Abstract
The identification of normal and cancerous stem cells and the recent advances made in isolation and culture of stem cells have rapidly gained attention in the field of drug discovery and regenerative medicine. The prospect of performing screens aimed at proliferation, directed differentiation, and toxicity and efficacy studies using stem cells offers a reliable platform for the drug discovery process. Advances made in the generation of induced pluripotent stem cells from normal or diseased tissue serves as a platform to perform drug screens aimed at developing cell-based therapies against conditions like Parkinson’s disease and diabetes. This review discusses the application of stem cells and cancer stem cells in drug screening and their role in complementing, reducing, and replacing animal testing. In addition to this, target identification and major advances in the field of personalized medicine using induced pluripotent cells are also discussed.
Introduction
The advent of technology and a plethora of experimental work have unequivocally established the existence of stem cells in many tissues.Citation1–Citation8 Cell self-renewal, ie, the ability to produce an identical copy of itself, and multipotency, ie, the ability to generate cells different from itself, are fundamental characteristics of a stem cell. The ability to self-renew or be multipotent varies between different stem cells and is referred to as “stemness”.Citation9 These are unique qualities that have allowed both academia and industry to foresee the application of stem cells in various diseases/disorders and medical challenges, like Parkinson’s disease, Alzheimer’s disease, diabetes, multiple sclerosis, heart disease, cancer, spinal cord injury, wound healing, and organ transplantation ().Citation10–Citation22 Recent advances in the treatment of graft versus host diseaseCitation23 and the success of hematopoietic stem cell transplantsCitation21 have made it possible to envisage stem cells as a potential reservoir to meet our unmet medical needs.
Figure 1 Overview of the focus areas for developing stem cell-based screening strategies and their potential application in different human diseases and disorders.
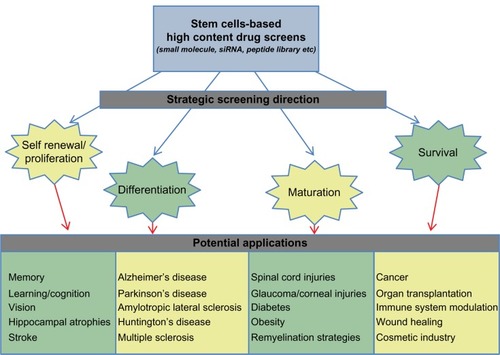
In addition to the wide array of applications in the field of regenerative medicine, the possibility of performing high-content drug screens as well as toxicity and efficacy studies has enabled stem cells to become a valuable resource in the field of drug discovery and pharmaceutical research.Citation24,Citation25 The recent demonstration of reprogramming of somatic cells into “embryonic stem cell-like” induced pluripotent stem cellsCitation26,Citation27 has revolutionized pharmaceutical research by linking human disease, patient specificity, and drug discovery. This process could not only lead to the development of various cellular therapies, but also push the boundaries of personalized medicine. The availability and ever-evolving methodologies used to isolate human (or animal) embryonic stem cells, adult tissue-specific neural stem cells, and human induced pluripotent stem cellsCitation28 have led to the development of various high throughput and combinatorial screening technologies,Citation29–Citation31 thereby augmenting the role of stem cell models in drug discovery.
Stem cells as in vitro models for drug discovery
Drug testing using in vitro models has been a major boost, not only in identifying potential therapeutic compounds, but also in increasing our understanding of their absorption, distribution, metabolism, and excretion (ADME) properties.Citation32 The development of various in vitro ADME models has made a clear impact on the pathway towards modernizing and accelerating drug discovery and development. A clear proof of principle can be seen in the case of terfenadine in the late 1980s,Citation33 whereby use of an in vitro ADME system enabled researchers to understand torsades de pointes, an often fatal cardiac arrhythmia.Citation33,Citation34 Tumor-derived or engineered immortalized cells obtained from human (or animal) sources have been the most common in vitro models used by the biotechnology and pharmaceutical industries.Citation35,Citation36 While these cell lines have the advantages of convenience and scalability of the screening process, they show high variability in their growth, abnormal genotype, and physiological response to drugs. The abnormalities associated with these immortalized cells restrict the confidence value and number of lead molecules for drug development. The use of specialized primary culture models like hepatocytes, human umbilical endothelial cells, and keratinocytes offer limited usage due to their restricted expandability.Citation37–Citation39
The need for an improved and uniform physiological response, normal genotype, and growth pattern has redirected drug discovery efforts towards stem cells. The possibility of isolating stem cells from a wide spectrum of tissuesCitation1–Citation8 and growing them in vitro, as well as their ability to differentiate into a number of specialized cell types has provided an invaluable tool for drug/target discovery and validation.Citation40 The use of stem cells will not only bring drug discovery costs down, but also improve the chances of identifying leads with a target or pathway relevant to the disease process and with a stronger potential for translation to clinical settings.
