Abstract
Chronic lipopolysaccharide (LPS) exposure may contribute to the pathogenesis of a number of lung diseases including COPD and emphysema. We sought to develop a large- animal model of emphysema using repeated LPS administration into sheep lung segments. An experimental protocol was designed to facilitate comparisons with elastase-treated and control segments within the same lung of individual sheep. Histopathologic evaluation of segments treated with LPS demonstrated low-grade inflammation characterized by an increase in the number of intra-alveolar macrophages and lymphocytes. Treated segments demonstrated a significant reduction in airspace surface area (ASA), an increase in percent disrupted alveolar attachments and the distance between normal alveolar attachments, and a reduction in the number of normal alveolar attachments surrounding nonrespiratory bronchioles. Coefficient of variation of individual ASA measurements in elastase-treated segments was indicative of a heterogeneous parenchymal response, in contrast to that associated with chronic LPS treatment. Our results demonstrate that chronic LPS treatment of individual lung segments in sheep induces microscopic emphysema qualitatively and quantitatively consistent with both accepted pathologic definitions of this condition and with that produced by airway instillation of elastolytic enzymes. Development of this phenotype is associated with evidence of downregulated activation of transforming growth factor beta.
Introduction
Emphysema is mostly associated with smoking (CitationJanoff 1983) and is also seen in individuals with a severe genetic deficiency in serum levels of a-1 antiprotease (CitationLaurell and Ericksson 1963). The pathology, featuring permanent enlargement of airspaces distal to the terminal bronchiole, is linked to the destruction of alveolar walls (CitationSnider et al 1986) in a process characterized by degradation and fraying of the elastic fibre network (CitationFukuda et al 1989; CitationWright 1961). These features together with the initial observation of CitationGross et al (1964) that intratracheal administration of papain to rats caused similar pathology, led to the concept that emphysema might be mediated at least in part through proteolytic damage.
This hypothesis is largely accepted and serine proteases and metalloproteases from neutrophils and macrophages respectively are thought to be the major source of elastolytic activity (CitationShapiro 1994; CitationFinlay et al 1997; CitationPardo and Selman 1999) in emphysema. Cigarette smoking leads to the recruitment of these inflammatory cells and also damages the antiprotease screen as a consequence of free radical production.
Emphysema has been extensively modeled in animals (CitationSnider et al 1986), most models relying upon the direct intratracheal instillation or inhalation of proteolytic enzymes. The early tissue damage arising as a consequence is characterized by edema, hemorrhage, and a modest infiltration of neutrophils and macrophages (CitationSnider et al 1986). Thereafter there is evidence of tissue repair involving synthesis of new elastin (CitationMercer and Crapo 1992).
A common and justified criticism of such models is that the acute nature of disease induction fails to represent the slow progression of the disease in man. As CitationSnider et al (1986) point out “The artificiality of putting a large quantity of an elastolytic enzyme into the lungs of an animal severely limits the usefulness of this model in answering questions relating to mechanisms of human emphysema.”
This concern has led to a number of groups developing small-animal models in which inflammatory cell recruitment with presumed release of endogenous proteinase is induced by repeated instillation of lipopolysaccharide (LPS) and leads to the development of emphysematous lesions (CitationStolk et al 1992; CitationRudolphus et al 1993; CitationLawson et al 1995; CitationVernooy et al 2002).
It was our contention that such a strategy could equally be applied within the context of a large-animal model system. The major advantage of using such a system, namely the ability to differentially and repeatedly treat different areas within the same lung, allows for the direct and meaningful comparison of different treatment protocols. Such an approach has obvious advantages with respect to being able to treat each animal as its own control, thereby counteracting inter-animal variation and reducing the group sizes required to adequately test hypotheses. Further, treatments administered at the segmental level are potentially less likely to clinically affect the animal, hold clear relevance for future evaluation of therapeutic strategies delivered directly to the lung, and hold potential for translation into therapeutic protocols directed at human subjects. Our experience (CitationCollie et al 1999, Citation2001), together with that of others (CitationBegin et al 1981), that sheep tolerate repeated anesthesia and bronchoscopic intervention particularly well, further complemented our approach.
We determined to assess the response of the sheep lung to repeated endobronchial administration of LPS. Our hypothesis was that such a strategy would engender a state of chronic inflammation and that this inflammation would persistently imbalance the relative influence of proteases and antiproteases in the lung, leading to the development of pulmonary emphysema. We sought to compare this model with the established principle of producing emphysema through local lung administration of elastase. Further, recognizing the potential role for pulmonary remodeling involving collagen and elastin synthesis in emphysema (CitationVlahovic et al 1999), we sought to define the pattern of regulation of the pro-fibrotic cytokine, transforming growth factor beta (TGF-β) in this model.
Our results demonstrate that chronic LPS treatment of individual lung segments in sheep induces microscopic emphysema qualitatively and quantitatively consistent with accepted pathologic definitions of this condition, and that the development of this phenotype is associated with evidence of mild mononuclear alveolitis and downregulated activation of TGF-β. Our results also demonstrate changes common to all lung segments, regardless of treatment schedule. These changes consist of a progressive low-grade neutrophilic inflammation coupled with evidence of a reduction in the elastase inhibitory capacity present in the bronchoalveolar space.
