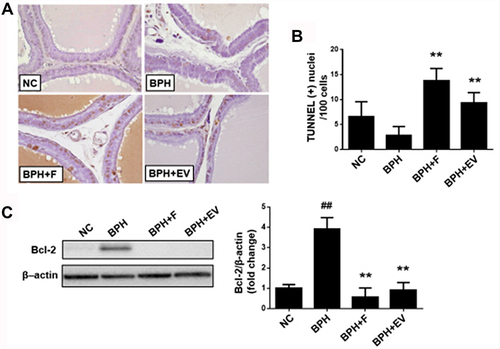
Effects of EV on Inflammation in a Rat Model of Testosterone-Induced BPH
Considering that inflammation plays an important role in BPH,Citation4 we sought to evaluate the involvement of inflammatory processes and the effects of EV on inflammatory cytokines within the BPH rat model. The morphology of the prostatic tissue was normal, with few inflammatory cells in the NC group, whereas more inflammatory cells were detected in the prostatic interstitial lesions of the BPH group animals. In contrast, the number of inflammatory cells in the prostatic tissues of finasteride- and EV-treated animals was similar to that in the NC group animals ().
Figure 6 Effects of EV on inflammatory cytokines in prostatic tissue. (A) Representative prostate tissues showing stromal infiltration of inflammatory cells in benign prostatic hyperplasia (BPH) groups (magnification, ×200). (B) RT-qPCR was performed to measure mRNA expression levels of IL-6 and IL-8 in prostate tissue. (C) The expression levels of COX-2 measured using Western blotting; β-actin was used as a normalized control. NC, corn oil-injected and PBS-treated rats; BPH, testosterone (3 mg kg−1)-injected and PBS-treated rats; BPH + F, testosterone-injected and finasteride (10 mg kg−1)-treated rats; BPH + EV, testosterone (3 mg kg−1)-injected and EV (150 mg kg−1)-treated rats. Data are expressed as the mean ± SD. ##p <0.01 compared with the NC group; *p <0.05 and **p <0.01 compared with the BPH group.
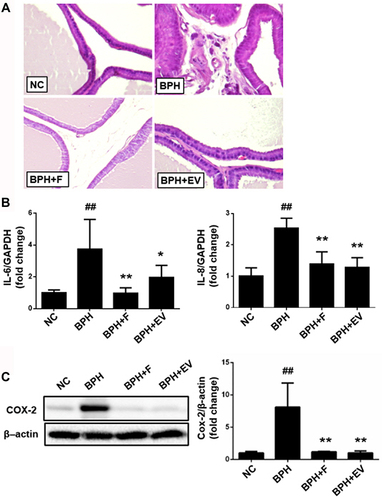
Additionally, the relative expression of IL-6 and IL-8 was higher in prostatic tissues from the BPH group, while being significantly lower in tissue from the finasteride- and EV-treated groups (). Similarly, COX-2 expression increased in the BPH group, while being notably reduced in the finasteride- and EV-treated groups ().
Effects of EV on Oxidative Stress
To examine the involvement of oxidative stress in the testosterone-induced BPH rat model and the effect of EV on oxidative stress, MDA levels were evaluated in the prostatic tissues. MDA level increased in the BPH group, and decreased in the finasteride- and EV-treated group (). Moreover, the expression of catalase (CAT), an antioxidant enzyme, decreased in the BPH group, and increased in the finasteride- and EV-treated groups (). These data suggest that EV prevents BPH progression, at least partially, through the antioxidative signaling pathway.
Figure 7 Effects of EV on oxidative stress in prostatic tissue. (A) malondialdehyde (MDA) levels in prostatic tissue. (B) The expression levels of catalase measured using Western blotting; β-actin was used as a normalized control. NC, corn oil-injected and PBS-treated rats; benign prostatic hyperplasia (BPH), testosterone (3 mg kg−1)-injected and PBS-treated rats; BPH + F, testosterone-injected and finasteride (10 mg kg−1)-treated rats; BPH + EV, testosterone (3 mg kg−1)-injected and EV (150 mg kg−1)-treated rats. Data are expressed as the mean ± SD. ##p <0.01 compared with the NC group; *p <0.05 and **p <0.01 compared with the BPH group.
