Abstract
Continued improvements in the understanding and application of mesenchymal stem cells (MSC) have revolutionized tissue engineering. This is particularly true within the field of skeletal regenerative medicine. However, much remains unknown regarding the native origins of MSC, the relative advantages of different MSC populations for bone regeneration, and even the biologic safety of such unpurified, grossly characterized cells. This review will first summarize the initial discovery of MSC, as well as the current and future applications of MSC in bone tissue engineering. Next, the relative advantages and disadvantages of MSC isolated from distinct tissue origins are debated, including the MSC from adipose, bone marrow, and dental pulp, among others. The perivascular origin of MSC is next discussed. Finally, we briefly comment on pluripotent stem cell populations and their possible application in bone tissue engineering. While continually expanding, the field of MSC-based bone tissue engineering and regeneration shows potential to become a clinical reality in the not-so-distant future.
Introduction
Continued improvements in the understanding and application of adult mesenchymal stem cells (MSC) have revolutionized tissue engineering, particularly within the field of skeletal regenerative medicine. Although still in their relative infancy, multiple stem cell-based products have been used tentatively in humans for boneCitation1 and cartilageCitation2 regeneration. Furthermore, the delivery modality upon which these stem cell-based products are implanted is currently being investigated. To optimize bone healing, investigators must select a scaffold capable of maintaining, inducing, and restoring biological function. Scaffolds must be evaluated for their ability to not only maintain MSC survival, but also to promote MSC proliferation and differentiation. Moreover, such a scaffold must have appropriate degradation and nonimmunogeneic properties.Citation3 The currently studied in vivo scaffolds differ widely, encompassing organic autogenic, allogeneic, and demineralized bone matrix grafts, as well as synthetic polymers, bioactive ceramics, cross-linked hydrophilic hydrogels, metals, and composite scaffolds, which range from osteoinductive to relatively inert.Citation4,Citation5
The widespread clinical use of MSC, although not a present reality, is conversely not a distant possibility. This review will first summarize the discovery of MSC, their applications in bone tissue engineering, and drawbacks of various MSC populations. The perivascular origin of MSC will then be discussed. Subsequently, we will briefly discuss pluripotent stem cell populations and their possible application in bone tissue engineering, including embryonic and induced pluripotent stem cells. Nonetheless, despite the great progress made since the discovery and characterization of MSC, certain key areas of MSC identity, safety, and efficacy must be addressed before the clinical translation of MSC-based bone tissue engineering becomes a reality.
MSC defined
MSC are in vitro cultured cells capable of multilineage mesenchymal differentiation and self-renewal.Citation6,Citation7 In 2006, the Mesenchymal and Tissue Stem Cell Committee of the International Society for Cellular Therapy published the four minimal criteria that classify a cell as a MSC.Citation8 First, MSC are plastic-adherent when kept in standard culture conditions. Second, they must be multipotent stromal cells that can differentiate into osteoblasts, chondrocytes, and adipocytes. Third, they express the biomarkers CD73, CD90, and CD105. Finally, they must not express CD14, CD11b, CD34, CD45, CD19, and CD79 alpha.Citation8
It is interesting to note that differentiation down neurogenic or myogenic lineages is not necessarily required for MSC, as put forth by the Mesenchymal and Tissue Stem Cell Committee.Citation8 This qualification is consistent with the observed heterogeneity of the MSC populations isolated from different donors that contain subsets of cells that differ in terms of cell surface marker expression and transcriptional profiles, multidifferentiation potential, and clonogenicity.Citation9–Citation11 Moreover, others have protested this definition, stating that the unequivocal immunophenotyping hampers studies in other animal models due to the lack of a single specific marker and the limited availability of monoclonal antibodies.Citation12 Due to these issues, no unifying criteria have been uniformly embraced.
Despite the parameters set forth for the retrospective identification of MSC in culture, the in situ identity of MSC has long been elusive.Citation13 After the initial discovery of MSC in bone marrow, numerous other sources of MSC were identified, including tissues of fetal origin (umbilical cord, umbilical cord blood, or placenta), as well as diverse adult tissues (dermis, dental pulp, periosteum, blood vessels, synovial membrane, endometrium, and adipose tissue, among others) ().Citation14–Citation19 In fact, nearly every tissue type and organ has been cited as housing MSC-type cells, leading some to hypothesize that blood vessels themselves are the in situ origin for the MSC progenitor cellsCitation20 (see the “Perivascular stem cells [PSC]” section). Although MSC-type cells have been identified and isolated from various tissue sources using the same criteria, differences in therapeutic efficacy exist, which we will discuss in context with their tissue of origin.
Figure 1 Representative sites of MSC isolation.
Abbreviations: DMSC, dental pulp mesenchymal stem cells; FACS, fluorescence-activated cell sorting; PSC, perivascular stem cells; BMSC, bone marrow mesenchymal stem cells; ASC, adipose-derived stem cells; MSC, mesenchymal stem cell.