Materials and Methods
Animals
Eight adult crossbred sheep (Suffolk cross; 5 F and 3 MN) (bodyweight: 48–57 kg) were used in this study. Animals were judged to be free from significant pulmonary disease on the basis of clinical examination by a veterinary surgeon, a subjective opinion subsequently confirmed at necropsy. All experimental procedures were subject to ethical review and were performed under license, as required by the United Kingdom’s Animals (Scientific Procedures) Act 1986.
Materials
Four hundred units of purified porcine elastase (PPE) were prepared in 20 mL saline (200 units/mL) for each segmental instillation. LPS was derived from Mannheimia haemolytica by phenol extraction. Three millilitres of a sterile, 150 μg/mL suspension of M. haemolytica LPS in saline (SAL) were prepared for each segmental instillation. A volume of 3 mL SAL was prepared for each segmental instillation.
Experimental design
Four spatially disparate lung segments were selected on bronchoscopic examination and their position carefully mapped. The segments were randomly assigned to one of four treatment protocols. A sample of bronchoalveolar lavage fluid (BALF) was collected from each segment for cytological examination. Treatment protocols consisted of an initial instillation of either SAL (segment A), LPS (segment B), or PPE (segments C and D) followed, at 4- to 7-day intervals by 7 instillations of either SAL (segments A and C) or LPS (segments B and D). Additional BALF was collected after 3 instillations (day 14) and 1 week after the 8th instillation (day 39). Sheep were killed on day 39 and submitted for post-mortem examination and collection of tissues for morphometric evaluation. The treatment protocol is outlined in .
Table 1 Experimental protocol. Four segments (A–D) were randomly assigned to treatment protocols. Bronchoalveolar lavage (BAL) was employed to collect fluid from segments A–D for cytological examination prior to (day −7), during (day 14), and at the completion (day 39) of the study. Treatment protocols consisted of an initial instillation of either saline (SAL) (segment A), lipopolysaccharide (LPS) (segment B), or purified porcine elastase (PPE) (segments C and D) followed at 4- to 7-day intervals by 7 instillations of either SAL (segments A and C) or LPS (segments B and D). Animals were killed on day 39 and submitted for post-mortem examination (PME) and collection of tissues for morphometric assessment.
Anesthesia and ventilation
Food was withheld for 12 hours prior to anesthesia which was achieved by intravenous administration of a single bolus of thiopentone sodium (Intraval sodium; Merial Animal health Ltd, Harlow, Essex, UK) at a dose rate of 20 mg/kg bodyweight. Thereafter sheep were intubated and anesthesia maintained using gaseous halothane (2%–3%) in oxygen and nitrous oxide. The sheep were placed in sternal recumbency in a large plexiglass whole-body respirator (internal volume 388 L). The proximal end of the endotracheal tube was connected to the anesthetic circuit through a connector in the wall of the box. Pressure in the box was varied by appropriate connection to a large bellows pump (Cuirass; Cape Warwick, Warwick, UK) which induced a sinusoidal tidal respiratory pattern, the rate and magnitude of which could be controlled by adjustment of the pump itself. The magnitude of pressure fluctuations were adjusted to maintain a tidal volume of 10 mL/kg bodyweight. Respiratory rate was adjusted to maintain end-tidal CO2 measurements in the range 4.5%–5.5% (Oxicap Monitor Model 4700; Ohmeda, Louisville, CO, USA).
Bronchoalveolar lavage
A flexible fibre optic bronchoscope (5.3 mm OD) (Model FG-16X; Pentax UK Ltd, Slough, UK) was advanced and wedged in selected segmental bronchi. The subtended segments were lavaged by installation and withdrawal of 2 20-mL aliquots of normal saline (0.9% NaCl solution). BALF was immediately placed on ice until subsequent analysis.
Sample handling
Lavage fluid from each lung lobe was separated into supernatant and cells by centrifugation at 400 g for 7 minutes and the resultant cell pellets were resuspended in phosphate buffered saline (PBS) before differential cytology was determined. Supernatants were recentrifuged at 1000 g at 4°C for 20 minutes. The samples were stored at −70oC until analysis of TGF-β1, elastase, and inhibitor levels.
Differential cytology
Cells were counted using a Neubauer hemocytometer and values expressed per millilitre BALF. Cytocentrifuge slides were prepared and stained using Leishmann’s stain for differential counts on 400 cells. Cells were classified as neutrophils, macrophages, eosinophils, lymphocytes, or mast cells according to standard morphological criteria. Cells not classified in the aforementioned groups were recorded as “other” cells.
Neutrophil elastase and neutrophil elastase inhibitor assays
BALF samples were concentrated 10× by freeze drying and re-dissolving in a 1/10 volume of sterile water. A stock concentration of 10 mg/mL neutrophil elastase substrate (N-methoxysuccinyl-Ala-Ala-Pro-Val p-Nitroanalide [Sigma] in DMSO [Sigma]) was prepared and stored at 4°C. A 50 μl volume of this stock was diluted to 0.1 mg/mL in 50 mM Tris; 0.5 M NaCl, 0.1% Triton X-100, pH 8.0. Standards of human sputum elastase (HSE, Elastin Products Company, Owensville MO, USA) ranging from 250 μg/50mL to 10 ng/50 μL were prepared in 50 mM sodium acetate, 0.5M NaCl, pH 4.5. To a 96-well microtitre plate, 50 ml of concentrated BALF or HSE standard solution was incubated with 50 μl of substrate. After equilibration for 1 minute the absorbance was measured at 405 nm in a Dynatech MRX plate reader at 37°C for each sample at 1-minute intervals for 15 minutes. The optimal elastase activity reading of the BALF was taken to be the time point at which the readings ceased to be linear. Absorbances were compared with control samples and the levels of elastase in the samples estimated.
