Abstract
Fundamental physical properties, such as the intrinsic recoil of the lung, are governed by the extracellular matrix. The prototypical roles of the matrix proteins, collagen and elastin, in pulmonary fibrosis and emphysema have long been recognized, and much research effort has been devoted to understanding mechanisms of extracellular matrix synthesis and turnover in the lung. Yet, despite extensive knowledge of the biochemical properties of collagen and elastin, none of the present clinical strategies for treating COPD directly target the extracellular matrix. From a matrix perspective, therapeutic interventions that limit elastic fiber destruction and/or restore function to damaged alveolar units merit particular consideration as clinical strategies for treating the emphysema component of COPD. Effective treatment of the bronchiolar component of COPD requires a better understanding of the relationship between airway fibrosis and airflow obstruction. Translating basic knowledge of extracellular matrix biology into the clinical venue will be essential in the development of new approaches to COPD treatment.
Introduction
The extracellular matrix is assembled into distinct three dimensional structures that play a key role in determining the physical and mechanical properties of an organ. In the lung, extracellular matrix organization is highly specialized to facilitate gas exchange. Lung extracellular matrix is partitioned into cartilage, provisional matrix, basement membrane, and interstitium. A brief discussion of the compartmentalization of lung extracellular matrix is found in the first part of this review. The extracellular matrix is composed of discrete gene products with specific functions. The basic biology of collagens and elastic fibers, matrix components that are fundamental to lung structure and function, is summarized in the next section. The review concludes with a description of COPD pathology that is associated with the extracellular matrix and a perspective on therapeutic strategies for COPD based on matrix-related pathology.
Extracellular matrix compartments in the lung
Cartilage
The walls of the trachea and large bronchi are lined by cartilage. Collagen fibers located in the outer layers of cartilage oppose tensile forces and proteoglycans in the central zone resist compression forces on the airways so that the changes in intrathoracic pressure that occur during breathing do not effect airway collapse (CitationRoberts et al 1998).
Provisional matrix
Provisional matrices are assembled during development or wound repair and are degraded when normal tissue architecture is achieved. The most common provisional matrix components are fibronectin and fibrin. Thrombospondin, tenascin, and SPARC may also form provisional matrix in the lung.
Basement membranes
Basement membranes are found wherever parenchymal cells juxtapose connective tissue (). Basement membranes provide physical support for an organ and also regulate macromolecular diffusion. In the lung, the type I alveolar epithelial and capillary endothelial basement membranes are fused thus providing a minimal barrier for gas diffusion. At the biochemical level, specific basement membrane components can modulate cell phenotype through direct interactions with cell surface receptors. Integrins are the best characterized cell surface receptors for matrix proteins and mediate both mechanical and chemical signals which activate a variety of intracellular signaling pathways to affect cell proliferation, survival, and differentiation (for review see CitationHynes 2002; CitationDanen and Sonnenberg 2003; CitationHynes 2004). Airway branching and alveolar epithelial cell localization are influenced by the interactions of basement membrane components such as type IV collagen, laminin, fibronectin, and proteoglycans with cell surface receptors.
Figure 1 Basement membrane organization. Epithelial cells are linked to connective tissue via a network of matrix proteins. Laminin 5 connects integrins on the basal surface of epithelial cells to the type IV collagen network in the lamina densa of the basement membrane. Anchoring fibrils composed of type VII and type XV collagen link the basement membrane to the interstitial matrix where type I collagen, type III collagen and elastic fibers are found. Integrins located on the fibroblast cell surface interact with many matrix proteins including type I collagen. Copyright © 2003, 2007. Modified with permission from Dunsmore SE, Chambers RC, Laurent GJ. 2003. Matrix Proteins. Figure 2.1.2. In: Respiratory Medicine, 3rd ed. London. Saunders, p. 83; Dunsmore SE, Laurent GJ. 2007. Lung Connective Tissue. Figure 40.1. In: Chronic Obstructive Pulmonary Disease: A Practical Guide to Management, 1st ed. Oxford. Wiley-Blackwell, p. 467.