Although stem cells offer huge potential in the field of pharmaceutical research, their immediate applicability in the field of drug discovery is limited due to a variety of factors. For example, stem cells from different tissues are not the same,Citation19 and in vitro culture where the stem cells are flooded with growth factors is different from the microenvironment in which stem cells reside in vivo.Citation41,Citation42 Stem cells have a slower cell cycle than their progenitor cells in vivo, so the degree of sensitivity of readouts obtained in vitro may not be the same as in vivo.Citation41,Citation42 Stem cells isolated from tissues such as the liver, muscle, and adipose tissue have a limited ability to expand when compared with bone marrow-derived mesenchymal stem cells ().Citation19 In addition, stem cells derived from tissues like muscle and the liver lose their potential to differentiate upon repeated expansion, while undifferentiated hematopoietic stem cells expand poorly but can differentiate into all types of blood cells.Citation43,Citation44
Figure 2 Different types of stem cells and their potential to be used in large-scale screens. The different types of cells are arranged in three boxes indicating high, medium, and low expandability.
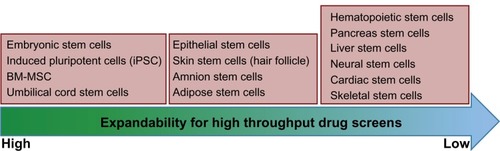
Bone marrow-derived mesenchymal stem cells offer an attractive high throughput screening platform for new target/drug discovery. These stem cells can be readily expanded in vitro and can be isolated from a variety of tissue sources, such as brain, lung, heart, muscle, and the umbilical cord. Bone marrow-derived mesenchymal stem cells have demonstrated scalability for drug screens and can be differentiated into neurons, adipocytes, muscle cells, chondrocytes, and osteocytes. These features of bone marrow-derived mesenchymal stem cells allow drug screens to be directed towards stem cell self-renewal, proliferation, differentiation, and a variety of disease-related drug discovery programs, eg, for cancer, obesity, diabetes, and central or peripheral nervous system disorders ( and ).Citation45–Citation49 The true power of stem cells in drug discovery programs will be fully realized when a readily expandable diverse panel of stem cells becomes available.
Cancer stem cells in drug discovery
Cancerous cells also exploit the same properties of self-renewal and multipotency that make stem cells so attractive in regenerative medicine and drug discovery. Recent studies have identified a small unique population of cells known as cancer stem cells, or tumor-inducing cells, that reside in tumors.Citation50 These cells arise from oncogenic transformation of either stem cells or progenitor cells. Cancer stem cells or tumor-inducing cells have “stem-like” character, a slow proliferation rate, a high capacity for self-renewal, resistance to standard chemical/radiation therapy, and a propensity to differentiate into actively proliferating tumor cells.Citation50,Citation51 Cancer stem cells have been isolated from many tumor types, including brain, renal, colon, and prostate tumors, as well as hematopoietic cancers, and therapeutic strategies are being developed to target cancer stem cells for apoptosis or cell cycle arrest specifically and thereby eradicate tumors more effectively than current treatments.Citation51,Citation52 Cancer drug discovery programs have, to a large extent, employed immortalized cell lines or primary tumor tissue for in vitro assay. This approach has been largely unsatisfactory in developing effective therapeutics for cancer. Cancer drug discovery programs involving cancer stem cells as a platform offer a discovery process with a high degree of therapeutic efficiency.Citation53–Citation55 This is particularly evident from the drug discovery process in leukemia.Citation53 The fact that cancer stem cells feed the cancer growth and promote resistance to existing chemotherapeutic drugs makes them candidate cells for drug discovery screens.Citation53–Citation55 In cancer of the hematopoietic system, cancer stem cells have been well characterized in chronic myelogenous leukemia, acute myelogenous leukemia, and acute lymphoblastic leukemia.Citation56–Citation58 Drug discovery programs targeting CD33 in acute myelogenous leukemia and the ABL kinase inhibitor, imatinib mesylate, in chronic myelogenous leukemia have been very effective. Biomarkers such as CD33 have been shown to be uniquely characteristic of the cancer stem cells of acute myelogenous leukemia, and efforts to design immunotherapy against such biomarkers are becoming very useful.Citation58–Citation61
