Abstract
Respiratory diseases provide an attractive target for gene silencing using small nucleic acids since the respiratory epithelium can be reached by inhalation therapy. Natural surfactant appears to facilitate the uptake and distribution of these types of molecules making aerosolized nucleic acids a possible new class of therapeutics. This article will review the rationale for the use of External Guide Sequence (EGS) in targeting specific mRNA molecules for RNase P-mediated intracellular destruction. Specific destruction of target mRNA results in gene-specific silencing similar to that instigated by siRNA via the RISC complex. The application of EGS molecules specific for influenza genes are discussed as well as the potential for synergy with siRNA. Furthermore, EGS could be adapted to target other respiratory diseases of viral etiology as well as conditions such as asthma.
This article will review the use of External Guide Sequence (EGS) directed gene expression silencing which promotes messenger RNA (mRNA) cleavage by RNase P, and the potential applications of this technology to treatment and prophylaxis of influenza and other respiratory diseases such as post viral exacerbations of asthma (CitationDreyfus et al 2004; CitationNyce 1997; CitationNyce and Metzger 1997; CitationPopescu 2005; CitationTrian et al 2006; CitationUlanova et al 2006). Gene silencing with RNase P is based upon the discovery that the ubiquitous RNA enzyme RNase P, required for processing of precursor transfer RNA (pre-tRNA) to the mature transfer RNA (tRNA) can also be programmed to degrade mRNA (CitationBaer et al 1988; CitationGopalan et al 2002; CitationGuerrier-Takada et al 1988; CitationGuerrier-Takada et al 1989; CitationGuerrier-Takada and Altman 2000; CitationRaj and Liu 2003). Since RNase P recognizes the structure of pre-tRNA and certain conserved sequence elements of that structure (), a single stranded RNA termed EGS, can be designed to bind non-covalently to target mRNA resulting in a bimolecular structure that resembles pre-tRNA and is recognized and cleaved by RNase P (). EGSs, as a therapeutic agent, fall into the category of oligonucleotides – respiratory diseases as a whole may be particularly amenable to gene silencing using small nucleic acids since the respiratory epithelium is accessible by inhalation delivery and appears to spontaneously take up these types of molecules (CitationFinotto, Buerke et al 2001; CitationFinotto, De Sanctis et al 2001; CitationMassaro et al 2004; CitationMoschos et al 2007; CitationNyce 1997; CitationThomas et al 2007).
Figure 1 (A) The structure of pre-tRNA (Gln): The structure of precursor transfer RNA (pre-tRNA), a typical substrate for RNase P. RNase P is an abundant RNA enzyme that processes a precursor tRNA transcript through cleavage of a 5′ leader sequence from the transcript. Following processing, the tRNA can accept an amino acid and function in protein synthesis. (B) A synthetic external guide sequence (EGS, highlighted) derived from the structure of pre-tRNA (Gln, Figure 1A) bound to target mRNA forms a pre-tRNA (Gln)-like structure resulting in cleavage of a non-natural substrate mRNA (eg, target). RNase P recognizes the structure as precursor RNA and cleaves the mRNA (depicted as scissors). Thus an EGS can be designed that binds to a target mRNA through altered stem sequences maintaining a conserved stem and loop structure resembling a tRNA precursor. The EGS mRNA hybrid is then recognized as a substrate for RNase P.
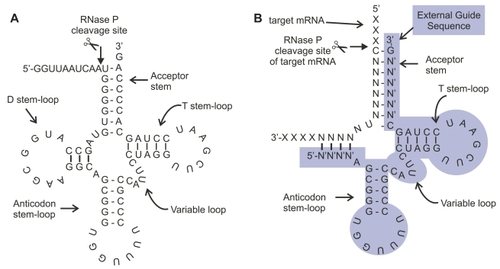
EGS, a short single-stranded RNA, binds by Watson-Crick base-pairing to target mRNA and directs it to RNase P (CitationGuerrier-Takada and Altman 2000). The bimolecular structure of EGS and mRNA forms a substrate for site specific cleavage of the mRNA moiety and inactivation of the mRNA target (). After cleavage of the target, the EGS is released and can bind new target, thereby facilitating additional cycles of target cleavage. The rate of scissile bond cleavage is limited primarily by the concentrations of EGS and mRNA species complementary to the EGS in the nucleus and the binding affinity (Km) of the bimolecular structure to RNase P, which for the native pre-tRNA is a relatively low 9 nM (CitationZiehler et al 2000) RNase P concentrations are high because it is utilized for generation of tRNA in all cell types with a nucleus and active protein synthesis, thus this molecule is not rate-limiting in the reaction (CitationDoersen et al 1985; CitationKoski et al 1976; CitationRobertson et al 1972). In HeLa cells, it is estimated there are 20,000 copies of RNase P per cell (CitationBartkiewicz et al 1989).