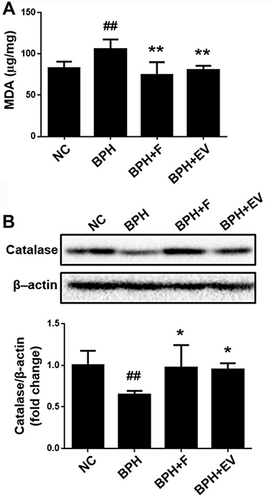
Effect of EV in LNCaP Cells
Androgens stimulate prostatic growth and PSA level is related to BPH pathogenesis.Citation1 Thus, we examined the effect of EV on androgen receptor (AR) and prostate-specific antigen (PSA) expression in human prostatic cancer cell lines. Testosterone treatment significantly increased the expression of AR and PSA, and that treatment with finasteride or EV (50 to 200 µg mL−1) significantly attenuated this effect (). EV did not induce cytotoxicity up to 200 µg mL−1 as shown . These findings suggest that EV suppresses androgen signaling in prostatic cancer cells.
Figure 8 Effects of EV on protein expression of prostate-specific antigen (PSA) and AR and viability in LNCaP Cell Line. (A) The cells were treated with testosterone propionate (10 µM), finasteride (30 µM) or EV at the indicated concentrations for 72 h, and cell lysates (30 µg) were subjected to Western blot analysis using antibody against PSA and AR. (B) Cell viability was determined by MTT assay. CON, vehicle-treated control; TP, testosterone-treated; F, testosterone and finasteride-treated; EV, testosterone and EV- treated. Data are expressed as the mean ± SD.
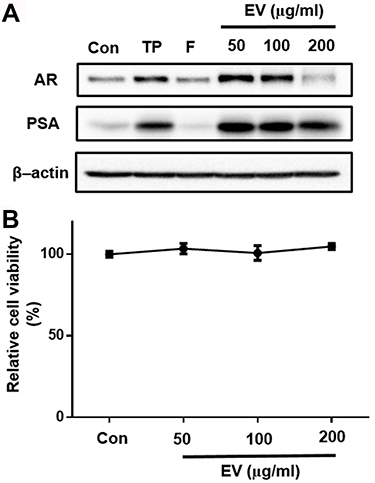
Discussion
BPH is characterized by an enlarged prostate and LUTS, which usually require medical consultation.Citation1 Androgen hormones, such as testosterone and DHT, play a major role in the development and progression of BPH.Citation18 The 5-ARIs, including finasteride and dutasteride, suppress the conversion of testosterone to DHT and are currently used for the treatment of BPH.Citation19 In the present study, EV decreased relative prostatic weight and the levels of DHT and testosterone in serum, similar to finasteride-treated animals.
To date, the pathogenesis and etiology of BPH have not been clearly elucidated.Citation20 However, an imbalance of prostate cell proliferation and apoptotic cell death contribute to BPH pathogenesis.Citation21 In the present study, cell proliferation and apoptosis were analyzed to examine the underlying mechanism of BPH, using TUNEL assay, as well as quantifying PCNA and Bcl-2 proteins. PCNA protein has key role in DNA replication, repair of DNA damage, and cell-cycle progression, and various physiological conditions, including BPH.Citation22,Citation23 Similarly, Bcl-2 inhibits apoptosis and binds to, and inactivates, pro-apoptotic proteins such as Bax.Citation24 In the present study, EV notably decreased the testosterone-induced increase in PCNA-positive cells. In addition, apoptotic indices, namely, TUNEL assay and Bcl-2 expression indicated that EV activated the apoptotic pathway. These results demonstrate that EV inhibits proliferation and promotes apoptosis of prostatic tissue in this BPH rat model, indicating an improvement in the balance of cell proliferation and apoptotic process.
Inflammatory process of prostatic tissue may be critical point of BPH progression.Citation25 However, the origin of prostate inflammation has not been fully elucidated and various factors could influence the generation of inflammation.Citation26 Both acute and chronic inflammation lead to recruitment of inflammatory cells into prostatic tissue, such as T lymphocytes and macrophages, neutrophils, eosinophils, and mast cells and recruited inflammatory cell type is dependent on the stimulant.Citation26 Prostate epithelial, stromal, and inflammatory cells produce cytokines, including CCL family (CCL-5, CCL-2), IL-1, IL-6, IL-18, and hypoxia-inducible factor-1α (HIF-1α), inducing local inflammation.Citation27,Citation28 IL-6 could be released by epithelial and stromal cells during inflammation and published report showed that IL-6 was upregulated in prostatic tissues of BPH compared to the normal prostate.Citation29 IL-8 is a proinflammatory cytokine and secretion of IL-8 by prostate epithelial cells and is involved in leukocyte chemotaxis. The levels of IL-8 was elevated in prostatic tissue of BPH patient and men with chronic prostatitis.Citation30 Moreover, cyclooxygenase (COX) activity plays a key role in the association of inflammation and proliferation of prostatic tissue.Citation20 Elevated COX-2 activity was found in various inflammatory cells of the epithelium and interstitium of human prostate and was observed in proliferative inflammatory lesions.Citation31,Citation32 Consistent with previous reports, in the present study, inflammatory cell infiltration was observed in the prostatic stroma and the levels of IL-6 and IL-8, as well as the expression of COX-2 were significantly higher in the BPH group animals; meanwhile, EV treatment significantly reversed these effects.