From the perspective of drug discovery and translational research, cancer stem cells provide a solid platform with significant implications for designing therapeutic approaches and developing long-term treatment ().Citation51,Citation52 It has to be noted that in vitro propagation of cancer stem cells does not mimic factors governing the microenvironment of cancer stem cells, such as hypoxia,Citation62 but they do offer a platform for evaluating drug screens with a higher degree of confidence, thereby facilitating preclinical trials and clinical trials of antitumor therapies.Citation54 Research reports indicating that cancer stem cells can be selectively targeted without harming normal stem cells offers a possibility to perform targeted drug discovery screens against cancer stem cells from different tumors.Citation63
Stem cells complement animal models in drug discovery
Stem cells have already received enormous attention for their potential in refining drug screens. Stem cell screens are a more cost-effective strategy than animal testing. They are also seen as a process of refining, reducing, and possibly replacing animal testing procedures. However, animal models offer a whole system setup for testing the effects and side effects of drugs.Citation64–Citation66 It has to be noted that the response to drugs in animal models may not be the same as in humans. Animal models differ from those in humans in a number of ways. In particular, they do not reflect the ethnic diversity of humans and their response to drugs may be different to that seen in humans. In addition, animal models are costly and time-consuming. Stem cells offer an alternate platform to overcome the limitations of ethnic diversity, provide a uniform response to a drug which can correlate with the human response, and are cost- and time-effective. Unfortunately, the current status of stem cell research does not allow us to substitute for whole animal testing, as seen with animal models. However, stem cells are very promising substitutes in terms of single organ toxicity.Citation67 Embryonic or adult tissue-specific stem cells that can ultimately be grown into an entire human organ will be a gold standard for single organ drug testing.
Cell-based in vitro assays provide a scaffold to perform high throughput or high content screens (), but they do not reflect the complex in vivo scenario. Cell-based screens do not take into consideration the cross-talk between organs (or different cell types) or the general metabolism and side effects seen in vivo. Hence in vitro assays have to be complemented with various in vivo assays using different animal models. Currently, various invertebrate and vertebrate models are available for screening the toxicity and efficacy of lead molecules.
Invertebrate models of Drosophila and Caenorhabditis elegans offer a powerful model suitable for drug discovery research.Citation68–Citation71 Their small size, short generation time, relatively low cost of housing and maintenance, highly conserved molecular pathways, and availability of various genetic and biochemical tools have made it possible to include them in multistep drug screening processes.Citation69 The ease with which transgenics, overexpression, and mutation of proteins can be done, as well as the relatively simple genetic cascade involved have enabled their use in performing screens directed against Alzheimer’s disease, Huntington’s disease, oncogenic transformation, stem cell niche, metastases, neurodegeneration, apoptosis, and behavioral analyses.Citation72–Citation76 These simple models offer a setup whereby a particular process such as neurodegeneration can be quickly screened. For example, a Drosophila Huntington’s disease model and transgenic line provide an ideal in vivo system for not only examining mutant Huntington gene-mediated cellular defects, such as impairment of axonal transport, but also facilitate rapid assays for screening and validating potential treatment to alleviate the observed cellular defects.Citation77,Citation78 The relatively simple metabolic and genetic cascades and the long evolutionary separation from humans are major disadvantages limiting the use of these models in drug development research.
Higher vertebrate models such as rabbits, dogs, monkeys, and rodents have been extensively used in drug safety testing. Among them, rodents are a very good complement to stem cell-based screening. The availability of knockins, conditional knockouts, and transgenic models forms a powerful support system to evaluate the in vivo response of various lead molecules identified in stem cell-based in vitro screens.Citation79,Citation80 The identification of a tissue-specific stem cell niche in rodent modelsCitation81 offers the advantage of developing screens directed towards manipulation of the stem cell microenvironment to aid in understanding and developing therapeutic strategies for various diseases, such as neurodegenerative disorders, stroke, organ transplant, brain trauma, wound healing, and cancer. Such screens have identified molecules that affect the progenitor pool size of the adult neural stem cell population.Citation82 However, the traditional methods of analysis in rodent models are slow and rely extensively on analyses of tissues collected from sacrificed animals.