Figure 2 EGS can be minimized to approximately 32 nucleotides of single stranded nuclease resistant RNA by incorporation of modified bases conserving the T stem loop necessary for recognition by RNase P. The yellow highlighted nucleotides correspond to regions in the target sequences homologous to the EGS. The pink highlighted regions are 2′-O-methyl modified nuclease resistant nucleotide moieties. The minimized EGS contains only the T stem-loop structure. Other sequences such as the variable and anti-codon loops of tRNA are not required for cleavage by RNase P but may affect binding affinity.
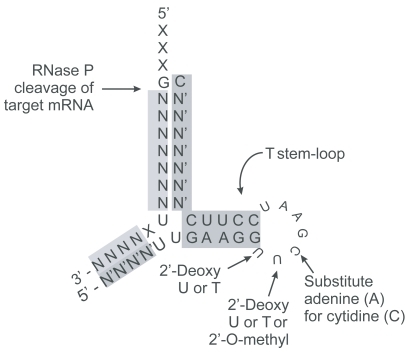
EGS can be expressed from viral or other expression systems in different cell types and directed at different targets (CitationDreyfus et al 2004; CitationKovrigina et al 2005; CitationZhang and Altman 2004, CitationKovrigina et al 2003; CitationGuerrier-Takada and Altman 2000; CitationPlehn-Dujowich and Altman 1998; CitationLi et al 2006; CitationBarnor et al 2004; CitationZhu et al 2004; CitationKitano et al 2000; CitationEndo et al 2001; CitationKraus et al 2002; CitationHnatyszyn et al 2001). Alternatively, EGS can be transiently expressed following high copy number transfection or electroporation of plasmid containing an RNA Pol III promoter driving the EGS sequence (CitationDreyfus et al 2004; CitationRangarajan et al 2004). For therapeutic applications in humans such as for asthma or influenza, EGS can be chemically synthesized as nuclease resistant small molecules (CitationMa et al 1998, Citation2000; CitationZhu et al 2004), avoiding the need for vector and promoter sequences (). Transient transfection of these nuclease resistant molecules circumvents the risk of somatic gene mutations which could lead to malignant transformation of recipient cells when viruses or virally derived DNA sequences are used.
Figure 3 Comparison of (A) EGS directed targeting of mRNA to RNase P with (B) small double-stranded RNA (dsRNA) or regulatory short hairpin RNA (shRNA) directed targeting of mRNA to RISC. Both pathways require a nucleotide guide sequence – for RNase P, EGS is the guide while for RISC, the antisense strand of a double-stranded small interfering RNA (siRNA) is incorporated into the RISC complex. siRNAs can be directly introduced into the cell or are generated by the enzyme, Dicer from short hairpin RNA generated from a DNA template or from dsRNA. In this view, the EGS that binds to target mRNA and forms a substrate recognized by RNase P is directly analogous by function to the antisense strand of the 21–23 nucleotide siRNA that is incorporated into RISC and guides Slicer mediated mRNA cleavage. Both RNase P and the argonaute protein Slicer introduce a single cleavage site in the target – the cleaved mRNA is recognized as abnormal and further degraded by cellular nucleases.
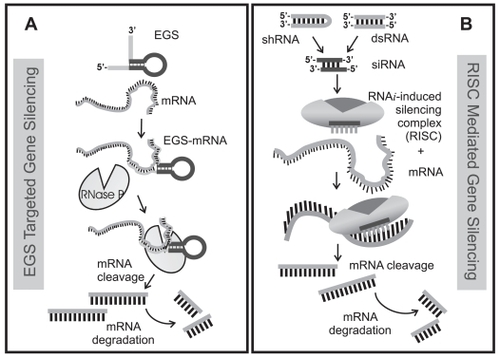
In a research setting, using EGS expressed at high copy number in vivo from vectors is more convenient and cost effective for screening large numbers of constructs than using stably transfected cell lines or transfection of chemically synthesized EGS (CitationDreyfus et al 2004). We described a relatively simple screening assay in which EGS and reporter genes are transiently electroporated into a well characterized and publicly available B-lymphoblastoid cell line that expresses a functional IL-4 receptor to demonstrate the ability of EGS to silence IL-4 signaling using the human IL-4 promoter fused to the luciferase gene. We have also used a Jurkat T lymphoblastoid cell line expressing both functional IL-4and adenosine receptors important mediators of asthma and other respiratory diseases (CitationGeoras et al 1998), to confirm these results. In this type of in vitro cellular assay, EGS potency and specificity are assessed by silencing of a luciferase reporter gene. Moreover, the assay can be scaled up and automated for large numbers of candidate EGS.