Alterations in the redox status play a role in the pathophysiology of inflammation. Previous studies have suggested that oxidative stress provoked chronic inflammation inducing various chronic prostatic diseases, such as chronic prostatitis and prostate cancer.Citation33 MDA levels in the plasma or serum reflect the value of lipid peroxidation acts as a biomarker of oxidative stress.Citation34 Serum MDA levels are significantly higher in BPH patients and showed strong correlation with PSA levels.Citation35 Meanwhile, the activity of antioxidant enzymes, including CAT and SOD, is downregulated in most prostatic tissue of BPH patient than normal prostatic tissues.Citation36 Similarly, the overall antioxidant activity is decreased in BPH patients compared to controls, and negative correlation has been reported between the levels of plasma peroxides and overall antioxidant activity of patients with BPH.Citation37 In the present study, EV notably alleviated the increase in MDA levels and increased CAT expression, suggesting antioxidative activity.
Androgen hormone has a key role in prostatic growth, and AR and PSA level is related to BPH pathogenesis.Citation1 In our previous reports, several extracts inhibited expressions of AR and PSA induced by testosterone and these extract showed therapeutic effect in BPH rat model.Citation38,Citation39 Consistently, in this study, testosterone increased expression of AR and PSA in LNCaP cells. Notably, EV treatment attenuated expression of AR and PSA. These findings suggest that EV suppresses androgen signaling in this cell system.
The present study results suggest that EV attenuated BPH progression by cell growth, anti-inflammation and anti-oxidative stress in testosterone-induced rat model.
Conclusion
This study demonstrates that EV treatment significantly prevents the progression of testosterone-induced prostatic enlargement and subsequent histological alterations in a rat model of BPH. More specifically, EV lowers the levels of DHT and testosterone and ameliorates the testosterone-induced imbalance between prostatic proliferation and apoptotic cell death. Furthermore, the expression of testosterone-induced inflammatory cytokine and oxidative stress markers is significantly suppressed in EV-treated animals. In LNCaP cells, EV significantly inhibited expression of AR and PSA induced by testosterone treatment. Collectively, these results suggest that EV exhibits therapeutic potential for preventing BPH progression.
Abbreviations
5-ARIs, 5α-reductase inhibitors; α1-blockers, alpha1-adrenergic antagonists; Bcl-2, B-cell lymphoma 2; BPH, benign prostatic hyperplasia; COX-2, cyclooxygenase-2; DHT, dihydrotestosterone; EV, Eriochloa villosa; H&E, hematoxylin and eosin; IL, interleukin; LUTS, lower urinary tract symptoms; MDA, malondialdehyde; MRA, muscarinic receptor antagonists; PCNA, proliferating cell nuclear antigen; PDE5Is, phosphodiesterase 5 inhibitors; QOL, quality of life; SC, subcutaneous; TUNEL, terminal deoxynucleotidyl transferase dUTP nick-end labeling; UHPLC-MS/MS, ultra-high performance liquid chromatography-tandem mass spectrometry.
Data Sharing Statement
All the data generated and analyzed in this study are included in this manuscript.
Disclosure
The authors report no conflicts of interest in this work.
Additional information
Funding
References
- Madersbacher S, Sampson N, Culig Z. Pathophysiology of benign prostatic hyperplasia and benign prostatic enlargement: a mini-review. Gerontology. 2019;65(5):458–464. doi:10.1159/000496289
- Kyprianou N, Tu H, Jacobs SC. Apoptotic versus proliferative activities in human benign prostatic hyperplasia. Hum Pathol. 1996;27(7):668–675. doi:10.1016/s0046-8177(96)90396-2
- Soler R, Andersson KE, Chancellor MB, et al. Future direction in pharmacotherapy for non-neurogenic male lower urinary tract symptoms. Eur Urol. 2013;64(4):610–621. doi:10.1016/j.eururo.2013.04.042
- Chughtai B, Lee R, Te A, Kaplan S. Role of inflammation in benign prostatic hyperplasia. RevUrol. 2011;13(3):147–150.