In recent years, teleost vertebrate models, in particular zebrafish and medaka, have become popular models for studying various aspects of developmental biology and genetics. Their rapid external development, transparency of embryos, husbandry, and large sample size are some of the advantages readily offered by these models.Citation83–Citation85 The possibility of performing toxicity and efficacy screening of chemicals, pharmaceuticals, and pesticides that can be correlated in terms of human health risks are propelling this model as a choice for toxicological or pharmacological screens. Small molecule screening to identify and characterize a molecule that produces specific effects against various disease processes in humans has been successfully developed.Citation86,Citation87 The zebrafish (Danio rerio) has been more extensively used than the medaka (Oriyzas latipes), but both show a high degree of anatomical and physiological homology with that of other higher order vertebrates, and also have very similar cellular structure, signaling processes, and cognitive behavior.Citation86,Citation87 The collection of various mutant lines with defects in the development and function of the various metabolic processes, and the availability of various biochemical, molecular, and genetic techniques has facilitated the development of various in vivo drug screens targeting development, metabolism, and physiological conditions in terms of various human diseases.Citation88,Citation89
Target identification and evaluation tools aiding stem cell-based drug discovery
Drug discovery screens using stem cells are a new and immensely necessary resource (). The most commonly used screening process involves growing cells as a monolayer culture and exposing them to libraries of new chemical entities. The final readout in most of the high throughput scenarios is based on imaging.Citation90 Imaging-based screens are quick and can be automated with ease. Time-lapse assays can also be performed to understand the onset of the phenotype. Imaging-based readouts can simply employ bright field images to understand the impact of the compound on colony size, morphology, proliferation, and cell number. These image-based readouts can also be combined with immunofluorescence to test the expression/inhibition of markers of interest. Commercially available assays, which use fluorescent readouts to measure cell proliferation, apoptosis, and toxicity, can be employed with relative ease in a high throughput screen scenario.Citation90–Citation94 Other methodologies, such as quantitative polymerase chain reaction and fluorescence-activated cell sorting, can be performed at a low to medium throughput screening level and offer wide endpoint assays, such as measuring cell cycle and proliferation, as well as quantifying expression of biomarkers at the RNA or protein level. Techniques such as microarrays, chip-sequencing, single cell illumina-based sequencing, and proteomic approaches, eg, affinity column purification and matrix-assisted laser desorption ionization time-of-flight mass spectrometry, can be used effectively as a high-end analysis for target identification and characterization of the lead compound ().
Applications of stem cells in drug discovery
One of the straightforward applications of stem cells lies in clarifying disease mechanisms and toxicity. Testing lead compounds for neuronal, hepatic, and cardiac toxicity would provide direct assessment of the effects and side effects of drugs.Citation25 Embryonic or tissue-specific stem cells from normal individuals and diseased patients can be obtained and differentiated in neurons, hepatocytes, or cardiomyocytes for toxicity testing.Citation95 In a scenario where stem cells are not available, somatic cells from normal individuals or diseased patients can be reprogrammed into induced pluripotent stem cells for further differentiation and toxicity testing. However, it has to be noted that the complete molecular nature of induced pluripotent stem cells has not been thoroughly evaluated, hence direct clinical translation of any screen done on induced pluripotent stem cells will not be possible in the near future.Citation96 In neurodegenerative conditions like Parkinson’s disease, stroke, Alzheimer’s disease, and Huntington’s disease, stem cell-based drug discovery programs will transform the way in which therapies are discovered and designed.Citation19,Citation95 Screens aimed at enriching stem cell populations or directing their differentiation into a particular cell type would be immensely beneficial in faster recovery from disease (). Currently, various screens to isolate lead molecules capable of increasing the proliferation of stem cells, while preserving their “stem-like” properties and screens aimed at directed differentiation of stem cells are being performed in vitro.Citation40 The recent development of technology that allows the reprogramming of somatic cells into induced pluripotent stem cells presents an opportunity to conduct screens on induced pluripotent stem cells derived from patients with the aim of finding lead molecules that delay the onset of disease, speed up the recovery process, or possibly cure the disease.Citation97 Stem cells or induced pluripotent stem cells could also act as transport agents for delivery of small molecules, therapeutic agents, or the correct version of a diseased gene in patients.Citation98–Citation100 Screens aimed at cancer stem cells would not only help us in designing better therapy, but also enable discovery of molecules that could result in a long-term disease-free state.Citation101 It is very realistic to imagine creating normal or disease-specific human stem cell panels to aid in efforts for screening, discovery, and development of drugs.
