Abstract
Axonal regeneration does not occur easily after an adult central nervous system (CNS) injury. Various attempts have partially succeeded in promoting axonal regeneration after the spinal cord injury (SCI). Interestingly, several recent therapeutic concepts have emerged from or been tightly linked to the researches on brain development. In a developing brain, remarkable and dynamic axonal elongation and sprouting occur even after the injury; this finding is essential to the development of a therapy for SCI. In this review, we overview the revealed mechanism of axonal tract formation and plasticity in the developing brain and compare the differences between a developing brain and a lesion site in an adult brain. One of the differences is that mature glial cells participate in the repair process in the case of adult injuries. Interestingly, these cells express inhibitory molecules that impede axonal regeneration such as myelin-associated proteins and the repulsive guidance molecules found originally in the developing brain for navigating axons to specific routes. Some reports have clearly elucidated that any treatment designed to suppress these inhibitory cues is beneficial for promoting regeneration and plasticity after an injury. Thus, understanding the developmental process will provide us with an important clue for designing therapeutic strategies for recovery from SCI.
Introduction
Axonal regeneration is a fundamental step in the process of recovering from spinal cord injury (SCI). However, the axons in the adult central nervous system (CNS) cannot regenerate easily, which primarily causes the lack of adequate restorative therapy for the SCI so far. Several attempts have been made to promote regeneration, and some advances have been obtained. Importantly, these attempts appear to be the applications of certain extensively revealed mechanisms of brain development. Although the axons cannot regenerate easily in an adult brain, in the developing brain, differentiating neurons elongate the axons easily to very distal areas; this information is of utmost importance and is required to be considered post SCI. What is the difference between the ability of elongating the axons in the adult and developing brain? Understanding the mechanism of tremendous axonal elongation and navigation during development and the differences between the environments of adult and developing CNS has provided us with important clues for succeeding in regenerating axons after brain injuries. Although several allusions for the therapy have also been brought by the comparison between the peripheral nervous system (PNS) and the CNS because axons can regenerate in the former system but not in the latter, in this review, we will focus on and compare the differences between the adult and developing brain. We have briefly summarized the differences discussed in this review in . From this view, we can consider how the recent concepts and strategies for regenerating axons in the adult CNS have emerged and can be developed. Although several excellent reviews have yet described the importance of the relation of regenerative therapy with the developmental mechanisms (CitationSchwab and Bartholdi 1996; CitationHarel and Strittmatter 2006), we will overview the whole aspects of its relations as much as possible with recent reports, and will emphasize that collaboration with developmental and clinical neuroscience is more needed.
Figure 1 Comparison between the environments of a developing brain and an adult brain after SCI. A: Axons (blue line; in this case, CST) projected up to a long distance through a specific route. Even after the injury (red), axons can regenerate more extensively than in adults. Compensatory sprouting also occurs with high plastic ability (orange arrow). B: In the adult brain, axons (green line; in this case, CST) cannot regenerate after the injury (red), but compensatory sprouting occur in the rostral positions (orange arrows); however, the extent to which this occurs is not greater than that in the developing brain. C: Different components and their properties involved in the developing and adult brains after the SCI. Each function is represented as the role toward axonal outgrowth (regeneration) and sprouting (plasticity). The properties of the components are presented within the parentheses. The strategies for the therapy targeting each component are represented in the right column. The details can be found in the text.
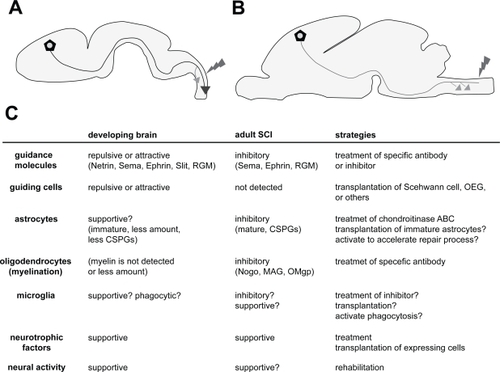
Axonal regeneration in the developing brain
Many earlier reports indicate that the axons in the early postnatal spinal cord can regenerate more easily after an injury compared to those in an adult (: CitationBerstein and Stelzner 1983; CitationBates and Stelzner 1993; CitationFirkins et al 1993). This finding provides two main important suggestions. First, early brain tissue possesses favorable factors and environment for axonal growth, and the second, younger neurons themselves have a greater capacity for outgrowth than adult neurons. The first suggestion is a well-accepted concept, and many inhibitory factors in the adult brain have been identified up till now. We have reviewed these factors in the coming chapters; however, the important point is that understanding the favorable environment for axonal growth in the developing brain may generate a new approach in the designing of a therapy for SCI. Indeed, in many classical experiments, embryonic spinal cord was transplanted in the lesion site of the postnatal and adult spinal cord (CitationBregman et al 1989, Citation1993; CitationIwashita et al 1994). In the early postnatal case, injured axons demonstrated immense regeneration passing through the transplanted embryonic tissue. In the adult case, axons could regenerate slightly but not dramatically. This also supports the abovementioned two suggestions, that early tissue is favorable for axonal growth, and younger neurons may possess a greater capacity of growth. It may be clear that younger neurons or certain types of neurons possess a much higher ability of elongating axons compared to other neurons, as suggested by the reports indicating that transplanted embryonic cortical neurons in the injured cortex can extend the axons even through the adult spinal cord (CitationGaillard et al 2007), and that neurons in different mouse strains have different regenerative abilities after SCI (CitationDimou et al 2006). However, many of the recent approaches have partially succeeded in regenerating axons by suppressing the inhibitory cues in the adult brain, suggesting that even the adult neurons have the ability to re-elongate axons. From this view, generating a favorable environment for axonal growth in the injured site should be one of the important goals for establishing therapeutic interventions.
Axonal network formation by guidance molecules in the developing brain
We should first understand how the complicated and precise axonal network is formed in the developing brain. During development, several steps are required to form a complex structure and for the functioning of the brain. First, undifferentiating neural stem cells or progenitor cells proliferate extensively around the ventricle called ventricular zone to reserve a large number of neurons and glial cells for the future adult brain. Next, some of these cells start migrating to specific areas and differentiating into neurons. After reaching the final position, the neurons start projecting the axons to the target area. In many cases, the axons are facilitated to pass through specific routes and targeted to specific areas. This process is very surprising because this navigation is quite precise, and in some cases, the neurons project the axons to a great distance from the cell bodies. Since 1990’s, the mechanism of this precise navigation has been revealed by various studies identifying the axonal guidance molecules. Netrin, Ephrin, Semaphorin, and Slit are the representative guidance molecules (for review CitationHuber et al 2003). In vitro culture system and the analyses from knockout mice have clearly revealed that these molecules have an important role in navigating axons in the developing brain. For example, corticospinal tract (CST), the main tract connecting the sensorimotor cortex to the spinal cord for regulating the motor function (), is also navigated by these molecules. In the first step, Sema3A expressed in the upper layers of the cortex repulses the axons of neurons in the deeper layer (layer V in the future) to the deeper white matter (CitationPolleux et al 1998, Citation2000). Slit2 then repulses the axons after passing the internal capsule, for projecting the specific route into the cerebral peduncle (CitationBagri et al 2002). After having passed the cerebral peduncle, most of the fibers cross the midline and go through to the contralateral side. It is indicated that Netrin and its receptors, Dcc and Unc5H3 contribute to this decussation (CitationFinger et al 2002). While passing the spinal cord, Ephrin-B3 repulses the CST not to cross the midline (CitationKullander et al 2001; CitationYokoyama et al 2001).