- Yu ZJ, Yan HL, Xu FH, et al. Efficacy and side effects of drugs commonly used for the treatment of lower urinary tract symptoms associated with benign prostatic hyperplasia. FrontPharmacol. 2020;11:658. doi:10.3389/fphar.2020.00658
- Szilágyi A, Radócz L, Hájos MT, et al. The impacts of woolly Cupgrass on the antioxidative system and growth of a maize hybrid. Plants. 2021;10(5):982. doi:10.3390/plants10050982
- Sharma N, Sharma VK, Seo SY. Screening of some medicinal plants for anti-lipase activity. J Ethnopharmacol. 2005;97(3):453–456. doi:10.1016/j.jep.2004.11.009
- Naveed M, Hejazi V, Abbas M, et al. Chlorogenic acid (CGA): a pharmacological review and call for further research. Biomed Pharmacother. 2018;97:67–74. doi:10.1016/j.biopha.2017.10.064
- Hwang SJ, Kim YW, Park Y, Lee HJ, Kim KW. Anti-inflammatory effects of chlorogenic acid in lipopolysaccharide-stimulated RAW 264.7 cells. Inflamm Res. 2014;63(1):81–90. doi:10.1007/s00011-013-0674-4
- He M, Min JW, Kong WL, He XH, Li JX, Peng BW. A review on the pharmacological effects of vitexin and isovitexin. Fitoterapia. 2016;115:74–85. doi:10.1016/j.fitote.2016.09.011
- Lam KY, Ling AP, Koh RY, Wong YP, Say YH. A review on medicinal properties of Orientin. Adv Pharmacol Sci. 2016;2016:4104595. doi:10.1155/2016/4104595
- Das M, Prakash S, Nayak C, et al. Dihydroactinidiolide, a natural product against Abeta25-35 induced toxicity in Neuro2a cells: synthesis, in silico and in vitro studies. Bioorg Chem. 2018;81:340–349. doi:10.1016/j.bioorg.2018.08.037
- Kim YJ, Jeon WY, Hwang YH, Lee MY. Inhibitory effects of Gyeji-tang on MMP-9 activity and the expression of adhesion molecules in IL-4- and TNF-alpha-stimulated BEAS-2B cells. Plants. 2021;10(5):951. doi:10.3390/plants10050951
- Seo CS, Song KH. Phytochemical characterization for quality control of Phyllostachys pubescens Leaves using high-performance liquid chromatography coupled with diode array detector and tandem mass detector. Plants. 2021;11(1):50. doi:10.3390/plants11010050
- Rho J, Seo CS, Park HS, et al. Ulmus macrocarpa Hance improves benign prostatic hyperplasia by regulating prostatic cell apoptosis. J Ethnopharmacol. 2019;233:115–122. doi:10.1016/j.jep.2018.11.042
- Park HS, Wijerathne CUB, Jeong HY, Seo CS, Ha H, Kwun HJ. Gastroprotective effects of Hwanglyeonhaedok-tang against Helicobacter -induced gastric cell injury. J Ethnopharmacol. 2018;216:239–250. doi:10.1016/j.jep.2018.01.025
- Jiang R, Xu XH, Wang K, et al. Ethyl acetate extract from panax ginseng C.A. Meyer and its main constituents inhibit alpha-melanocyte-stimulating hormone-induced melanogenesis by suppressing oxidative stress in B16 mouse melanoma cells. J Ethnopharmacol. 2017;208:149–156. doi:10.1016/j.jep.2017.07.004
- Andriole G, Bruchovsky N, Chung LW, et al. Dihydrotestosterone and the prostate: the scientific rationale for 5alpha-reductase inhibitors in the treatment of benign prostatic hyperplasia. J Urol. 2004;172(4 Pt 1):1399–1403. doi:10.1097/01.ju.0000139539.94828.29
- Wurzel R, Ray P, Major-Walker K, Shannon J, Rittmaster R. The effect of dutasteride on intraprostatic dihydrotestosterone concentrations in men with benign prostatic hyperplasia. Prostate Cancer Prostatic Dis. 2007;10(2):149–154. doi:10.1038/sj.pcan.4500931
- Minciullo PL, Inferrera A, Navarra M, Calapai G, Magno C, Gangemi S. Oxidative stress in benign prostatic hyperplasia: a systematic review. Urol Int. 2015;94(3):249–254. doi:10.1159/000366210
- Pawlicki B, Zieliński H, Dabrowski M. Znaczenie apoptozy i przewlekłego zapalenia gruczołu krokowego w patogenezie łagodnego rozrostu. [Role of apoptosis and chronic prostatitis in the pathogenesis of benign prostatic hyperplasia]. Pol Merkur Lekarski. 2004;17(100):307–310. Polish.
