Abstract
Leukocyte adhesion contributes to perfusion abnormalities and tissue damage during trauma, shock or overwhelming inflammation. This study was performed to determine whether the lipoxygenase inhibitor phenidone and derivatives decrease the expression of adhesion molecules on tumor necrosis factor-α (TNF-α) stimulated endothelial cells and attenuate leukocyte-endothelial interactions under flow in vitro. TNF-α stimulated human umbilical venous endothelial cells (HUVECs) were incubated with phenidone, 4-methyl-phenidone, 4-4-dimethyl-phenidone, 5-methyl-phenidone, 5-phenyl-phenidone, and 5-methyl-1,(2,5-di-chloro-phenyl)-3-pyrazolidone. We tested the inhibition of adhesion molecule expression at different inhibitor concentrations before, during, and after the stimulation of HUVECs. The inhibition of endothelial cell expression on HUVECs was measured by flow cytometry. Rolling and firm adhesion of leukocytes to pretreated endothelium was examined in a parallel plate flow chamber. Phenidone inhibited the expression of intercellular adhesion molecule-1, vascular cell adhesion molecule-1, and endothelial-leukocyte adhesion molecule-1 on HUVECs when added prior to HUVEC stimulation. The inhibitory effect of phenidone was still observed when added simultaneously, but not when added after HUVEC stimulation. 4-4-dimethyl-phenidone and 5-phenyl-phenidone inhibited the expression of adhesion molecules more effectively than phenidone. The attenuation of leukocyte rolling under flow conditions was also significantly more effective with 4-4-dimethyl-phenidone than with phenidone. Lipoxygenase inhibitors might be of therapeutically interest for the treatment of overwhelming systemic inflammation during shock, trauma, and sepsis.
Introduction
The infiltration of infected tissue by leukocytes is important for the innate immune response. Before leaving the vascular system, the leukocytes must make contact with the endothelial cells of the vessel wall. Once this contact has been established, the leukocytes transmigrate through the tissue and fight local inflammatory processes by various defense mechanisms.
Systemic inflammatory response syndrome (SIRS) is a systemic event and is defined by clinical parameters such as body temperature, heart rate, respiratory insufficiency, and leukocyte count in the context of a typical clinical setting with or without a confirmed infection (CitationBone 1992). Leukocyte adhesion is a hallmark of shock, overwhelming inflammation and ischemia/reperfusion injury (CitationVedder et al 1988; CitationEppihimer and Granger 1997; CitationSingbartl et al 2000). In the case of systemic inflammation, there is widespread transmigration of leukocytes from the vascular system through the activated tissue. The high mortality rate associated with this syndrome is due in part to the fact that the inflammatory process is not kept at a local level, but spreads throughout the body. Leukocyte diapedesis has detrimental effects on innate immunity and causes the failure of organs such as kidneys, liver, lungs, etc.
On endothelial cells, the expression of endothelial-leukocyte adhesion molecule-1 (E-selectin), ICAM-1 (intercellular adhesion molecule-1), and VCAM-1 (vascular cell adhesion molecule-1) leads to a multistep process of leukocyte-endothelial interaction. Following transient rolling interactions mediated by selectins, the binding of ICAM-1 and VCAM-1 to their integrin counterreceptors results in firm adhesion of the leukocytes, which finally transmigrate into the subendothelial tissue (CitationJaeschke and Smith 1997; CitationRamos et al 1997). Concomitant signaling via adhesion molecules activates the interacting cells (CitationHlasta et al 1991). Since leukocyte-endothelial interactions are a crucial contributor to many aspects of organ dysfunction in critical illness (CitationSingbartl et al 2000), the reduction of adhesion molecule expression on activated endothelial cells could be a therapeutic option to attenuate leukocyte-mediated tissue injury.
5-lipoxygenase pathways are widely distributed within mammalian cells and catalyze the production of leukotrienes, which act as potent biological mediators (CitationCuzzocrea et al 2004; CitationZhao et al 2004). Leukotrienes can be synthetized by vascular endothelial cells, smooth muscle cells, neutrophils, macrophages, platelets, etc., and themselves can trigger the expression of adhesion molecules on the surface of endothelial cells and leukocytes (CitationReilly et al 2004). The principal substrate for leukotrienes is arachidonic acid. In a next step, the enzymes cyclooxygenase and lipoxygenase catalyze the production of prostaglandins and leukotrienes, respectively. The lipoxygenases are classified according to the position at which they oxidize arachidonic acid. Leukotriene B4 (LTB4) is a potent activator of human leukocytes and can induce inflammatory reactions such as polymorphonuclear activation, migration and adhesion by nuclear factor-kappa B (NF-κB) activation. The biological potency of leukotrienes has stimulated the search for 5-lipoxygenase inhibitors with antiinflammatory potency in a multitude of medical fields (CitationDe Caterina and Zampolli 2004; CitationGaddi et al 2004; CitationWang et al 2004).