Recent reports have demonstrated other important molecules responsible for guiding axons, morphogens. Morphogens – such as Sonic hedgehog (Shh), Fibroblast growth factor (Fgf), Bone morphogenetic protein (Bmp), and Wnts – are the signaling molecules that diffuse and establish a gradient in the embryonic tissues. This gradient signal plays a crucial role in arealizing the tissues by changing the cells from single type to heterogeneous populations. These molecules play a similar role in the developing CNS. The famous example is that of the developing spinal cord in which Shh, which is expressed in the most ventral place called floor plate, is diffused dorsally and establishes a gradient concentration. As a result, several clusters of neuronal populations are generated in the ventral spinal cord depending on the concentration of Shh (CitationJacob and Briscoe 2003). Intriguingly, recent papers have indicated that these morphogens regulate not only the specification of tissue areas and cell population, but also navigate the axons (for review CitationCharron and Tessier-Lavigne 2005). Again, CST passes through the spinal cord up to a long distance mainly in the first postnatal week in rodents (CitationGianino et al 1999; CitationJoosten and Bär 1999). Interestingly, developing CST expresses Ryk, one of the Wnts receptors, and is pushed down by Wnts signals in the spinal cord after decussation (CitationLiu et al 2005). Wnt1 and 5a are expressed in a rostral to caudal gradient that repulses the CST into lower levels. Thus, CST can be guided for a long distance to the final targets by Wnts signaling.
One ultimate strategy for facilitating definite regeneration after the SCI may be to reconstruct these guidance cues in precise positions spatially and temporally like in the developing brain. CitationJoosten et al (1995) showed that injured CST in adult rats could regenerate by the local application of cervical spinal cord extracts which were harvested at the time that developing CST axons reached this spinal cord level. On the other hand, the injured axons could not regenerate when spinal cord extracts were harvested at younger or older age. This data implied that specifically organized expression of guidance molecules seen in the development is also optimal for regeneration. It is known, however, that some of these guidance molecules are still expressed in the adult developed brain in a different manner or are abnormally re-expressed at the lesion site. This appears to make the precise reconstruction of guidance cues difficult. In addition, some of these molecules are reported to suppress the regeneration of injured axons. Alternatively, recent researches have partly succeeded in promoting regeneration by suppressing the expression of these guidance molecules. We will focus on this issue in the later chapters.
Guiding cells that facilitate axonal network formation in the developing brain and their transplantation
Besides the axon guidance molecules, certain types of specific guiding cells navigate the axons in the developing brain. These cells were reported earlier as “guidepost cells” in the embryonic limb bud of grasshopper (CitationBentley and Caudy 1983), and in the following papers, several other types of navigating cells were identified in the corpus callosum (CitationSilver and Ogawa 1983; CitationShu and Richards 2001), olfactory tract (CitationSato et al 1998), and thalamocortical axons (CitationLópez-Bendito et al 2006) of the developing rodent brain. Although the existence of guiding cells in the developing CST is also speculated from the observation of immature astrocytes discovered in the route of CST in early postnatal days (CitationJoosten and Gribnau 1989), unfortunately, there are no clear evidences regarding their role in guiding axons.
As developmentally, early brain tissues possess a favorable environment for axonal growth, implanting fetal nerve tissue may be a simple way of promoting regeneration after SCI. However, obtaining this tissue is extremely difficult, thus rendering its implantation for clinical therapy rather questionable. The next strategy is to transplant guiding cells specific to the axons in the spinal cord; however, as mentioned earlier, specific guiding cells for the CST or other axons in the spinal cord have not been identified yet. Hence, the ongoing approach is to transplant guiding or supportive cells that can be obtained from other parts of the body. Historically, two types of cell have mainly been attempted for conducting transplantation: the peripheral nerve (Schwann cells) (CitationRichardson et al 1980; CitationDavid and Aguayo 1981; CitationTakami et al 2002) and olfactory ensheathing glial cells (OEG) (CitationLi et al 1997; CitationRamón-Cueto et al 1998). These approaches were not designed from the mechanisms of developmental processes; rather, they were developed from the mechanism of regeneration and axonal outgrowth in the adult brain. Axons of PNS can regenerate more easily than those of CNS, raising the possibility that peripheral nerves or Schwann cells act as favorable substrates for axonal growth. OEGs are the specialized glial cells ensheathing olfactory axons of neurosensory cells in the olfactory epithelium. Neurosensory cells (and their axons, olfactory axons) are continuously replaced and newly formed in the adult, suggesting that OEGs support axonal outgrowth. These transplanted cells bridge the lesion site after SCI and, to a certain extent, effect regeneration and functional recovery. These experiments may provide us a new insight in to establishing therapeutic methods from the basic research on brain development: discoveries and identification of guiding cells in the developing brain may open a new strategy to promote regeneration.
Distinct tissue responses in young and adult injured CNS: the role of glial cells
One of the points of differences between adult and developing brain is that adult CNS includes not only neurons but also a large number of matured glial cells. These cells are clearly activated in the injured site to repair the tissue, which appears to affect the outgrowth of injured axons.
Astrocytes are the cells that are activated after the injury and form a glial scar to repair the injured tissue. Glial scar formation is an important process for tissue repair. Conditional and selective ablation of activated astrocytes after SCI delays the repair process and caused motor deficits (CitationFaulkner et al 2004). Conditional ablation of astrocytic activation using Stat3 conditional knockout model also diminishes the recovery (CitationOkada et al 2006). These reports indicate that a glial scar plays an important role in tissue repair by protecting the neighboring intact tissues from excessive degeneration, inflammation, demyelination, etc. Unfortunately, although the glial scar is a key contributor to repair, it simultaneously inhibits axonal regeneration (CitationRudge and Silver 1990). An important factor that suppresses the regeneration expressed by astrocytes is chondroitin sulfate proteoglycans (CSPGs) (CitationMckeon et al 1991; CitationJones et al 2003; CitationTang et al 2003). CSPGs are glycoproteins within extracellular matrices that function as barriers that inhibit the penetration of regenerating axons into the lesion sites. By either treating chodroitinase ABC, which is the bacterial enzyme that digests CSPGs, or by using transgenic mice that express chodroitinase ABC in astrocytes, it was possible to promote regeneration after SCI (CitationBradbury et al 2002; CitationCafferty et al 2007); although, functional recovery was not achieved in transgenic mice. In the developing brain, astrocytes are generated from neural progenitor cells in the later period of development compared with neurons (CitationTemple 2001). Thus, astrocytic responses do not occur in early embryonic brain injury (CitationUeno et al 2006). SCI in early postnatal days activates astrocytes but no more remarkably than in adults (CitationBarrett et al 1984; CitationFirkins et al 1993) possibly due to the lower number of astrocytes and lesser extent of maturation. Interestingly, although CSPGs are basically considered as barriers to axonal elongation in the developing brain (CitationSnow et al 1990; CitationKatoh-Semba et al 1995), they are not upregulated remarkably in the young reactive astrocytes after an injury (CitationMckeon et al 1991; CitationDow et al 1994). It may imply that axons in a younger brain can regenerate easily due to the lack of inhibitory factors from activated astrocytes. Moreover, others reported that reactive astrocytes in the younger brains could be permissive for or promote axonal growth (CitationRudge and Silbver 1990; CitationBähr et al 1995). This suggests that there may be different types of astrocytes, particularly, an inhibitory and permissive type for axonal growth as indicated even in the amphibian which has a greater potential for regeneration (CitationReier 1979; CitationSinger et al 1979). The permissive type also looks like a similar phenotype as seen in several regions of the developing brain as guiding cells (see previous chapter). Thus, understanding the mechanism of astroglial activation into different types and implanting supportive astrocytes into the lesion may establish a new approach to promote axonal outgrowth (CitationDavies et al 2006).