The relevance of IL-4/13 receptor as a target for EGS or other gene silencing has been reviewed previously (CitationDreyfus et al 2004). The effects of active EGS that are RNase P dependent can be distinguished from non-specific effects of the EGS on reporter expression since they are decreased by mutation of the EGS T loop required for cleavage by RNase P. Some interesting conclusions are evident regarding potency and specificity of the anti-IL4 receptor EGS in this type of assay (Dreyfus and Fuleihan, unpublished observations). Two EGS molecules designed to target two distinct regions in the IL-4/13 common receptor mRNA each independently exhibited RNase P dependent inactivation of IL-4 signaling. Of the two EGSs, one which targets a site near the start codon of the IL-4/13 common receptor mRNA is more potent than another EGS targeting a sequence located more distal to the start codon even though both target sequences are similarly accessible based on digestion by nuclease T1 in vitro. Also, an EGS targeting the murine homologue of the human IL-4/13 receptor chain was still able to cause RNase P dependent inactivation of the human target despite the presence of mismatches in 2 of 11 nucleotides. However, these mismatches resulted in a greater than 50% decrease in target site inactivation when tested in tissue culture.
Thus, RNase P dependent EGS targeting is dependent on multiple factors related to target site accessibility and conformation in vivo – factors that are not entirely predictable using sequence based algorithms or in vitro methods. Targets can be cleaved in vivo even if significant mismatches are present between EGS and target although this cleavage is less efficient than correctly paired EGS. Methods such as DNA microarray analysis could be used to look for evidence of off target/non-specific effects of EGS. Once an optimal candidate EGS or multiple EGS are identified using an unbiased automated process capable of screening large numbers of randomized or otherwise modified EGS, these optimized EGS would then be further characterized in vitro and in vivo in human primary cells and animal models prior to human clinical trials. In the absence of a readily available reporter gene for monitoring EGS activity in vivo, a reporter gene could be constructed which expresses the target gene mRNA fused upstream of the luciferase mRNA so that cleavage of the target mRNA would proportionately reduce luciferase expression.
For in vivo applications, EGS delivery methods must be optimized to permit sufficient concentrations of EGS to enter the respiratory tract and gain access to cells of the respiratory epithelium as nuclease resistant small molecules with minimal non-specific or pro-inflammatory properties. To accomplish this, an inhaler could be optimized for delivery of EGS in a transfection reagent composed of lipids. The concentration of EGS in the cell nuclei will be the limiting factor in the rate of EGS-directed target cleavage. Since EGS molecules are RNA oligonucleotides, many of the issues encountered in delivering siRNA are applicable to EGS. In the case of siRNA, published data suggest a therapeutic effect against influenza even with the administration of unmodified RNA duplex by hydrodynamic tail vein injection in mice (CitationTompkins et al 2004; CitationLarson et al 2007; CitationHerweijer and Wolff 2007; CitationBradley et al 2005).
RNAi is an evolutionarily conserved expression silencing pathway which also plays a role in antiviral defense, particularly against those viruses with an RNA genome that replicate in the cytoplasm. However, RNA viruses such as influenza and vaccinia have developed means of undermining the RNAi pathway (CitationLi and Ding 2005; CitationSchott et al 2005; CitationSchutz and Sarnow 2006). Viral proteins often target the enzymes in RISC, thereby attenuating the RNAi pathway rather than directly targeting the regulatory RNA mediator molecules. While the role of RNase P in antiviral defense has not specifically been explored, it is interesting to note that a putative RNase P RNA gene has been identified in camel-pox and vaccinia viruses, members of the orthopoxviruses (CitationYang et al 2005). These RNase P molecules were found to be enzymatically inactive and a role for these RNA enzymes in the viral life cycle has yet to be established.