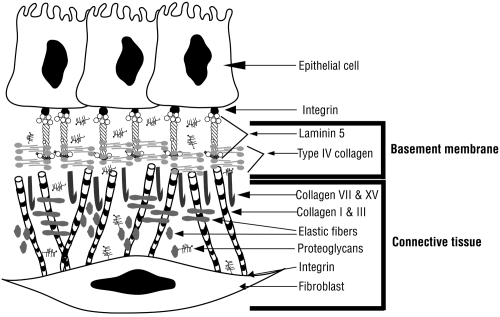
The pulmonary interstitium
The pulmonary interstitium is located between the airspace epithelium and pleural mesothelium. Fibers of the matrix proteins, collagen and elastin, are the major components of the pulmonary interstitium. The primary function of these fibers is to form a mechanical scaffold that maintains structural integrity during ventilation (). It has long been recognized that devastating pathology can result from disruption of pulmonary interstitial matrix homeostasis. Increased extracellular matrix synthesis and deposition, particularly of types I and III collagen, epitomizes pulmonary fibrosis. In fibrotic lungs, gas exchange is adversely affected by the obliteration of capillary beds and decreased regional compliance caused by the thickening of the pulmonary interstitium. Conversely, extracellular matrix degradation is a characteristic of pulmonary emphysema (CitationMandl et al 1977; CitationBarnes 2004). In this disease, ventilation is negatively affected by the decreased intrinsic recoil of the lung that results from proteolytic destruction of elastic fibers (CitationChristie 1934; CitationStead et al 1952; CitationMead et al 1955; CitationCherniack 1956).
Figure 2 Pulmonary interstitium. At the gas exchange interface alveolar capillaries and alveolar type I epithelial cells share a basement membrane. In other portions of the alveolar wall, collagen fibrils and elastic fibers are the major components of the pulmonary interstitium which also contains fibroblasts and proteoglycans. Copyright © 2003, 2007. Modified with permission from Dunsmore SE, Chambers RC, Laurent GJ. 2003. Matrix Proteins. Figure 2.1.1. In: Respiratory Medicine, 3rd ed. London. Saunders, p. 83; Dunsmore SE, Laurent GJ. 2007. Lung Connective Tissue. Figure 40.2. In: Chronic Obstructive Pulmonary Disease: A Practical Guide to Management, 1st ed. Oxford. Wiley-Blackwell, p. 468.
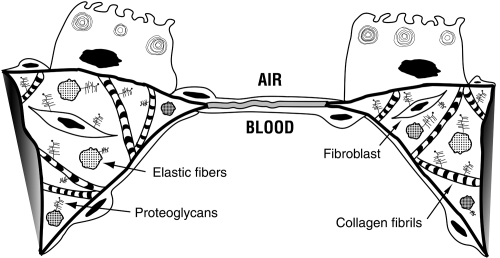
Structural components of the pulmonary interstitium
Collagens
In simple terms, collagens may be thought of as the ‘struts’ of the lung; rod-like structures that limit lung deformation. The rod-like structures are composed of different types of collagen chains all of which contain a characteristic repeating sequence of three amino acids (Gly-x-y). Gly-x-y sequences associate to form triple helices, and by definition, all collagens contain at least one triple helical region (Citationvan der Rest and Garrone 1991). Collagen function is dependent on the formation of supramolecular structures from specific collagen polypeptide chains ().
Figure 3 Collagen supramolecular structures. Copyright © 2005. Modified with permission from Dunsmore SE. 2005. Extracellular Matrix: Collagens. In: Encyclopedia of Respiratory Medicine, 1st ed. London. Academic Press, p. 173; Dunsmore SE, Laurent GJ. 2007. Lung Connective Tissue. Figure 40.3. In: Chronic Obstructive Pulmonary Disease: A Practical Guide to Management, 1st ed. Oxford. Wiley-Blackwell, p. 470.
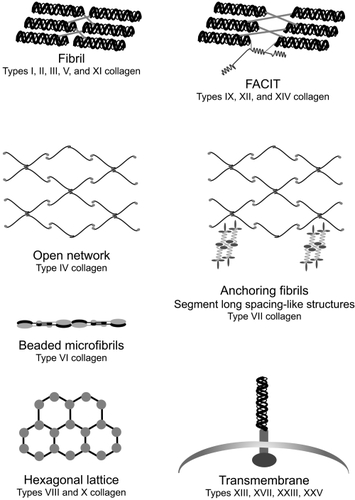
Fibrillar collagens (types I, II, III, V, and XI) are the most abundant proteins in the lung constituting approximately 15% to 20% of the dry weight of the tissue (CitationPierce and Hocott 1960). The primary function of fibrillar collagens is to provide tensile strength to all distensible components of the lung. Types I and III collagen perform this function in the alveolar interstitium, pulmonary blood vessels, visceral pleura, and connective tissue sheaths that surround the tracheobronchial tree. Types II and XI collagen are responsible for the tensile strength of bronchial and tracheal cartilage. Type IV collagen, the predominant component of basement membranes, is the most abundant nonfibrillar collagen in the lung. The amino and carboxy terminal regions of the type IV collagen molecule laterally associate to form open-network structures that confer tensile strength to the blood-gas barrier and prevent stress failure of the pulmonary capillaries under normal conditions (CitationWest and Mathieu-Cosello 1999).