Although, the reason for which scar formation is required to block regeneration is not clear, at least, the most important role of the scar should be finishing and enclosing the repairing (inflammatory) response to protect from neighboring intact CNS tissue. Thus, scar response should not be eliminated from the list of possible strategies that can be considered for the therapy. Instead, because scar inhibits the outgrowth of axons, completing the repair process speedily and compacting the scar to as small as possible, or deleting specific molecules that suppresses regeneration but not scar formation, should be the considered approach.
Oligodendrocytes are the glial cells that are involved in myelination. It is widely accepted that these cells (or its components and debris) also inhibit axonal regeneration post SCI. Earlier experiments performed by Schwab’s group revealed that in the rats in which oligodendrocytes (and myelin) are deleted, axonal regeneration after SCI is promoted more than in control rats (CitationSavio and Schwab 1990). Subsequently, they generated a monoclonal antibody known as IN-1 that recognizes myelin-associated inhibitory proteins, and revealed that treatment using this antibody promotes axonal regeneration (CitationSchnell and Schwab 1990; CitationBregman et al 1995). Then, Nogo was identified as the inhibitory protein that is recognized by IN-1 (CitationChen et al 2000; CitationGrandPré et al 2000; CitationPrinjha et al 2000). Myelin-associated glycoprotein (MAG) (CitationMukhopadhyay et al 1994; CitationMcKerracher et al 1994), and oligodendrocyte myelin protein (OMgp) (CitationKottis et al 2002; CitationWang et al 2002) were also identified as the inhibitory proteins for axonal outgrowth. Surprisingly, all of these three inhibitory proteins exert their inhibitory function through one common receptor, Nogo-66 receptor (NgR) (CitationFournier et al 2001; CitationDomeniconi et al 2002; CitationLiu et al 2002; CitationWang et al 2002) and its receptor complex p75 (CitationWang et al 2002; CitationWong et al 2002; CitationYamashita et al 2002), Lingo-1 (CitationMi et al 2004), and TROY (CitationPark et al 2005; CitationShao et al 2005) (for review, CitationYamashita et al 2005). Extensive studies using Nogo antibody (CitationSchnell and Schwab 1990; CitationBregman et al 1995) and Nogo knockout mice (CitationKim et al 2003; CitationSimonen et al 2003) and NgR (CitationKim et al 2004) have demonstrated that this is one of the critical factors inhibiting regeneration in SCI; although, several reports were unable to prove similar roles of Nogo and NgR as inhibitors (CitationZheng et al 2003, Citation2005). Similarly, MAG (CitationBartsch et al 1995), p75 (CitationSong et al 2004) deficient mice do not undergo regeneration after SCI, but treatment using Lingo-1 antagonist promotes axonal sprouting (CitationJi et al 2006). Several controversial results regarding the role of myelin-associated proteins in regeneration suggest that the inhibitory cues may affect the injured axons through more varieties of molecules and through complicated mechanisms.
Myelination starts later in CNS development after P14 in the spinal cord of the rodents (CitationSchwab and Schnell 1989; CitationJoosten et al 1989; CitationHsu et al 2006). This may also imply that the strong capacity of regeneration in younger animals is achieved due to lesser amounts of myelin components, although Nogo is expressed early in postnatal period by neurons (CitationHuber et al 2002). Interestingly, less amount of myelin can also enable the developing axons to display the plastic changes (plasticity) during the early postnatal period for learning and adapting to their external environment. This fact could also lead to understanding the mechanism that promotes plasticity after an adult CNS injury. Indeed, some studies succeeded in promoting plasticity after the injury by modifying myelin-components. We will discuss this issue in the next section.
Microglia is the last glial cell that is believed to be originated from mesenchymal lineage (monocyte or myeloid) (CitationChan et al 2007), which is different from other glial cells that are generated from neural progenitor cells (CitationTemple 2001). One interesting aspect regarding microglia is that this cell has different phases or types that are beneficial and detrimental for axons. One direct evidence that microglia is an inhibitory factor for regeneration is that treatment using minocycline, an inhibitor of activation of microglia, promotes recovery by decreasing the dieback of CST and cell death of oligodendrocytes (CitationStirling et al 2004; CitationFestoff et al 2006; CitationYune et al 2007). Depletion of hematogenous macrophages also promotes partial recovery (CitationPopovich et al 1999). Since minocycline has an additional neuroprotective effect (CitationYong et al 2004), a selective ablation of microglial cells using transgenic mice (CitationLalancette-Hébert et al 2007) may provide us with more convincing results regarding the actual role of microglia in SCI. The beneficial aspects of these cells are suggested from the fact that coculturing microglia with neurons can promote the extension of neurites (CitationNakajima et al 1989; CitationChamak et al 1994; CitationBouhy et al 2006). Indeed, microglia express neurotrophic factors (CitationElkabes et al 1996; CitationDougherty et al 2000). One report suggested that later activation of microglial cells in vivo by treatment of GM-CSF after SCI facilitates regeneration possibly due to BDNF expression by microglia (CitationBouhy et al 2006). Some other studies also succeeded in promoting recovery by transplanting microglia/macrophages (CitationPrewitt et al 1997; CitationRabchevsky and Streit 1997). Another beneficial role of microglia may be phagocytosis. As mentioned above, myelin-related protein is one of the key factors that inhibit regeneration. Microglia can phagocytose the myelin debris after CNS injury but not speedily and sufficiently (CitationGeorge and Griffin 1994; CitationBuss and Schwab 2003). Thus, promoting the phagocytic ability may be one of the strategies that can be employed for therapy (CitationVallières et al 2006). Transplantation of macrophages that are prestimulated by peripheral nerves (myelin components) into injured site promotes the recovery of motor function (CitationRapalino et al 1998). Although it is not clear whether this beneficial effect is due to the enhancement of phagocytic ability, one can deduce that in some way, an appropriate activation of microglia/macrophage targeted to autologous tissue (possibly myelin) is protective and effective in repair and regeneration (for review, CitationSchwartz et al 2006). In the developing brain, microglia infiltrate into the CNS around E10 in rodents and are believed to play a role in engulfing the dying apoptotic cells (CitationAshwell 1991). Interestingly, microglial cells express neurotrophic factors even in the developing brain (CitationElkabes et al 1996), and it is assumed that these cells may have some role to play in axonal growth (CitationChamak et al 1994; CitationStreit 2001), although a direct in vivo evidence is deficient. In this case, understanding the role of microglia in the injured brain may offer an important clue regarding its role in development.