Elastase inhibitor activity assay
HSE stock was diluted to 100 ng/10 μl in 50 mM sodium acetate, 0.5 M NaCl, pH 4.5. BAL was serially diluted down to 1/572 in 50 mM Tris, 0.5 M NaCl, 0.1% Triton X-100, pH 8.0. To each well of a 96-well microtitre plate, 10 ml of BAL dilution was incubated with 30 μl 50 mM 0.5 M NaCl, 0.1% Triton X-100, pH 8.0 and 10 ml HSE. Following incubation at 37°C, 5% CO2 for 15 minutes, 50 μl of substrate was added and equilibrated for 1 minute. The absorbance was read at 405 nm at 1-minute intervals for 15 minutes. The level of inhibition (decrease in absorbance at 405 nm) of the HSE was directly proportional to the amount of elastase inhibitors present in the BAL.
Measurement of TGF-β in BALF
TGF-β levels in BALF supernatant were measured using minor modifications of the procedure described by CitationAbe et al (1994). Briefly, BALF supernatant was filtered through 2 layers of sterile muslin and pre-spun in Vectaspin micro anopore filters (Whatman International Ltd., Maidstone, Kent, UK) at 13 000 rpm for 5 minutes to remove cell debris. The supernatant was then concentrated 5 × by means of Vivaspin 0.5 mL concentrators (Vivascience Ltd, Gloucestershire, UK; 10 000 MW cut-off) used according to the manufacturer’s instructions.
Because TGF-β is secreted in a latent form acid activation is required to release active TGF-β from the latent complex. Relevant aliquots were acid-activated by the addition of 5M HCl. Thereafter the samples were neutralized and submitted for assay.
Mink lung epithelial cells (MLEC) stably transfected with an expression construct containing a truncated plasminogen activator inhibitor-1 (PAI-1) promoter fused to the firefly luciferase gene were used as the reporter system. TGF-β, through its induction of PAI-1 expression, causes dose-dependent increases in luciferase activity in this system (CitationAbe et al 1994). MLECs were maintained at 37°C in Dulbecco’s Modified Eagles Medium (DMEM), 10% fetal bovine serum containing penicillin–streptomycin, L-glutamine, and G418 (geneticin 200 mg/ml) (Life Technologies, Cambridge, UK). The sample assay involved removing and replacing cell medium with 100 μl BALF supernatant. Assay plates were incubated overnight. Thereafter the cells were washed and lysed and the lysate added to opaque-walled 96-well plates (Dynex Technologies, Middlesex, UK). Samples were read in a Reporter™ microplate luminometer (Turner Designs Inc., Sunnyvale, CA, USA) using a luciferase substrate for a single flash reaction. Samples were read against a standard curve prepared using appropriate dilutions of human recombinant TGF-β1 (R&D Systems Europe Ltd, Oxon, UK).
Tissue handling
Lung tissue was fixed by airway instillation of 4% paraformaldehyde (pH 7.0) at an inflation pressure of 2.5 to 3.0 kPa for 3 days. Following fixation, 3 tissue blocks were sampled from each lung segment and routinely processed and embedded in paraffin. Serial 4-μm sections were cut from the tissue blocks and stained with hematoxylin and eosin for morphometric analysis.
Tissue sections were blinded for the purposes of histopathologic and morphometric evaluation.
Morphometric analysis
Twelve sections were available from each animal for analysis (3 from each of 4 segments). Fields (0.07 mm2) within each section were randomly selected. If the examined fields satisfied defining criteria for parenchyma (absence of airways or vessels over 1 mm diameter) morphometric measurements were made. Airspace wall tissue was selected using an image analyzer (Quantimet Q500C, Leica, Cambridge, UK) and, after interactive editing to fill in small vessels and remove debris, total airspace wall length per unit area (ASA) was measured as mm per mm2. To ensure that a stable running mean was obtained, the relative standard error (RSE) of measurements from fields, ie, the size of the standard error relative to the mean value, was reduced to less than 3%. Heterogeneity of ASA measurements within segments was estimated by calculating the coefficent of variation for measurements (this is a unitless quantity indicating the variability around the mean in relation to the size of the mean).
The loss of alveolar attachments extending radially from the outer wall of nonrespiratory bronchioles less than 1 mm diameter was also quantified in a manner similar to that described by CitationSaetta et al (1985). Ten airways were selected from each segment and the number of complete and abnormal alveolar attachments were counted. Tangentially or partially cut bronchioles were excluded from analysis. The outer circumference of the bronchiole was interactively measured using the image analysis package and the results expressed as the average number of normal attachments, the distance between normal attachments (distance = external circumference/[no. attached]) and the percentage of abnormal attachments (% abnormal = [number of abnormal/(no. attached + number of abnormal) ×100).
