Abstract
This study investigated the possibility of simultaneously extracting glucose and lactate from human subjects, at the same skin location, using transdermal reverse iontophoresis. Transdermal monitoring using iontophoresis is made possible by the skin’s permeability to small molecules and the nanoporous and microporous nature of the structure of skin. The study was intended to provide information which could be used to develop a full, biosensor-based, monitoring system for multiple parameters from transdermal extraction. As a precursor to the human study, in vitro reverse iontophoresis experiments were performed in an artificial skin system to establish the optimum current waveforms to be applied during iontophoresis. In the human study, a bipolar DC current waveform (with reversal of the electrode current direction every 15 minutes) was applied to ten healthy volunteers via skin electrodes and utilized for simultaneous glucose and lactate transdermal extraction at an applied current density of 300 μA/cm2. Glucose and lactate were successfully extracted through each subject’s skin into the conducting gel that formed part of each iontophoresis electrode. The results suggest that it will be possible to noninvasively and simultaneously monitor glucose and lactate levels in patients using this approach and this could have future applications in diagnostic monitoring for a variety of medical conditions.
Introduction
Epidemiological studies have shown that the prevalence of diabetes is steadily increasing and is a widespread problem in modern society (CitationKing et al 1993; CitationAmos et al 1997; CitationWild et al 2004). The number of cases of diabetes worldwide in 2000 among adults ≥20 years of age was estimated to be 171 million (CitationWild et al 2004) and in 2003 this was estimated to be 194 million (CitationIDF 2003). By 2025, the number of people with diabetes is expected to exceed 333 million.
Diabetes increases the risk of ill health and shortens life. It is the leading cause of blindness and visual impairment (CitationEvans 1995), amputation (CitationDavis et al 2004), cardiovascular disease (CitationLaing et al 1999a, Citation1999b), stroke (CitationBell 1994; CitationStephenson et al 1995) and kidney failure (CitationWang et al 1996; CitationBrancati et al 1997).
Tight monitoring and control of blood glucose levels can reduce the prevalence of complications in both Type 1 and Type 2 diabetes (CitationUK Prospective Diabetes Study Group, 1998a,Citation1998b, Citation1998c, Citation1998d). To date, most Type 1 and Type 2 diabetic patients measure their own blood glucose several times a day by obtaining finger-prick capillary samples and applying the blood to a reagent strip for analysis in a portable meter (CitationPickup 2003). However, this method is painful, cumbersome, aesthetically unpleasant and inconvenient and blood glucose monitoring cannot continue during sleep. This provides considerable impetus for the development of noninvasive methods for continuous blood glucose monitoring.
Skin provides a unique gateway for noninvasive, transdermal monitoring. Recently, reverse iontophoresis techniques have been used for patient monitoring (CitationRao et al 1995; CitationTamada et al 1999; CitationPitzer et al 2001; CitationTierney et al 2001; CitationPotts et al 2002; CitationDegim et al 2003) and noninvasive diagnosis (CitationNumajiri et al 1993; CitationMize et al 1997; CitationMerino et al 1999), most notably the GlucoWatch® biographer which proposed a solution for diabetics which avoided the frequent need for blood sampling (CitationTamada et al 1999). Reverse iontophoresis refers to the passage of a low level of current through the skin, normally via a pair of skin electrodes containing a conducting gel, to promote the extraction of both charged and neutral molecules. The major mechanisms for transport of ions and molecules are; the electromigration of charged species to the electrode of opposite polarity, electroosmosis of neutral molecules to the cathode or anode, or a combination of these two processes. The most successful reverse iontophoresis application for patient monitoring to date, as mentioned, has been in noninvasive and continuous blood glucose detection utilising the GlucoWatch®. The GlucoWatch® biographer utilized the techniques of reverse iontophoresis and biosensing to noninvasively and continuously monitor blood glucose. However, the GlucoWatch® had some problems, for example it was reported that it does not reliably detect hypoglycemia in children (CitationThe Diabetes Research in Children Network 2004). In addition there have been no further developments of commercial systems to monitor any other blood analytes transdermally. Consequently there is room for further study, understanding and development of transdermal reverse iontophoresis for noninvasive patient monitoring.
The present study investigated the possibility that other blood parameters could be monitored simultaneously with glucose through the skin utilizing a single device on a patient. Lactate was selected as a parameter of interest for a variety of clinical conditions. Glucose and lactate levels are metabolically linked and therefore are indicative of the healthy functioning of an organism or tissue section when monitored simultaneously
Monitoring of lactate is known to be beneficial to the critical care patient as the trend in lactate levels is an indicator of patient viability (CitationAbramson et al 1993). Blood lactate levels have been widely used for prognosis of critical illness and assessing effects of therapeutic maneuvers (CitationCairns et al 1997; CitationVincent 1998; CitationMooney et al 1999). Blood lactate is an extremely fast indicator of oxygen deprivation at the cellular level, increasing within seconds of a life threatening event and decreasing within seconds of appropriate therapy (CitationBakker et al 1991). Blood lactate has also been reported to be superior to other variables (eg, oxygen-derived variables) as a predictor of outcome (CitationMooney et al 1999). In critical care units, high lactate levels in the general circulation have a strong prognostic value (CitationVincent 1996), with lactate levels over 5 mM (normally 0.6–2.4 mM) associated with a poor outcome. Therefore, it is possible to predict organ failure from serial lactate measurements (CitationGersh and Anderson 1993). Lactate monitoring has been suggested as valuable for many patients in emergency medicine: for surgical patients (CitationElia et al 1991; CitationHildingsson et al 1996; CitationCarrier et al 1998; CitationHabicht et al 1998), for trauma patients (CitationSlomovitz 1998), for patients with head injury or cerebral ischemia (CitationValadka 1998; CitationMenzel et al 1999), acute intestinal ischemia (CitationMurray et al 1994), liver ischemia (CitationRasmussen et al 1994), transplanted organ surveillance eg, myocutaneous flaps (CitationRojdmark et al 1998), intrapartum for the fetus (CitationWestgren et al 1999), and patients with septic shock (CitationBoer et al 1994).