We suggest that synergy may be possible between gene silencing with EGS/RNase P and gene silencing with siRNA/RNAi. As shown in , gene silencing with EGS and RNase P and gene silencing with siRNA and the RNA-induced silencing complex (RISC) share similar characteristics. In both cases a small RNA sequence with complementarity to the target serves as a guide to cleavage of the mRNA by an endogenous RNase. Both schemes result in the cleavage of many targets per guide sequence. In the RNase P mediated gene silencing pathway, the small single stranded RNA is termed an EGS, while with RISC, the RNA is termed siRNA. In contrast, antisense technology utilizes modified DNA oligonucleotides which act pleiotropically at the level of transcription, splicing, protein synthesis and can also activate RNase H resulting in destruction of the complementary mRNA (CitationMonia et al 1996; CitationMonia et al 1993; CitationLima et al 1992; CitationChan et al 2006).
What then are the relative advantages and disadvantages of the RNase P pathway compared to RNAi? Advantages of gene silencing with EGS/RNase P may include more rapid onset of action than siRNA/RNAi (CitationZhang and Altman 2004). Experiments using EGS and shRNA expressed from identical vectors against an identical target suggest that the onset of action of EGS can be detected within 24 hours while under identical conditions, shRNA effects were evident only after 48 hours. If this 24 hour difference in onset of action is a general phenomenon that distinguishes the two, it could be highly significant for therapeutic applications. For example, in the treatment of respiratory viral infections such as influenza, a difference in onset of action of 24 hours could result in significantly different outcomes in viral titer as well as severity of symptoms and complications. In addition, where a gene silencing strategy is used for prophylactic purposes following exposure to virus, a 24 hour difference in onset of action could be highly significant since viral replication peaks within 48 hours of exposure.
Another potential advantage is that EGS is essentially single-stranded. Thus, Toll receptor 3 recognizing short, double-stranded RNA would not be expected to be activated (CitationKariko, Bhuyan et al 2004; CitationKariko, Ni et al 2004; CitationAkira 2006; CitationMatsukura et al 2006).
Another important point of comparison between EGS and siRNA is potency and specificity which are related, but not identical issues. A less potent drug can have a similar efficacy to a more potent drug if a higher concentration of the less potent compound is used. However, where there are significant off-target effects, increasing dosage will almost certainly lead to more non-specific side-effects such as inflammatory responses or the triggering of pro-apoptotic proteins such as p21 in the case of siRNA (CitationBridge et al 2003; CitationJackson et al 2003; CitationJackson and Linsley 2004; CitationScacheri et al 2004). At the current time, very little is known about the specificity of either siRNA or EGS under in vivo conditions and particularly in the inflammatory setting predicted to be elicited by a viral infection such as influenza, or a chronic inflammatory disease, such as asthma. Further experiments using comprehensive methods such as DNA microarrays and proteomics will be required to conclusively resolve issues of specificity of both siRNA and EGS in specific in vivo applications.
Limited in vitro evidence suggests siRNA appear equipotent to EGS if one can design the EGS based on RNase accessibility. However, an EGS targeted to an identical site as siRNA may or may not be as potent. This is due primarily to the accessibility of the EGS to its target site. For RISC, these rules are less well understood because of the involvement of helicases which interact with RISC and functions in the modulation of the secondary structure of the target mRNA and/or RISC loading (CitationBernstein et al 2001; CitationRobb and Rana 2007). The availability of premade cocktails of siRNA as well as the widespread use of siRNA render this the method of choice for gene expression silencing in life sciences discovery. However, in many applications, it is also preferable to use a second, independent method, to validate results. In this context, EGS may be particularly useful given its specificity and relative ease of design and use. Finally, EGS may be the tool of choice where effective and specific siRNAs cannot be designed.
Once a target is validated, EGS can be optimized through unbiased randomization procedures leading to perhaps 100-fold or more increased potency against a specified mRNA target with only small changes in the EGS sequence (CitationYang et al 2006; CitationZhou et al 2002). EGS optimization would not be expected to increase non-specific inflammatory and/or pro-apoptotic responses in vivo however, this remains to be tested. siRNA, in contrast, cannot be optimized beyond the point at which the enzyme Slicer, a component of RISC, becomes rate-limiting (CitationKoller et al 2006). In the context of influenza treatment, inflammation may have a beneficial additive or synergistic effect with siRNA but could also exert detrimental effects leading to the development of acute lung injury (ALI) or adult respiratory distress syndrome (ARDS) in the case of reassorted zoonotic strains. Additionally, post-viral exacerbations such as asthma may also be promoted by siRNA induced inflammatory responses. It would probably be most fair to say that the relative potency and specificity of EGS vs siRNA in vivo for treatment of disease cannot be determined on theoretical grounds because of the many interacting and unknown variables, but will require direct comparisons of the two therapies against a particular target when this is possible. It is certainly plausible that there will be some genes or tissues for which EGS silencing is more potent and specific in vivo, and other genes for which the opposite is found, which would suggest a rationale for using both drugs to target a gene of interest in therapeutic applications.