All morphometric analysis was completed by the same operator (NMcL). Reproducibility of ASA measurements was assessed by repeated analysis of stored images from 6 parenchymal fields over 2 weeks. Similarly 15 bronchioles were analyzed on a repeated basis for loss of alveolar attachments. The coefficient of variation for repeated measurements by the same operator was 2% for ASA measurements, and 7%, 4%, and 7% for the number of alveolar attachments, the distance between attachments, and the percentage of abnormal attachments, respectively.
Data analysis
Within sheep, between-segment comparisons and within-segment, over-time comparisons were assessed using a Friedman two-way analysis of variance followed by Wilcoxon matched pairs signed rank tests where significant differences were indicated.
Results
Animals tolerated the procedure well. No cumulative effect of repeated anesthesia was apparent, nor was there any observed clinical evidence of respiratory dysfunction as a result of the treatment protocol.
Qualitative and quantitative changes in BALF cellularity as a consequence of the experimental protocol were apparent in all segments including the SAL:SAL segment. Changes were consistent between segments and comprised increased absolute numbers of neutrophils, and decreased numbers of mast cells and eosinophils over time (). The magnitude of change was also consistent between segments. A nonsignificant trend towards increased neutrophils (p = 0.07), and no significant change in eosinophil cell numbers (p = 0.26) in the PPE:LPS segments were the exceptions to the above. Segment-specific changes in absolute neutrophil cell numbers in BALF are depicted in . At no time did the median percentage of neutrophils rise above 3% in any one segment (data not shown).
Table 2 Absolute cell counts (cells/mL BALF × 104; median [range]) in bronchoalveolar lavage fluid (BALF) obtained prior to (day −7), during (day 14), and at the conclusion of (day 39) the treatment protocol. Dynamic changes over time within each segment, and differences between segments at specific time points for each variable, were assessed using a Friedman nonparametric analysis of variance. Where significance (p < 0.05) could be demonstrated, further analysis, using Wilcoxon signed rank procedures, compared results obtained on day 14 and day 39 with those obtained on day −7, and compared results from treated segments with those from SAL:SAL segments at each time point.
Figure 1 Boxplot depicting the change in absolute neutrophil counts (cells/mL BALF × 104; median [range]) over time with individual symbols shown. Changes over time were significant (p < 0.05) in all bar the PPE:LPS segment (p = 0.07).
![Figure 1 Boxplot depicting the change in absolute neutrophil counts (cells/mL BALF × 104; median [range]) over time with individual symbols shown. Changes over time were significant (p < 0.05) in all bar the PPE:LPS segment (p = 0.07).](/cms/asset/45633d6a-3cb5-4d97-b1b3-89b8c585feae/dcop_a_12189_f0001_b.jpg)
Compared with recoveries on day −7, significantly less BALF was recovered on day 39 in PPE:LPS segments, and on day 14 and day 39 in LPS:LPS segments.
Comparing between segments at specific time points highlighted a significant increase in the numbers of macrophages and lymphocytes in BALF from the treated segments at day 14 compared with SAL:SAL segments (p < 0.05). The number of macrophages in BALF from PPE:SAL segments was also significantly increased at day 39 compared with SAL:SAL segments (p < 0.05).
Histopathological evaluation of PPE:LPS and LPS:LPS segments revealed a low-grade alveolitis characterized by increased numbers of intra-alveolar macrophages and lymphocytes. Airways were largely unaffected. PPE:SAL and SAL:SAL segments were considered histologically normal but for incidental changes consistent with sheep managed under identical circumstances.
All 3 treatments caused a significant drop in ASA relative to SAL:SAL segments (p < 0.05; ). ASA from PPE:SAL- and PPE:LPS-treated segments was significantly lower than ASA from LPS:LPS-treated segments. ASA measurements were significantly more variable, as assessed using the coefficient of variation (CV), in segments treated with PPE compared with measurements obtained from SAL:SAL segments (p < 0.05; ). Although ASA measurements from PPE:SAL segments were significantly more variable than those from LPS:LPS segments (p < 0.05), comparisons between LPS:LPS and PPE:LPS segments for this measurement were nonsignificant (p = 0.093). The CV for ASA measurements was 6.7% (4.9–10.7) [median (range)], 7.5% (3.4–15.2), 12.4% (10.0–20.7), and 10.9% (7.3–17.4) for SAL:SAL, LPS:LPS, PPE:SAL, and PPE:LPS segments, respectively. There were significantly fewer normal alveolar attachments in the airways from treated segments relative to the SAL:SAL segment (). There was a significantly greater distance between normal alveolar attachments in the treated segments compared with the SAL:SAL segment (). The distance between alveolar attachments surrounding airways from PPE:LPS segments was significantly greater than from LPS:LPS segments. Airways from treated segments had a significantly greater percentage of disrupted alveolar attachments than airways from SAL:SAL segments (). Airways from PPE:LPS segments had a significantly greater percentage of disrupted alveolar attachments than airways from PPE:SAL segments.
Figure 2 Airspace surface area (ASA) measurement values (mm/mm2) in lung segments. Each dot represents the mean value calculated on the basis of measurements made on randomly selected fields in sections taken from blocks (n = 3) from each segment. Different symbols represent individual sheep. The extremes of the box represent the upper and lower quartiles. The median value of the data set is represented by a horizontal line within the box. Each “whisker” represents 25% of the data and the extremities of these whiskers are the minimum and maximum values of the data. The horizontal lines at the top of the graph indicate where significant differences exist between groups (p < 0.05).