Elastic fibers
Simplistically, elastic fibers are the springs that snap the lung back to resting volume following inflation. The primary component of elastic fibers (CitationKielty et al 2002), elastin (CitationMithieux and Weiss 2005), is composed mainly of hydrophobic amino acids (44%), glycine (33%), and proline (10%–13%). Two pentapeptides Val-Pro-Gly-Val-Gly and Pro-Gly-Val-Gly-Val repeat frequently in the molecule and are thought to form large spiral regions which contribute to the unique distensibility of the protein (CitationLi and Daggett 2002). In the lung, elastic fibers are predominately found in the parenchyma where together with collagen fibrils, an integral fiber network which comprises the architectural skeleton of the lung is formed. Supramolecular assembly of elastic fibers in the lung is distinct. In the walls of the pulmonary artery and arterioles, elastic fibers are organized into concentric sheets or lamellae. Elastic fibers encircle respiratory bronchioles and alveolar ducts in a helical fashion and appear as a fine mesh in alveolar walls.
When appropriately assembled, elastic fibers will last for the entire life span of an organism (CitationShapiro et al 1991). Enzymatic cross-linking of tropelastin lysine residues (CitationBedell-Hogan et al 1993; CitationThomassin et al 2005) and a transglutaminase cross-link between tropoelastin and fibrillin-1 (CitationRock et al 2004) are required for elastic fiber assembly (). Other molecules such as fibulins (CitationNakamura et al 2002; CitationYanagisawa et al 2002; CitationMcLaughlin et al 2006) play important roles in tethering elastic fibers to cells during the assembly process. Elastic fibers cannot be repaired or replaced, thus irreversible pathology will result from elastin degradation. Recent evidence suggests that defects in elastic fiber assembly may enhance susceptibility to proteolysis (CitationKelleher et al 2005).
Figure 4 Elastic fiber assembly. Fiber assembly begins on the surface of elastogenic cells where interactions with integrins are important for microassembly of tropoelastin and fibrillin. The enzymes, lysyl oxidase (LOX) and lysyl oxidase-like 1 (LOXL) begin to catalyze the oxidative deamination of lysine and hydrolysine residues on the tropoelastin globules (

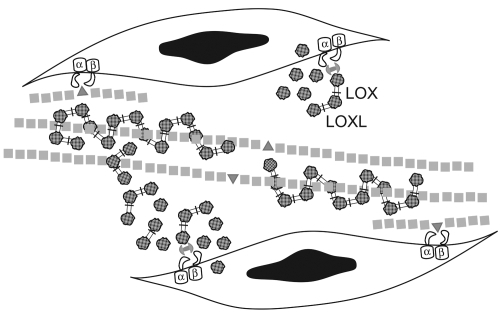
Matrix-related pathology of COPD
Although the etiology of COPD can often be ascribed to a single factor (α1antitrypsin deficiency or exposure to cigarette smoke), the extracellular matrix-related pathology observed in COPD patients is rarely uniform. Airway fibrosis and emphysema are the most obvious pathological changes and are described in further detail below. Cartilage degeneration may accompany airway fibrosis in COPD patients (CitationHaraguchi et al 1999). Marked changes in the airway (CitationCosio et al 1980; CitationJeffery 2001) and alveolar epithelium (CitationOtto-Verberne et al 1991) occur in COPD implying coexisting basement membrane aberrations. The compartmentalization and complexity of the lung extracellular matrix and the nonuniform nature of its response to the intrinsic and extrinsic factors associated with COPD should be taken into account when designing therapeutic strategies for COPD treatment.