- Glover M, Soni S, Ren Q, Maclennan GT, Fu P, Gupta S. Influence of chronic inflammation on Bcl-2 and PCNA expression in prostate needle biopsy specimens. Oncol Lett. 2017;14(4):3927–3934. doi:10.3892/ol.2017.6668
- Schönenberger F, Deutzmann A, Ferrando-May E, Merhof D. Discrimination of cell cycle phases in PCNA-immunolabeled cells. BMC Bioinform. 2015;16:180. doi:10.1186/s12859-015-0618-9
- Tzifi F, Economopoulou C, Gourgiotis D, Ardavanis A, Papageorgiou S, Scorilas A. The role of BCL2 family of apoptosis regulator proteins in acute and chronic leukemias. AdvHematol. 2012;524308. doi:10.1155/2012/524308
- Robert G, Descazeaud A, Nicolaïew N, et al. Inflammation in benign prostatic hyperplasia: a 282 patients’ immunohistochemical analysis. Prostate. 2009;69(16):1774–1780. doi:10.1002/pros.21027
- Krušlin B, Tomas D, Džombeta T, Milković-Periša M, Ulamec M. Inflammation in prostatic hyperplasia and carcinoma-basic scientific approach. FrontOncol. 2017;7:77. doi:10.3389/fonc.2017.00077
- Mishra VC, Allen DJ, Nicolaou C, et al. Does intraprostatic inflammation have a role in the pathogenesis and progression of benign prostatic hyperplasia? BJU Int. 2007;100(2):327–331. doi:10.1111/j.1464-410X.2007.06910.x
- Nickel JC. Prostatic inflammation in benign prostatic hyperplasia—the third component? Can J Urol. 1994;1(1):1–4.
- Mechergui YB, Ben Jemaa A, Mezigh C, et al. The profile of prostate epithelial cytokines and its impact on sera prostate specific antigen levels. Inflammation. 2009;32(3):202–210. doi:10.1007/s10753-009-9121-7
- Liu LR, Li QJ, Han P, et al. Evaluation of interleukin-8 in expressed prostatic secretion as a reliable biomarker of inflammation in benign prostatic hyperplasia. Urology. 2009;74(2):340–344. doi:10.1016/j.urology.2009.02.064
- Palapattu GS, Sutcliffe S, Bastian PJ, et al. Prostate carcinogenesis and inflammation: emerging insights. Carcinogenesis. 2009;26(7):1170–1181. doi:10.1093/carcin/bgh317
- Sugar LM. Inflammation and prostate cancer. Can J Urol. 2006;13(suppl 1):46–47.
- Paulis G. Inflammatory mechanisms and oxidative stress in prostatitis: the possible role of antioxidant therapy. Res RepUrol. 2018;10:75–87. doi:10.2147/RRU.S170400
- Meagher EA, FitzGerald GA. Indices of lipid peroxidation in vivo: strengths and limitations. Free Radic Biol Med. 2000;28(12):1745–1750. doi:10.1016/s0891-5849(00)00232-x
- Merendino RA, Salvo F, Saija A, et al. Malondialdehyde in benign prostate hypertrophy: a useful marker? Mediators Inflamm. 2003;12(2):127–128. doi:10.1080/0962935031000097745
- Olinski R, Zastawny TH, Foksinski M, Barecki A, Dizdaroglu M. DNA base modifications and antioxidant enzyme activities in human benign prostatic hyperplasia. Free Radic Biol Med. 1995;18(4):807–813. doi:10.1016/0891-5849(94)00171-f
- Pace G, Di Massimo C, De Amicis D, et al. Oxidative stress in benign prostatic hyperplasia and prostate cancer. Urol Int. 2010;85(3):328–333. doi:10.1159/000315064
- Ub Wijerathne C, Park HS, Jeong HY, et al. Quisqualis indica improves benign prostatic hyperplasia by regulating prostate cell proliferation and apoptosis. Biol Pharm Bull. 2017;40(12):2125–2133. doi:10.1248/bpb.b17-00468
- Park HS, Seo CS, Wijerathne CU, et al. Effect of veratrum maackii on testosterone propionate-induced benign prostatic hyperplasia in rats. Biol Pharm Bull. 2019;42(1):1–9. doi:10.1248/bpb.b18-00313