Plasticity in development, and adult brain injuries
It is known that after SCI, compensatory axonal sprouting occurs in the upper level of the lesion (; CitationAoki et al 1986; CitationLi et al 1994; CitationWeidner et al 2001; CitationFouad et al 2001). For a long time, the reason behind the occurrence of slight functional recovery, unless spinal axons regenerate, was unclear; however, Bareyre et al suggested that the new sprouting axons in the upper level establish a new contact with the intraspinal interneurons and form a new neural circuit that may contribute to partial recovery (CitationBareyre et al 2004). Interestingly, the temporal pattern of the newly generated sprouting appears to be significantly similar to the developmental process of CST. As described above, axons in the CST are elongated in early postnatal life, and after some “waiting period” (approximately 3 days), passing axons start establishing collaterals; in other words, they begin sprouting (O’Leary and Terashima 1988; CitationGianino et al 1999; CitationJoosten and Bär 1999). Thus, an understanding of the mechanism of collateral formation in the developing brain will lead us to a new approach for promoting sprouting that may lead to functional recovery after SCI. In the developing brain, axonal collateral formation appears to be initiated by diffusible factors that emanate from their targets (CitationSato et al 1994; CitationJoosten et al 1994). Although the cues that induce collateral formation in the spinal cord are unidentified, a potential cue may be neurotrophic factors. NT-3 and BDNF are known to promote axonal branching in a variety of neurons. Indeed, NT-3 enhances developmental sprouting in the spinal cord, and also in the injured adult spinal cord (CitationSchnell et al 1994; CitationGrill et al 1997; CitationZhou et al 2003). Although some recent papers reveal contrasting results on NT-3, and propound instead that providing BDNF treatment to the cell body of CST promotes sprouting (CitationHiebert et al 2002; CitationHagg et al 2005; CitationVavrek et al 2006).
Interestingly, similar factors appear to be used for controlling the developmental plasticity and plasticity after adult brain injury. Neural activity is the first factor that influences the plastic changes. Developing visual cortex is one of the extensively studied areas regarding plasticity in development. Neural inputs from both eyes through the thalamus compete with each other in the developing visual cortex through neural activity. This competition normally forms specific ocular dominance columns in the visual cortex that respond to alternative eyes. Intriguingly, monocular deprivation during the critical period, which is the window period during early postnatal days when plastic changes can occur, shifts the response of neurons in both columns toward the dominantly activated input from the opened eye (for review, CitationHensch 2005). Thus, neural activity appears to be the first step in inducing plasticity. In the case of SCI, many reports have indicated that rehabilitation – such as locomotor training and environmental enrichment, which supposedly enhance neural activity – promotes functional recovery in rodents (CitationLankhorst et al 2001; CitationVan Meeteren et al 2003; CitationHutchinson et al 2004; CitationEngesser-Cesar et al 2007). The mechanisms that improve function after rehabilitation are not fully understood; however, neural plasticity must be involved.
Neurotrophin is the next factor involved in plasticity. In the developing visual cortex, excess treatment or removal of BDNF, NT-4/5 prevents or delays ocular dominance formation (CitationCabelli et al 1995, Citation1997), and focal injection of NT-4/5 prevents the plastic shift in the visual cortex (CitationRiddle et al 1995). This implies that axons from each eye compete with each other through limited amount of neurotrophins in an activity-dependent manner, and only the ones that can receive neurotrophic factors are stabilized. In the SCI, neurotrophic factors appear to enhance plastic changes via sprouting, as mentioned above. Thus, the precise treatment using neurotrophic factors and stimulation of neural activity (it is related to good rehabilitation strategies) should be one of the key strategies to enhance plasticity after SCI.
The last factor contributing to plasticity is inhibitory molecules. The increasing number of inhibitory factors during postnatal development appears to terminate or decrease the overall plasticity of neural connections. CSPGs that inhibit regeneration after SCI are one of the inhibitory factors that also decrease plasticity through the developing brain. CSPGs organize a perineuronal net as the extracellular matrix. Treatment using chondroitinase ABC degrades CSPGs and reactivates the plasticity toward monocular deprivation in the adult visual cortex (CitationPizzorusso et al 2002). Interestingly, increasing myelin formation also appears to terminate the plastic changes in the visual cortex of younger brain. It is reported that the critical period is delayed in the visual cortex of NgR deficient mice (CitationMcGee et al 2005). As observed in younger animals, myelin components also inhibit plasticity after adult brain injury. For example, in Nogo–/– and NgR–/– mice, sprouting (plasticity) of axons was promoted after CNS injury (CitationLee et al 2004; CitationCafferty and Strittmatter 2006), and blocking NgR signals also enhanced sprouting rostral to the lesion site after SCI (CitationLi et al 2004, Citation2005; CitationLi and Strittmatter 2003).
In conclusion, all these instances suggest that plasticity of axons in the developing brain and adult brain after the injury appear to involve similar mechanisms. Thus, understanding the mechanism of plasticity during development will offer an important clue for developing a new therapeutic strategy for SCI by promoting plasticity.
Strategy for therapy: insights from guidance molecules
The last insights obtained from the researches on development are that a number of axonal guidance molecules guiding axons during development are re-expressed by the glial and inflammatory cells after the injury or continuously expressed in adult CNS cells. By now, Netrin1 (CitationWehrle et al 2005), EphA4 (CitationGoldshmit et al 2004), EphB2 (CitationBundesen et al 2003), Sema3, 7a (CitationDe Winter et al 2002; CitationPasterkamp et al 2003), and Slit1, 3 (CitationWehrle et al 2005) have been revealed to be expressed in the lesion site of SCI. Although these molecules have various roles in the repair process, such as activation of astrocytes (EphA4; CitationGoldshmit et al 2004), scar formation (EphB2; CitationBundesen et al 2003), and migration of adult progenitor cells (Netrin1; CitationPetit et al 2007), there are no doubts that some of these molecules also affect the outgrowth of injured axons. Sema3A is expressed mainly in the fibroblasts after injury (CitationDe Winter et al 2002), and the specific inhibitor of Sema3A clearly promotes the regeneration of axons (CitationKaneko et al 2006). We also demonstrated an additional example. Repulsive guidance Molecule (RGM), a glycosylphosphatidylinositol (GPI)-anchored membrane-bound protein, is another family of guidance molecules important for axonal development, which had been shown to navigate the optic nerve in the chick tectum to form a topographic map (CitationStahl et al 1990; CitationMonnier et al 2002; for review, CitationYamashita et al 2007). We as well as others elucidated that RGMa is also expressed in the injured tissue including astrocytes, microglia, oligodendrocytes, and neurons (CitationSchwab et al 2005; CitationHata et al 2006). Treatment with RGMa antibody after the SCI in a rat promotes axonal regeneration and synapse formation, and recovers behavioral function, as evaluated by BBB test (CitationHata et al 2006; CitationKyoto et al 2007). This data suggests that RGMa is one of the key inhibitory factors for axonal regeneration. Morphogens are also candidate molecules that inhibit regeneration. We have recently reported that BMP-2/4 expression is elevated in the lesion of SCI and administration of Noggin, a soluble BMP antagonist, promotes regeneration of CST (CitationMatsuura et al 2008). BMP is known as a repulsive guidance molecule to commissural axons in the developing spinal cord (CitationAugsburger et al 1999; CitationButler and Dodd 2003), suggesting that similar repulsive factors re-expressed in the adult lesion site.
One important issue is that the spinal cord has various descending and ascending axons and these have different regenerative reaction to therapeutic approaches (CitationDeumens et al 2005). In the developing brain, axons in each axon tract have different set of receptors for guidance molecules to select specific route for the targets. It appears that each tract in adult also has specific molecular profiles for regeneration. For example, treatment of Sema3A-inhibitor promotes regeneration of raphespinal tract but not CST (CitationKaneko et al 2006). It was shown that raphespinal axons express neuropilin-1, the receptor to mediate repulsive effect of Sema3A. Netrin-1 is expressed in oligodendrocytes, cells of central canal, and the meninges in adult SCI, and inhibits axonal growth through the receptor, Unc5 (CitationLöw et al 2008). Implantation of Netrin-1 expressing fibroblasts inhibits the regeneration of rubrospinal fibers which express Unc5A, but not CGRP-positive nociceptive axons which do not express Unc5. Thus, when the effects of the treatment are examined, we should consider which axons express receptors for guidance molecules, which axons regenerate after treatments, and how the regeneration of specific axons contribute to behavioral appearance.