Airway fibrosis
Deposition of collagenous and noncollagenous matrix proteins in the airways is part of a larger remodeling process which also includes changes in airway smooth muscle and mucous glands. Historically, the ratio of mucous gland size to bronchial wall thickness (CitationReid 1960) was used to pathologically diagnose chronic bronchitis in the central airways (>4 mm in diameter). Thickening of the bronchial walls due to TGF-β mediated collagen deposition is evident in the central airways of patients with chronic bronchitis (CitationVignola et al 1997), but the symptoms of chronic bronchitis do not appear to be predictive of the rapid decline in forced expiratory volume that occurs in COPD (CitationPeto et al 1983; CitationVestbo and Lange 2002).
Airway obstruction in the smaller conducting airways (<2 mm in diameter) does correlate with the overall severity of COPD (CitationHogg et al 2004). In severe disease (GOLD stage 3 and GOLD stage 4), thickness of all components of the small airway wall (epithelium, lamina propria, smooth muscle, adventitia) is increased (CitationHogg et al 2004). Fibrosis in the small airways is not well characterized. It is assumed that persistent inflammation in the small airways initiates a process of epithelial injury and repair and that fibrosis results from the subepithelial deposition of fibronectin and tenascin and the adventitial accumulation of collagen types I and III. Some modeling studies suggest that extracellular matrix deposition in the airways may be a teleological response to prevent airway collapse (CitationPare 1997). In COPD, however, the increased thickness of the walls of the small airways appears to directly lead to pathological narrowing of the airway lumen (CitationBosken et al 1990).
Emphysema
Emphysema is typically classified as either panacinar or centrilobular. Panacinar emphysema predominates in the lower lung zones and results from α1-antitrypsin deficiency. Uniform destruction of the alveolar walls and permanent enlargement of the alveoli are observed. As the disease progresses, all respiratory airspaces distal to a terminal bronchiole are affected. Centrilobular emphysema predominates in the upper lung zones and begins with inflammation in the terminal and respiratory bronchioles with subsequent enlargement of the alveoli and more distal respiratory airspaces. Centrilobular emphysema is a consequence of prolonged exposure to cigarette smoke.
Therapeutic strategies for COPD
Current COPD treatment regimens are designed to slow progression of the disease. Therapies may be behavioral (smoking cessation), pharmacological (bronchodilators, corticosteroids), replacement (α1-antitrypsin augmentation), or supportive (oxygen supplementation, pulmonary rehabilitation, nutritional support). In severe COPD cases, surgical intervention (lung volume reduction, transplant) may be an option. From a matrix perspective, therapeutic strategies that limit elastic fiber destruction and/or restore function to damaged alveolar units will most likely improve the clinical outcome of COPD. It is unclear if specific treatments for airway fibrosis, which remain to be developed, will prove to be beneficial in the COPD patient. Perspectives on protease inhibition, fibrosis reversal and alveolar regeneration in the context of COPD treatment ensue.
Protease inhibition
Ever since the discovery of the association of α1-antitrypsin deficiency with emphysematous lung disease (CitationLaurell and Ericksson 1963; CitationEricksson 1964), much effort has been focused on the design of neutrophil elastase inhibitors for COPD treatment. Indeed, the elastase-antielastase hypothesis has dominated the field of COPD research for the past 40 years. Many compounds designed to inhibit neutrophil elastase have been tested in animal models and in clinical trials (CitationOhbayashi 2002), but α1-antitrypsin remains the only neutrophil elastase inhibitor used in the routine clinical treatment of COPD. The design of therapeutic strategies based on antiproteases is complicated by the broad spectrum of extracellular and intracellular proteases, many with elastolytic capacity, that are present in the COPD patient (CitationShapiro 2001). Data from animal models (CitationHautamaki et al 1997; CitationZheng et al 2000) indicate that proteases other than neutrophil elastase may be important in elastic fiber destruction and COPD pathogenesis. Though it appears that the protease-antiprotease hypothesis may be more extensive than originally envisioned, effective inhibition of elastolytic proteases will continue to be an attractive strategy for attenuating COPD progression. Inhibition of nonelastolytic proteases may also be an effective strategy for limiting structural damage to the epithelium and basement membrane.