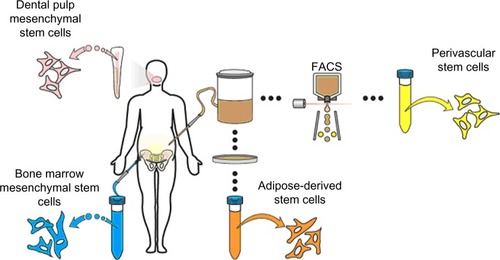
Bone marrow stromal cells (BMSC)
As demonstrated in a series of experiments performed in the mid-1960s, reviewed by Friedenstein et al, BMSC could be co-isolated in vitro alongside hematopoietic cells.Citation21 BMSC are classified as MSC; however, one must note that bone marrow consists of many stromal cells, which are not – and do not – give rise to MSC. Nonetheless, the purified BMSC are considered MSC as they abide by the 2006 criteria and exhibit both plasticity and the ability to differentiate into chondrocytes, osteocytes, and adipocytes in vitro and in vivo.Citation22,Citation23 In 1997, Komori et al discovered that some marrow stromal cells express the master osteogenic transcription factor, runt-related transcription factor 2 (Runx2).Citation24 Interestingly, regardless of differentiation, the BMSC retain Runx2 expression, suggesting an ability to shift phenotype and redifferentiate into osteoblasts.Citation25,Citation26
Since their discovery, the BMSC have been extensively studied from a preclinical perspective to investigate their role and osteogenic potential. BMSC in vivo studies have used a wide array of both organic materials (eg, bovine cancellous boneCitation27 and allogeneic bone graftsCitation28–Citation30) and inorganic materials (eg, macroporous calcium phosphate cement,Citation31 collagen sponge,Citation32 polylactic acid,Citation33 hydroxyapatite [HA],Citation28,Citation34 HA/tricalcium phosphate [TCP],Citation34,Citation35 and β-TCPCitation36), among others. Numerous small animal studies have revealed the ability for BMSC to induce the healing of critical-sized craniomaxillofacial defects in a murine model,Citation27,Citation31 repair critical-sized femoral defects in ratsCitation32 and rabbits,Citation33 and induce spinal fusion in rabbits.Citation28
Moreover, several investigators have also shown the efficacy of local implantation of BMSC in various large animal models, including maxillofacial regeneration,Citation37 spinal fusion,Citation27,Citation32 and in the healing of the critical-sized appendicular defects in dogs.Citation32,Citation35 Similar studies have been performed in sheep and have demonstrated the efficacy of BMSC to promote posterolateral spinal fusion,Citation34 as well as repair long bone defects.Citation29,Citation30 In 2008, Gan et alCitation36 translated these preclinical findings into a clinical setting and demonstrated the efficacy of BMSC to induce posterolateral spinal fusion in humans. Thus, BMSC have shown immense potential in multiple small and large animal studies and in a wide range of medical applications involving fracture and bone reconstruction.
In a clinical setting, however, the therapeutic use of MSC is hindered by several drawbacks that include the low yield of BMSC procurement, the use of growth factors and extensive in vitro culture for their expansion to therapeutically useful levels, and the inherent heterogeneity they display in their multidifferentiation potential. Because of the extremely low yields of BMSC progenitors (typically 0.001%–0.01%) obtained from bone marrow aspirates, large quantities of bone marrow must be procured, which can cause additional donor site morbidity.Citation38
Moreover, BMSC require several passages in culture to purify the original heterogeneous population comprised of hematopoietic and other cell types.Citation39 When poorly purified from phenotypically different cells, BMSC possess variable morphology, a limited capacity for self-renewal, and are less efficiently differentiated.Citation40,Citation41 Last, the conditions and duration of culture can also alter their differentiation potential, as can the growth factors that are often used to promote the proliferation of BMSC.Citation42–Citation44 These significant limitations have hindered the translation of BMSC into a clinical setting, leading investigators to seek alternative tissue sources from which to isolate MSC.
Even with seemingly pure preparations of BMSC, only a percentage of BMSC in the population are susceptible to osteogenesis.Citation45 In clonal studies characterizing adipogenic, osteogenic, and chondrogenic potentials, three categories comprised of four subgroups were shown to account for ~90% of all colony-forming MSC in healthy donors: tripotent; bipotent (both osteoadipogenic and osteochondrogenic); and unipotent (osteogenic) colonies.Citation11 Tripotent cells, which constituted ~50% of the clones in this study, were highly proliferative, possessed large colony-forming efficiencies (35%–90%), and accumulated a more highly mineralized extracellular matrix than did cells from unipotent osteogenic clones, which formed colonies 5% or less of the time.Citation46 Other research groups have enumerated this tripotent fraction of BMSC-derived clones at between 7%–13.3%, and in the latter case, have exhibited an associated expression of the surface marker CD271 (the low-affinity nerve growth factor receptor, a p75 neurotrophin receptor) with tripotent clones.Citation47,Citation48 Although specific estimates as to the number of tripotent cells BMSC vary, it is obvious that unfractioned BMSC expanded in culture exhibit variable functional efficacy.
Adipose-derived stem cells (ASC)
An alternative site for MSC isolation is adipose tissue. ASC, initially termed processed lipoaspirate, were first discovered in 2001 by Zuk et alCitation49 and have been extensively evaluated for tissue engineering – in particular, for bone formation. Abundant in supply and readily and safely accessible, subcutaneous fat obtained via routine liposuction can be digested with collagenase immediately postisolation to produce a mixed-cell homogenate termed the stromal vascular fraction (SVF), which contains ASC in addition to endothelial, hematopoietic, and stromal cells.Citation50,Citation51 Although SVF has been studied in the confines of bone biology, the cells yield poor bone formation upon implantation, presumably due to the presence of mixed stromal and endothelial cells that both dilute and interact with the ASC present in the SVF.Citation52 Fortunately, MSC may be produced by culturing the SVFs that are more homogenous for expression of the cell markers CD73, CD90, CD44, and CD9.Citation53 Following flow cytometry characterization, it was determined that adipose stem cells also express the progenitor cell marker CD34 and secrete vascular endothelial growth factor, in addition to many other trophic factors.Citation50,Citation51,Citation54 Upon allocation for culture, ASC have been shown to possess trilineage potential and to undergo osteogenic differentiation when treated with ascorbic acid, bone morphogenetic protein-2, and β-glycerophosphate.Citation55
Like BMSC, population heterogeneity is also exhibited by ASC (selected by adherence to plastic). In aggregate, ASC represent a significant stem cell population, as they are abundant in supply and can be easily procured and isolated. However, at the clonal level, only 21% of the population of plastic-adherent ASC clones was determined to be tripotent, with an additional 31% and 29% possessing bipotent and unipotent behavior, respectively.Citation56 Interestingly, only 48% of the clones were osteogenic, which suggests that the surface marker prognostic for osteogenic potency would improve the efficacy and consistency of these cells for bone tissue engineering.
The immense osteogenic potential of ASC has been demonstrated in numerous preclinical animal studies. In small animal models, ASC have been demonstrated to repair critical-sized calvarial defects,Citation57,Citation58 to promote bone formation in appendicular defects,Citation59–Citation61 and to induce spinal fusion in murine models.Citation62 Similarly, in a large animal canine model, implanted ASC were observed to promote bone formation for craniomaxillofacial repair.Citation63 A wide variety of scaffolds have been used in ASC-mediated bone repair, such as organic apatite-coated poly(lactic-co-glycolic) acid scaffolds,Citation57,Citation58 collagen-ceramic carriers,Citation60 type I collagen matrix,Citation62 and coral scaffolds,Citation63 among others. Thus, ASC have been validated to possess significant efficacy and consistent osteogenic potential, as witnessed in various small and large animal models.