Other targets are the downstream signaling molecules of inhibitory cues. Recent reports indicate that the small GTP-binding protein Rho and its effectors, ie, ROCK, are the key molecules that mediate inhibitory signals for axonal growth (for review see CitationMueller et al 2005; CitationKubo et al 2007). Importantly, Rho is a common downstream molecule of many repulsive cues, including RGM (CitationHata et al 2006), myelin-associated proteins through p75 (CitationYamashita and Tohyama 2003), CSPGs (CitationMonnier et al 2003), and members of the Semaphorin and Ephrin families (CitationWahl et al 2000; CitationSwiercz et al 2002). Thus, inhibiting the common pathway that mediates repulsion is one of the promising targets for therapy. Indeed, treatment with ROCK inhibitors, promotes axonal regeneration after SCI (CitationDergham et al 2002; CitationFournier et al 2003; CitationTanaka et al 2004). Understanding the detailed molecular mechanism of the inhibitory signals will reveal new targets for therapy.
Many discoveries regarding the role of various molecules and their effects in the case of SCI have emerged. This indicates that multiple inhibitory factors suppress regeneration. In fact, regeneration can be achieved but not to a great extent in each report. The approaches therefore shift to the use of a combination of several therapeutic approaches. For example, treatment with NT3 and an antibody of myelin-associated inhibitory proteins (IN-1) (CitationSchnell et al 1994), transplantation of fetal spinal cord and neurotrophic factors (CitationBregman et al 1997), chondroitinase ABC and cellular transplantation (CitationFouad et al 2005) etc have succeeded in promoting regeneration to a much greater extent than individual therapeutic approaches. Overall, combinational strategies that promote the completion of the repair process including inflammation and scar formation rapidly and in a compact manner, excluding the inhibitory factors for regeneration, bridging the lesion site, and facilitating the regeneration and sprouting of axons, should be considered for designing new therapeutic approaches. As the number of possible combinations that should be tested is already very large, greater efforts will be required to establish appropriate therapeutic methods. The scientific community working on SCI therefore needs to be increased and collaborate with each other. Furthermore, developmental neuroscientists can rightly contribute to this community.
In conclusion, an important aspect gathered from a large number of studies is that behavioral recovery after the injury by various treatments and methods is well correlated with the histological changes that occur in axons, ie axonal regeneration and sprouting. This implies that axonal regeneration and sprouting are crucial for behavioral recovery. Thus, promoting axonal elongation and sprouting is now one of the most important strategies to be employed for developing a new therapeutic method. In this case, basic research on brain development will reveal important clues.
References
- AokiMFujitoYSatomiH1986The possible role of collateral sprouting in the functional restitution of corticospinal connections after spinal hemisectionNeurosci Res3617273774241
- AshwellK1991The distribution of microglia and cell death in the fetal rat forebrainBrain Res Dev Brain Res58112
- AugsburgerASchuchardtAHoskinsS1999BMPs as mediators of roof plate repulsion of commissural neuronsNeuron241274110677032
- BagriAMarínOPlumpAS2002Slit proteins prevent midline crossing and determine the dorsoventral position of major axonal pathways in the mammalian forebrainNeuron332334811804571
- BährMPrzyrembelCBastmeyerM1995Astrocytes from adult rat optic nerves are nonpermissive for regenerating retinal ganglion cell axonsExp Neurol131211207895822
- BareyreFMKerschensteinerMRaineteauO2004The injured spinal cord spontaneously forms a new intraspinal circuit in adult ratsNat Neurosci72697714966523
- BarrettCPDonatiEJGuthL1984Differences between adult and neonatal rats in their astroglial response to spinal injuryExp Neurol84374856370713
- BartschUBandtlowCESchnellL1995Lack of evidence that myelin-associated glycoprotein is a major inhibitor of axonal regeneration in the CNSNeuron151375818845160
- BatesCAStelznerDJ1993Extension and regeneration of corticospinal axons after early spinal injury and the maintenance of corticospinal topographyExp Neurol123106178405271
- BentleyDCaudyM1983Pioneer axons lose directed growth after selective killing of guidepost cellsNature3046256866090
- BernsteinDRStelznerDJ1983Plasticity of the corticospinal tract following midthoracic spinal injury in the postnatal ratJ Comp Neurol2213824006662981
- BouhyDMalgrangeBMultonS2006Delayed GM-CSF treatment stimulates axonal regeneration and functional recovery in paraplegic rats via an increased BDNF expression by endogenous macrophagesFASEB J2012394116636109
- BradburyEJMoonLDPopatRJ2002Chondroitinase ABC promotes functional recovery after spinal cord injuryNature4166364011948352
- BregmanBSKunkel-BagdenEMcAteeM1989Extension of the critical period for developmental plasticity of the corticospinal pathwayJ Comp Neurol282355702715387
- BregmanBSKunkel-BagdenEReierPJ1993Recovery of function after spinal cord injury: mechanisms underlying transplant-mediated recovery of function differ after spinal cord injury in newborn and adult ratsExp Neurol1233168405277
- BregmanBSKunkel-BagdenESchnellL1995Recovery from spinal cord injury mediated by antibodies to neurite growth inhibitorsNature3784985017477407
- BregmanBSMcAteeMDaiHN1997Neurotrophic factors increase axonal growth after spinal cord injury and transplantation in the adult ratExp Neurol148475949417827
- BundesenLQScheelTABregmanBS2003Ephrin-B2 and EphB2 regulation of astrocyte-meningeal fibroblast interactions in response to spinal cord lesions in adult ratsJ Neurosci23778980012944508
- BussASchwabME2003Sequential loss of myelin proteins during Wallerian degeneration in the rat spinal cordGlia424243212730963
- ButlerSJDoddJ2003A role for BMP heterodimers in roof plate-mediated repulsion of commissural axonsNeuron3838940112741987
- CabelliRJHohnAShatzCJ1995Inhibition of ocular dominance column formation by infusion of NT-4/5 or BDNFScience267166267886458
- CabelliRJSheltonDLSegalRA1997Blockade of endogenous ligands of trkB inhibits formation of ocular dominance columnsNeuron1963769247264
- CaffertyWBStrittmatterSM2006The Nogo-Nogo receptor pathway limits a spectrum of adult CNS axonal growthJ Neurosci26122425017122049
- CaffertyWBYangSHDuffyPJ2007Functional axonal regeneration through astrocytic scar genetically modified to digest chondroitin sulfate proteoglycansJ Neurosci2721768517329414
- ChamakBMorandiVMallatM1994Brain macrophages stimulate neurite growth and regeneration by secreting thrombospondinJ Neurosci Res38221338078107
- ChanWYKohsakaSRezaieP2007The origin and cell lineage of microglia: new conceptsBrain Res Rev533445417188751