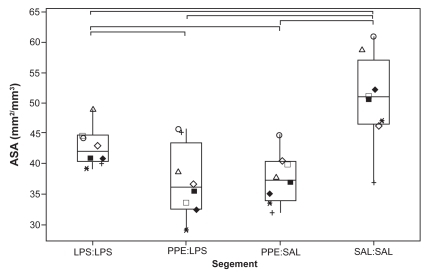
Figure 3 Coefficient of variation of individual ASA measurement values. Each dot represents the value calculated on the basis of all individual ASA measurements made on each segment from each sheep. See legend for explanation of boxplots.
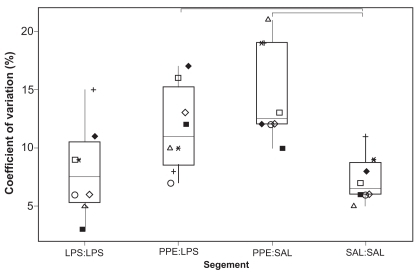
Figure 4 Number of normal alveolar attachments surrounding nonrespiratory bronchioles < 1 mm diameter. Each dot represents the mean value calculated on the basis of measurements made on 10 airways randomly selected from sections taken from 3 blocks from each segment. See legend for explanation of boxplots.
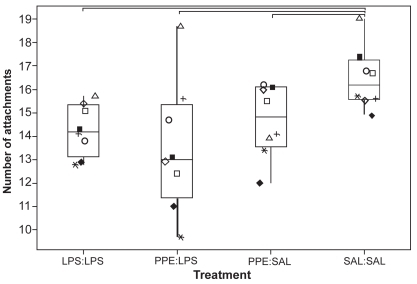
Figure 5 Distance between normal alveolar attachments surrounding nonrespiratory bronchioles < 1 mm diameter. Each dot represents the mean value calculated on the basis of measurements made on 10 airways randomly selected from sections taken from 3 blocks from each segment. See legend for explanation of boxplots. ◂ denotes an outlier.
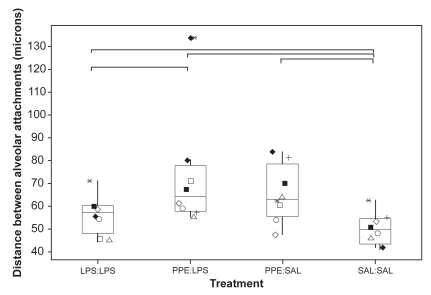
Figure 6 The percentage disruption of normal alveolar attachments (distance = external circumference/(no. attached)) and the percentage of abnormal attachments (% abnormal = [number of abnormal/(no. attached + number of abnormal)] × 100). Each dot represents the mean value calculated on the basis of measurements made on 10 airways randomly selected from sections taken from 3 blocks from each segment. See legend for explanation of boxplots. ◂ denotes an outlier.
![Figure 6 The percentage disruption of normal alveolar attachments (distance = external circumference/(no. attached)) and the percentage of abnormal attachments (% abnormal = [number of abnormal/(no. attached + number of abnormal)] × 100). Each dot represents the mean value calculated on the basis of measurements made on 10 airways randomly selected from sections taken from 3 blocks from each segment. See Figure 2 legend for explanation of boxplots. ◂ denotes an outlier.](/cms/asset/87a49696-924a-4d6c-bc62-c6e0b58e37fc/dcop_a_12189_f0006_b.jpg)
Levels of neutrophil elastase in BALF did not show any evidence of significant change, either within any segment over time or between segments within animals (data not shown). Elastase inhibitor activity in BALF declined over time in all segments, with the decline in activity between day −7 and day 39 being significant for all bar the LPS:LPS segment in which a nonsignificant trend was apparent (p = 0.093; ). Overall, pooling all the data from individual lung segments, the decline in elastase inhibitory activity was significantly negatively correlated with the absolute number of neutrophils in BALF (p = 0.02).
Figure 7 Plots showing elastase inhibitory activity in BALF obtained from individual lung segments both prior to (day −7) and after (day 39) the different treatment protocols. Different symbols represent individual sheep. Levels of significance of changes are shown (Wilcoxon signed rank test). The horizontal bars depict mean values.
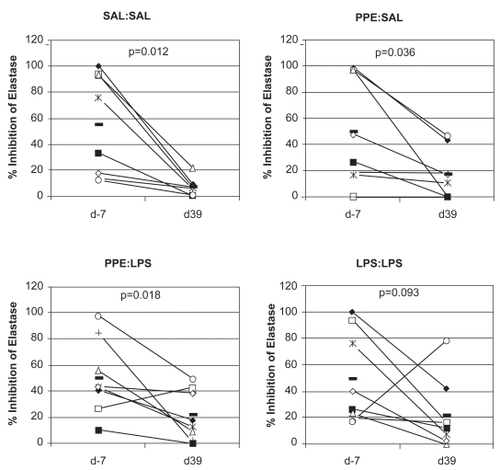
Total TGF-β levels in BALF did not show any evidence of significant change, over time. In contrast, in LPS:LPS segments, levels of active TGF-β in BALF decreased significantly (p < 0.05) over time. Similarly the reduction in levels of active TGF-β in BALF from PPE:LPS segments approached significance (p = 0.05; ).