Although robust quantities of bone are induced when ASC are implanted in vivo, the SVF must be passaged several times in culture to reduce ASC contamination by other cell types present in the SVF, which precludes their use at the point of care. Furthermore, the in vitro cultivation has been shown to decrease stemness, self-renewal, and multipotencyCitation64 and, in some cases, has resulted in genomic instability – a potential concern for spontaneous malignant transformation.Citation65 The proliferative capacity of ASC is also inversely correlated with patient age – a significant drawback for applications involving geriatric and osteoporotic patients.Citation66
Although also observed in other MSC populations, the ASC have been reported to release trophic factors, such as angiopoietin-like 1, EGF, FGF, HGF, TGFβ, and CXCL12, as well as the immunosuppressive cytokines prostaglandin E2 (PGE2) and indoleamine 2,3-dioxygenase (IDO).Citation67–Citation70 While growth and stem cell recruitment factors stimulate tissue regeneration, the immunosuppressive properties of these cells suggest that either allogeneic or autologous ASC can be successfully used in tissue repair without triggering a lymphocyte reaction.Citation71 Further work, however, must be done to investigate whether an immune reaction may be triggered when ASC differentiate. This dual mitogenic/immunomodulatory potential of ASC, however, may have a dark side, as it may stimulate cancer cell proliferation, while at the same time diminish T-cell proliferation, which could have taken part in a cytotoxic, antineoplastic response.Citation72–Citation79 Furthermore, mechanisms, such as the ASC-mediated secretion of CXCL12 and FGFR2, have been shown to increase tumor cell migration and angiogenesis, respectively.Citation66,Citation80 Thus, ASC may not be the best choice for bone reconstruction after tumor resection. Overall, while ASC have significant advantages in their relative abundance and bone-forming potential, culture requirement and trophic factor elaboration remain safety concerns for their future use.
Perivascular stem cells (PSC)
As MSC are consistently isolated from tissues rich in vasculature, such as bone marrow and adipose tissue, we and other investigators have recently sought to determine whether MSC ancestors are natively associated with blood vessels. In 2008, the pericytes, which line capillaries and microvasculature, were discovered to possess multipotent differentiation potential.Citation51,Citation81 This led Professor Arnold Caplan of Case Western Reserve University to speculate and propose that all MSC are, in fact, derived from pericytes.Citation20 In 2012, Corselli et alCitation82 reported that in addition to MSC being derived from pericytes, adventitial cells could also give rise to MSC. Adventitial cells are a population of CD34+, multipotent cells that are antigenically distinct to pericytes and are observed to mobilize in response to trauma and hypoxia.Citation82–Citation85 In a recent article by Zhao et al,Citation86 it was demonstrated that during incisor trauma, periarterial cells (pericytes and adventitial cells) are recruited to modulate hemostasis and repair. Further, in vitro, these cells were shown to exhibit typical MSC characteristics.Citation86 Thus, when taken together, pericytes (CD31−/CD45−/CD34−/CD146+), found encompassing microvessels and capillaries, and adventitial cells (CD31−/CD45−/CD34+/CD146−), localized in the outmost layer of larger arteries and veins, compose PSC.Citation87
As adipose is a highly vascularized tissue and is ample in supply, most PSC safety and efficacy studies have isolated PSC from routine liposuction procedures. Unlike plastic-selected ASC, which require weeks of culture, pericytes (CD45−/CD146−/CD34+) and adventitial cells (CD45−/CD146+/CD34−) are purified via fluorescent-activated cell sorting (FACS) in a process that requires merely a few hours ().Citation87,Citation88 Moreover, the PSC obtained from fat are superior to BMSC and dental pulp mesenchymal stem cells (DMSC), as they can be procured in abundance with minimal patient morbidity. Further, as the PSC are purified via FACS instead of being cultured, and since they are autologous, the minimal risk of in vitro transformation and immunogeneic rejection exists. Thus, PSC are a novel and promising stem cell population shown to possess trilineage potential and are superior to the aforementioned stem cell populations, as they can be isolated in large quantities and can be purified in a relatively short time.
Figure 2 Purification process for PSC.
Abbreviations: FACS, fluorescence-activated cell sorting; PSC, perivascular stem cells; SVF, stromal vascular fraction.
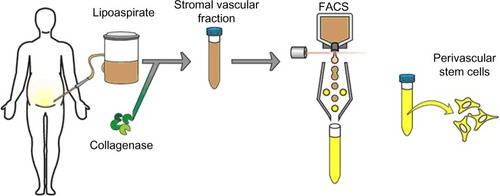
Since their discovery, PSC have been studied in various preclinical models to investigate their osteogenic potential. PSC have been delivered on demineralized bone matrixCitation89–Citation91 or via HA-coated poly(lactic-co-glycolic) acid.Citation92 Human PSC significantly improved healing in a mouse critical-size calvarial defect model when compared to human SVF (ie, SVF or unpurified lipoaspirates).Citation92 Moreover, the PSC also induced robust bone formation following implantation in a murine muscle pouch model and femoral segmental defect model.Citation91 In a recent study, PSC implantation induced successful lumbar spinal fusion in rats, with markedly increased bone formation.Citation89 Samples implanted with PSC expressed increased bone stiffness and capacity to withstand force via finite elemental analysis – a nondestructive biomechanical assay.Citation90 Thus, PSC combine two separately studied perivascular stem cell populations that can be readily isolated to homogeneity from lipoaspirates via FACS, whose capability has been validated in the induction of robust osteogenesis.
Dental pulp mesenchymal stem cells
MSC have also been purified from human exfoliated deciduous teeth, and termed DMSC.Citation93 In 2003, Shi and GronthosCitation94 successfully utilized the antibody STRO-1 to probe and isolate dental pulp stem cells through immunoselection. Through immunohistochemistry, FACS, and immunomagnetic bead selection, Shi and GronthosCitation94 determined that dental pulp stem cells express the pericyte markers CD146 and 3G5. This interesting observation brings up the question as to the overlapping identity of DMSC and PSC.
