Abstract
Oxidative stress has been implicated in the pathogenesis and progression of COPD. Both reactive oxidant species from inhaled cigarette smoke and those endogenously formed by inflammatory cells constitute an increased intrapulmonary oxidant burden. Structural changes to essential components of the lung are caused by oxidative stress, contributing to irreversible damage of both parenchyma and airway walls. The antioxidant N-acetylcysteine (NAC), a glutathione precursor, has been applied in these patients to reduce symptoms, exacerbations, and the accelerated lung function decline. This article reviews the available experimental and clinical data on the antioxidative effects of NAC in COPD, with emphasis on the role of exhaled biomarkers.
Introduction
Inflammation and oxidative stress play an important role in the pathogenesis of many chronic inflammatory lung disorders such as COPD, asthma, bronchiectasis, and cystic fibrosis (CF). A complex interplay between the specific cause of the disease, host characteristics, and the type and intensity of inflammation and oxidative stress results in the clinical picture and likelihood of progression of that specific disease. Noninvasively obtained biomarkers of inflammation and oxidative stress have been identified, and, ideally, should represent the intensity of the ongoing pathological processes within the lungs. This review focuses on the role of oxidative stress in the pathogenesis of COPD and the ability of N-acetylcysteine (NAC) to attenuate this process. Specific attention is paid to the role of biomarkers that follow the course of the disease and the effect of antioxidant interventions.
Oxidants and antioxidants in the lungs of patients with COPD
Several reviews have summarised the available data on the presence and consequences of oxidative stress in the lungs of “healthy” smokers and smokers with COPD (CitationRepine et al 1997; CitationMacNee and Rahman 1999; CitationRahman and MacNee 1999; CitationBarnes 2000; CitationMacNee 2000) (see ). Cigarette smoke is a major source of oxidants in the lungs, eg, free radicals, nitric oxide (NO), semiquinone radicals, hydroxyl radicals, and hydrogen peroxide (H2O2) (CitationMacNee and Rahman 1999). Furthermore, cigarette smoke promotes the influx and activation of neutrophils and macrophages. Leukocytes from smokers release more oxidants like superoxide anion (O2−) and H2O2 than leukocytes from nonsmokers (CitationMorrison et al 1999). The alveolar macrophages of smokers contain increased amounts of iron and release more free iron than those of nonsmokers CitationMateos et al (1998). The presence of free iron facilitates the generation of very reactive hydroxyl radicals.
Table 1 Indices of increased oxidative stress in COPD. Modified after CitationRahman and MacNee (1999)
An important part of the pulmonary antioxidant defense is located in the epithelial lining fluid (ELF). Vitamin C and vitamin E levels in ELF are depleted in smokers, but glutathione (GSH) levels are increased (CitationCantin et al 1987; CitationRahman and MacNee 1996; CitationMorrison et al 1999). These effects depend on the time-course of exposure to tobacco smoke. Acute exposure markedly depletes antioxidants in plasma (CitationRahman et al 1996, Citation1997), intracellular GSH of erythocytes (CitationMaranzana and Mehlhorn 1998), and GSH levels in ELF (CitationCantin et al 1987; CitationMorrison et al 1999).
Alterations in the lung caused by oxidative stress
Both in vitro and in vivo experiments have demonstrated that oxidative stress may cause alterations in essential components of the lung, contributing to pathological abnormalities and functional changes () (CitationRahman and MacNee 1996; CitationWarren et al 1997).
Table 2 Alterations in components of the lung caused by oxidative stress. Modified after CitationRahman and MacNee (1996), CitationWarren et al (1997), and CitationDekhuijzen (2004)
Increased numbers of reactive oxygen species (ROS) have been shown to reduce the synthesis of elastin and collagen (CitationLaurent et al 1983; CitationCantin and Crystal 1985). ROS may also affect the structure of components of the extracellular matrix, such as hyaluronate (CitationWarren et al 1997). Depolymerisation of the proteoglycans in the lung reduces the viscosity of the extracellular matrix. Oxidative stress may also initiate or amplify alterations in the airway wall. Lipid peroxidation may initiate the release of arachidonic acid from membrane phospholipids, in this way leading to release of prostaglandins and leukotrienes. Increased levels of ROS may also increase interleukin-1 (IL-1) and IL-8 production in several cell systems (CitationGhezzi et al 1991; CitationMetinko et al 1992). Other effects include changes in protein structure leading to altered antigenicity and thus immune responses, contraction of smooth muscle, impairment of beta-adrenoceptor function, stimulation of airway secretion, pulmonary vascular smooth muscle relaxation or contraction, and activation of mast cells (CitationWarren et al 1997). ROS may inactivate antiproteases such as alpha-1-proteinase inhibitor and secretory leukoprotease inhibitor (CitationAbboud et al 1985). Changes in the alveolar epithelial cell layer occur both as a direct result of inhaled ROS and through the aforementioned alterations (CitationCotgreave and Moldeus 1987). Sequestration of neutrophils may occur in the lung microcirculation, initiated by inhaled tobacco smoke (CitationMacNee et al 1989). The increased and prolonged presence of these inflammatory cells contributes to, for example, the cycle of locally increased ROS production and attraction of new inflammatory cells. Finally, oxidative stress activates the transcription factor nuclear factor kappa-beta (NF-kb), which switches on the genes for tumor necrosis factor-alpha (TNF-alpha), IL-8, and other inflammatory proteins (CitationBarnes 2000; CitationRahman and MacNee 2000), in this way enhancing inflammation.