Figure 8 Plots showing levels of active transforming growth factor-β1 (TGF-β1) in BALF obtained from individual lung segments both prior to (day −7) and after (day 39) the different treatment protocols. Different symbols represent individual sheep. Levels of significance of changes are shown (Wilcoxon signed rank test). The horizontal bars depict mean values.
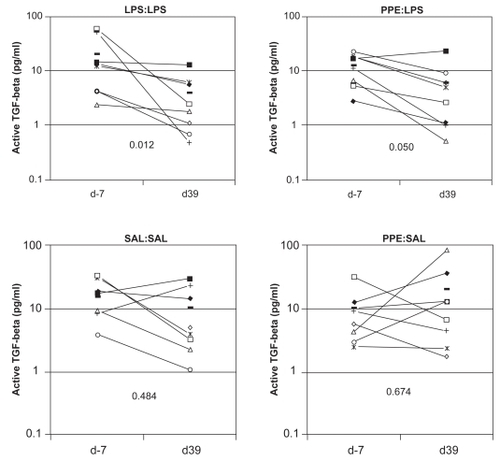
Discussion
Of the indices studied, a reduction in ASA reflects an increase in the size of individual airspaces. In humans, such measurements are particularly sensitive to detecting panacinar emphysema, in which the enlargement of airspaces involves the whole acinar unit and the distinction between alveoli and alveolar ducts is lost. In contrast, with centriacinar emphysema, where sharply demarcated emphysematous spaces are separated from the acinar periphery by intact normal alveolar ducts and alveolar sacs, there may be minimal influence on the overall ASA value. In the latter instance early focal changes are often detectable as an increased CV of individual measurements (CitationLamb 1996). The significant decrease in ASA in LPS:LPS, PPE:SAL, and PPE:LPS segments relative to SAL:SAL segments suggests that either the pattern of disruption is panacinar in nature or that the extent of centriacinar disruption is sufficiently widespread to influence the measurement of ASA. The greater CV of ASA measurements in PPE-treated segments suggests the latter. What is of interest is that in the LPS:LPS segments a significant and uniform reduction in ASA occurred, suggesting differences in the model phenotype and possibly also in the pathogenesis when compared with elastase-treated segments. Such findings are consistent with observations from tissue sections from lungs of LPS-instilled hamsters, in which the emphysematous lesions appeared more diffuse in the lung compared with elastase-induced emphysema, in which patchy airspace enlargement is mainly located around the airways (CitationSnider et al 1984; CitationStolk et al 1992).
An increase in the interalveolar attachment distance is recognized in emphysema (CitationLinhartova et al 1982). Such measurement reflects the degree of parenchymal support to bronchioles and small bronchi. Loss of support as a consequence of airspace enlargement or selective loss of alveolar wall attachments may lead to distortion of small airways with obvious implications for airflow limitation. As pointed out by CitationSaetta et al (1985), all three alveolar attachment indices measured in this study can reflect the destruction of alveolar attachments, but only the percentage abnormal attachments quantifies this destruction directly. In the total absence of an alveolar wall it would not be counted as abnormal, but such an instance would be reflected in a reduction in the number of intact attachments and a greater distance between intact attachments. All three treatment protocols (LPS:LPS, PPE:SAL, and PPE:LPS) significantly increased percentage of abnormal attachments and the distance between normal attachments, and significantly reduced the number of normal attachments. The extent of change was generally more marked in the PPE:LPS segment.
The evidence presented suggests that chronic treatment of sheep with LPS at the lung segmental level induces structural changes within the treated segment consistent with the definition of emphysema as defined at the National Heart, Lung and Blood Institute Workshop on the Definition of Emphysema in 1985 (CitationSnider et al 2003). Namely, “a condition of the lung characterized by abnormal, permanent enlargement of air spaces distal to the terminal bronchioles accompanied by destruction of their walls and without obvious fibrosis”. Further, although qualitative and quantitative similarities exist in relation to segmental treatment with elastase, there is a suggestion that chronic LPS treatment produces a more diffuse change throughout the lung segment, whereas elastase treatment is particularly focused around the airways.
A mild increase in absolute numbers of neutrophils was apparent in all segments at the culmination of the study in relation to the baseline evaluation on day −7. In a study involving repeated bronchoalveolar lavage at 4- to 7-day intervals, we were unable to demonstrate any significant changes in absolute BALF cell numbers over time (CitationCollie et al 1999), implying that the observed increase is unlikely to be related to the procedural effect of repeated bronchoscopy. In sheep, treatment of lung segments with elastase induces an intense local neutrophilic inflammatory response in the first week, which changes in character to mononuclear in the second week and resolves over the course of the third week (CitationSusskind et al 1985). Thus, changes in the PPE:SAL segments seen at day 39 are considered unlikely to be a direct consequence of the initial elastolytic insult. Intratracheal treatment of lambs with LPS causes an acute fibrinopurulent pneumonia, which resolves within 72 hours. Neutrophils are sequestered in the capillaries of the lungs and surfactant aggregates in the alveoli. Swelling of the alveolar and capillary endothelia, edema, hemorrhage, and emigration of neutrophils into the interstitium and small air spaces of the lungs occur subsequently (CitationCutlip et al 1998). Although it is likely that the interval between treatments would be sufficient for the major part of the above processes to resolve, it is unlikely that complete resolution would be realized, and it is therefore possible that the “lung-wide” changes are a consequence of the repeated inflammatory insult in just two lung segments. The specific changes are likely to involve modified adhesion molecule expression on pulmonary vascular endothelia (CitationStrieter and Kunkel 1994). Further, changes in the neutrophil vascular pool cannot be discounted. The exact nature of such change is speculative but might involve a shift in the proportion of blood neutrophils that are either normal, primed, activated, or senescent with according changes in the proportion that are phagocytically, chemotactically, and oxidatively active.