The preclinical studies of DMSC have shown some promise in bone biology. Several small animal studies have demonstrated the potential of DMSC in the regeneration of critical-sized calvarial defects in mice.Citation95,Citation96 For instance, MSC derived from the periodontal ligament and gingival tissue possess the ability to repair critical-size calvarial defects in mice by promoting the formation of mineralized tissue on arginine–glycine–aspartic acid tripeptide-modified alginate scaffolds, as well as on gelatin sponge scaffolds.Citation95,Citation96 In canine and swine models, the local implantation of DMSC with β-TCP and HA/TCP scaffolds has been shown to induce successful bone regeneration in critical-sized orofacial bone defects.Citation97,Citation98 Although less efficacious than BMSC, the DMSC were able to regenerate alveolar bone at 8 weeks postimplantation in a canine model.Citation98 In fact, DMSC have also been shown to repair alveolar ridge and mandibular defects in humans, utilizing collagen sponge scaffolds.Citation99,Citation100
While dental pulp might pose as an alternative site for MSC isolation, the use of DMSC is limited by the small quantity of pulp in a tooth, the proportionally smaller DMSC yield that necessitates their expansion by long-term culture, and the corresponding costs and risks that are incurred. Aside from the pragmatic concerns, several investigators have also reported the limited potential of DMSC to regenerate bone in vivo.Citation101–Citation105 For instance, while DMSC were observed to promote bone formation in a human mandibular defect, the de novo bone formed was primarily composed of cortical bone, lacking vasculature.Citation100 Moreover, in a manuscript by Annibali et al,Citation101 DMSC did not significantly induce more bone than did controls in a critical-sized calvarial defect in mice. This observation may be a consequence of the fact that as DMSC undergo high-passage propagation, fewer and fewer stem cells are present.Citation106 So, despite the fact that DMSC show some utility for bone regeneration, they do not supplant therapies enlisting BMSC or PSC.
Embryonic stem cells (ESC)
Several investigators have also explored the regenerative potential of ESC for applications involving bone. The ESC are a pluripotent stem cell population which, by definition, possess an unlimited potential to differentiate into all three germ tissue lineages: ectoderm; endoderm; and mesoderm. An unlimited capacity for self-renewal is highly valuable for continued research and affords ESC a considerable advantage for regenerative medicine and the consistent manufacture of an allogeneic product for bone repair. In this context, ESC have been studied in various preclinical assays to investigate their innate osteogenic potential as well as their influence in coculture with other stem cells in the presence of growth factors. Alone, ESC have been observed to possess robust osteogenic potential and are capable of osteogenic differentiation with or without the stage-specific changes that occur in the normal development of the embryoid body.Citation107 When cocultured with human BMSC that had undergone osteogenesis, the bone nodules formed from human ESC (hESC) were more mineralized and were richer in osteocalcin, an extracellular bone matrix protein. These findings suggest that paracrine factors secreted from differentiated human BMSC (hBMSC) promote osteogenic differentiation in hESC.Citation108 Independently, when ESC are treated with numerous growth factors, a synergistic effect is observed where osteogenic differentiation of hESC is enhanced compared to using a single growth factor.Citation109
From these studies, it appears that the extracellular matrix plays a crucial role in contributing to osteogenic lineage specification during embryonic development;Citation110–Citation112 thus, in order to successfully optimize ESC for bone regeneration, scaffolds must be implemented that mimic the natural extracellular matrix of bone tissue and act as a directive template for the osteogenic specification of ESC.Citation113
Major obstacles exist, however, in realizing the translational potential of ESC, including allogeneic rejection and teratoma formation. Previous investigators have witnessed allogeneic rejection upon implantation, with marked rejection of donor cells possessing foreign human leukocyte antigens.Citation114 In a recent study by Aldahmash et al,Citation115 it was observed that the implantation of undifferentiated ESC induced teratoma formation, a tumor comprised of all three cell lineages. As such, the punctilious removal of any undifferentiated cell is imperative prior to administration. Although efficacy studies have shown promise in the osteogenic potential of ESC, safety issues limit the potential clinical use of ESC for bone tissue engineering.
Induced pluripotent stem cells (iPSC)
In 2006, the iPSC were created in Shinya Yamanaka’s laboratory at Kyoto University from mouse fibroblasts by the retroviral ectopic transduction of the genes Oct4, Sox2, Klf4, and c-Myc.Citation116 Although most commonly derived from primary dermal fibroblasts, iPSC can be created from many different adult cell lineages via their cellular reprogramming to a pluripotent state.Citation117–Citation119 Because iPSC possess a pluripotent potential similar to that of ESC, but they are derived from the cells of the adult patient undergoing treatment, they do not generate an allograft immune response.Citation120
In a preclinical setting, iPSC have been observed to promote robust bone regeneration both in vitro and in vivo. In fact, in a 2010 study by Kao et al,Citation121 the iPSC were observed to possess comparable osteogenic potential to that of ESC. Implantation studies have demonstrated the potential of iPSC to promote bone formation in a mouse knee defect as well as in the murine ectopic subcutaneous injection models, utilizing collagen hydrogen scaffolds and macrochanneled poly(caprolactone) scaffolds.Citation122,Citation123 Furthermore, in 2012, Levi et alCitation124 demonstrated complete healing of critical-sized calvarial defects in mice implanted with human iPSC. Unfortunately, like ESC, the iPSC that have not been thoroughly differentiated prior to implantation can undergo teratoma formation.Citation116,Citation125 Although the frequency of teratoma formation with iPSC is not as prevalent as with ESC, the presence of teratomas, again, shows the instability of pluripotent stem cell application in bone and tissue engineering.