Taken together, these data strongly suggest that oxidative stress is an important pathogenetic factor in the alterations in the lungs of patients with COPD. Attenuation of oxidative stress would be expected to result in reduced pulmonary damage and a decrease of local infections, in this way contributing to attenuation of the progression of COPD. At present the only antioxidant widely available for the treatment in patients with COPD is NAC.
Antioxidant therapy with NAC in COPD
Antioxidant properties
NAC has direct and indirect antioxidant properties. Its free thiol group is capable of interacting with the electrophilic groups of ROS (CitationMoldeus et al 1986; CitationAruoma et al 1989). This interaction with ROS leads to an intermediate formation of NAC thiol, with NAC disulfide as a major end product (CitationCotgreave 1997). NAC also exerts an indirect antioxidant effect related to its role as a GSH precursor. GSH is a tripeptide made up of glutamic acid, cysteine, and glycine. It serves as a central factor in protecting against internal toxic agents (such as cellular aerobic respiration and metabolism of phagocytes) and external agents (such as NO, sulfur oxide, and other components of cigarette smoke, and pollution). The sulfydryl group of cysteine neutralises these agents. In vitro, NAC acts as a precursor of GSH as it can penetrate cells easily and is subsequently deacylated to deliver cysteine (CitationMoldeus et al 1986).
Clinical pharmacology
NAC is rapidly absorbed after oral administration (CitationSheffner et al 1966; CitationRodenstein et al 1978; CitationBorgstrom et al 1986). Maximum plasma concentration after oral administration is reached after 2–3 hours (CitationBridgeman et al 1991) and plasma half-life is 6.3 hours. NAC undergoes extensive hepatic metabolism, resulting in a low bioavailability of about 10% of the unchanged molecule. Cysteine and GSH levels are increased transiently in plasma (CitationCotgreave et al 1987; CitationBridgeman et al 1991) and lung (CitationBridgeman et al 1991) after oral administration of NAC 600 mg once daily. In patients with COPD, 600 mg three times daily increased plasma GSH levels (CitationBridgeman et al 1994). With this dose, administered for 5 days to patients who underwent lung resection surgery, cysteine and GSH levels were increased by ~50% compared with untreated patients. These data suggest that there is a transient dose-dependent effect of NAC on lung cysteine and GSH levels.
Antioxidant and anti-inflammatory effects
The efficacy of NAC as a precursor of GSH synthesis has been studied in isolated mouse lungs (CitationMoldeus et al 1986). Cigarette smoke administered directly to the lung through the trachea caused a dose-dependent reduction in total pulmonary GSH. Administering NAC together with cigarette smoke prevented the loss of pulmonary GSH and abolished the effects of cigarette smoke. NAC reduced H2O2-induced damage to epithelial cells in vitro (CitationCotgreave and Moldeus 1987) and NF-kb activation in some cells (CitationSchreck et al 1992). In addition, NAC treatment reduced cigarette smoke-induced abnormalities in polymorphonuclear leukocyte (PMN) (CitationBridges 1985), alveolar macrophages, fibroblasts, and epithelial cells in vitro (CitationMoldeus et al 1985; CitationVoisin 1987; CitationLinden et al 1988; CitationDrost et al 1991). Treatment with NAC also attenuated rat secretory cell hyperplasia induced by tobacco smoke (CitationJeffery et al 1985) and prevented hypochlorous acid (HOCl)-mediated inactivation of alpha-1-proteinase inhibitor in vitro (CitationBorregaard 1987). In a rat model of cigarette smoke-induced alterations in small airways, NAC prevented thickening of the airway wall and improved distribution of ventilation (CitationRubio et al 2000).