Conclusion
In conclusion, stem cells (both normal and cancerous) are a valuable tool in the drug discovery process. They enable rapid identification of therapeutically useful molecules that can modulate normal and tumorigenic stem cell behavior (). Although the direct clinical applications of human embryonic stem cells are limited due to ethical concerns and the potential for teratoma formation, they can still be heavily exploited as a tool in various high content screens directed towards self-renewal, multipotency, and differentiation. The identification of tissue-specific stem cells, cancer stem cells, and the recent development of induced pluripotent stem cells, offers an attractive opportunity for developing drug screens aimed at various human disease states. Drug screens and stem cell research have the potential to develop novel cellular and gene therapies that could be directly applied in the clinical treatment of various genetic and degenerative disorders.
Acknowledgments
The authors would like to thank Karolinska Institutets Forskningsstiftelser 2010 for financial support, Mr KV Padmanabhan and Mrs Srividya Seshadri for proof reading the text.
Disclosure
The authors report no conflicts of interest in this work.
References
- BrittanMWrightNAGastrointestinal stem cellsJ Pathol2002197449250912115865
- BryderDRossiDJWeissmanILHematopoietic stem cells: the paradigmatic tissue-specific stem cellAm J Pathol2006169233834616877336
- BussolatiBBrunoSGrangeCIsolation of renal progenitor cells from adult human kidneyAm J Pathol2005166254555515681837
- DhawanJRandoTAStem cells in postnatal myogenesis: molecular mechanisms of satellite cell quiescence, activation and replenishmentTrends Cell Biol2005151266667316243526
- KimCFJacksonELWoolfendenAEIdentification of bronchioalveolar stem cells in normal lung and lung cancerCell2005121682383515960971
- PeaultBRudnickiMTorrenteYStem and progenitor cells in skeletal muscle development, maintenance, and therapyMol Ther200715586787717387336
- SchafferABuchlerCConcise review: adipose tissue-derived stromal cells – basic and clinical implications for novel cell-based therapiesStem Cells200725481882717420225
- Van VlietPSluijterJPDoevendansPAGoumansMJIsolation and expansion of resident cardiac progenitor cellsExpert Rev Cardiovasc Ther200751334317187455
- BurnsCEZonLIPortrait of a stem cellDev Cell20023561261312431368
- BehfarAPerez-TerzicCFaustinoRSCardiopoietic programming of embryonic stem cells for tumor-free heart repairJ Exp Med2007204240542017283208
- BujanJPascualGCorralesCGomez-GilVGarcia-HonduvillaNBellonJMMuscle-derived stem cells used to treat skin defects prevent wound contraction and expedite reepithelializationWound Repair Regen200614221622316630112
- ChangYCShyuWCLinSZLiHRegenerative therapy for strokeCell Transplant200716217118117474298
- Gangaram-PandaySTFaasMMde VosPTowards stem-cell therapy in the endocrine pancreasTrends Mol Med200713416417317307397
- Gharaee-KermaniMGyetkoMRHuBPhanSHNew insights into the pathogenesis and treatment of idiopathic pulmonary fibrosis: a potential role for stem cells in the lung parenchyma and implications for therapyPharm Res200724581984117333393
- GimbleJMKatzAJBunnellBAAdipose-derived stem cells for regenerative medicineCirc Res200710091249126017495232
- GoldmanSADisease targets and strategies for the therapeutic modulation of endogenous neural stem and progenitor cellsClin Pharmacol Ther200782445346017713467
- MaYXuYXiaoZReconstruction of chemically burned rat corneal surface by bone marrow-derived human mesenchymal stem cellsStem Cells200624231532116109757
- McMullenNMPasumarthiKBDonor cell transplantation for myocardial disease: does it complement current pharmacological therapies?Can J Physiol Pharmacol200785111517487241
- MimeaultMBatraSKConcise review: recent advances on the significance of stem cells in tissue regeneration and cancer therapiesStem Cells200624112319234516794264