Another major drawback of iPSC is their poor yield of induction; <1% success was observed in the induction of iPSC from murine adult somatic cells. As such, clinical translation is rendered difficult due to the necessity of starting with large quantities of tissue.Citation126,Citation127
Conclusion
In conclusion, although several MSC populations hold great promise in bone-forming potential, the clinical application will require that the identity, safety, and efficacy of these cells be well validated.Citation128 Currently, the very native identity of MSC is ensconced in some mystery and remains a controversial subject with different investigators isolating MSC via differing techniques (eg, selection by culture versus immunophenotype) or establishing MSC identity by different parameters. In addition, the perivascular/pericytic origin of MSC is becoming an increasingly well-accepted theory, although complete agreement as to the origin, function, and clinical potential of these cells is lacking, as are the changes that these cells undergo during isolation, cultivation, and reintroduction to the host. In terms of safety, tumor formation and malignant transformation of MSC remain a possible risk,Citation129 although most studies suggest that this is a theoretical rather than a tangible risk. On the other hand, ESC and iPSC, while capable of bone formation, possess tumorigenic potential by forming teratomas. Finally, in terms of efficacy, the question remains as to whether different MSC depots or subpopulations are more osteogenic than others. Comparisons have been made across MSC obtained from donors of different ages and sexes, and from different anatomic locations and tissues, using different cell surface markers for identification. Yet, an unequivocal cell source (or the gold standard) for MSC-based bone tissue engineering and regeneration has not yet been defined. By utilizing a prospectively purified, noncultured population of stem cells, such as PSC, future studies can alleviate the number of drawbacks associated with the utilization of long-term cultured convention MSC. Thus, despite the great progress made since the discovery and characterization of MSC, certain key areas of MSC identity, safety, and efficacy must be addressed before the clinical translation of MSC-based bone tissue engineering becomes a reality.
Disclosure
The authors report no conflicts of interest in this work.
References
- KnightMNHankensonKDMesenchymal stem cells in bone regenerationAdv Wound Care (New Rochelle)20132630631624527352
- DengSHuangRWangJMiscellaneous animal models accelerate the application of mesenchymal stem cells for cartilage regenerationCurr Stem Cell Res Ther20149322323324524791
- BillströmGHBlomAWLarssonSBeswickADApplication of scaffolds for bone regeneration strategies: current trends and future directionsInjury201344Suppl 1S28S3323351866
- YarlagaddaPKChandrasekharanMShyanJYRecent advances and current developments in tissue scaffoldingBiomed Mater Eng200515315917715911997
- TevlinRMcArdleAAtashrooDBiomaterials for Craniofacial Bone EngineeringJ Dent Res2014
- OswaldJBoxbergerSJørgensenBMesenchymal stem cells can be differentiated into endothelial cells in vitroStem Cells200422337738415153614
- BiancoPRobeyPGSimmonsPJMesenchymal stem cells: revisiting history, concepts, and assaysCell Stem Cell20082431331918397751
- DominiciMLe BlancKMuellerIMinimal criteria for defining multipotent mesenchymal stromal cells. The International Society for Cellular Therapy position statementCytotherapy20068431531716923606
- MosnaFSensebéLKramperaMHuman bone marrow and adipose tissue mesenchymal stem cells: a user’s guideStem Cells Dev201019101449147020486777
- WagnerWFeldmannREJrSeckingerAThe heterogeneity of human mesenchymal stem cell preparations – evidence from simultaneous analysis of proteomes and transcriptomesExp Hematol200634453654816569600
- RussellKCPhinneyDGLaceyMRBarrilleauxBLMeyertholenKEO’ConnorKCIn vitro high-capacity assay to quantify the clonal heterogeneity in trilineage potential of mesenchymal stem cells reveals a complex hierarchy of lineage commitmentStem Cells201028478879820127798
- De SchauwerCMeyerEVan de WalleGRVan SoomAMarkers of stemness in equine mesenchymal stem cells: a plea for uniformityTheriogenology20117581431144321196039
- da Silva MeirellesLCaplanAINardiNBIn search of the in vivo identity of mesenchymal stem cellsStem Cells20082692287229918566331
- PojdaZMachajEKurzykAMesenchymal stem cellsPostepy Biochemi2013592187197 Polish
- YoungHESteeleTABrayRAHuman reserve pluripotent mesenchymal stem cells are present in the connective tissues of skeletal muscle and dermis derived from fetal, adult, and geriatric donorsAnat Rec20012641516211505371
- NakaharaHGoldbergVMCaplanAICulture-expanded human periosteal-derived cells exhibit osteochondral potential in vivoJ Orthop Res1991944654762045973
- DiefenderferDLBrightonCTMicrovascular pericytes express aggrecan message which is regulated by BMP-2Biochem Biophys Res Commun2000269117217810694496
- De BariCDell’AccioFLuytenFPHuman periosteum-derived cells maintain phenotypic stability and chondrogenic potential throughout expansion regardless of donor ageArthritis Rheum2001441859511212180
- SpitzerTLRojasAZelenkoZPerivascular human endometrial mesenchymal stem cells express pathways relevant to self-renewal, lineage specification, and functional phenotypeBiol Reprod20128625822075475
- CaplanAIAll MSCs are pericytes?Cell Stem Cell20083322923018786406
- FriedensteinAJOsteogenic stem cells in bone marrowHeerscheJNMKanisJABone and Mineral ResearchAmsterdamElsevier1990243272
- BennettJHJoynerCJTriffittJTOwenMEAdipocytic cells cultured from marrow have osteogenic potentialJ Cell Sci199199Pt 11311391757497
- BeresfordJNBennettJHDevlinCLeboyPSOwenMEEvidence for an inverse relationship between the differentiation of adipocytic and osteogenic cells in rat marrow stromal cell culturesJ Cell Sci1992102Pt 23413511400636