Treatment with NAC in humans alters the pulmonary oxidant–antioxidant imbalance. NAC 600 mg/day given orally increased lung lavage GSH levels (CitationBridgeman et al 1991), reduced O2− production by alveolar macrophages (CitationLinden et al 1988), and decreased bronchoalveolar lavage (BAL) polymorphonuclear leukocyte (PMN) chemiluminescence in vitro (CitationJankowska et al 1993). NAC 600 mg/day in COPD patients also reduced sputum eosinophilic cation protein (ECP) concentrations and the adhesion of PMNs (CitationSadowska et al 2005). In vitro, NAC lowered adhesion of H. influenzae and S. pneumoniae to oropharyngeal epithelial cells (CitationRiise et al 2000).
Effects on cigarette smoke-induced changes
Three studies have investigated the effects of NAC 600 mg/ day p.o. on parameters of inflammation in BAL fluid of “healthy” smokers (CitationBergstrand et al 1986; CitationEklund et al 1988; CitationLinden et al 1988). NAC resulted in a tendency towards normalization of the cell composition with an increase in lymphocyte concentration (CitationLinden et al 1988). In addition, improvements were observed in phagocytic activity of alveolar macrophages (CitationLinden et al 1988) and an increase in the secretion of leukotriene B4 (CitationLinden et al 1988). NAC also reduced the stimulated production of superoxide radicals (CitationBergstrand et al 1986). Finally, markers of inflammatory activity, such as eosinophil cationic protein, lactoferrin, and antichymotrypsin (p < 0.05) were reduced after administration of NAC (CitationEklund et al 1988).
Effects on elastase activity
NAC treatment considerably reduced elastase activity, both in the bronchoalveolar cavity and in the plasma, which is related to its property to scavenge HOCl (CitationAruoma 1989).
Modulatory effect on genes
Redox signalling forms part of the fundamental mechanisms of inflammation, such as cytokine induction, proliferation, apoptosis, and gene regulation for cell protection. Oxidants act as mediators of signal transduction, eg, activation of NF-kb and activator protein-1 (AP-1). NAC has been shown to inhibit activation of NF-kb, which controls the cellular genes for intracellular adhesion molecules in intact cells (CitationSchreck et al 1992). In addition, NAC has been shown to inhibit the expression of vascular cell adhesion molecule-1 in human endothelial cells (CitationMarui et al 1993).
Effects on oxidative stress induced by viruses
Oxidant production in respiratory cells rises when they become infected with pathogenic viruses and this is accompanied by increased production of a variety of inflammatory mediators. NAC has been shown to have a protective role by increasing the resistance of mice to influenza virus (Streightoff et al 1966). Influenza virus increased the production of ROS in epithelial cells, and activated NF-kb (CitationKnobil et al 1998). Pretreatment with NAC attenuated virus-induced NF-kb and IL-8 release. Mice infected intranasally with influenza virus APR/8 showed high BAL levels of xanthine oxidase, TNF, and IL-6 as early as 3 days after infection (CitationAkaike et al 1990). Xanthine oxidase was also elevated in serum and lung tissue. Administration of oral NAC 1 g/kg daily significantly reduced the mortality of the infected mice (p < 0.005). Rhinoviruses also stimulated increased production of H2O2 and oxidative stress of human respiratory epithelial cells (CitationBiagioli et al 1999). Oxidative stress, in turn, caused activation of NF-kb and release of IL-8 and this effect was blocked by NAC in a dose-dependent manner.
Exhaled biomarkers of oxidative stress
Clinically, physicians may expect that exhaled markers reveal insight into the pathophysiology, have a role in the assessment of the severity of inflammation–oxidative stress, predict lung function deterioration, and provide individual guidance during (pharmaco)therapy. Some biomarkers cannot be measured directly in exhaled air, but have to be measured in exhaled breath condensate (EBC), because they are not gaseous. The principle is to cool the exhaled air to a temperature around 0°C, so that the warm air condensates. The most common approach is to ask the subject to breathe tidally via a mouthpiece through a non-rebreathing valve in which inspiratory and expiratory air are separated. During expiration the exhaled air flows trough a condenser, which is cooled to 0°C by, for example, melting ice, and breath condensate is then collected into a collection vessel. Several markers can be measured in exhaled breath condensate, including H2O2, isoprostanes, NO metabolites, and thiobarbituric acid-reactive substances (TBARs).
Hydrogen peroxide
In both asthma and COPD, airway inflammation is the most prominent characteristic. Activated inflammatory cells respond with a “respiratory burst”, which results in the production of ROS. Naturally occurring free radicals have an oxygen- or nitrogen-based unpaired electron. Classical examples are superoxide anion (O2−), hydroxyl radical (OH−), and NO. O2− is formed from oxygen. Reaction of O2−and H2O2 in the presence of transition metal produces OH. When catalyzed by neutrophil myeloperoxidase, H2O2 and a chloride form HOCl. H2O2 acts as a central precursor. H2O2 levels reflect the underlying state of oxidative stress in the lungs. The level of exhaled H2O2 significantly depends on expiratory flow rate, indicating that the exhaled H2O2 is at least partially produced within the airways (CitationSchleiss et al 2000).