Fibrosis reversal
Since bronchodilators do appear to alleviate some COPD symptoms (CitationWeder and Donohue 2005), discussion of whether treatments that reverse airway fibrosis will be a stable and long-term means of preventing airflow obstruction may be warranted. Bronchial wall thickness and core-rind heterogeneity are the most predictive features of declining pulmonary function in COPD (CitationAziz et al 2005), but it is not known if modulation of bronchial wall thickness will correlate with improved pulmonary function in COPD. Data from computed tomography analyses may be used to develop prognostic algorithms for how changing bronchial wall thickness in an emphysematous lung affects pulmonary function. Animal models in which airway fibrosis can be uncoupled from emphysema in the presence and absence of inflammation are technically feasible and will be available for in vivo proof of concept testing of candidate molecules with fibrosis-reversing properties. Antifibrotic actions of potential therapeutics are likely to be cell type-specific, and potential antifibrotic agents as well as anti-inflammatory compounds already in clinical use should be tested in in vitro models in which the matrix-producing capacity of the structural cells of the airway wall can be assessed. Recent evidence suggests that therapies that are equivalent in reducing inflammation may have dissimilar effects on fibrosis (CitationBurgess et al 2006).
Several pharmacologics designed to inhibit or prevent fibrosis in the lung are in various stages of clinical development (). Most of these compounds have been designed to block the effects of cytokines on fibroblasts so that fibroblast proliferation and excess matrix production can be inhibited. Clinical trials testing the anti-fibrotic efficacy of drugs originally developed to treat other indications have also been conducted (). At the present time, however, the optimal pharmacotherapy for fibrosis reversal in any lung disease remains elusive.
Table 1 Compounds in clinical development for treatment of fibrotic lung disease
Table 2 FDA-approved drugs tested for efficacy in treatment of fibrotic lung disease
Airway fibrosis in COPD is preceded by chronic bronchial inflammation. Thus, anti-inflammatory treatment if administered at the appropriate disease stage could potentially prevent the development of pathologic airway fibrosis in the COPD patient. Furthermore, many current anti-inflammatory therapies may have direct effects on the production of matrix proteins. For instance, glucocorticoids can inhibit the synthesis of collagen (CitationBavetta et al 1962; CitationShull and Cutroneo 1983; CitationOikarinen et al 1988). Phosphodiesterase 4 inhibitors block TGF-β induced collagen production in airway smooth muscle cells (CitationBurgess et al 2006). Animal model data suggest that corticosteroids (CitationMcMillan et al 2005; CitationCho et al 2005; CitationMiller et al 2006) and antileukotrienes (CitationHenderson et al 2006) may be effective at reversing airway fibrosis. Effects of anti-inflammatory therapeutics on airway fibrosis merit consideration as strategies for application of pharmacotherapy to COPD become more specifically adapted to disease stage and genetic background.
Alveolar regeneration
To completely restore normal lung function to the COPD patient, repair and regeneration of damaged alveolar units is necessary. Clinical implementation of regenerative medicine remains a revolutionary and futuristic goal, but data from animal studies is providing evidence of some critical components of this process. The complexity of alveolar development is illustrated by the reports of defective alveogenesis in at least 11 distinct ‘knock-out’ mice (CitationMahadeva and Shapiro 2002; Banerjee et al 2004; CitationChiang et al 2005; CitationMandeville et al 2006). Alveolar enlargement in mice deficient in surfactant protein D (CitationWert et al 2000), TIMP-3 (CitationLeco et al 2001), or the β6 integrin subunit (CitationMorris et al 2003) underscores the importance of these molecules in regulating alveolar homeostasis. Models in which emphysema is induced by apoptosis of alveolar endothelial (CitationKasahara 2000) or epithelial cells (CitationAoshiba et al 2003) highlight the role of parenchymal cell turnover in the maintenance of alveolar structure. Perhaps the most promising finding from animal studies is that in elastase-damaged lungs, alveolar function and architecture can be restored by retinoic acid treatment (CitationMassaro and Massaro 1997). Translation of these findings in animal studies to clinical practice represents one of the most promising and challenging areas of COPD research.
In COPD, the capacity of the type II pneumocyte to proliferate and to restore the alveolar epithelium may be exhausted. Thus, alveolar regeneration might depend on the repopulation of damaged areas of the lung by stem cells. Recent progress indicates that “ethically acceptable” sources of human embryonic stem cells may be available for clinical use (CitationKlimanskaya et al 2006). Understanding the pathways by which embryonic stem cells can be induced to differentiate into type II pneumocytes (CitationAli et al 2002), however, may provide sufficient information to develop either a pharmacological or biological means of stimulating proliferation of epithelial cells in emphysematous lungs.