- OhSHWitekRPBaeSHBone marrow-derived hepatic oval cells differentiate into hepatocytes in 2-acetylaminofluorene/partial hepatectomy-induced liver regenerationGastroenterology200713231077108717383429
- SmallTNYoungJWCastro-MalaspinaHIntravenous busulfan and melphalan, tacrolimus, and short-course methotrexate followed by unmodified HLA-matched related or unrelated hematopoietic stem cell transplantation for the treatment of advanced hematologic malignanciesBiol Blood Marrow Transplant200713223524417241929
- TrzaskaKARameshwarPCurrent advances in the treatment of Parkinson’s disease with stem cellsCurr Neurovasc Res2007429910917504208
- AbdallahBMKassemMThe use of mesenchymal (skeletal) stem cells for treatment of degenerative diseases: current status and future perspectivesJ Cell Physiol2009218191218726996
- BarbaricIGokhalePJAndrewsPWHigh-content screening of small compounds on human embryonic stem cellsBiochem Soc Trans20103841046105020659001
- LaustriatDGideJPeschanskiMHuman pluripotent stem cells in drug discovery and predictive toxicologyBiochem Soc Trans20103841051105720659002
- YamanakaSInduction of pluripotent stem cells from mouse fibroblasts by four transcription factorsCell Prolif200841Suppl 1515618181945
- YamanakaSBlauHMNuclear reprogramming to a pluripotent state by three approachesNature2010465729970471220535199
- FierabracciARecent patents for isolating, delivering and tracking adult stem cells in regenerative medicineRecent Pat Drug Deliv Formul20104210511320156176
- MaltmanDJPrzyborskiSADevelopments in three-dimensional cell culture technology aimed at improving the accuracy of in vitro analysesBiochem Soc Trans20103841072107520659006
- DanoviDFalkAHumphreysPImaging-based chemical screens using normal and glioma-derived neural stem cellsBiochem Soc Trans20103841067107120659005
- KobelSLutolfMHigh-throughput methods to define complex stem cell nichesBiotechniques2010484ixxxii20569203
- BahadduriPMPolliJESwaanPWEkinsSTargeting drug transporters – combining in silico and in vitro approaches to predict in vivoMethods Mol Biol20106376510320419430
- IshizakiTStrategic proposals to avoid drug interactions during drug development: a lesson from a terfenadine-related drug interactionJ Toxicol Sci19962153013039035041
- RodenDMTorsade de pointesClin Cardiol19931696836867902224
- BradlawJAEvaluation of drug and chemical toxicity with cell culture systemsFundam Appl Toxicol1986645986063519343
- Gomez-LechonMJLahozAGombauLCastellJVDonatoMTIn vitro evaluation of potential hepatotoxicity induced by drugsCurr Pharm Des201016171963197720236064
- DonatoMTLahozACastellJVGomez-LechonMJCell lines: a tool for in vitro drug metabolism studiesCurr Drug Metab20089111118220566
- PolABergersMvan RuissenFPfundtRSchalkwijkJA simple technique for high-throughput screening of drugs that modulate normal and psoriasis-like differentiation in cultured human keratinocytesSkin Pharmacol Appl Skin Physiol200215425226112218287
- TremblayPLBerthodFGermainLAugerFAIn vitro evaluation of the angiostatic potential of drugs using an endothelialized tissue-engineered connective tissueJ Pharmacol Exp Ther2005315251051616055674
- NirmalanandhanVSSittampalamGSStem cells in drug discovery, tissue engineering, and regenerative medicine: emerging opportunities and challengesJ Biomol Screen200914775576819675315
- AugelloAKurthTBDe BariCMesenchymal stem cells: a perspective from in vitro cultures to in vivo migration and nichesEur Cell Mater20102012113321249629
- KeungAJHealyKEKumarSSchafferDVBiophysics and dynamics of natural and engineered stem cell microenvironmentsWiley Interdiscip Rev Syst Biol Med201021496420836010
- GardnerRLStem cells and regenerative medicine: principles, prospects and problemsC R Biol20073306–746547317631439
- GreenbergerJSGoffJPBushJExpansion of hematopoietic stem cells in vitro as a model system for human tissue engineeringOrthop Clin North Am200031349951010882474