Similarly a significant reduction in the elastase inhibitory capacity of BALF from all segments was observed at the culmination of the study relative to baseline evaluation on day −7. Notably, on the pooled data from all segments a significant negative correlation was demonstrated between absolute numbers of neutrophils and elastase inhibitory capacity, perhaps implying that neutrophil influx is linked to a progressive impairment of the elastase inhibitory screen. The failure to demonstrate any change in free neutrophil elastase in any segment could be interpreted as representing evidence that neutrophil elastase specific inhibitors in the bronchoalveolar space have not been completely overwhelmed at the point of sampling. Further, the evident lack of association between neutrophils, free neutrophil elastase, or elastase inhibitory capacity and the emphysema phenotype poses a question as to the relevance of these variables in the pathogenesis of emphysema in this model. Naturally the caveat that measurements are taken a week after the preceding treatment and do not in any case necessarily reflect the potential for interstitial proteolysis should caution against overinterpretation of this lack of association. Acute neutrophilic influx, with attendant release of neutrophil elastase, may still play a predominant role in the pathogenesis of emphysema in this model.
TGF-βs are multifunctional cytokines that are usually secreted in a biologically latent form called either small or large latent TGF-β. The major aspect of controlling the activity of these cytokines rests with the conversion of latent to active TGF-β through proteolytic cleavage or conformational alteration of the latent complex. The former is believed to be largely mediated through plasmin and the latter through the interaction of RGD-motifs on the latency associated peptide with the integrin αvβ6 expressed on epithelial cells. Although much less is known in relation to the mechanisms that conspire to downregulate TGF-β activation, it is clear that a reduction in plasmin synthesis through TGF-β-mediated induction of plasminogen activator inhibitors or a reduction in the expression of the integrin αvβ6 might serve this purpose. The evidence that levels of active TGF-β are significantly less than pre-treatment levels in LPS:LPS segments implies that control mechanisms seek to regulate a basal level of activation in the bronchoalveolar milieu, but that a consequence of chronic LPS administration is a downward regulation of this level. Whether reduced activation of TGF-β is a cause or consequence of the emphysema phenotype in this model system remains to be determined. However, downregulated activity of this cytokine has been clearly linked to an emphysema phenotype and an upregulation of the monocyte–macrophage-expressed elastase, matrix metalloproteinase-12, in αvβ6 gene deleted mice (CitationMorris et al 2003), illustrating that the potential for a causative role certainly exists.
The presence of increased numbers of alveolar macrophages and lymphocytes in all treated segments at day 14 relative to the SAL:SAL segment are, in the context of the elastase-treated segments, in common with the observations of CitationSusskind et al (1985), who commented that during the first week following elastase instillation there was an acute inflammatory response, primarily polymorphonuclear leukocytes, in the enzyme-treated lung, which changed to mononuclear cell infiltrate during the course of the second week, and essentially disappeared by the third week. Although not a feature recognized in BALF collected at the culmination of the study, a mononuclear alveolitis was identified at the histopathologic level in LPS-treated segments 7 days after the last treatment. Such an alveolitis is also a feature of lung pathology following long-term LPS instillation in mice (CitationVernooy et al 2002) and is accompanied, in this model, by dense peribronchial and perivascular lymphocytic aggregates.
The reduced numbers of eosinophils and mast cells seen in these sheep should be viewed in the context of the low absolute numbers involved, ie, in only 5% of the BALF samples did the percentage of eosinophils or mast cells exceed 1% of the total cell count. Thus caution should be extended in interpreting these data. Nevertheless, it is conceivable that the reduced numbers present in BALF may be a reflection of increased degranulation of this cell type in tissue compartments as a consequence of tissue repair processes in treated lung. Certainly, mast cells play a fundamental role in tissue remodeling processes including degradation of matrix proteins, promotion of vascular and epithelial permeability, and recruitment of inflammatory cells and eosinophils, though the production of matrix metalloproteinases may play a similar role (CitationDavis et al 1984; CitationOhno et al 1997).
In conclusion, our results indicate that repeated administration of LPS at the lung segmental level in sheep results in the development of mild mononuclear alveolitis and downregulated activation of TGF-β in BALF. Although these features appear to be associated with the development of emphysema in treated lung segments, the relative role of neutrophil and mononuclear cell infiltrates in contributing to such tissue-remodeling processes remains to be determined in this model.
Acknowledgments
The authors wish to thank the animal care and welfare personnel at the Easter Bush Veterinary Centre, Roslin for their assistance in this research. The research was funding by University of Edinburgh, Faculty Research Fund.