Methods of H2O2 measurement in breath condensate are based on the ability of H2O2 to react with suitable substrates leading to the release of colour, light, or fluorescence. Two methods of analysis have been used most: the spectrophoto-metrical method and the fluorimetrical method.
The concentration in normal individuals is almost undetectable and in many diseases the levels found are at the lower limit of detection of the assays employed. In COPD patients, the exhaled H2O2 shows a circadian rhythm and significant variability over a period of one day and several weeks (CitationJeffery 2002). The concentration of H2O2 in EBC is increased in stable COPD patients with a further increase during exacerbations (CitationDekhuijzen et al 1996).
Increased levels of H2O2 in EBC have been shown in stable COPD patients, with a further increase during exacerbations (CitationDekhuijzen et al 1996). Treatment with NAC 600 mg once daily for 12 months reduced the concentration of H2O2 in EBC compared with placebo in stable COPD patients (FEV1 ~60%–70% of predicted) (CitationKasielski and Nowak 2001). This effect was observed in the second 6 months of the treatment period. A higher dose of NAC (1.2 g once daily) reduced the concentration of H2O2 in EBC within 30 days, suggesting that there is a dose-dependent effect on this marker of oxidative stress (CitationDe Benedetto et al 2005).
Isoprostanes
Oxygen radicals such as O2 and O2− react with unsaturated bonds of arachidonic acid, leading to the formation of bicycloendoperoxide intermediates. The reduction of these intermediates leads to the formation of isoprostanes. Reactive nitrogen species and polyunsaturated fatty acids other than arachidonic acid may also participate in the production of isoprostanes. Isoprostanes can be measured with analytic techniques such as high pressure liquid chromatography, gas chromatography–mass spectrometry or radio-immunoassay. They are stable in isolated samples of body fluids and their measured values do not exhibit diurnal variations.
Montuschi and co-workers (CitationMontuschi et al 2000) showed significantly higher 8-isoprostane levels in COPD patients than in healthy subjects and found no difference between current smokers and ex-smokers, indicating that in COPD, exhaled 8-isoprostane is derived largely from oxidative stress from airway inflammation rather than from cigarette smoking. There was no relation between 8-isoprostane and age, sex, FEV1, and history of smoking.
NO metabolites
NO is a highly reactive molecule with a relatively short half-life in vivo. It can be oxidized or complexed with other biomolecules. The stable oxidation end products of NO metabolism are nitrite (NO2−) and nitrate (NO3−). NO can undergo a reaction with superoxide anion (O2−) to form peroxynitrite (ONOO−). Peroxynitrite reacts with tyrosine residues in proteins to form the stable product nitrotyrosine. NO and NO metabolites can also react with thiols (the major enzymatic antioxidants) to produce S-nitrosothiols. The concentration of nitrite can be assessed by a fluorimetric assay. Incubation of samples with nitrate reductase allows the nitrate to be converted to nitrite, which can be measured subsequently.
Recently, Corradi and co-workers compared nitrosothiols levels in EBC of healthy subjects (smoker and nonsmokers), asthma patients (mild and severe), COPD patients, and patients with CF (CitationCorradi et al 2001). Levels of nitrosothiols were elevated in COPD patients compared with nonsmoking controls.
TBARs
TBARs are also a product of lipid peroxidation; they are volatile and may be present in expired breath. They are low-molecular-weight compounds formed by the decomposition of certain primary and secondary lipid peroxidation products. At a low pH and elevated temperature, the lipid peroxidation products participate in a nucleophilic addition reaction with thiobarbituric acid, generating a red fluorescent complex. They can be measured colorimetrically or fluorimetrically.
In a cross-sectional study the concentration of TBARs was elevated in stable COPD patients compared with healthy subjects. TBARs and H2O2 concentration were elevated in asthma patients compared with both healthy control subjects and COPD patients. A significant positive correlation was found between H2O2 concentration and TBARs concentration (CitationNowak et al 1999).
Effects of NAC on lung function and exacerbations
Lung function
In an open, observational survey in Sweden, the decline in FEV1 in COPD patients who took NAC for 2 years was less than in a reference group on usual care (CitationLundbäck et al 1992). This favorable effect was particularly apparent in COPD patients over 50 years of age (yearly decline of 30 mL in FEV1) compared with the reference group (yearly decline of 54 mL in FEV1). After 5 years, the loss of FEV1 in the NAC group was less than in the reference group (Lundbäck B 1993, pers comm). Clearly, it should be noted that the nature of the study design precludes firm conclusions on the effect of NAC on lung function decline in COPD.