1-phenyl-3-pyrazolidinone (phenidone) is a combined 5-lipoxygenase and cyclooxygenase inhibitor. Increased lipophilicity of phenidone derivatives is correlated with increased inhibitor potency. By preventing the oxidation of arachidonic acid to 5-HPETE we may be able to inhibit 5-lipoxygenase.
In this study, we tested the ability of phenidone and potent derivatives to inhibit the expression of adhesion molecules E-selectin, ICAM-1, and VCAM-1 on activated human vascular endothelial cells (HUVECs). We also measured their potential to inhibit leukocyte rolling and firm adhesion in a dynamic flow assay.
Materials and methods
Antibodies and reagents
Fluorochrome-conjugated murine monoclonal antibodies (mAb) against CD11b (IgG1, fluorescein conjugates, Caltag, San Francisco, CA), CD15 (IgM, phycoerythrin-conjugate), CD45, CD62L (IgG1, fluorescein-conjugates, Becton Dickinson, San Jose, CA), Lewis X, E-selectin, VCAM-1 (CD15, CD62E and CD106; IgM and IgG1, fluorescein-conjugates, Southern Biotechnologies, Birmingham, AL), and ICAM-1 (CD54; IgG1, phycoerythrin-conjugate, Immunotech, Marseille, France) were used in saturating amounts for subsequent flow cytometric analysis of cell surface antigen expression (FACSort™, Becton Dickinson, San Jose, CA). Isotype-matched control antibodies were purchased from the manufacturers of the corresponding specific antibodies.
Phenidone and derivatives were obtained from Hampford Research Inc., Stratford, Connecticut, USA (). Derivatives were dissolved with DMSO to a final concentration of 0.2% in cell culture medium. In control experiments, no influence of DMSO on the adhesion molecule expression of activated HUVECs was observed.
Cell culture and human polymorphonuclear neutrophil isolation
Human umbilical venous endothelial cells (HUVECs) were harvested from freshly prepared umbilical cords by enzymatic treatment of the umbilical vein with collagenase A 0.1% (CitationWakatsuki et al 1999). The cells were grown at 37 °C in endothelial cell growth medium containing and 5% CO2 fetal calf serum 2%, epidermal growth factor 0.1 ng/ml, basic fibroblast growth factor 1.0 ng/ml, gentamicin 50 μg/ml, amphotericin B 0.05 μg/ml and 20 μl endothelial cell growth supplement/heparin. This preparation yielded a purity and viability of >90% as confirmed by staining for von Willebrand factor and trypan blue exclusion. After the first passage, the cells were plated at high density on either collagen-treated 12-well plates or rectangular coverslips (No. 1.5 thickness, Kindler, Freiburg, Germany) precoated with collagen I and used for the experiment 48 h later after they had reached confluency.
Human polymorphonuclear neutrophils (PMN) were isolated from citrated blood by density gradient centrifugation at 1700 rpm on a discontinuous Percoll gradient with 63% and 72% Percoll in phosphate buffer solution (PBS) (Percoll, 1.130 g/ml; Amersham Pharmacia Biotech, Uppsala, Sweden). The bottom layer, containing the polymorphonuclear neutrophil granulocytes (PMN), was collected; contaminating erythrocytes were removed by hypotonic lysis in 10% NH4Cl on ice. Following a final wash with cold PBS, the PMN pellet was resuspended in cold medium 199 at 5 × 107/ml. As determined by light scatter and cell surface antigen analysis of CD15, CD45, CD62L, and CD11b, separation yielded functionally intact, non-activated PMNs at >90% purity, with only minimal alterations in surface molecules that are sensitive markers for activation. Samples were obtained after obtaining written consent and approval of the local ethics committee.