- KomoriTYagiHNomuraSTargeted disruption of Cbfa1 results in a complete lack of bone formation owing to maturational arrest of osteoblastsCell19978957557649182763
- GalottoMCampanileGRobinoGCanceddaFDBiancoPCanceddaRHypertrophic chondrocytes undergo further differentiation to osteoblast-like cells and participate in the initial bone formation in developing chick embryoJ Bone Miner Res199498123912497976506
- GentiliCBiancoPNeriMCell proliferation, extracellular matrix mineralization, and ovotransferrin transient expression during in vitro differentiation of chick hypertrophic chondrocytes into osteoblast-like cellsJ Cell Biol199312237037128393014
- ZhaoJYangCSuCReconstruction of orbital defects by implantation of antigen-free bovine cancellous bone scaffold combined with bone marrow mesenchymal stem cells in ratsGraefes Arch Clin Exp Ophthalmol201325151325133323519882
- MinamideAYoshidaMKawakamiMThe use of cultured bone marrow cells in type I collagen gel and porous hydroxyapatite for posterolateral lumbar spine fusionSpine (Phila Pa 1976)200530101134113815897826
- FernandesMBGuimarãesJACasadoPLThe effect of bone allografts combined with bone marrow stromal cells on the healing of segmental bone defects in a sheep modelBMC Vet Res2014103624495743
- VélezRHernández-FernándezACaminalMTreatment of femoral head osteonecrosis with advanced cell therapy in sheepArch Orthop Trauma Surg2012132111611161822821379
- ChenWLiuJManuchehrabadiNWeirMDZhuZXuHHUmbilical cord and bone marrow mesenchymal stem cell seeding on macroporous calcium phosphate for bone regeneration in rat cranial defectsBiomaterials201334389917992524054499
- RoseTPengHShenHCThe role of cell type in bone healing mediated by ex vivo gene therapyLangenbecks Arch Surg2003388534735514534793
- YueyiCXiaoguangHJingyingWCalvarial defect healing by recruitment of autogenous osteogenic stem cells using locally applied simvastatinBiomaterials201334379373938024016857
- ShamsulBSTanKKChenHCAminuddinBSRuszymahBHPosterolateral spinal fusion with ostegenesis induced BMSC seeded TCP/HA in a sheep modelTissue Cell201446215215824630213
- ArinzehTLPeterSJArchambaultMPAllogeneic mesenchymal stem cells regenerate bone in a critical-sized canine segmental defectJ Bone Joint Surg Am200385-A101927193514563800
- GanYDaiKZhangPTangTZhuZLuJThe clinical use of enriched bone marrow stem cells combined with porous beta-tricalcium phosphate in posterior spinal fusionBiomaterials200829293973398218639333
- LiuXLiaoXLuoEChenWBaoCXuHHMesenchymal stem cells systemically injected into femoral marrow of dogs home to mandibular defects to enhance new bone formationTissue Eng Part A2014203–488389224125551
- BonabMMAlimoghaddamKTalebianFGhaffariSHGhavamzadehANikbinBAging of mesenchymal stem cell in vitroBMC Cell Biol200671416529651
- IzadpanahRTryggCPatelBBiologic properties of mesenchy-mal stem cells derived from bone marrow and adipose tissueJ Cell Biochem20069951285129716795045
- IzadpanahRKaushalDKriedtCLong-term in vitro expansion alters the biology of adult mesenchymal stem cellsCancer Res200868114229423818519682
- WagnerWHornPCastoldiMReplicative senescence of mesenchymal stem cells: a continuous and organized processPLoS One200835e221318493317
- ShoshaniOZiporiDMammalian cell dedifferentiation as a possible outcome of stressStem Cell Rev20117348849321279479
- BianchiGBanfiAMastrogiacomoMEx vivo enrichment of mesenchymal cell progenitors by fibroblast growth factor 2Exp Cell Res200328719810512799186
- SotiropoulouPAPerezSASalagianniMBaxevanisCNPapamichailMCharacterization of the optimal culture conditions for clinical scale production of human mesenchymal stem cellsStem Cells200624246247116109759
- PhinneyDGFunctional heterogeneity of mesenchymal stem cells: implications for cell therapyJ Cell Biochem201211392806281222511358
- RussellKCLaceyMRGilliamJKTuckerHAPhinneyDGO’ConnorKCClonal analysis of the proliferation potential of human bone marrow mesenchymal stem cells as a function of potencyBiotechnol Bioeng2011108112716272621538337
- KuçiZSeiberthJLatifi-PupovciHClonal analysis of multipotent stromal cells derived from CD271+ bone marrow mononuclear cells: functional heterogeneity and different mechanisms of allosuppressionHaematologica201398101609161623975178
- LeeCCChristensenJEYoderMCTarantalAFClonal analysis and hierarchy of human bone marrow mesenchymal stem and progenitor cellsExp Hematol2010381465419900502
- ZukPAZhuMMizunoHMultilineage cells from human adipose tissue: implications for cell-based therapiesTissue Eng20017221122811304456
- RehmanJTraktuevDLiJSecretion of angiogenic and antiapoptotic factors by human adipose stromal cellsCirculation2004109101292129814993122
- TraktuevDOMerfeld-ClaussSLiJA population of multipotent CD34-positive adipose stromal cells share pericyte and mesenchymal surface markers, reside in a periendothelial location, and stabilize endothelial networksCirc Res20081021778517967785
- JamesAWZaraJNZhangXPerivascular stem cells: a prospectively purified mesenchymal stem cell population for bone tissue engineeringStem Cells Transl Med20121651051923197855
- HamidAAIdrusRBSaimABSathappanSChuaKHCharacterization of human adipose-derived stem cells and expression of chondrogenic genes during induction of cartilage differentiationClinics (Sao Paulo)20126729910622358233
- CaplanAICorreaDThe MSC: an injury drugstoreCell Stem Cell201191111521726829
- SchäfflerABüchlerCConcise review: adipose tissue-derived stromal cells – basic and clinical implications for novel cell-based therapiesStem Cells200725481882717420225
- GuilakFLottKEAwadHAClonal analysis of the differentiation potential of human adipose-derived adult stem cellsJ Cell Physiol2006206122923716021633
- CowanCMShiYYAalamiOOAdipose-derived adult stromal cells heal critical-size mouse calvarial defectsNat Biotechnol200422556056715077117
- LeviBJamesAWNelsonERHuman adipose derived stromal cells heal critical size mouse calvarial defectsPLoS One201056e1117720567510