Recently, the Bronchitis Randomized on NAC Cost-Utility Study (BRONCUS) trial was conducted, testing the hypothesis that treatment with the antioxidant NAC would reduce the rate of lung function decline, reduce yearly exacerbation rate, and improve outcome variables (CitationDecramer et al 2005). Patients with COPD (n = 523) were randomized in a multicenter, placebo-controlled study of NAC administered orally in a dose of 600 mg once daily. Patients were followed for 3 years. The rate of decline in FEV1 or vital capacity (VC) was not different in the 256 patients in the NAC group and the 267 patients in the placebo group. Secondary analysis in patients completing the trial showed that after 3 years, functional residual capacity (FRC) was decreased (− 0.374 L) in the NAC group, while it was slightly increased (+ 0.008 L) in the placebo group (p = 0.008).
Exacerbations
In a recent systematic review by CitationStey et al (2000), data on prevention of exacerbation, improvement of symptoms, and adverse effects were extracted from original reports (). The relative benefit and number-needed-to-treat were calculated for both individual trials and combined data. In 9 studies, 351 of 723 (48.5%) patients receiving NAC had no exacerbation compared with 229 of 733 (31.2%) patients receiving placebo: relative benefit 1.56 (95% confidence interval [CI] 1.37–1.77), number-needed-to-treat 5.8 (95% CI 4.5–8.1). There was no evidence of any effect of study period (12–24 weeks) or cumulative dose of NAC on efficacy. In 5 trials, 286 of 466 (61.4%) patients receiving NAC reported improvement of their symptoms compared with 160 of 462 (34.6%) patients receiving placebo: relative benefit 1.78 (95% CI 1.54–2.05), number-needed-to-treat 3.7 (95% CI 3.0–4.9). These findings are in line with the outcomes of two previous meta-analyses using a less precise selection of these studies (CitationGrandjean et al 2000; CitationPoole and Black 2001) and confirm that NAC has a clinically significant effect on the number and impact of exacerbations. Again, it should be stressed that patients included in these studies were not characterised as detailed, as currently would be demanded according to, eg, the GOLD guidelines (CitationPauwels et al 2001).
Figure 1 Absence of any exacerbation with oral N-acetylcysteine (NAC) or placebo (P) in patients with COPD and/or chronic bronchitis. Each symbol represents one trial. Symbol sizes are proportional to trial sizes. Arrows are weighted means. -------- = line of equality. Source: CitationStey et al. 2000. The effect of oral N-acetylcysteine in chronic bronchitis: a quantitative systematic review. Eur Respir J, 16:253–62. Copyright © 2000. Reproduced with permission of the European Respiratory Society Journals Ltd.
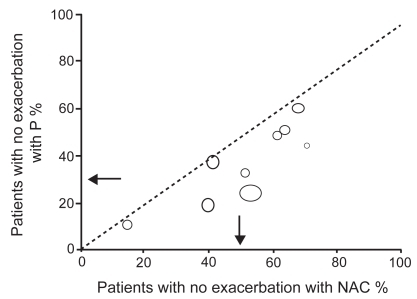
In the abovementioned BRONCUS trial, NAC did not influence the yearly exacerbation rate, but the hazard ratio for an exacerbation decreased significantly by 22% in patients treated with NAC and not taking inhaled corticosteroids (p= 0.040) (CitationDecramer et al 2005).
These effects may be explained in part by the protective effect of NAC on viral infections. The effects of NAC on influenza and influenza-like episodes have been studied in 262 patients suffering from nonrespiratory chronic degenerative diseases (CitationDe Flora et al 1997). Compared with placebo, NAC 600 mg twice daily for 6 months significantly decreased both the frequency and severity of influenza-like episodes. Local and systemic symptoms were also significantly reduced in the group receiving NAC. Although the seroconversion towards influenza virus was similar in the two groups, only 25% of the virus-infected subjects treated with NAC developed the symptomatic form of the condition compared with 79% of the placebo group.
Is NAC 600 mg once daily the right dose in patients with COPD?
It may be questioned if NAC 600 mg once daily is the right dose for an optimal effect in patients with COPD. The abovementioned studies on exhaled biomarkers indicate that NAC in a dose of 1200 mg daily is superior in reducing oxidative stress, measured by the concentration of exhaled H2O2. A recent study compared NAC 1200 mg daily, 600 mg daily, and placebo on markers of systemic inflammation and symptoms in patients with COPD GOLD II–III (CitationZuin et al 2005). NAC 1200 mg daily significantly reduced C-reactive protein and IL-8 levels compared with NAC 600 mg daily and placebo. Both dosages were well tolerated.