Endothelial cell activation
To determine the inhibitory effect of lipoxygenase-cyclooxygenase inhibitors on adhesion molecule expression on endothelial cells, HUVECs were stimulated with tumor necrosis factor-α (TNF-α) (0.5 ng/mL) (Sigma, St. Louis, MO, USA) 2 h before (preincubation), simultaneously with (coincubation), or 2 h after (postincubation) the addition of the lipoxygenase-cyclooxygenase inhibitor (). The inhibitor was added in increasing concentrations up to 2 mMol for 4 h (pre- and postincubation) or 6 h (coincubation). The final concentrations of lipoxygenase-cyclooxygenase inhibitors employed in flow assays were determined in preliminary experiments examining the effects of different concentrations of inhibitors on adhesion molecule expression and cell viability. Control experiments consisted of the incubation of HUVECs with medium alone, or the activation of adhesion molecule expression without the addition of an inhibitor. At the end of a 6 h incubation period, the response to stimulation was determined by flow cytometric analysis of endothelial cell adhesion molecules. Prior to the measurement, HUVECs were labelled with 20 μl of mAb against E-selectin, ICAM-1, and VCAM-1 for 30 min at room temperature, washed, and trypsinized.
Endothelial-PMN interactions in the flow chamber
Cell adhesion was examined morphometrically from digitized video recordings of perfusion experiments using computer-based image recognition. Corresponding to the above experiments, HUVEC monolayers were grown on coverslips and treated according to the pre-incubation setup described above. After washing, the coverslips were placed within a heatable metal case located on the stage of an inverted microscope (DMIRB, Leica, Bensheim, Germany). The flow rate Q in ml/sec necessary to produce the desired shear stress τw could be calculated according to the formula Q = (h2wτw)/6μ, in which h is the chamber height, w is the width and μ is the viscosity of the perfusate (0.007 poise for isolated neutrophils). We performed the experiments at a shear stress of 2 dynes/cm2. PMN suspension (106 cells/mL) was perfused through the flow chamber via a syringe pump. Total PMN adhesion, including rolling and firm interactions with the endothelial monolayer, was determined from five randomly chosen fields of view (200 × magnification) at the end of each 10 min perfusion period. PMN were considered to be adherent (sticking) when a cell was moving less than one cell diameter within 10 sec (). The number of rolling interactions between PMN and the endothelial cell monolayer were calculated from 60 video sequences derived from a single field of view over a period of 3–4 min during each experimental run. Rolling velocities (μm/s) were calculated as previously described (CitationNohé et al 2002, Citation2003). PMN with rolling velocities of 0.7 μm/s were counted as rollers. Data are expressed as percent sticking or rolling cells compared with controls.
Statistical analysis
Cell surface antigen expression was measured on the basis of mean fluorescence intensities calculated from 5000 single events detected by the flow cytometer for each sample. The effects of the lipooxygenase inhibitors in the flow cytometer analysis are expressed as percent expression of TNF-α activation alone minus the unstimulated expression of adhesion molecules on HUVECs. All data were tested for normal distribution and transformed where necessary. Effects of treatment (pre-, co-, post-inhibition) and effects of the derivative concentration on adhesion molecule expression were examined using an analysis of variance (ANOVA) followed by Bonferroni-Holm adjustment. As indicated by an R-square of 0.82, the ANOVA attributed 82% of the variation to the model. The inhibitor concentration that lead to a 50% inhibition of adhesion molecule expression (IC50) was calculated, assuming a logarithmic dose-response relationship. Unless otherwise stated, data are presented as means ± standard deviation. Statistical significance was set at p ≤ 0.05. Statistical computation was carried out using JMP software (SAS Institute, Cary, NC, USA).
Results
Effects of the timing and concentration of phenidone administration on the adhesion molecule expression of activated HUVECs
The expression of ICAM-1, VCAM-1, and E-selectin on HUVECs was stimulated by TNF-α (0.5 ng/mL). We measured the inhibitory effect of phenidone on adhesion molecule expression when added either two hours before (pre-incubation), at the same time as (co-incubation), or two hours after (post-incubation) the stimulation of HUVECs by TNF-α. The experimental setup is shown in . Three different phenidone concentrations were tested: 0.5, 1.0, and 2.0 mM. The results are shown in . Phenidone significantly decreased the expression of adhesion molecules on the surface of HUVECs when administered two hours before the stimulation of HUVECs by TNF-α (p < 0.01, (n = 4). The inhibition of adhesion molecule expression was still significant when phenidone and TNF-α were co-administered (co-incubation). In contrast, when phenidone was given two hours after the stimulation of HUVECs by TNF-α no decrease in adhesion molecule expression was observed. Furthermore, the inhibition of adhesion molecule expression was concentration-dependent (p < 0.01). The highest phenidone concentration (2.0 mM) led to the greatest inhibition of adhesion molecule expression on HUVECs. There was no statistical difference between the individual inhibitions of the three adhesion molecules tested (p = 0.14).