- CharronFTessier-LavigneM2005Novel brain wiring functions for classical morphogens: a role as graded positional cues in axon guidanceDevelopment13222516215857918
- ChenMSHuberABvan der HaarME2000Nogo-A is a myelin-associated neurite outgrowth inhibitor and an antigen for monoclonal antibody IN-1Nature403434910667796
- DavidSAguayoAJ1981Axonal elongation into peripheral nervous system “bridges” after central nervous system injury in adult ratsScience21493136171034
- DaviesJEHuangCProschelC2006Astrocytes derived from glial-restricted precursors promote spinal cord repairJ Biol5716643674
- De WinterFOudegaMLankhorstAJ2002Injury-induced class 3 semaphorin expression in the rat spinal cordExp Neurol175617512009760
- DerghamPEllezamBEssagianC2002Rho signaling pathway targeted to promote spinal cord repairJ Neurosci226570712151536
- DeumensRKoopmansGCJoostenEA2005Regeneration of descending axon tracts after spinal cord injuryProg Neurobiol77578916271433
- DimouLSchnellLMontaniL2006Nogo-A-deficient mice reveal strain-dependent differences in axonal regenerationJ Neurosci26559160316723516
- DomeniconiMCaoZSpencerT2002Myelin-associated glycoprotein interacts with the Nogo66 receptor to inhibit neurite outgrowthNeuron352839012160746
- DoughertyKDDreyfusCFBlackIB2000Brain-derived neurotrophic factor in astrocytes, oligodendrocytes, and microglia/macrophages after spinal cord injuryNeurobiol Dis75748511114257
- DowKEEthellDWSteevesJD1994Molecular correlates of spinal cord repair in the embryonic chick: heparan sulfate and chondroitin sulfate proteoglycansExp Neurol12823388076667
- ElkabesSDiCicco-BloomEMBlackIB1996Brain microglia/macrophages express neurotrophins that selectively regulate microglial proliferation and functionJ Neurosci162508218786427
- Engesser-CesarCIchiyamaRMNefasAL2007Wheel running following spinal cord injury improves locomotor recovery and stimulates serotonergic fiber growthEur J Neurosci251931917439482
- FaulknerJRHerrmannJEWooMJ2004Reactive astrocytes protect tissue and preserve function after spinal cord injuryJ Neurosci2421435514999065
- FestoffBWAmeenuddinSArnoldPM2006Minocycline neuroprotects, reduces microgliosis, and inhibits caspase protease expression early after spinal cord injuryJ Neurochem9713142616638021
- FingerJHBronsonRTHarrisB2002The netrin 1 receptors Unc5h3 and Dcc are necessary at multiple choice points for the guidance of corticospinal tract axonsJ Neurosci22103465612451134
- FirkinsSSBatesCAStelznerDJ1993Corticospinal tract plasticity and astroglial reactivity after cervical spinal injury in the postnatal ratExp Neurol1201157682966
- FouadKPedersenVSchwabME2001Cervical sprouting of corticospinal fibers after thoracic spinal cord injury accompanies shifts in evoked motor responsesCurr Biol1117667011719218
- FouadKSchnellLBungeMB2005Combining Schwann cell bridges and olfactory-ensheathing glia grafts with chondroitinase promotes locomotor recovery after complete transection of the spinal cordJ Neurosci2511697815689553
- FournierAEGrandPreTStrittmatterSM2001Identification of a receptor mediating Nogo-66 inhibition of axonal regenerationNature409341611201742
- FournierAETakizawaBTStrittmatterSM2003Rho kinase inhibition enhances axonal regeneration in the injured CNSJ Neurosci2314162312598630
- GaillardAPrestozLDumartinB2007Reestablishment of damaged adult motor pathways by grafted embryonic cortical neuronsNat Neurosci101294917828256
- GeorgeRGriffinJW1994Delayed macrophage responses and myelin clearance during Wallerian degeneration in the central nervous system: the dorsal radiculotomy modelExp Neurol129225367957737
- GianinoSSteinSALiH1999Postnatal growth of corticospinal axons in the spinal cord of developing miceBrain Res Dev Brain Res112189204
- GeorgeRGriffinJW1994Delayed macrophage responses and myelin clearance during Wallerian degeneration in the central nervous system: the dorsal radiculotomy modelExp Neurol129225367957737
- GoldshmitYGaleaMPWiseG2004Axonal regeneration and lack of astrocytic gliosis in EphA4-deficient miceJ Neurosci24100647315537875
- GrandPréTNakamuraFVartanianT2000Identification of the Nogo inhibitor of axon regeneration as a Reticulon proteinNature4034394410667797
- GrillRMuraiKBleschA1997Cellular delivery of neurotrophin-3 promotes corticospinal axonal growth and partial functional recovery after spinal cord injuryJ Neurosci175560729204937
- HaggTBakerKAEmsleyJG2005Prolonged local neurotrophin-3 infusion reduces ipsilateral collateral sprouting of spared corticospinal axons in adult ratsNeuroscience1308758715652986
- HarelNYStrittmatterSM2006Can regenerating axons recapitulate developmental guidance during recovery from spinal cord injury?Nat Rev Neurosci76031616858389
- HataKFujitaniMYasudaY2006RGMa inhibition promotes axonal growth and recovery after spinal cord injuryJ Cell Biol173475816585268
- HenschTKCritical period plasticity in local cortical circuits2005Nat Rev Neurosci687788816261181
- HiebertGWKhodarahmiKMcGrawJ2002Brain-derived neurotrophic factor applied to the motor cortex promotes sprouting of corticospinal fibers but not regeneration into a peripheral nerve transplantJ Neurosci Res69160812111797
- HsuJYSteinSAXuXM2006Development of the corticospinal tract in the mouse spinal cord: a quantitative ultrastructural analysisBrain Res1084162716616050
- HuberABKolodkinALGintyDD2003Signaling at the growth cone: ligand-receptor complexes and the control of axon growth and guidanceAnnu Rev Neurosci265096312677003
- HuberABWeinmannOBrösamleC2002Patterns of Nogo mRNA and protein expression in the developing and adult rat and after CNS lesionsJ Neurosci2235536711978832
- HutchinsonKJGómez-PinillaFCroweMJ2004Three exercise paradigms differentially improve sensory recovery after spinal cord contusion in ratsBrain12714031415069022
- IwashitaYKawaguchiSMurataM1994Restoration of function by replacement of spinal cord segments in the ratNature367167708114911
- JacobJBriscoeJ2003Gli proteins and the control of spinal-cord patterningEMBO Rep4761512897799
- JiBLiMWuWT2006LINGO-1 antagonist promotes functional recovery and axonal sprouting after spinal cord injuryMol Cell Neurosci333112017011208
- JonesLLMargolisRUTuszynskiMH2003The chondroitin sulfate proteoglycans neurocan, brevican, phosphacan, and versican are differentially regulated following spinal cord injuryExp Neurol18239941112895450
- JoostenEABärDP1999Axon guidance of outgrowing corticospinal fibres in the ratJ Anat194153210227663
- JoostenEABärDPGispenWH1995Directional regrowth of lesioned corticospinal tract axons in adult rat spinal cordNeuroscience69619268552254
- JoostenEAGispenWHBärPR1994Tropism and corticospinal target selection in the ratNeuroscience5933418190270
- JoostenEAGribnauAA1989Astrocytes and guidance of outgrowing corticospinal tract axons in the rat. An immunocytochemical study using anti-vimentin and anti-glial fibrillary acidic proteinNeuroscience31439522797445