- AnzaloneRLo IaconoMCorraoSNew emerging potentials for human Wharton’s jelly mesenchymal stem cells: immunological features and hepatocyte-like differentiative capacityStem Cells Dev201019442343819958166
- BarryFPMurphyJMMesenchymal stem cells: clinical applications and biological characterizationInt J Biochem Cell Biol200436456858415010324
- Beyer NardiNda Silva MeirellesLMesenchymal stem cells: isolation, in vitro expansion and characterizationHandb Exp Pharmacol200617424928216370331
- MinguellJJEricesACongetPMesenchymal stem cellsExp Biol Med (Maywood)2001226650752011395921
- TocciAForteLMesenchymal stem cell: use and perspectivesHematol J200342929612750726
- SinghSKClarkeIDHideTDirksPBCancer stem cells in nervous system tumorsOncogene200423437267727315378086
- ReyaTMorrisonSJClarkeMFWeissmanILStem cells, cancer, and cancer stem cellsNature2001414685910511111689955
- Al-HajjMBeckerMWWichaMWeissmanIClarkeMFTherapeutic implications of cancer stem cellsCurr Opin Genet Dev2004141434715108804
- BonnetDDickJEHuman acute myeloid leukemia is organized as a hierarchy that originates from a primitive hematopoietic cellNat Med1997377307379212098
- CrokerAKAllanALCancer stem cells: implications for the progression and treatment of metastatic diseaseJ Cell Mol Med200812237439018182063
- DalerbaPChoRWClarkeMFCancer stem cells: models and conceptsAnnu Rev Med20075826728417002552
- RiceKNJamiesonCHMolecular pathways to CML stem cellsInt J Hematol201091574875220533007
- RubnitzJEGibsonBSmithFOAcute myeloid leukemiaHematol Oncol Clin North Am2010241356320113895
- YamazakiHNishidaHIwataSDangNHMorimotoCCD90 and CD110 correlate with cancer stem cell potentials in human T-acute lymphoblastic leukemia cellsBiochem Biophys Res Commun2009383217217719341705
- BomkenSFiserKHeidenreichOVormoorJUnderstanding the cancer stem cellBr J Cancer2010103443944520664590
- BrownPSmithFOMolecularly targeted therapies for pediatric acute myeloid leukemia: progress to datePaediatr Drugs2008102859218345718
- MartinelliGIacobucciIPapayannidisCSoveriniSNew targets for Ph+ leukaemia therapyBest Pract Res Clin Haematol200922344545419959093
- LiZBaoSWuQHypoxia-inducible factors regulate tumorigenic capacity of glioma stem cellsCancer Cell200915650151319477429
- GuzmanMLRossiRMKarnischkyLThe sesquiterpene lactone parthenolide induces apoptosis of human acute myelogenous leukemia stem and progenitor cellsBlood2005105114163416915687234
- AmoreBMGibbsJPEmeryMGApplication of in vivo animal models to characterize the pharmacokinetic and pharmacodynamic properties of drug candidates in discovery settingsComb Chem High Throughput Screen201013220721820053166
- LeBaronMJRasoulpourRJKlapaczJEllis-HutchingsRGHollnagelHMGollapudiBBEpigenetics and chemical safety assessmentMutat Res20107052839520399890
- ShahAGarzon-MuvdiTMahajanRDuenasVJQuinones-HinojosaAAnimal models of neurological diseaseAdv Exp Med Biol2010671234020455493
- Guguen-GuillouzoCCorluAGuillouzoAStem cell-derived hepatocytes and their use in toxicologyToxicology201027013919815049
- DasTCaganRDrosophila as a novel therapeutic discovery tool for thyroid cancerThyroid201020768969520578898
- GiacomottoJSegalatLHigh-throughput screening and small animal models, where are we?Br J Pharmacol2010160220421620423335
- GosaiSJKwakJHLukeCJAutomated high-content live animal drug screening using C. elegans expressing the aggregation prone serpin alpha1-antitrypsin ZPloS One2010511e1546021103396
- SmithKARexEBKomunieckiRWAre Caenorhabditis elegans receptors useful targets for drug discovery: pharmacological comparison of tyramine receptors with high identity from C. elegans (TYRA-2) and Brugia malayi (Bm4)Mol Biochem Parasitol20071541526117537528
- LinkEMHardimanGSluderAEJohnsonCDLiuLXTherapeutic target discovery using Caenorhabditis elegansPharmacogenomics20001220321711256592
- PagliariniRAXuTA genetic screen in Drosophila for metastatic behaviorScience200330256481227123114551319