Figure 3 Inhibition of adhesion molecule expression by phenidone.
The decrease of adhesion molecule expression (ICAM-1, VCAM-1, E-selectin) is plotted in a logarithmic dose-response relationship (curve fitting). X-axis: Concentration of phenidone (μM). Y-axis: Per cent inhibition of adhesion molecule expression in relation to maximal TNF-α induced expression. Fine-dotted lines: Pre-incubation – phenidone was added 2 hours before TNF-α stimulation. Coarse-dotted line: Co-incubation – simultaneous application of phenidone and TNF-α. Solid line: Post-incubation – phenidone was added 2 hours after TNF-α stimulation.
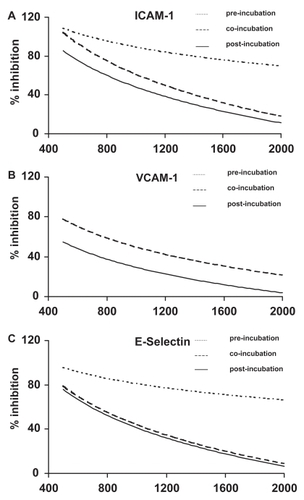
Static effects of phenidone derivatives on the adhesion molecule expression of activated HUVECs
The ability of the phenidone derivatives 4-methyl-phenidone, 5-methyl-phenidone, 4-4-dimethyl-phenidone, 5-phenyl-phenidone, and 5-methyl-1,(2,5-di-chloro-phenyl)-3-pyrazolidone to inhibit the adhesion molecule expression on HUVECs was tested when they were administered before the activation of HUVECs (pre-incubation). HUVECs were incubated with increasing concentrations of the derivatives between 125 and 2000 μM. All experiments were repeated at least three times. To quantify the ability of the derivatives to inhibit adhesion molecule expression, we calculated the inhibitor concentration that lead to a 50% inhibition of adhesion molecule expression (IC50), assuming a logarithmic dose-response relationship. The results are shown in . The expression of ICAM-1 was significantly reduced after pre-incubation of HUVECs with 4-4-dimethyl-phenidone and 5-phenyl-phenidone (IC50 382 ± 67 μM and 202 ± 102 μM, respectively) (p < 0.01) compared with the inhibition of adhesion molecule expression by phenidone (IC50 1128 ± 371 μM). Similarly, the expression of E-selectin was significantly reduced with these two compounds (IC50 216 ± 138 μM and 169 ± 41 μM) compared to phenidone (IC50 861 ± 338 μM). In contrast, 4-methyl-phenidone and 5-methyl-phenidone did not reduce adhesion molecule expression more effectively than phenidone (4-methyl-phenidone: IC50 of 1542 ± 1062 μM for ICAM-1 and 619 ± 183 μM for E.-selectin; 5-methyl-phenidone: IC50 1615 ± 819 for ICAM-1 and 1461 ± 774 μM for E-selectin). No inhibition of the adhesion molecule expression was observed with 5-methyl-1,(2,5-di-chloro-phenyl)-3-pyrazolidone (not shown). Also, compared with phenidone, the expression of VCAM-1 was not significantly reduced after pre-incubation with any of the tested derivatives.
Figure 4 Inhibition of ICAM-1, VCAM-1, and E-selectin by phenidone and derivatives.
IC50: The inhibitor concentration yielding 50% inhibition of adhesion molecule expression. *=significant reduction of ICAM-1, and E-selectin expression after preincubation with the denoted derivative, compared to phenidone (p < 0.01).
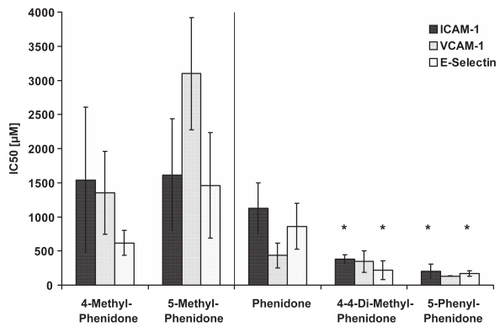
Figure 5 Endothelial-PMN interactions in the flow chamber.