References
- AbeMHarpelJGMetzCN1994An assay for transforming growth factor-beta using cells transfected with a plasminogen activator inhibitor-1 promoter- luciferase constructAnal Biochem216276848179182
- BeginRRola-PleszczynskiMSiroisP1981Sequential analysis of the bronchoalveolar milieu in conscious sheepJ Appl Physiol50665717251456
- CollieDDMacaldowieCNPembertonAD2001Local lung responses following local lung challenge with recombinant lungworm antigen in systemically sensitized sheepClin Exp Allergy3116364711678866
- CollieDDSBakerAMauchlineS1999Ovine bronchoalveolar lavage cellularity: Reproducibility and the effect of multiple repeated lavageRes Vet Sc671374010502482
- CutlipRCBrogdenKALehmkuhlHD1998Changes in the lungs of lambs after intratracheal injection of lipopolysaccharide from Pasteurella haemolytica A1J Comp Pathol11816379573513
- DavisWBFellsGASunXH1984Eosinophil-mediated injury to lung parenchymal cells and interstitial matrix. A possible role for eosinophils in chronic inflammatory disorders of the lower respiratory tractJ Clin Invest74269786330175
- FinlayGAO’DriscollLRRussellKJ1997Matrix metalloproteinase expression and production by alveolar macrophages in emphysemaAm J Respir Crit Care Med15624079230755
- FukudaYMasudaYIshizakiM1989Morphogenesis of abnormal elastic fibers in lungs of patients with panacinar and centriacinar emphysemaHum Pathol2065292661409
- GrossPBabyakMATolkerE1964Enzymatically produced pulmonary emphysema: a preliminary reportJ Occup Med6481414241128
- JanoffA1983Biochemical links between cigarette smoking and pulmonary emphysemaJ Appl Physiol55285936352576
- LambDHasletonPS1996Chronic bronchitis, emphysema, and the pathological basis of chronic obstructive pulmonary diseaseSpencer’s pathology of the lung5th edNew YorkMcGraw-Hill597629
- LaurellCBErickssonS1963The electrophoretic alpha-1-globulin pattern of serum in alpha-1-antitrypsin deficiencyScand J Clin Lab Invest1513240
- LawsonMFMcGarryMDonaldsonK1995Induction of microscopic emphysema in the rat after serial instillation of lipopolysaccharideAm J Respir Crit Care Med151A66
- LinhartovaAAndersonAEJrForakerAG1982Affixment arrangements of peribronchiolar alveoli in normal and emphysematous lungsArch Pathol Lab Med10604995026896979
- MercerRRCrapoJD1992Structural changes in elastic fibers after pancreatic elastase administration in hamstersJ Appl Physiol72147391592740
- MorrisDGHuangXKaminskiN2003Loss of integrin alphavbeta6-mediated TGF-beta activation causes Mmp12-dependent emphysemaNature42216917312634787
- OhnoIOhtaniHNittaY1997Eosinophils as a source of matrix metalloproteinase-9 in asthmatic airway inflammationAm J Respir Cell Mol Biol16212199070604
- PardoASelmanM1999Proteinase-antiproteinase imbalance in the pathogenesis of emphysema: the role of metalloproteinases in lung damageHistol Histopathol14227339987667
- RudolphusAStolkJDijkmanJH1993Inhibition of lipopolysaccharide-induced pulmonary emphysema by intratracheally instilled recombinant secretory leukocyte proteinase inhibitorAm Rev Respir Disease14744278094278
- SaettaMGhezzoHKimWD1985Loss of alveolar attachments in smokers. A morphometric correlate of lung function impairmentAm Rev Respir Disease1328949004051324
- ShapiroSD1994Elastolytic metalloproteinases produced by human mononuclear phagocytes. Potential roles in destructive lung diseaseAm J Respir Crit Care Med150S16047952653
- SniderGLKleinermanJThurlbeckWM2003The definition of emphysema. Report of the National Heart, Lung and Blood Institute, Division of Lung Diseases WorkshopAm Rev Respir Disease1321825
- SniderGLLuceyECChristensenTG1984Emphysema and bronchial secretory cell metaplasia induced in hamsters by human neutrophil productsAm Rev Respir Disease129155606561016
- SniderGLLuceyECStonePJ1986Animal models of emphysemaAm Rev Respir Disease133149693510579
- StolkJRudolphusADaviesP1992Induction of emphysema and bronchial mucus cell hyperplasia by intratracheal instillation of lipopolysaccharide in the hamsterJ Pathol167349561517904
- StrieterRMKunkelSL1994Acute lung injury: the role of cytokines in the elicitation of neutrophilsJ Investig Med4264051
- SusskindHChananaADJoelDD1985Assessment of elastase-induced structural and functional changes in sheep lungsBull Eur Physiopathol Respir21149573846460
- VernooyJHDentenerMAvan SuylenRJ2002Long-term intratracheal lipopolysaccharide exposure in mice results in chronic lung inflammation and persistent pathologyAm J Respir Cell Mol Biol26152911751215
- VlahovicGRussellMLMercerRR1999Cellular and connective tissue changes in alveolar septal walls in emphysemaAm J Respir Crit Care Med16020869210588633
- WrightRR1961Elastic tissue of normal and emphysematous lungsAm J Pathol393556313786819