A clear advantage of siRNA is the ability to inactivate viruses such as the respiratory pathogens RSV (Respiratory Syncytial Virus) and rhinovirus that replicate in the cell cytoplasm (CitationPhipps et al 2004; CitationStrayer et al 2005; CitationKong et al 2007). Since RNase P is only present in the cell nucleus, pathogens whose transcripts reside exclusively in the cell cytoplasm cannot be targeted directly. However, a number of viruses and intracellular pathogens rely on host proteins for key steps in their life cycle (CitationElliott et al 2007; CitationFauci 1996; CitationFauci and Challberg 2005; CitationGu et al 2004). Where the identity of such proteins is known, it may be possible to target these using EGS. Furthermore, the pathogenicity of these viruses may occur through induction of cellular inflammatory cytokines such as interferon or IL-13 (CitationLiu et al 2005); inactivation of these virally triggered cytokines by EGS or inhibition of the NF-κB transcription factor which acts as a master switch, might still be an effective immunotherapy for these cytoplasmic pathogens. Using EGS, it may be possible to differentially inactivate specific subunits of NF-κB which would still allow other subunits to engage in transcriptional activation. Alternatively, the receptors for the pathogens could also be silenced with EGS (CitationBarth, Liang et al 2006; CitationBarth, Schnober et al 2006; CitationJulg and Goebel 2005; CitationWeber et al 2006; CitationLederman et al 2006), preventing infection or reducing spread and virus load in an ongoing infection. Since the pathogenesis of influenza as well as RSV involves modulation of cytokines such as decreased expression of interferon, and increased synthesis of IL-4 and IL-13 that play a role in post viral exacerbations of asthma (CitationElliott et al 2007; CitationOh et al 2002; CitationMinor et al 1976; CitationSingh and Busse 2006; CitationTekkanat et al 2001; CitationWang et al 2004; CitationYasuda et al 2005), it is likely that a combination of anti-viral and anti-cytokine therapies may be required for efficacy in both influenza and post viral asthma.
Perhaps the most intriguing possibility is that EGS and siRNA gene silencing may synergize since they use different mechanisms to inactivate target mRNA yet utilize similar or identical methods of delivery. depicts a scheme by which synergy might be attained by the co-expression of EGS and short hairpin RNA if expressed from DNA or EGS and siRNA if administered as RNA oligonucleotides. Advances in delivery of one modality will almost certainly benefit the delivery of the other in a particular disease process. We hypothesize that for pharmacological applications against human respiratory diseases such as influenza and asthma, as well as other diseases which are accessible to either modality, some combination of EGS and siRNA may be optimal in terms of onset of action, specificity, and potency.
Figure 4 (A) Potential for synergy between EGS and siRNA: Co-expression of EGS in the nucleus and siRNA in the cytoplasm could effectively synergize to promote gene expression silencing since the two use different pathways. (B) Stable transfection of cDNA constructs encoding EGS and shRNA could also achieve the same results – as shown, we propose a self-cleaving transcript using hammerhead ribozymes encoded in the flanking regions of the shRNA sequences.
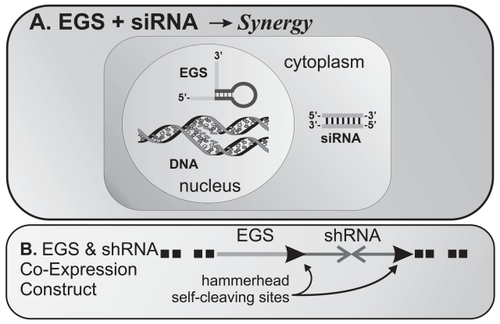
Acknowledgments and disclosures
The authors thank Dr Sidney Altman of Yale University for his generous assistance and for making available constructs and supplies for the synthesis of EGS directed at the human and murine IL-4 receptor described in this work. Dr David H Dreyfus is currently a member of the Clinical Faculty at the Yale University School of Medicine, Department of Pediatrics, New Haven, CT and is also the founder and Medical Director of Keren Pharmaceutical, Inc. a biotechnology company focused on the use of EGS technology in human therapeutics and research applications. Dr Fuleihan’s present address is the Department of Pediatrics, Children’s Memorial Hospital, Chicago, IL ([email protected]). Dr S Mark Tompkins is an Assistant Professor in the Department of Infectious Diseases, at the University of Georgia College of Veterinary Medicine and is a consultant to Keren Pharmaceutical. Dr Lucy Y Ghoda, is an Investigator at the Webb-Waring Institute, and is a faculty member in the Division of Pulmonary Sciences, Department of Medicine, School of Medicine, University of Colorado Health Sciences Center. She is Scientific Director of Keren Pharmaceutical.