- JoostenEAGribnauAADederenPJ1989Postnatal development of the corticospinal tract in the rat. An ultrastructural anterograde HRP studyAnat Embryol179449562729608
- KanekoSIwanamiANakamuraM2006A selective Sema3A inhibitor enhances regenerative responses and functional recovery of the injured spinal cordNat Med121380917099709
- Katoh-SembaRMatsudaMKatoK1995Chondroitin sulphate proteoglycans in the rat brain: candidates for axon barriers of sensory neurons and the possible modification by laminin of their actionsEur J Neurosci7613217620612
- KimJELiSGrandPréT2003Axon regeneration in young adult mice lacking Nogo-A/BNeuron381879912718854
- KimJELiuBPParkJH2004Nogo-66 receptor prevents raphespinal and rubrospinal axon regeneration and limits functional recovery from spinal cord injuryNeuron444395115504325
- KottisVThibaultPMikolD2002Oligodendrocyte-myelin glycoprotein (OMgp) is an inhibitor of neurite outgrowthJ Neurochem821566912354307
- KuboTHataKYamaguchiA2007Rho-ROCK inhibitors as emerging strategies to promote nerve regenerationCurr Pharm Des132493917692017
- KullanderKCrollSDZimmerM2001Ephrin-B3 is the midline barrier that prevents corticospinal tract axons from recrossing, allowing for unilateral motor controlGenes Dev158778811297511
- KyotoAHataKYamashitaT2007Synapse formation of the corticospinal axons is enhanced by RGMa inhibition after spinal cord injuryBrain Res1186748617996222
- Lalancette-HébertMGowingGSimardA2007Selective ablation of proliferating microglial cells exacerbates ischemic injury in the brainJ Neurosci27259660517344397
- LankhorstAJter LaakMPvan LaarTJ2001Effects of enriched housing on functional recovery after spinal cord contusive injury in the adult ratJ Neurotrauma182031511229712
- LeeJKKimJESivulaM2004Nogo receptor antagonism promotes stroke recovery by enhancing axonal plasticityJ Neurosci2462091715240813
- LiSStrittmatterSM2003Delayed systemic Nogo-66 receptor antagonist promotes recovery from spinal cord injuryJ Neurosci2342192712764110
- LiSLiuBPBudelS2004Blockade of Nogo-66, myelin-associated glycoprotein, and oligodendrocyte myelin glycoprotein by soluble Nogo-66 receptor promotes axonal sprouting and recovery after spinal injuryJ Neurosci24105112015548666
- LiSKimJEBudelS2005Transgenic inhibition of Nogo-66 receptor function allows axonal sprouting and improved locomotion after spinal injuryMol Cell Neurosci29263915866044
- LiWWYewDTChuahMI1994Axonal sprouting in the hemisected adult rat spinal cordNeuroscience6113397969888
- LiYFieldPMRaismanG1997Repair of adult rat corticospinal tract by transplants of olfactory ensheathing cellsScience277200029302296
- LiuBPFournierAGrandPréT2002Myelin-associated glycoprotein as a functional ligand for the Nogo-66 receptorScience2971190312089450
- LiuYShiJLuCC2005Ryk-mediated Wnt repulsion regulates posterior-directed growth of corticospinal tractNat Neurosci81151916116452
- López-BenditoGCautinatASánchezJA2006Tangential neuronal migration controls axon guidance: a role for neuregulin-1 in thalamocortical axon navigationCell1251274216615895
- LöwKCulbertsonMBradkeF2008Netrin-1 is a novel myelin-associated inhibitor to axon growthJ Neurosci28109910818234888
- MatsuuraITaniguchiJHataK2008BMP inhibition enhances axonal growth and functional recovery after spinal cord injuryJ Neurochem34[Epub ahead of print].
- McGeeAWYangYFischerQS2005Experience-driven plasticity of visual cortex limited by myelin and Nogo receptorScience3092222616195464
- McKeonRJSchreiberRCRudgeJS1991Reduction of neurite outgrowth in a model of glial scarring following CNS injury is correlated with the expression of inhibitory molecules on reactive astrocytesJ Neurosci1133984111719160
- McKerracherLDavidSJacksonDL1994Identification of myelin-associated glycoprotein as a major myelin-derived inhibitor of neurite growthNeuron13805117524558
- MiSLeeXShaoZ2004LINGO-1 is a component of the Nogo-66 receptor/p75 signaling complexNat Neurosci7221814966521
- MonnierPPSierraAMacchiP2002RGM is a repulsive guidance molecule for retinal axonsNature419392512353034
- MonnierPPSierraASchwabJM2003The Rho/ROCK pathway mediates neurite growth-inhibitory activity associated with the chondroitin sulfate proteoglycans of the CNS glial scarMol Cell Neurosci223193012691734
- MuellerBKMackHTeuschN2005Rho kinase, a promising drug target for neurological disordersNat Rev Drug Discov43879815864268
- MukhopadhyayGDohertyPWalshFS1994A novel role for myelin-associated glycoprotein as an inhibitor of axonal regenerationNeuron13757677522484
- NakajimaKHamanoueMShimojoM1989Characterization of microglia isolated from a primary culture of embryonic rat brain by a simplified methodBiomed Res1041123
- O'LearyDDTerashimaT1988Cortical axons branch to multiple subcortical targets by interstitial axon budding: implications for target recognition and “waiting periods”Neuron1901103272157
- OkadaSNakamuraMKatohH2006Conditional ablation of Stat3 or Socs3 discloses a dual role for reactive astrocytes after spinal cord injuryNat Med128293416783372
- ParkJBYiuGKanekoS2005A TNF receptor family member, TROY, is a coreceptor with Nogo receptor in mediating the inhibitory activity of myelin inhibitorsNeuron453455115694321
- PasterkampRJPeschonJJSpriggsMK2003Semaphorin 7A promotes axon outgrowth through integrins and MAPKsNature42439840512879062
- PetitASellersDLLieblDJ2007Adult spinal cord progenitor cells are repelled by netrin-1 in the embryonic and injured adult spinal cordProc Natl Acad Sci USA104178374217978191
- PizzorussoTMediniPBerardiN2002Reactivation of ocular dominance plasticity in the adult visual cortexScience2981248125112424383
- PolleuxFGigerRJGintyDD1998Patterning of cortical efferent projections by semaphorin-neuropilin interactionsScience282190469836643
- PolleuxFMorrowTGhoshA2000Semaphorin 3A is a chemoattractant for cortical apical dendritesNature4045677310766232
- PopovichPGGuanZWeiP1999Depletion of hematogenous macrophages promotes partial hindlimb recovery and neuroanatomical repair after experimental spinal cord injuryExp Neurol1583516510415142
- PrewittCMNiesmanIRKaneCJ1997Activated macrophage/microglial cells can promote the regeneration of sensory axons into the injured spinal cordExp Neurol148433439417823
- PrinjhaRMooreSEVinsonM2000Inhibitor of neurite outgrowth in humansNature403383410667780
- Ramón-CuetoAPlantGWAvilaJ1998Long-distance axonal regeneration in the transected adult rat spinal cord is promoted by olfactory ensheathing glia transplantsJ Neurosci183803159570810
- RabchevskyAGStreitWJ1997Grafting of cultured microglial cells into the lesioned spinal cord of adult rats enhances neurite outgrowthJ Neurosci Res4734488981236
- RapalinoOLazarov-SpieglerOAgranovE1998Implantation of stimulated homologous macrophages results in partial recovery of paraplegic ratsNat Med4814219662373
- ReierPJ1979Penetration of grafted astrocytic scars by regenerating optic nerve axons in Xenopus tadpolesBrain Res164618427571
- RichardsonPMMcGuinnessUMAguayoAJ1980Axons from CNS neurons regenerate into PNS graftsNature28426457360259