Another indication that NAC in a higher dose might be more effective is provided by the recent data on the IFIGENIA study in patients with idiopathic pulmonary fibrosis (IPF) (CitationDemedts et al 2005). This was a phase III, double-blind, randomised, placebo-controlled study that assessed the effectiveness over 1 year of high-dose NAC (1800 mg daily) on top of the recommended standard therapy of prednisone–azathioprine in 155 IPF patients. The IPF diagnosis was confirmed by independent histology and radiology expert committees.
This study showed that NAC, on top of prednisone–azathioprine, had a significant and clinically relevant effect on VC and diffusion capacity (DLCO test) at 6 and 12 months. NAC was well tolerated and no differences in side-effects were observed between the two groups.
Conclusions
Oxidative stress is considered to be an important part of the inflammatory response to both environmental and internal signals. Transcription factors like NF-kb and AP-1 are activated by oxidative stress and in turn amplify the inflammatory response to noxious stimuli. In this way, both oxidative stress and inflammation are involved in the complex pathophysiology of COPD, both in terms of pathogenesis and progression of the disease.
In vitro and in vivo data show that NAC protects the lungs against toxic agents by increasing pulmonary defence mechanisms through its direct antioxidant properties and its indirect role as a precursor of GSH synthesis. Indeed, reductions in exhaled biomarkers like H2O2 by NAC have been demonstrated in intervention studies with NAC.
In patients with COPD, treatment with NAC in a dose of 600 mg once daily reduces the risk of exacerbations and improves symptoms compared with placebo. The BRONCUS trial showed that this is especially the case in those COPD patients not using inhaled corticosteroids.
The partial activity of NAC in the BRONCUS trial might be explained by the relatively low dose administered (ie, 600 mg once daily). Data on exhaled biomarkers and markers of systemic inflammation, as well as the recent IFIGENIA study, indicate that higher dosages such as 600 mg twice daily should be administered in patients with COPD.
References
- AbboudRTFeraTRichterA1985Acute effect of smoking on the functional activity of alpha1- protease inhibitor in bronchoalveolar lavage fluidAm Rev Respir Dis13179853871315
- AkaikeTAndoMOdaT1990Dependence on O2− generation by xanthine oxidase of pathogenesis of influenza virus infection in miceJ Clin Invest85739452155924
- AruomaOIHalliwellBHoeyBM1989The antioxidant action of N-acetylcysteine: its reaction with hydrogen peroxide, hydroxyl radical, superoxide, and hypochlorous acidJ Free Radic Biol Med65937
- BarnesPJ2000Chronic obstructive pulmonary diseaseN Engl J Med34342698010911010
- BergstrandHBjörnsonAEklundA1986Stimuli-induced superoxide radical generation in vitro by human alveolar macrophages from smokers: modulation by N-acetylcysteine treatment in vivoJ Free Radic Biol Med2119273029208
- BiagioliMCKaulPSinghI1999The role of oxidative stress in rhinovirus induced elaboration of IL-8 by respiratory epithelial cellsJ Free Radic Biol Med2645462
- BridgemanMMMarsdenMMacNeeW1991Cysteine and glutathione concentrations in plasma and bronchoalveolar lavage fluid after treatment with N-acetylcysteineThorax4639421871695
- BridgemanMMMarsdenMSelbyC1994Effect of N-acetyl cysteine on the concentrations of thiols in plasma, bronchoalveolar lavage fluid, and lung tissueThorax4967058066561
- BridgesRB1985Protective action of thiols on neutrophil functionEur J Respir Dis66Suppl 1394082858401
- BorgstromLKagedalBPaulsenO1986Pharmacokinetics of N-acetylcysteine in manEur J Clin Pharmacol31217223803419
- BorregaardNJensenHSBjerrumOW1987Prevention of tissue damage: inhibition of myeloperoxidase mediated inactivation of alpha 1-proteinase inhibitor by N-acetyl cysteine, glutathione, and methionineAgents Actions22255602833080
- CantinACrystalRG1985Oxidants, antioxidants and the pathogenesis of emphysemaEur J Respir Dis66Suppl 139717
- CantinAMNorthSLHubbardR1987Normal alveolar epithelial lining fluid contains high levels of glutathioneJ Appl Physiol6315273040659
- CorradiMMontuschiPDonnellyLE2001Increased nitrosothiols in exhaled breath condensate in inflammatory airway diseasesAm J Respir Crit Care Med163854811282756