Endothelial cell surface layer after activation with TNF-α for 4 hours. The experiments were performed at a shear stress of 2 dyn/cm2 with PMN (106 cells/mL). A: PMN were considered to be adherent (sticking) when a cell moved less than one cell diameter within 10 sec. Four adherent PMNs (flashes) were counted from digitized video recording. B: The same endothelial cell surface as shown in A. PMNs with rolling velocities of 0,7 μm/s were counted as rollers. In A and B four rolling PMNs were marked by circles. C: Endothelial cell surface layer after pre-incubation with 4-4- dimethyl-phenidone (400 μM). No adherent PMNs were counted from this sequence. D: The same endothelial cell surface as shown in C. Only 1 rolling PMN is counted.
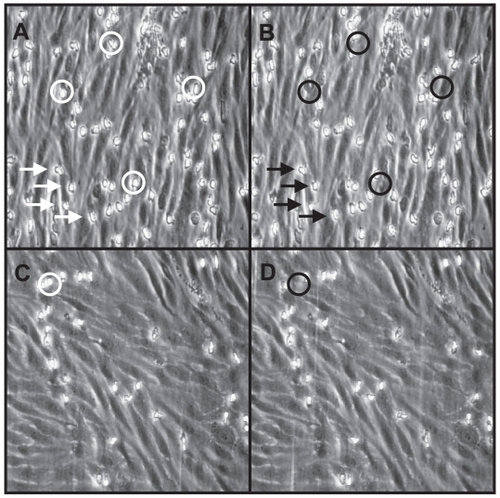
Dynamic adhesion of polymorphonuclear granulocytes to HUVECs
Under venular shear forces (2 dynes/cm2) PMN adhesion to untreated endothelial cells was negligible after 10 min of perfusion (not shown). On cytokine-activated HUVECs, total PMN adhesion increased 10-fold (). In subsequent experiments, the effect of 4-4-dimethyl-phenidone, which significantly reduced adhesion molecule expression in the static experiments, was compared to phenidone. Pretreatment of endothelial cells with 4-4-dimethyl-phenidone or phenidone (both 400 μM) prior to cytokine-activation significantly reduced total PMN adhesion (p < 0.01; ) compared with untreated activated HUVECs. When rolling and firm adhesion were examined separately, it became obvious that 4-4-dimethyl-phenidone had a significantly stronger inhibitory effect on rolling interactions than phenidone (p < 0.05). However, the number of adherent PMN was not significantly reduced after preincubation of HUVECs with 4-4-dimethyl-phenidone rather than phenidone ().
Figure 6 Rolling and adhesion of PMN to TNF-α stimulated HUVECs after pre-incubation with phenidone or 4-4-dimethyl-phenidone.
The ability of phenidone and 4-4-dimethyl-phenidone to inhibit rolling and adhesion of PMNs is shown as per cent of rolling and adhesion to untreated HUVECs. *=significant reduction of rolling when the HUVECs were pre-incubated with 4-4-dimethyl-phenidone instead of phenidone (p < 0.01).
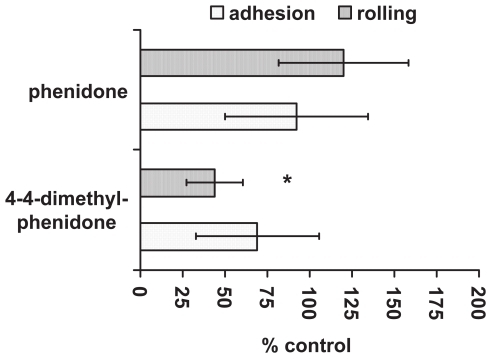
Discussion
The cytokine-triggered expression of adhesion molecules on the surface of vascular endothelial cells is observed in states of severe sepsis, ischemia reperfusion injury, or SIRS. The inhibition of adhesion molecule expression on activated vascular endothelial cells could be of therapeutic interest for the attenuation of leukocyte-mediated tissue injury (CitationSingbartl and Ley 2000; CitationPeters et al 2003). Using cell-surface antigen analysis and a dynamic adhesion assay, we were able to demonstrate that treatment of cytokine-activated HUVECs with the lipoxygenase inhibitor 1-phenyl-3-pyrazolidinone (phenidone) reduced both adhesion molecule expression and subsequent adhesion of PMN to vascular endothelial cells under postcapillary shear stress. Compared to phenidone, treatment of HUVECs with the derivatives 4-4-dimethyl-phenidone and 5-phenyl-phenidone was significantly more effective.