References
- AkiraS2006TLR signalingCurr Top Microbiol Immunol31111617048703
- BaerMFReillyRMMccorkleGM1988The recognition by RNase P of precursor tRNAsJ Biol Chem2632344513123492
- BarnorJSEndoYHabuY2004Effective inhibition of HIV-1 replication in cultured cells by external guide sequences and ribonuclease PBioorg Med Chem Lett144941415341956
- BarthHLiangTJBaumertTF2006Hepatitis C virus entry: molecular biology and clinical implicationsHepatology445273516941688
- BarthHSchnoberEKZhangF2006Viral and cellular determinants of the hepatitis C virus envelope-heparan sulfate interactionJ Virol80105799016928753
- BartkiewiczMGoldHAltmanS1989Identification and characterization of an RNA molecule that copurifies with RNase P activity from HeLa cellsGenes Dev3488992470644
- BernsteinECaudyAAHammondSM2001Role for a bidentate ribonuclease in the initiation step of RNA interferenceNature409363611201747
- BradleySPRastelliniCDa CostaMA2005Gene silencing in the endocrine pancreas mediated by short-interfering RNAPancreas31373916258373
- BridgeAJPebernardSDucrauxA2003Induction of an interferon response by RNAi vectors in mammalian cellsNat Genet34263412796781
- ChanJHLimSWongWS2006Antisense oligonucleotides: from design to therapeutic applicationClin Exp Pharmacol Physiol335334016700890
- DoersenCJGuerrier-TakadaCAltmanS1985Characterization of an RNase P activity from HeLa cell mitochondria. Comparison with the cytosol RNase P activityJ Biol Chem260594292581945
- DreyfusDHMatczukAFuleihanR2004An RNA external guide sequence ribozyme targeting human interleukin-4 receptor alpha mRNAInt Immunopharmacol410152715222976
- ElliottJLynchOTSuessmuthY2007Respiratory syncytial virus NS1 protein degrades STAT2 by using the Elongin-Cullin E3 ligaseJ Virol8134283617251292
- EndoYMiyano-KurosakiNKitanoM2001Effective inhibition of HIV-1 replication in cultured cells by external guide sequences and ribonucleaseNucleic Acids Res Suppl2131412836340
- FauciAS1996Host factors and the pathogenesis of HIV-induced diseaseNature384529348955267
- FauciASChallbergMD2005Host-based antipoxvirus therapeutic strategies: turning the tablesJ Clin Invest115231315690079
- FinottoSBuerkeMLingnauK2001Local administration of antisense phosphorothioate oligonucleotides to the c-kit ligand, stem cell factor, suppresses airway inflammation and IL-4 production in a murine model of asthmaJ Allergy Clin Immunol1072798611174194
- FinottoSDe SanctisGTLehrHA2001Treatment of allergic airway inflammation and hyperresponsiveness by antisense-induced local blockade of GATA-3 expressionJ Exp Med19312476011390432
- GeorasSNCumberlandJEBurkeTF1998Stat6 inhibits human interleukin-4 promoter activity in T cellsBlood924529389845517
- GopalanVVioqueAAltmanS2002RNase P: variations and usesJ Biol Chem27767596211741968
- GuWOgoseAKawashimaH2004High-level expression of the coxsackievirus and adenovirus receptor messenger RNA in osteosarcoma, Ewing’s sarcoma, and benign neurogenic tumors among musculoskeletal tumorsClin Cancer Res103831815173092
- Guerrier-TakadaCAltmanS2000Inactivation of gene expression using ribonuclease P and external guide sequencesMethods Enzymol3134425610595372
- Guerrier-TakadaCLumelskyNAltmanS1989Specific interactions in RNA enzyme-substrate complexesScience2461578842480641
- Guerrier-TakadaCVan BelkumAPleijCW1988Novel reactions of RNAase P with a tRNA-like structure in turnip yellow mosaic virus RNACell53267723359488
- HerweijerHWolffJA2007Gene therapy progress and prospects: hydrodynamic gene deliveryGene Ther149910717167496
- HnatyszynHSpruillGYoungA2001Long-term RNase P-mediated inhibition of HIV-1 replication and pathogenesisGene Ther818637111821940
- JacksonALBartzSRSchelterJ2003Expression profiling reveals off-target gene regulation by RNAiNat Biotechnol21635712754523
- JacksonALLinsleyPS2004Noise amidst the silence: off-target effects of siRNAsTrends Genet20521415475108
- JulgBGoebelFD2005CCR5 antagonists: a new tool in fighting HIVJ HIV Ther10687116519245