- PlowmanGDSudarsanamSBinghamJWhyteDHunterTThe protein kinases of Caenorhabditis elegans: a model for signal transduction in multicellular organismsProc Natl Acad Sci U S A19999624136031361010570119
- TickooSRussellSDrosophila melanogaster as a model system for drug discovery and pathway screeningCurr Opin Pharmacol20022555556012324259
- ShulmanJMShulmanLMWeinerWJFeanyMBFrom fruit fly to bedside: translating lessons from Drosophila models of neurodegenerative diseaseCurr Opin Neurol200316444344912869801
- BatesGPHocklyEExperimental therapeutics in Huntington’s disease: are models useful for therapeutic trials?Curr Opin Neurol200316446547012869804
- GunawardenaSHerLSBruschRGDisruption of axonal transport by loss of huntingtin or expression of pathogenic polyQ proteins in DrosophilaNeuron2003401254014527431
- ZambrowiczBPSandsATKnockouts model the 100 best-selling drugs – will they model the next 100?Nat Rev2003213851
- ZambrowiczBPTurnerCASandsATPredicting drug efficacy: knockouts model pipeline drugs of the pharmaceutical industryCurr Opin Pharmacol20033556357014559104
- SpradlingADrummond-BarbosaDKaiTStem cells find their nicheNature200141468599810411689954
- PieperAAXieSCapotaEDiscovery of a proneurogenic, neuroprotective chemicalCell20101421395120603013
- Furutani-SeikiMWittbrodtJMedaka and zebrafish, an evolutionary twin studyMech Dev20041217–862963715210172
- MitaniHKameiYFukamachiSThe medaka genome: why we need multiple fish models in vertebrate functional genomicsGenome Dyn2006216518218753778
- ScholzSMayerIMolecular biomarkers of endocrine disruption in small model fishMol Cell Endocrinol20082931–2577018619515
- KitambiSSMcCullochKJPetersonRTMalickiJJSmall molecule screen for compounds that affect vascular development in the zebrafish retinaMech Dev20091265–646447719445054
- SukardiHUngCYGongZLamSHIncorporating zebrafish omics into chemical biology and toxicologyZebrafish201071415220384484
- CurriePDSchillingTFInghamPWSmall-scale marker-based screening for mutations in zebrafish developmentMethods Mol Biol200846149351219030819
- HaffterPNusslein-VolhardCLarge scale genetics in a small vertebrate, the zebrafishInt J Dev Biol19964012212278735932
- BiecheleTLCampNDFassDMChemical-genetic screen identifies riluzole as an enhancer of Wnt/beta-catenin signaling in melanomaChem Biol201017111177118221095567
- ChiaNYChanYSFengBA genome-wide RNAi screen reveals determinants of human embryonic stem cell identityNature2010468732131632020953172
- JiangSQPaulusHA high-throughput, homogeneous, fluorescence polarization assay for inhibitors of hedgehog protein autoprocessingJ Biomol Screen20101591082108720930213
- LangDAHadleyDMTeasdaleGMMacphersonPTeasdaleEGadolinium-DTPA enhanced magnetic resonance imaging in human head injuryActa Neurochir (Wien)199051293295
- ThorneCAHansonAJSchneiderJSmall-molecule inhibition of Wnt signaling through activation of casein kinase 1alphaNat Chem Biol201061182983620890287
- RubinLLStem cells and drug discovery: the beginning of a new era?Cell2008132454955218295572
- JozefczukJPrigioneAChavezLAdjayeJComparative analysis of human embryonic stem cell and induced pluripotent stem cell-derived hepatocyte-like cells reveals current drawbacks and possible strategies for improved differentiationStem Cells Dev20112071259127521162674
- ParkIHLerouPHZhaoRHuoHDaleyGQGeneration of human-induced pluripotent stem cellsNat Protoc2008371180118618600223
- KanemuraYDevelopment of cell-processing systems for human stem cells (neural stem cells, mesenchymal stem cells, and iPS cells) for regenerative medicineKeio J Med2010592354520601839
- KnorrDAKaufmanDSPluripotent stem cell-derived natural killer cells for cancer therapyTransl Res2010156314715420801411
- SchwarzSCSchwarzJTranslation of stem cell therapy for neurological diseasesTransl Res2010156315516020801412
- VisvaderJELindemanGJCancer stem cells in solid tumours: accumulating evidence and unresolved questionsNat Rev2008810755768