- AbudusaimiAAihemaitijiangYWangYHCuiLMaimaitimingSAbulikemuMAdipose-derived stem cells enhance bone regeneration in vascular necrosis of the femoral head in the rabbitJ Int Med Res20113951852186022117986
- PetersonBZhangJIglesiasRHealing of critically sized femoral defects, using genetically modified mesenchymal stem cells from human adipose tissueTissue Eng2005111–212012915738667
- SunayOCanGCakirZAutologous rabbit adipose tissue-derived mesenchymal stromal cells for the treatment of bone injuries with distraction osteogenesisCytotherapy201315669070223522867
- HsuWKWangJCLiuNQStem cells from human fat as cellular delivery vehicles in an athymic rat posterolateral spine fusion modelJ Bone Joint Surg Am20089051043105218451397
- CuiLLiuBLiuGRepair of cranial bone defects with adipose derived stem cells and coral scaffold in a canine modelBiomaterials200728365477548617888508
- RequichaJFViegasCAAlbuquerqueCMAzevedoJMReisRLGomesMEEffect of anatomical origin and cell passage number on the stemness and osteogenic differentiation potential of canine adipose-derived stem cellsStem Cell Rev2012841211122222773405
- BellottiCStancoDRagazziniSAnalysis of the karyotype of expanded human adipose-derived stem cells for bone reconstruction of the maxillo-facial regionInt J Immunopathol Pharmacol201326Suppl 13924046944
- GuercioADi BellaSCasellaSDi MarcoPRussoCPiccioneGCanine mesenchymal stem cells (MSCs): characterization in relation to donor age and adipose tissue-harvesting siteCell Biol Int201337878979823505013
- De MiguelMPFuentes-JuliánSBlázquez-MartínezAImmunosuppressive properties of mesenchymal stem cells: advances and applicationsCurr Mol Med201212557459122515979
- LeeSHJinSYSongJSSeoKKChoKHParacrine effects of adipose-derived stem cells on keratinocytes and dermal fibroblastsAnn Dermatol201224213614322577262
- MoonKMParkYHLeeJSThe effect of secretory factors of adipose-derived stem cells on human keratinocytesInt J Mol Sci20121311239125722312315
- NakaoNNakayamaTYahataTAdipose Tissue-Derived Mesenchymal Stem Cells Facilitate Hematopoiesis in Vitro and in VivoAm J Pathol2010177254755420558580
- GimbleJMGraysonWGuilakFLopezMJVunjak-NovakovicGAdipose tissue as a stem cell source for musculoskeletal regenerationFront Biosci (Schol Ed)20113698121196358
- Ostrand-RosenbergSMyeloid-derived suppressor cells: more mechanisms for inhibiting antitumor immunityCancer Immunol Immunother201059101593160020414655
- Carrade HoltDDWoodJAGranickJLWalkerNJClarkKCBorjessonDLEquine mesenchymal stem cells inhibit T cell proliferation through different mechanisms depending on tissue sourceStem Cells Dev201423111258126524438346
- KimJHLeeYTHongJMHwangYISuppression of in vitro murine T cell proliferation by human adipose tissue-derived mesenchymal stem cells is dependent mainly on cyclooxygenase-2 expressionAnat Cell Biol201346426227124386599
- MohammadzadehAPourfathollahAAShahrokhiSHashemiSMMoradiSLSoleimaniMImmunomodulatory effects of adipose-derived mesenchymal stem cells on the gene expression of major transcription factors of T cell subsetsInt Immunopharmacol201420231632124704622
- DjouadFPlencePBonyCImmunosuppressive effect of mesenchymal stem cells favors tumor growth in allogeneic animalsBlood2003102103837384412881305
- CuiffoBGKarnoubAEMesenchymal stem cells in tumor development: emerging roles and conceptsCell Adh Migr20126322023022863739
- KarnoubAEDashABVoAPMesenchymal stem cells within tumour stroma promote breast cancer metastasisNature2007449716255756317914389
- KarnoubAEWeinbergRAChemokine networks and breast cancer metastasisBreast Dis200626758517473367
- MuehlbergFLSongYHKrohnATissue-resident stem cells promote breast cancer growth and metastasisCarcinogenesis200930458959719181699
- CrisanMYapSCasteillaLA perivascular origin for mesenchymal stem cells in multiple human organsCell Stem Cell20083330131318786417
- CorselliMChenCWSunBYapSRubinJPPéaultBThe tunica adventitia of human arteries and veins as a source of mesenchymal stem cellsStem Cells Dev20122181299130821861688
- LinGGarciaMNingHDefining stem and progenitor cells within adipose tissueStem Cells Dev20081761053106318597617
- SiowRCMallawaarachchiCMWeissbergPLMigration of adventitial myofibroblasts following vascular balloon injury: insights from in vivo gene transfer to rat carotid arteriesCardiovasc Res200359121222112829192
- CorselliMChenCWCrisanMLazzariLPéaultBPerivascular ancestors of adult multipotent stem cellsArterioscler Thromb Vasc Biol20103061104110920453168
- ZhaoHFengJSeidelKSecretion of shh by a neurovascular bundle niche supports mesenchymal stem cell homeostasis in the adult mouse incisorCell Stem Cell201414216017324506883
- CorselliMCrisanMMurrayIRIdentification of perivascular mesenchymal stromal/stem cells by flow cytometryCytometry A201383871472023818229
- AskarinamAJamesAWZaraJNHuman perivascular stem cells show enhanced osteogenesis and vasculogenesis with Nel-like molecule I proteinTissue Eng Part A20131911–121386139723406369
- AsatrianGCCJamesAWLiangPHuman Perivascular Mesenchymal Stem cells Promote Lumbar Spinal Fusion Via Induction of Osteogenesis and VasculogenesisSeattle, WAInternational Association for Dental Research2013
- ChungCG KJVelascoOAsatrianGPerivascular Stem Cells with NELL-1 Protein Induce Robust Spinal FusionCharlotte, NCAmerican Association of Dental Research2014
- JamesAWZaraJNCorselliMUse of human perivascular stem cells for bone regenerationJ Vis Exp201263e295222664543
- JamesAWZaraJNCorselliMAn abundant perivascular source of stem cells for bone tissue engineeringStem Cells Transl Med20121967368423197874
- TellesPDMachadoMASakaiVTNörJEPulp tissue from primary teeth: new source of stem cellsJ Appl Oral Sci201119318919421625731
- ShiSGronthosSPerivascular niche of postnatal mesenchymal stem cells in human bone marrow and dental pulpJ Bone Miner Res200318469670412674330
- MoshaveriniaAChenCXuXBone regeneration potential of stem cells derived from periodontal ligament or gingival tissue sources encapsulated in RGD-modified alginate scaffoldTissue Eng Part A2014203–461162124070211