- KarikoKBhuyanPCapodiciJ2004Exogenous siRNA mediates sequence-independent gene suppression by signaling through toll-like receptor 3Cells Tissues Organs177132815388987
- KarikoKNiHCapodiciJ2004mRNA is an endogenous ligand for Toll-like receptor 3J Biol Chem279125425014729660
- KitanoMMiyano-KurosakiNEndoY2000Effective suppression of HIV-1 gene expression by a mammalian tRNA 3′ processing endoribonuclease and external guide sequence oligozymesNucleic Acids Symp Ser207812903341
- KollerEProppSMurrayH2006Competition for RISC binding predicts in vitro potency of siRNANucleic Acids Res3444677616945958
- KongXZhangWLockeyRF2007Respiratory syncytial virus infection in Fischer 344 rats is attenuated by short interfering RNA against the RSV-NS1 geneGenet Vaccines Ther5417270047
- KoskiRABothwellALAltmanS1976Identification of a ribonuclease P-like activity from human KB cellsCell91011610085
- KovriginaEWesolowskiDAltmanS2003Coordinate inhibition of expression of several genes for protein subunits of human nuclear RNase PProc Natl Acad Sci USA100159860212552092
- KovriginaEYangLPfundE2005Regulated expression of functional external guide sequences in mammalian cells using a U6 RNA polymerase III promoterRNA1115889516131590
- KrausGGeffinRSpruillG2002Cross-clade inhibition of HIV-1 replication and cytopathology by using RNase P-associated external guide sequencesProc Natl Acad Sci USA9934061111904403
- LarsonSDJacksonLNChenLA2007Effectiveness of siRNA uptake in target tissues by various delivery methodsSurgery142262917689694
- LedermanMMPenn-NicholsonAChoM2006Biology of CCR5 and its role in HIV infection and treatmentJAMA2968152616905787
- LiHTrangPKimK2006Effective inhibition of human cytomegalovirus gene expression and growth by intracellular expression of external guide sequence RNARNA12637216301604
- LiHWDingSW2005Antiviral silencing in animalsFEBS Lett57959657316154568
- LimaWFMoniaBPEckerDJ1992Implication of RNA structure on antisense oligonucleotide hybridization kineticsBiochemistry3112055611280997
- LiuLXuZFuhlbriggeRC2005Vaccinia virus induces strong immunoregulatory cytokine production in healthy human epidermal keratinocytes: a novel strategy for immune evasionJ Virol7973637015919891
- MaMBenimetskayaLLebedevaI2000Intracellular mRNA cleavage induced through activation of RNase P by nuclease-resistant external guide sequencesNat Biotechnol18586110625392
- MaMYJacob-SamuelBDignamJC1998Nuclease-resistant external guide sequence-induced cleavage of target RNA by human ribonuclease PAntisense Nucleic Acid Drug Dev8415269826268
- MassaroDMassaroGDClerchLB2004Noninvasive delivery of small inhibitory RNA and other reagents to pulmonary alveoli in miceAm J Physiol Lung Cell Mol Physiol287L10667015234906
- MatsukuraSKokubuFKurokawaM2006Synthetic double-stranded RNA induces multiple genes related to inflammation through Toll-like receptor 3 depending on NF-kappaB and/or IRF-3 in airway epithelial cellsClin Exp Allergy3610496216911361
- MinorTEDickECBakerJW1976Rhinovirus and influenza type A infections as precipitants of asthmaAm Rev Respir Dis11314953174464
- MoniaBPJohnstonJFSasmorH1996Nuclease resistance and antisense activity of modified oligonucleotides targeted to Ha-rasJ Biol Chem27114533408662854
- MoniaBPLesnikEAGonzalezC1993Evaluation of 2′-modified oligonucleotides containing 2′-deoxy gaps as antisense inhibitors of gene expressionJ Biol Chem26814514228390996
- MoschosSAWilliamsAELindsayMA2007Cell-penetrating-peptide-mediated siRNA lung deliveryBiochem Soc Trans358071017635153
- NyceJW1997Respirable antisense oligonucleotides as novel therapeutic agents for asthma and other pulmonary diseasesExpert Opin Investig Drugs6114956
- NyceJWMetzgerWJ1997DNA antisense therapy for asthma in an animal modelNature38572159034188