The inhibition of adhesion molecule expression was dependent on the time and concentration of the inhibitor. In addition, while treatment before or at the same time as cytokine-triggered activation of adhesion molecule expression led to a reduced adhesion molecule expression, no beneficial effect could be observed when phenidone was administered two hours after TNF-α treatment of HUVECs. Similarly, an effect of phenidone could be demonstrated on leukocyte adhesion in animal models. Leukocyte adhesion was decreased and capillary permeability increased during ischemia-reperfusion injury after pretreatment with phenidone in a hamster cheek pouch model and an ischemic rat brain damage model (CitationBertuglia et al 1993; CitationWakatsuki et al 1999). The results of these studies indicate that phenidone affects adhesion molecule expression in vivo. However, it is not clear from these functional studies whether phenidone affects adhesion primarily by inhibition of endothelial adhesion molecules or more proximal steps in the inflammation cascade. We observed a significant inhibitory effect of phenidone on the expression of the endothelial-cell-specific adhesion molecules ICAM-1, VCAM-1, and E-selectin only when it was administered two hours before or simultaneously with TNF-α.
In subsequent experiments, we tested five derivatives to determine whether they might have a stronger inhibitory effect on adhesion molecule expression than phenidone. In a previously published study, a series of 1-phenyl-3-pyrazolidinones were tested with respect to their ability to inhibit lipoxygenase. In this study, the lipoxygenase inhibitory potency was correlated with the lipophilicity of the compounds. Furthermore, the introduction of a substituent at position four of the pyrazolidinone improved the potency. In our study, we used those derivatives found in a previous study (CitationHlasta et al 1991) to inhibit 5-lipoxygenase most effectively, and tested them for their ability to inhibit adhesion molecule expression on activated HUVECs. The derivatives 4-4-dimethyl-phenidone and 5-phenyl-phenidone decreased the adhesion molecule expression of ICAM-1 and E-selectin significantly better than phenidone. We observed that the inhibitory potential of the derivatives on adhesion molecule expression increased as more and more lipohilic side groups were introduced in the molecule.
Pyrazolidinones are combined lipoxygenase and cyclooxygenase inhibitors (CitationHlasta et al 1991). Several mechanisms of action are conceivable behind adhesion molecule inhibition by pyrazolidinones. These include lipoxygenase inhibition, cyclooxygenase inhibition, antioxydative effects, or a combination of the above. A group tested the effect of nordihydroguaiaretic acid (NDGA), a potent lipoxygenase inhibitor, on the cytokine-triggered inhibition of ICAM-1 and VCAM-1 on HUVECs (CitationLee et al 1997). The authors of that study showed that both adhesion molecules were effectively inhibited at low concentrations of NDGA (IC50: 50 μM). Their results, like ours, demonstrated that an inhibitory effect only occurred when the inhibitor was added before the cytokine-triggered activation of adhesion molecule expression. Subsequent analysis showed that NDGA inhibited the promotor activity of the gene coding for VCAM-1 by decreasing the functional activity of NF-κB. Several studies demonstrated in similar series of experiments that adhesion molecule expression is inhibited by lipoxygenase inhibitors interacting with the nuclear transcription factor AP-1 (CitationAhmad et al 1994). The activation of transcription factors NF-κB and AP-1 by TNF-α triggers the transcription and expression of E-selectin, VCAM-1, and ICAM-1 in HUVECs. The promotors of the corresponding genes possess three, two, and one binding site(s) for NF-κB, respectively (CitationSchindler and Baichwal 1994; CitationLadebur and Parks 1995; CitationSheppard et al 1995). It has been shown that lipoxygenase is involved in the signal transduction pathway through the RhoA/protein kinase Ca-dependent activation of NF-κB (CitationLos et al 1995; CitationBolick et al 2005). Therefore, compounds with improved lipoxygenase inhibition activity could influence the adhesion molecule expression on HUVECs by affecting the activity of NF-κB and AP-1. However, we did not measure a possible correlation between the lipoxygenase activity of the derivatives and the inhibitory effect on the adhesion molecule expression. For most liopxygenase inhibitors, the reported IC50 for lipoxygenase inhibition is less than that for adhesion molecule inhibition by a factor of about ten (CitationHlasta et al 1991).