- RiddleDRLoDCKatzLC1995NT-4-mediated rescue of lateral geniculate neurons from effects of monocular deprivationNature378189917477322
- RudgeJSSilverJ1990Inhibition of neurite outgrowth on astroglial scars in vitroJ Neurosci1035946032230948
- SatoMLopez-MascaraqueLHeffnerCD1994Action of a diffusible target-derived chemoattractant on cortical axon branch induction and directed growthNeuron137918037946329
- SatoYHirataTOgawaM1998Requirement for early-generated neurons recognized by monoclonal antibody lot1 in the formation of lateral olfactory tractJ Neurosci187800109742149
- SavioTSchwabME1990Lesioned corticospinal tract axons regenerate in myelin-free rat spinal cordProc Natl Acad Sci USA87413032349222
- SchnellLSchneiderRKolbeckR1994Neurotrophin-3 enhances sprouting of corticospinal tract during development and after adult spinal cord lesionNature36717038114912
- SchnellLSchwabME1990Axonal regeneration in the rat spinal cord produced by an antibody against myelin-associated neurite growth inhibitorsNature343269722300171
- SchwabMEBartholdiD1996Degeneration and regeneration of axons in the lesioned spinal cordPhysiol Rev76319708618960
- SchwabJMConradSMonnierPP2005Spinal cord injury-induced lesional expression of the repulsive guidance molecule (RGM)Eur J Neurosci2115697615845084
- SchwabMESchnellL1989Region-specific appearance of myelin constituents in the developing rat spinal cordJ Neurocytol1816192471819
- SchwartzMButovskyOBrückW2006Microglial phenotype: is the commitment reversible?Trends Neurosci29687416406093
- ShaoZBrowningJLLeeX2005TAJ/TROY, an orphan TNF receptor family member, binds Nogo-66 receptor 1 and regulates axonal regenerationNeuron45353915694322
- ShuTRichardsLJ2001Cortical axon guidance by the glial wedge during the development of the corpus callosumJ Neurosci2127495811306627
- SilverJOgawaMY1983Postnatally induced formation of the corpus callosum in acallosal mice on glia-coated cellulose bridgesScience220106796844928
- SimonenMPedersenVWeinmannO2003Systemic deletion of the myelin-associated outgrowth inhibitor Nogo-A improves regenerative and plastic responses after spinal cord injuryNeuron382011112718855
- SingerMNordlanderRHEgarM1979Axonal guidance during embryogenesis and regeneration in the spinal cord of the newt: the blueprint hypothesis of neuronal pathway patterningJ Comp Neurol185121429610
- SnowDMSteindlerDASilverJ1990Molecular and cellular characterization of the glial roof plate of the spinal cord and optic tectum: a possible role for a proteoglycan in the development of an axon barrierDev Biol138359761690673
- SongXYZhongJHWangX2004Suppression of p75NTR does not promote regeneration of injured spinal cord in miceJ Neurosci24542614724254
- StahlBMüllerBvon BoxbergY1990Biochemical characterization of a putative axonal guidance molecule of the chick visual systemNeuron5735432171592
- StirlingDPKhodarahmiKLiuJ2004Minocycline treatment reduces delayed oligodendrocyte death, attenuates axonal dieback, and improves functional outcome after spinal cord injuryJ Neurosci2421829014999069
- StreitWJ2001Microglia and macrophages in the developing CNSNeurotoxicology226192411770883
- SwierczJMKunerRBehrensJ2002Plexin-B1 directly interacts with PDZ-RhoGEF/LARG to regulate RhoA and growth cone morphologyNeuron35516312123608
- TakamiTOudegaMBatesML2002Schwann cell but not olfactory ensheathing glia transplants improve hindlimb locomotor performance in the moderately contused adult rat thoracic spinal cordJ Neurosci2266708112151546
- TanakaHYamashitaTYachiK2004Cytoplasmic p21(Cip1/WAF1) enhances axonal regeneration and functional recovery after spinal cord injury in ratsNeuroscience1271556415219678
- TangXDaviesJEDaviesSJ2003Changes in distribution, cell associations, and protein expression levels of NG2, neurocan, phosphacan, brevican, versican V2, and tenascin-C during acute to chronic maturation of spinal cord scar tissueJ Neurosci Res714274412526031
- TempleS2001The development of neural stem cellsNature4141121711689956
- UenoMKatayamaKYamauchiH2006Repair process of fetal brain after 5-azacytidine-induced damageEur J Neurosci2427586817156202
- VallièresNBerardJLDavidS2006Systemic injections of lipopolysaccharide accelerates myelin phagocytosis during Wallerian degeneration in the injured mouse spinal cordGlia531031316206158
- Van MeeterenNLEggersRLankhorstAJ2003Locomotor recovery after spinal cord contusion injury in rats is improved by spontaneous exerciseJ Neurotrauma2010293714588119
- VavrekRGirgisJTetzlaffW2006BDNF promotes connections of corticospinal neurons onto spared descending interneurons in spinal cord injured ratsBrain12915344516632552
- WahlSBarthHCiossekT2000Ephrin-A5 induces collapse of growth cones by activating Rho and Rho kinaseJ Cell Biol1492637010769020
- WangKCKimJASivasankaranR2002P75 interacts with the Nogo receptor as a co-receptor for Nogo, MAG and OMgpNature42074812422217
- WangKCKoprivicaVKimJA2002Oligodendrocyte-myelin glycoprotein is a Nogo receptor ligand that inhibits neurite outgrowthNature417941412068310
- WehrleRCamandEChedotalA2005Expression of netrin-1, slit-1 and slit-3 but not of slit-2 after cerebellar and spinal cord lesionsEur J Neurosci2221344416262652
- WeidnerNNerASalimiN2001Spontaneous corticospinal axonal plasticity and functional recovery after adult central nervous system injuryProc Natl Acad Sci USA9835131811248109
- WongSTHenleyJRKanningKC2002A p75(NTR) and Nogo receptor complex mediates repulsive signaling by myelin-associated glycoproteinNat Neurosci51302812426574
- YamashitaTHiguchiHTohyamaM2002The p75 receptor transduces the signal from myelin-associated glycoprotein to RhoJ Cell Biol1575657012011108
- YamashitaTTohyamaM2003The p75 receptor acts as a displacement factor that releases Rho from Rho-GDINat Neurosci6461712692556
- YamashitaTFujitaniMYamagishiS2005Multiple signals regulate axon regeneration through the Nogo receptor complexMol Neurobiol321051116215275
- YamashitaTMuellerBKHataK2007Neogenin and repulsive guidance molecule signaling in the central nervous systemCurr Opin Neurobiol17293417169551
- YokoyamaNRomeroMICowanCA2001Forward signaling mediated by ephrin-B3 prevents contralateral corticospinal axons from recrossing the spinal cord midlineNeuron29859711182083
- YongVWWellsJGiulianiF2004The promise of minocycline in neurologyLancet Neurol37445115556807
- YuneTYLeeJYJungGY2007Minocycline alleviates death of oligodendrocytes by inhibiting pro-nerve growth factor production in microglia after spinal cord injuryJ Neurosci2777516117634369
- ZhengBHoCLiS2003Lack of enhanced spinal regeneration in Nogo-deficient miceNeuron382132412718856
- ZhengBAtwalJHoC2005Genetic deletion of the Nogo receptor does not reduce neurite inhibition in vitro or promote corticospinal tract regeneration in vivoProc Natl Acad Sci USA10212051015647357
- ZhouLBaumgartnerBJHill-FelbergSJ2003Neurotrophin-3 expressed in situ induces axonal plasticity in the adult injured spinal cordJ Neurosci2314243112598631