- OhJWLeeHBParkIK2002Interleukin-6, interleukin-8, interleukin-11, and interferon-gamma levels in nasopharyngeal aspirates from wheezing children with respiratory syncytial virus or influenza A virus infectionPediatr Allergy Immunol13350612431194
- PhippsKMMartinezALuJ2004Small interfering RNA molecules as potential anti-human rhinovirus agents: in vitro potency, specificity, and mechanismAntiviral Res61495514670593
- Plehn-DujowichDAltmanS1998Effective inhibition of influenza virus production in cultured cells by external guide sequences and ribonuclease PProc Natl Acad Sci USA957327329636148
- PopescuFD2005Antisense- and RNA interference-based therapeutic strategies in allergyJ Cell Mol Med98405316364194
- RajSMLiuF2003Engineering of RNase P ribozyme for gene-targeting applicationsGene313596912957377
- RangarajanSRajMLHernandezJM2004RNase P as a tool for disruption of gene expression in maize cellsBiochem J380611615096096
- RobbGBRanaTM2007RNA helicase A interacts with RISC in human cells and functions in RISC loadingMol Cell265233717531811
- RobertsonHDAltmanSSmithJD1972Purification and properties of a specific Escherichia coli ribonuclease which cleaves a tyrosine transfer ribonucleic acid presursorJ Biol Chem2475243514560501
- ScacheriPCRozenblatt-RosenOCaplenNJ2004Short interfering RNAs can induce unexpected and divergent changes in the levels of untargeted proteins in mammalian cellsProc Natl Acad Sci USA1011892714769924
- SchottDHCuretonDKWhelanSP2005An antiviral role for the RNA interference machinery in Caenorhabditis elegansProc Natl Acad Sci USA10218420416339901
- SchutzSSarnowP2006Interaction of viruses with the mammalian RNA interference pathwayVirology344151716364746
- SinghAMBusseWW2006Asthma exacerbations. 2: aetiologyThorax618091616936237
- StrayerDSFeitelsonMSunB2005Paradigms for conditional expression of RNA interference molecules for use against viral targetsMethods Enzymol3922274115644185
- TekkanatKKMaassabHFChoDS2001IL-13-induced airway hyperreactivity during respiratory syncytial virus infection is STAT6 dependentJ Immunol1663542811207314
- ThomasMLuJJChenJ2007Non-viral siRNA delivery to the lungAdv Drug Deliv Rev591243317459519
- TompkinsSMLoCYTumpeyTM2004Protection against lethal influenza virus challenge by RNA interference in vivoProc Natl Acad Sci USA1018682615173583
- TrianTGirodetPOOusovaO2006RNA interference decreases PAR-2 expression and function in human airway smooth muscle cellsAm J Respir Cell Mol Biol34495516195539
- UlanovaMSchreiberADBefusAD2006The future of antisense oligonucleotides in the treatment of respiratory diseasesBioDrugs2011116573347
- WangSZBaoYXRosenbergerCL2004IL-12p40 and IL-18 modulate inflammatory and immune responses to respiratory syncytial virus infectionJ Immunol1734040915356153
- WeberJPiontkivskaHQuinones-MateuME2006HIV type 1 tropism and inhibitors of viral entry: clinical implicationsAIDS Rev8607716848274
- YangLWesolowskiDLiY2005Analysis of putative RNase P RNA from orthopoxvirusesJ Mol Biol3545293516253270
- YangYHLiHZhouT2006Engineered external guide sequences are highly effective in inducing RNase P for inhibition of gene expression and replication of human cytomegalovirusNucleic Acids Res345758316432261
- YasudaHSuzukiTZayasuK2005Inflammatory and bronchospastic factors in asthma exacerbations caused by upper respiratory tract infectionsTohoku J Exp Med2071091816141679
- ZhangHAltmanS2004Inhibition of the expression of the human RNase P protein subunits Rpp21, Rpp25, Rpp29 by external guide sequences (EGSs) and siRNAJ Mol Biol34210778315351636
- ZhouTKimJKilaniAF2002In vitro selection of external guide sequences for directing RNase P-mediated inhibition of viral gene expressionJ Biol Chem277301122012050148
- ZhuJTrangPKimK2004Effective inhibition of Rta expression and lytic replication of Kaposi’s sarcoma-associated herpesvirus by human RNase PProc Natl Acad Sci USA1019073815184661
- ZiehlerWADayJJFierkeCA2000Effects of 5′ leader and 3′ trailer structures on pre-tRNA processing by nuclear RNase PBiochemistry3999091610933810