- MenicaninDMrozikKMWadaNPeriodontal-ligament-derived stem cells exhibit the capacity for long-term survival, self-renewal, and regeneration of multiple tissue types in vivoStem Cells Dev20142391001101124351050
- ZhengYLiuYZhangCMStem cells from deciduous tooth repair mandibular defect in swineJ Dent Res200988324925419329459
- KimSHKimKHSeoBMAlveolar bone regeneration by transplantation of periodontal ligament stem cells and bone marrow stem cells in a canine peri-implant defect model: a pilot studyJ Periodontol200980111815182319905951
- d’AquinoRDe RosaALanzaVHuman mandible bone defect repair by the grafting of dental pulp stem/progenitor cells and collagen sponge biocomplexesEur Cell Mater200918758319908196
- GiulianiAManescuALangerMThree years after transplants in human mandibles, histological and in-line holotomography revealed that stem cells regenerated a compact rather than a spongy bone: biological and clinical implicationsStem Cells Transl Med20132431632423502599
- AnnibaliSCicconettiACristalliMPA comparative morphometric analysis of biodegradable scaffolds as carriers for dental pulp and periosteal stem cells in a model of bone regenerationJ Craniofac Surg201324386687123714898
- MoradGKheiriLKhojastehADental pulp stem cells for in vivo bone regeneration: a systematic review of literatureArch Oral Biol201358121818182724095289
- ZhangWWalboomersXFvan OschGJvan den DolderJJansenJAHard tissue formation in a porous HA/TCP ceramic scaffold loaded with stromal cells derived from dental pulp and bone marrowTissue Eng Part A200814228529418333781
- YangXHanGPangXFanMChitosan/collagen scaffold containing bone morphogenetic protein-7 DNA supports dental pulp stem cell differentiation in vitro and in vivoJ Biomed Mater Res A2012
- ZhangWWalboomersXFvan KuppeveltTHDaamenWFBianZJansenJAThe performance of human dental pulp stem cells on different three-dimensional scaffold materialsBiomaterials200627335658566816916542
- YuJHeHTangCDifferentiation potential of STRO-1+ dental pulp stem cells changes during cell passagingBMC Cell Biol2010113220459680
- KarpJMFerreiraLSKhademhosseiniAKwonAHYehJLangerRSCultivation of human embryonic stem cells without the embryoid body step enhances osteogenesis in vitroStem Cells200624483584316253980
- TongWBrownSEKrebsbachPHHuman embryonic stem cells undergo osteogenic differentiation in human bone marrow stromal cell microenvironmentsJ Stem Cells20072313914720671800
- HuJSmithLAFengKLiuXSunHMaPXResponse of human embryonic stem cell-derived mesenchymal stem cells to osteogenic factors and architectures of materials during in vitro osteogenesisTissue Eng Part A201016113507351420594153
- HallBKMiyakeTDivide, accumulate, differentiate: cell condensation in skeletal development revisitedInt J Dev Biol19953968818938901191
- MacDonaldMEHallBKAltered timing of the extracellular-matrix-mediated epithelial-mesenchymal interaction that initiates mandibular skeletogenesis in three inbred strains of mice: development, heterochrony, and evolutionary change in morphologyJ Exp Zool2001291325827311598914
- AszódiABatemanJFGustafssonEBoot-HandfordRFässlerRMammalian skeletogenesis and extracellular matrix: what can we learn from knockout mice?Cell Struct Funct2000252738410885577
- MaPXBiomimetic materials for tissue engineeringAdv Drug Deliv Rev200860218419818045729
- GrinnemoKHKumagai-BraeschMMånsson-BrobergAHuman embryonic stem cells are immunogenic in allogeneic and xenogeneic settingsReprod Biomed Online200613571272417169186
- AldahmashAAtteyaMElsafadiMTeratoma formation in immunocompetent mice after syngeneic and allogeneic implantation of germline capable mouse embryonic stem cellsAsian Pac J Cancer Prev201314105705571124289566
- WorringerKARandTAHayashiYThe let-7/LIN-41 pathway regulates reprogramming to human induced pluripotent stem cells by controlling expression of prodifferentiation genesCell Stem Cell2014141405224239284
- WaliaBSatijaNTripathiRPGangenahalliGUInduced pluripotent stem cells: fundamentals and applications of the reprogramming process and its ramifications on regenerative medicineStem Cell Rev20128110011521671061
- TakahashiKYamanakaSInduction of pluripotent stem cells from mouse embryonic and adult fibroblast cultures by defined factorsCell2006126466367616904174
- YuJVodyanikMASmuga-OttoKInduced pluripotent stem cell lines derived from human somatic cellsScience200731858581917192018029452
- OhradanovaAGradinKBarathovaMHypoxia upregulates expression of human endosialin gene via hypoxia-inducible factor 2Br J Cancer20089981348135618813310
- KaoCLTaiLKChiouSHResveratrol promotes osteogenic differentiation and protects against dexamethasone damage in murine induced pluripotent stem cellsStem Cells and Dev2010192247258
- UtoSNishizawaSTakasawaYBone and cartilage repair by transplantation of induced pluripotent stem cells in murine joint defect modelBiomed Res201334628128824389404
- JinGZKimTHKimJHBone tissue engineering of induced pluripotent stem cells cultured with macrochanneled polymer scaffoldJ Biomed Mater Res A201310151283129123065721
- LeviBHyunJSMontoroIn vivo directed differentiation of pluripotent stem cells for skeletal regenerationProc Natl Acad Sci USA201210950203792038423169671
- KumazakiTKurataSMatsuoTMitsuiYTakahashiTEstablishment of human induced pluripotent stem cell lines from normal fibroblast TIG-1Hum Cell20112429610321562774
- BrambrinkTForemanRWelsteadGGSequential expression of pluripotency markers during direct reprogramming of mouse somatic cellsCell Stem Cell20082215115918371436
- StadtfeldMMaheraliNBreaultDTHochedlingerKDefining molecular cornerstones during fibroblast to iPS cell reprogramming in mouseCell Stem Cell20082323024018371448
- RomagnoliCBrandiMLAdipose mesenchymal stem cells in the field of bone tissue engineeringWorld J Stem Cells20146214415224772241
- FangDNguyenTKLeishearKA tumorigenic subpopulation with stem cell properties in melanomasCancer Res200565209328933716230395