In previous studies, it was shown that the cyclooxygenase inhibitors diclofenac, ibuprofen, and salicylates were able to decrease the expression of adhesion molecules on vascular endothelial cells (CitationKapiotis et al 1996; CitationPierce et al 1996; CitationSakai 1996). However, high concentrations were necessary to achieve an inhibition of ICAM-1 and VCAM-1. The majority of cyclooxygenase inhibitors did not affect E-selectin expression. Further, the cyclooxygenase inhibitor indometacin did not have any effect on adhesion molecule expression on vascular endothelial cells. In contrast to lipoxygenase inhibitors, which lead to an inhibition of adhesion molecule synthesis, cyclooxygenase inhibitors were able to downregulate the number of adhesion molecules already expressed on the surface of endothelial cells. We did not observe a postranslational effect in our experiments with combined lipoxygenase and cyclooxygenase inhibitors. Thus, cylooxygenase inhibition seems to be of less importance for the improved effectiveness of pyrazolidinones.
After treatment with phenidone, VCAM-1 expression on HUVECs was more effectively reduced than that of ICAM-1 and E-selectin. In addition, 4-4-dimethyl-phenidone and 5-phenyl-phenidone reduced the expression of ICAM-1 and E-selectin significantly better than phenidone. Both ICAM-1 and E-selectin participate in the transition from rolling to firm adhesion (CitationDe Caterina and Zampolli 2004; CitationWang et al 2004). Whereas E-selectin decelerates transiently rolling leukocytes, ICAM-1 and VCAM-1 contribute to firm adhesion by binding to β2-integrins on the neutrophil at slow rolling velocities (CitationSteeber et al 1998; CitationLey 2003). When we compared phenidone to 4-4-dimethyl-phenidone with respect to their ability to inhibit neutrophil rolling and firm adhesion (sticking) in dynamic flow chamber experiments, we found that rolling was significantly better inhibited after incubation with 4-4-dimethyl-phenidone than with phenidone. This result correlated with the significantly better inhibition of E-selectin by 4-4-dimethyl-phenidone than by phenidone.
We observed a higher rate of cell detachment when we incubated the HUVECs with inhibitor concentrations of 2000 μM. However, cell morphology was not altered after this treatment. In addition, adhesion molecule expression was preserved even at high concentrations of the inhibitors in our postincubation experiments. The preservation of functional activity as well as the unaltered cell morphology suggests that cell toxicity did not occur at these concentrations.
In summary, we found a correlation between reduced adhesion molecule expression on activated HUVECs and subsequently impaired adhesion of neutrophils following treatment with the phenidone derivatives 4-4-dimethyl-phenidone and 5-phenyl-phenidone. These lipoxygenase inhibitors might be of therapeutical interest in the treatment of overwhelming systemic inflammation during shock, trauma, and sepsis.
References
- AhmadMTheofanidisPMedfordRM1994Role of activating protein-1 in the regulation of the vascular cell adhesion molecule-1 gene expression by tumor necrosis factor-alphaJ Biol Chem2734616219468519
- BertugliaSColantuoniAIntagliettaM1993Effect of leukocyte adhesion and microvascular permeability on capillary perfusion during ischemia-reperfusion injury in hamster cheek pouchInt J Microcirc Clin Exp1313268262718
- BolickDTOrrAWWhetzelA200512/15-Lipoxygenase regulates intercellular adhesion molecule-1 expression and monocyte adhesion to endothelium through activation of RhoA and nuclear factor-kBArterioscler Thromb Vasc Biol252301716166569
- BoneRCBalkRACerraFB1992Definitions for sepsis and organ failure and guidelines for the use of innovative therapies in sepsis. The ACCP/SCCM Consensus Conference Committee. American College of Chest Physicians/Society of Critical Care MedicineChest1011644551303622
- CuzzocreaSRossiASerrainoI2004Role of 5-lipoxygenase in the multiple organ failure induced by zymosanIntens Care Med30193543