Hematopoietic stem cells can also serve as progenitors for the alveolar epithelium (CitationKrause et al 2001; CitationKotton et al 2001). Human pulmonary chimerism is observed following bone marrow transplant (CitationSuratt et al 2003; CitationMattsson et al 2004; CitationAlbera et al 2005) and appears to be increased in the setting of chronic injury (CitationKleeberger et al 2003). Although it is likely that proliferation of type II pneumocytes is the predominant mechanism by which alveolar repair occurs (CitationZander et al 2005), hematopoietic stem cell engraftment may be particularly important for repair of areas of the lung in which emphysema has begun to develop. Clinical protocols for mobilizing hematopoietic stem cells are available should the engraftment of bone marrow-derived cells in the emphysematous lung prove beneficial.
Conclusions
Matrix-related pathology underlies many COPD symptoms. Treatments that restore lung function in COPD are needed. Knowledge of extracellular matrix biology can provide a basis for the development of new approaches to COPD treatment.
Acknowledgements
The author would like to thank her former colleagues at Pennsylvania State University, Washington University in St. Louis, the Centre for Respiratory Research at University College London, and the Respiratory Disorders Program at Brigham and Women’s Hospital for providing a solid foundation in extracellular matrix biology, matrix metalloproteinases, pulmonary fibrosis and COPD. This review is based in part on previous work of the author published in: Respiratory Medicine, 3rd ed. London: Saunders; 2003. pp. 82–92; Encyclopedia of Respiratory Medicine, 1st ed. London. Academic Press, 2005. pp. 168–74; and Chronic Obstructive Pulmonary Disease: A Practical Guide to Management, 1st ed. Oxford. Blackwell Publishing, 2007. pp. 467–82. Huw Jones, UCL Media Resources, Illustration, assisted with the preparation of and . The author has no conflicts of interest to declare.
References
- AlberaCPolakJMJanesSRepopulation of human pulmonary epithelium by bone marrow cells: a potential means to promote repairTissue Eng20051111152116144447
- AliNNEdgarAJSamadikuchaksaraeiADerivation of type II alveolar epithelial cells from murine embryonic stem cellsTissue Eng200285415012201994
- AoshibaKYokohoriNNagaiAAlveolar wall apoptosis causes lung destruction and emphysematous changesAm J Respir Cell Mol Biol2003285556212707011
- AzizZAWellsAUDesaiSRFunctional impairment in emphysema: contribution of airway abnormalities and distribution of parenchymal diseaseAJR Am J Roentgenol200518515091516304005
- BarnesPJMediators of chronic obstructive pulmonary diseasePharmacol Rev2004565154815602009
- BavettaLABekhorINimniMEEffects of hormone administration on collagen biosynthesis in the ratProc Soc Exp Biol Med1962110294713865990
- Bedell-HoganDTrackmanPAbramsWOxidation, crosslinking, and insolubilization of recombinant tropoelastin by purified lysyl oxidaseJ Biol Chem199326810345508098038
- BoskenCHWiggsBRParePDSmall airway dimensions in smokers with obstruction to airflowAm Rev Respir Dis1990142563702389908
- BurgessJKOliverBGPonirisMHA phosphodiesterase 4 inhibitor inhibits matrix protein deposition in airways in vitroJ Allergy Clin Immunol20061186495716950284
- CherniackRMThe physical properties of the lung in chronic obstructive pulmonary emphysemaJ Clin Invest19563539440413306781
- ChiangMKLiaoYCKuwabaraYInactivation of tensin3 in mice results in growth retardation and postnatal lethalityDev Biol20052793687715733665
- ChoJYMillerMMcElwainKCombination of corticosteroid therapy and allergen avoidance reverses allergen-induced airway remodeling in miceJ Allergy Clin Immunol200511611162216275385
- ChristieRVThe elastic properties of the emphysematous lung and their clinical significanceJ Clin Invest19341329532116694212
- CosioMGHaleKANiewoehnerDEMorphologic and morphometric effects of prolonged cigarette smoking on the small airwaysAm Rev Respir Dis1980122265717416603