- CotgreaveIA1997N-acetylcysteine: pharmacological considerations and experimental and clinical applicationsAdv Pharmacol38205278895810
- CotgreaveIAEklundALarssonK1987No penetration of orally administered N-acetylcysteine into bronchoalveolar lavage fluidEur J Respir Dis707373817074
- CotgreaveIAMoldeusP1987Lung protection by thiol-containing antioxidantsBull Eur Physiopathol Respir2327573318962
- De BenedettoFAcetoADraganiB2005Long-term treatment oral N-actylcysteine (NAC) reduces exhaled hydrogen peroxide in stable COPDPulm Pharmacol Ther1841715607126
- DecramerMRutten-van MolkenMDekhuijzenPNR2005Effects of N-acetylcysteine on outcomes in chronic obstructive pulmonary diseases (Bronchitis Randomized on NAC Cost-Utility Study, BRONCUS): a randomised placebo-controlled trialLancet36515526015866309
- De FloraSGrassiCCaratiL1997Attenuation of influenza symptomatology and improvement of immunological parameters due to long-term treatment with N-acetylcysteineEur Respir J101535419230243
- DekhuijzenPNR2004Antioxidant properties of N-acetylcysteine: their relevance in relation to chronic obstructive pulmonary diseaseEur Respir J236293615083766
- DekhuijzenPNRAbenKKHDekkerI1996Increased exhalation of hydrogen peroxide in patients with stable and unstable COPDAm J Respir Crit Care Med15481368810624
- DemedtsMBehrJBuhlRIFIGENIA Study Group2005High-dose acetylcysteine in idiopathic pulmonary fibrosisN Engl J Med35322294216306520
- DrostELannanSBridgemanMME1991Lack of effect of N-acetylcysteine on the release of oxygen radicals from neutrophils and alveolar macrophagesEur Respir J472391889500
- EklundAErikssonOHakanssonL1988Oral N-acetylcysteine reduces selected humoral markers of inflammatory cell activity in BAL fluid from healthy smokers: correlation to effects on cellular variablesEur Respir J183282852604
- GhezziPDinarelloCABianchiM1991Hypoxia increases production of interleukin-1 and tumor necrosis factor by human mono-nuclear cellsCytokine3189941883957
- GrandjeanEMBerthetPRuffmannR2000Efficacy of oral long-term N-acetylcysteine in chronic bronchopulmonary disease: a meta-analysis of published double-blind, placebo-controlled clinical trialsClin Ther222092110743980
- JankowskaRPassowicz MuszynskaEMedralaW1993The influence of n-acetylcysteine on chemiluminescence of granulocytes in peripheral blood of patients with chronic bronchitisPneumonol Alergol Pol61586918148757
- JefferyPK2002The pathology of COPD and exacerbationsEur Respir Rev1224
- JefferyPKRogersDFAyersMM1985Effect of oral acetylcysteine on tobacco smoke-induced secretory cell hyperplasiaEur J Respir Dis66Suppl 13911722
- KasielskiMNowakD2001Long-term administration of N-acetylcysteine decreases hydrogen peroxide exhalation in subjects with chronic obstructive pulmonary diseaseRespir Med954485611421501
- KnobilKChoiAMWeigandGW1998Role of oxidants in influenza virus-induced gene expressionAm J Physiol274L134429458811
- LaurentPJanoffAKaganHM1983Cigarette smoke blocks cross-linking of elastin in vitroAm Rev Respir Dis127189926830036
- LindenMWieslanderEEklundA1988Effects of oral N-acetylcysteine on cell content and macrophage function in bronchoalveolar lavage from healthy smokersEur Respir J1645502846345
- LundbäckBLindströmMAnderssonS1992Possible effect of acetylcystein on lung functionEur Respir J5Suppl 15S289
- MacNeeW2000Oxidants/antioxidants and COPDChest117Suppl 1S30317
- MacNeeW2001Oxidative stress and lung inflammation in airways diseaseEur J Pharmacol42919520711698041
- MacNeeWRahmanI1999Oxidants and antioxidants as therapeutic targets in chronic obstructive pulmonary diseaseAm J Respir Crit Care Med160S586510556172
- MacNeeWWiggsBBelzbergAS1989The effect of cigarette smoking on neutrophil kinetics in human lungsN Engl J Med32192482779614
- MateosFBrockJHPerez-ArellanoJL1998Iron metabolism in the lower respiratory tractThorax535946009797761
- MaranzanaAMehlhornRJ1998Loss of glutathione, ascorbate recycling, and free radical scavenging in human erythrocytes exposed to filtered cigarette smokeArch Biochem Biophys350169829473290
- MaruiNOffermannMKSwerlickR1993Vascular cell adhesion molecule-1 (VCAM-1) gene transcription and expression are regulated through an antioxidant-sensitive mechanism in human vascular endothelial cellsJ Clin Invest921866747691889