- De CaterinaRZampolliA2004From asthma to atherosclerosis – 5-lipoxygenase, leukotrienes, and inflammationN Engl J Med3504714702420
- EppihimerMJGrangerDN1997Ischemia/reperfusion-induced leukocyte-endothelial interactions in postcapillary venulesShock816259249908
- GaddiACiceroAFPedroEJ2004Clinical perspectives of anti-inflammatory therapy in the elderly: the lipoxigenase (LOX)/cycloxigenase (COX) inhibition conceptArch Gerontol Geriatr382011215066307
- HlastaDJCaseyFBFergusonEW19915-Lipoxygenase inhibitors: the synthesis and structure-activity relationships of a series of 1-phenyl-3-pyrazolidinonesJ Med Chem341560701903450
- JaeschkeHSmithCW1997Mechanisms of neutrophil-induced parenchymal cell injuryJ Leukoc Biol61647539201255
- KapiotisSSengoelgeGSperrWR1996Ibuprofen inhibits pyrogen-dependent expression of VCAM-1 and ICAM-1 on human endothelial cellsLife Sci582167818649201
- LedeburHCParksTP1995Transcriptional regulation of the intercellular adhesion molecule-1 gene by inflammatory cytokines in human endothelial cells. Essential roles of a variant NF-kappa B site and p65 homodimersJ Biol Chem270933437822333
- LeeSFeltsKAParryGC1997Inhibition of 5-lipoxygenase blocks IL-1 beta-induced vascular adhesion molecule-1 gene expression in human endothelial cellsJ Immunol158340179120300
- LeyK2003The role of selectins in inflammation and diseaseTrends Mol Med9263812829015
- LosMSchenkHHexelK1995IL-2 gene expression and NF-kappa B activation through CD28 requires reactive oxygen production by 5-lipoxygenaseEmbo J143731407641692
- NoheBRuoffHJohannesT2002A fish oil emulsion used for parenteral nutrition attenuates monocyte-endothelial interactions under flowShock182172212353921
- NoheBKieferRTPloppaA2003The effects of fresh frozen plasma on neutrophil-endothelial interactionsAnesth Analg972162112818969
- PetersKUngerREBrunnerJ2003Molecular basis of endothelial dysfunction in sepsisCardiovasc Res60495714522406
- PierceJWReadMADingH1996Salicylates inhibit I kappa B-alpha phosphorylation, endothelial-leukocyte adhesion molecule expression, and neutrophil transmigrationJ Immunol156396198621937
- ReillyKBSrinivasanSHatleyME200412/15-Lipoxygenase activity mediates inflammatory monocyte/endothelial interactions and atherosclerosis in vivoJ Biol Chem27994405014676201
- RamosCLKunkelEJLawrenceMB1997Differential effect of E-selectin antibodies on neutrophil rolling and recruitment to inflammatory sitesBlood893009189108422
- SakaiA1996Diclofenac inhibits endothelial cell adhesion molecule expression induced with lipopolysaccharideLife Sci582377878691982
- SchindlerUBaichwalVR1994Three NF-kappa B binding sites in the human E-selectin gene required for maximal tumor necrosis factor alpha-induced expressionMol Cell Biol145820317520526
- SingbartlKGreenSALeyK2000Blocking P-selectin protects from ischemia/reperfusion-induced acute renal failureFaseb J14485410627279
- SingbartlKLeyK2000Protection from ischemia-reperfusion induced severe acute renal failure by blocking E-selectinCrit Care Med2825071410921586
- SheppardAMMcQuillanJJIademarcoMF1995Control of vascular cell adhesion molecule-1 gene promoter activity during neural differentiationJ Biol Chem270371097533155
- SteeberDACampbellMABasitA1998Optimal selectin-mediated rolling of leukocytes during inflammation in vivo requires intercellular adhesion molecule-1 expressionProc Natl Acad Sci USA95756279636189
- VedderNBWinnRKRiceCL1988A monoclonal antibody to the adherence-promoting leukocyte glycoprotein, CD18, reduces organ injury and improves survival from hemorrhagic shock and resuscitation in rabbitsJ Clin Invest81939443278007
- WakatsukiAIzumiyaCOkataniY1999Oxidative damage in fetal rat brain induced by ischemia and subsequent reperfusion. Relation to arachidonic acid peroxidationBiol Neonate76849110393992
- WangYZhouBLiJ2004Inhibitors of 5-lipoxygenase inhibit expression of intercellular adhesion molecule-1 in human melanoma cellsActa Pharmacol Sin25672715132836
- ZhaoLMoosMPGrabnerR2004The 5-lipoxygenase pathway promotes pathogenesis of hyperlipidemia-dependent aortic aneurysmNat Med109667315322539