- DanenEHSonnenbergAIntegrins in regulation of tissue development and functionJ Pathol20032004718012845614
- DunsmoreSELaurentGShapiroSCollagensEncyclopedia of Respiratory Medicine20061LondonAcad Pr16874
- DunsmoreSEChambersRCLaurentGJGibsonJGeddesDCostabelUMatrix proteinsRespiratory Medicine20033LondonSaunders8292
- DunsmoreSELaurentGJStockleyRARennardSIRabeKLung connective tissueChronic Obstructive Pulmonary Disease: A Practical Guide to Management20071OxfordWiley-Blackwell46782
- ErikssonSPulmonary emphysema and alpha1-antitrypsin deficiencyActa Med Scand196417519720514124635
- HaraguchiMShimuraSShiratoKMorphometric analysis of bronchial cartilage in chronic obstructive pulmonary disease and bronchial asthmaAm J Respir Crit Care Med199915910051310051285
- HautamakiRDKobayashiDKSeniorRMRequirement for macrophage elastase for cigarette smoke-induced emphysema in miceScience1997277200249302297
- HendersonWRJrChiangGKTienYTReversal of allergeninduced airway remodeling by CysLT1 receptor blockadeAm J Resp Crit Care Med20061737182816387808
- HoggJCChuFUtokaparchSThe nature of small-airway obstruction in chronic obstructive pulmonary diseaseN Engl J Med200435026455315215480
- HynesROIntegrins: bidirectional, allosteric signaling machinesCell20021106738712297042
- HynesROThe emergence of integrins: a personal and historical perspectiveMatrix Biol2004233334015533754
- JefferyPKRemodeling in asthma and chronic obstructive lung diseaseAm J Respir Crit Care Med2001164S28S3811734464
- KasaharaYTuderRMTaraseviciene-StewartLInhibition of VEGF receptors causes lung cell apoptosis and emphysemaJ Clin Invest200010613111911104784
- KelleherCMSilvermanEKBroekelmannTA functional mutation in the terminal exon of elastin in severe, early-onset chronic obstructive pulmonary diseaseAm J Respir Cell Mol Biol2005333556216081882
- KieltyCMSherrattMJShuttleworthCAElastic fibresJ Cell Sc200211528172812082143
- KleebergerWVersmoldARothamelTIncreased chimerism of bronchial and alveolar epithelium in human lung allografts undergoing chronic injuryAm J Pathol200216214879412707031
- KlimanskayaIChungYBeckerSHuman embryonic stem cell lines derived from single blastomeresNature2006444481516929302
- KottonDNMaBYCardosoWVBone marrow-derived cells as progenitors of lung alveolar epitheliumDevelopment200112851818811748153
- KrauseDSTheiseNDCollectorMIMulti-organ, multilineage engraftment by a single bone marrow-derived stem cellCell20011053697711348593
- LaurellC-BErikssonSThe electrophoretic α1-globulin pattern of serum in α1 antitrypsin deficiencyScandinav J Clin and Lab Investigation19631513240
- LecoKJWaterhousePSanchezOHSpontaneous air space enlargement in the lungs of mice lacking tissue inhibitor of metalloproteinases-3(TIMP-3)J Clin Invest20011088172911560951
- LiBDaggettVMolecular basis for the extensibility of elastinJ Muscle Res Cell Motil2002235617312785105
- MahadevaRShapiroSDChronic obstructive pulmonary disease * 3: Experimental animal models of pulmonary emphysemaThorax2002579081412324680
- MandevilleIAubinJLeBlancMImpact of the loss of Hoxa5 function on lung alveogenesisAm J Pathol200616913122717003488
- MandlIDarnuleTVFiererJAElastin degradation in human and experimental emphysemaAdv Exp Med Biol19777922131868637
- MassaroGDMassaroDRetinoic acid treatment abrogates elastaseinduced pulmonary emphysema in ratsNat Med19973675779176496
- MattssonJJanssonMWernersonALung epithelial cells and type II pneumocytes of donor origin after allogeneic hematopoietic stem cell transplantationTransplantation200478154715257055
- McLaughlinPJChenQHoriguchiMTargeted disruption of fibulin-4 abolishes elastogenesis and causes perinatal lethality in miceMol Cell Biol2006261700916478991
- McMillanSJXanthouGLloydCMTherapeutic administration of budesonide ameliorates allergen-induced airway remodelingClin Exp Allergy2005353889615784120
- MeadJLindgrenIGaenslerEAThe mechanical properties of the lungs in emphysemaJ Clin Invest19553410051614392216