- MetinkoAPKunkelSLStandifordTJ1992Anoxia-hyperoxia induces monocyte-derived interleukin-8J Clin Invest9079181522234
- MoldeusPBerggrenMGraffströmR1985N-acetylcysteine protection against the toxicity of cigarette smoke and cigarette smoke condensates in various tissues and cells in vitroEur J Respir Dis66Suppl 1391239
- MoldeusPCotgreaveIABerggrenM1986Lung protection by a thiol-containing antioxidant: N-acetylcysteineRespiration5031423809741
- MontuschiPCollinsJVCiabattoniG2000Exhaled 8-isoprostane as an in vivo biomarker of lung oxidative stress in patients with COPD and healthy smokersAm J Respir Crit Care Med1621175710988150
- MorrisonDRahmanILannanS1999Epithelial permeability, inflammation, and oxidant stress in the air spaces of smokersAm J Respir Crit Care Med15947399927360
- NowakDKasielskiMAntczakA1999Increased content of thiobarbituric acid-reactive substances and hydrogen peroxide in the expired breath condensate of patients with stable chronic obstructive pulmonary disease: no significant effect of cigarette smokingRespir Med933899610464820
- PauwelsRABuistASCalverleyPMAon behalf of the GOLD Scientific Committee2001Global strategy for the diagnosis, management, and prevention of chronic obstructive pulmonary disease: NHLBI Global Initiative for Chronic Obstructive Lung Disease (GOLD) Workshop SummaryAm J Respir Crit Care Med16312567611316667
- PoolePJBlackPN2001Oral mucolytic drugs for exacerbations of chronic obstructive pulmonary disease: systematic reviewBr Med J3221271411375228
- RahmanIMacNeeW1996Role of oxidants/antioxidants in smoking-induced lung diseasesFree Radic Biol Med21669818891669
- RahmanIMacNeeW1999Lung glutathione and oxidative stress: implications in cigarette smoke-induced airway diseaseAm J Physiol277L10678810600876
- RahmanIMacNeeW2000Oxidative stress and regulation of glutathione in lung inflammationEur Respir J165345411028671
- RahmanIMorrisonDDonaldsonK1996Systemic oxidative stress in asthma, COPD, and smokersAm J Respir Crit Care Med1541055608887607
- RahmanISkwarskaEMacNeeW1997Attenuation of oxidant/antioxidant imbalance during treatment of exacerbations of chronic obstructive pulmonary diseaseThorax5256589227727
- RepineJELankhorstILMDebackerWA1997Oxidative stress in chronic obstructive pulmonary diseaseAm J Respir Crit Care Med156341579279209
- RodensteinDDeCosterAGazzanigaA1978Pharmacokinetics of oral acetylcysteine: absorption, binding and metabolism in patients with respiratory disordersClin Pharmacokinet324754657688
- RiiseGCQvarfordtILarssonS2000Inhibitory effect of N-acetylcysteine on adherence of Streptococcus pneumoniae and Haemophilus influenzae to human oropharyngeal epithelial cells in vitroRespiration67552811070462
- RubioMLSanchez-CifuentesMVOrtegaM2000N-acetylcysteine prevents cigarette smoke induced small airways alterations in ratsEur Respir J155051110759444
- SadowskaAMvan OverveldFJGoreckaD2005The interrelationship between markers of inflammation and oxidative stress in chronic obstructive pulmonary disease: modulation by inhaled steroids and antioxidantRespir Med99241915715193
- SchleissMBHolzOBehnkeM2000The concentration of hydrogen peroxide in exhaled air depends on expiratory flow rateEur Respir J161115811292115
- SchreckRAlbermannKBaeuerlePA1992Nuclear factor kappa B: an oxidative stress-responsive transcription factor of eukaryotic cells (a review)Free Radic Res Commun17221371473734
- SheffnerALMedlerEMBaileyKR1966Metabolic studies with acetylcysteineBiochem Pharmacol151523355971807
- SteyCSteurerJBachmannS2000The effect of oral N-acetylcysteine in chronic bronchitis: a quantitative systematic reviewEur Respir J162536210968500
- StreightoffFRedmanCEDeLongDC1996In vivo antiviral chemotherapy. II. Anti-influenza action of compounds affecting mucous secretionsAntimicrobial Agents Chemother65038
- VoisinCAertsCWallaertB1987Prevention of in vitro oxidant-mediated alveolar macrophage injury by cellular glutathione and precursorsBull Eur Physiopathol Respir23309132825864
- WarrenJSJohnsonKJWardPACrystalRGWestJBWeibelER1997Consequences of oxidant injuryThe lung: scientific foundations2nd edNew YorkRaven Press, Ltd227988
- ZuinRPalamideseANegrinR2005High-dose N-acetylcysteine in patients with exacerbations of chronic obstructive pulmonary diseaseClin Drug Invest254018