- MillerMChoJYMcElwainKCorticosteroids prevent myofibroblast accumulation and airway remodeling in miceAm J Lung Cell Mol Physiol2006290L1629
- MithieuxSMWeissASElastinAdv Protein Chem2005704376115837523
- MorrisDGHuangXKaminskiNLoss of integrin alpha(v)beta6-mediated TGF-beta activation causes MMP12-dependent emphysemaNature20034221697312634787
- NakamuraTLozanoPRIkedaYFibulin-5/DANCE is essential for elastogenesis in vivoNature2002415171511805835
- OhbayashiHNeutrophil elastase inhibitors as treatment for COPDExpert Opin Investig Drugs20021196580
- OikarinenAIVuorioEIZaragozaEJModulation of collagen metabolism by glucocorticoids. Receptor-mediated effects of dexamethasone on collagen biosynthesis in chick embryo fibroblasts and chondrocytesBiochem Pharmacol1988371451623358778
- Otto-VerberneCJTen Have-OpbroekAAWillemsLNLack of type II cells and emphysema in human lungsEur Respir J19914316231864346
- PareODRobertsCRBaiTRThe functional consequences of airway remodeling in asthmaMonaldi Arch Chest Dis199752589969550873
- PetoRSpeizerFECochraneALThe relevance in adults of airflow obstruction, but not of mucous hypersecretion, to mortality from chronic lung diseaseAm Rev Respir Dis19831284915006614643
- PierceJAHocottJBStudies on the collagen and elastin content of the human lungJ Clin Invest19603981414432825
- ReidLMeasurement of the bronchial mucous gland layer: a diagnostic yardstick in chronic bronchitisThorax1960151324114437095
- RobertsCRRainsJKParePDUltrastructure and tensile properties of human tracheal cartilageJ Biomech1998318169596542
- RockMJCainSAFreemanLJMolecular basis of elastic fiber formation. Critical interactions and a tropoelastin-fibrillin-1 cross-linkJ Biol Chem2004279237485815039439
- ShapiroSDEndicottSKProvinceMAMarked longevity of human lung parenchymal elastic fibers deduced from prevalence of D-aspartate and nuclear weapons-related radiocarbonJ Clin Invest1991871828342022748
- ShapiroSDProteinases in chronic obstructive pulmonary diseaseBiochem Soc Trans2001309810212023833
- ShullSCutroneoKRGlucocorticoids coordinately regulate procollagens type I and type III synthesisJ Biol Chem1983258336496826565
- SteadWWFryDLEbertRVThe elastic properties of the lung in normal men and in patients with chronic emphysemaJ Lab Clin Med1952406748112990894
- SurattBTCoolCDSerlsAEHuman pulmonary chimerism after hematopoietic stem cell transplantationAm J Respir Crit Care Med20031683182212724127
- ThomassinLWerneckCCBroekelmannTJThe Pro-regions of lysyl oxidase and lysyl oxidase-like 1 are required for deposition onto elastic fibersJ Biol Chem2005280428485516251195
- van der RestMGarroneRCollagen family of proteinsFASEB J199152814231916105
- VestboJLangePCan GOLD Stage 0 provide information of prognostic value in chronic obstructive pulmonary disease?Am J Respir Crit Care Med2002663293212153965
- VignolaAMChanezPChiapparaGTransforming growth factor-beta expression in mucosal biopsies in asthma and chronic bronchitisAm J Respir Crit Care Med1997156591999279245
- WederMMDonohueJFRole of bronchodilators in chronic obstructive pulmonary diseaseSemin Respir Crit Care Med2005262213416088439
- WertSEYoshidaMLeVineAMIncreased metalloproteinase activity, oxidant production, and emphysema in surfactant protein D gene-inactivated miceProc Natl Acad Sci USA20009759727710801980
- WestJBMathieu-CostelloOStructure, strength, failure, and remodeling of the pulmonary blood-gas barrierAnnu Rev Physiol1999615437210099701
- YanagisawaHDavisECStarcherBCFibulin-5 is an elastinbinding protein essential for elastic fibre development in vivoNature20024151687111805834
- ZanderDSBazMACogleCRBone marrow-derived stem-cell repopulation contributes minimally to the Type II pneumocyte pool in transplanted human lungsTransplantation2005802061216041265
- ZhengTZhuZWangZInducible targeting of IL-13 to the adult lung causes matrix metalloproteinase- and cathepsin-dependent emphysemaJ Clin Invest200010610819311067861