Clinically, a patient’s blood lactate level can be tracked in a minimally-invasive manner by microdialysis (Volpe et al 1994) or through finger-stick sampling. Although the microdialysis sampling method, when combined with a biosensor or other analysis technique, can provide continuous lactate monitoring it still has a chance of inducing infection on the insertion site and surgical intervention is required for this technique. The microdialysis tubing generally needs replacement after a 48 hour period. On the other hand, the finger-stick method cannot provide continuous lactate monitoring.
The ability to monitor both glucose and lactate simultaneously and noninvasively would have applications in many of the clinical conditions described above. In addition this measurement technique could be applied to monitor the viability of skin grafts post operatively and to assess the vascular condition of skin and limbs.
The use of reverse iontophoresis in patient monitoring does present some concerns. For example, use of constant direct current (DC) during transdermal reverse iontophoresis may localise and generate hydroxyl ions in the anodal skin region and hydrogen ions in the cathodal skin region. This process, which disrupts local pH values, leads to stinging and erythrema (CitationHoward et al 1995). The use of pulsed DC, (a constant DC delivered periodically), has been suggested to prevent charge build up on the skin (polarization) and minimize pH changes (CitationChien et al 1987; CitationChien et al 1990). Polarization effects turn the skin into what is effectively a charged capacitor and this reduces the flow of current and charged species during iontophoresis. When current is switched off the skin naturally depolarizes and returns to its near initial electrical condition. The use of bipolar current, a constant DC source where direction of current flow through the skin electrodes is changed periodically, has also been suggested as a means to overcome skin polarisation and minimize pH changes (CitationHoward et al 1995; CitationTomohira et al 1997). CitationTomohira and colleagues (1997) used an in vivo rat abdominal skin model to study the effect of electrode polarity switching (ie, a bipolar DC current profile with the polarity of the electrodes switched over at specific intervals) in the iontophoresis of insulin and calcitonin for transdermal delivery. They found that applied bipolar DC current profiles could enhance the absorption of the insulin and calcitonin. CitationHirvonen and colleagues (1995) used a diffusion cell with mouse skin to study the impact of different applied current profiles (waveforms) in regulating the permeation of two charged amino acids, lysine and glutamic acid. They found that bipolar current waveforms or constant DC current waveforms resulted in comparable transport rates which are both higher than that of pulsed DC current waveforms. Bipolar current waveforms were selected for investigation in this study.
The aims of this study were; (1)to establish the optimum applied current waveforms for the transdermal reverse iontophoresis of both glucose and lactate and (2) to study the simultaneous reverse iontophoretic extraction of glucose and lactate across human skin barriers in healthy human subjects.
The effects of applied current waveforms on the reverse iontophoresis of glucose and lactate were initially investigated using in vitro diffusion cells designed to mimic human skin conditions. These were utilized with an artificial membrane as a skin substitute, avoiding the sample to sample variation found in human skin whilst allowing the study of how the electrical waveform affects the transport of glucose and lactate (CitationConnolly et al 2002). The optimum applied current waveform found from these experiments was then applied to healthy human volunteers, and glucose and lactate were extracted simultaneously from the subjects’ skin using a specially-designed collection electrode.
Materials and methods
Constant current source
A fully programmable, portable constant current source, designed and developed by the authors for iontophoresis applications (CitationChing et al 2005), was used to deliver iontophoretic current for both in vitro and human reverse iontophoresis experiments. The device is capable of delivering constant DC current, bipolar DC current waveforms and DC current pulses according to how it is programmed.
In vitro reverse iontophoresis experiments
A HEPES buffer solution was used for in vitro experiments. N-2-hydroxyethylpiperazine-N’-2-ethanesulphonic acid (HEPES), sodium chloride (NaCl), sodium hydroxide (NaOH), hydrochloric acid (HCl) and lactate were purchased from Sigma Chemical Co. (St. Louis, MO). Glucose was purchased from BDH Limited (Poole, England). Lactate reagents (LC 2389) and glucose reagents (GL 26233) were purchased from Randox Laboratories Limited (Antrim, UK). De-ionized water (resistivity ≥18M Ωcm) that had been purified by a Millipore System (Milli-Q UFplus; Bedford, MA) was used to prepare all solutions. Nanoporous membrane with a net negative charge at pH 7 was used as the artificial skin model (Spectra/Por® cellulose ester dialysis membrane MWCO: 500, Spectrum Laboratories, Inc., Canada) in accordance with earlier studies of the iontophoresis model (CitationConnolly et al 2002).
Silver-silver chloride (Ag/AgCl) electrodes were prepared by chloriding silver wire (1mm diameter, 25 mm length, 99.99% pure; Aldrich Chemical Company Inc., Milwaukee, WI) immersed in 0.1M HCl solution (Pt-cathode) for 90 minutes at an applied current of 314 μA.
All in vitro experiments were performed using model diffusion cells designed for iontophoresis experiments (CitationConnolly et al 2002), in which both electrode chambers were located on the same surface side of a nanoporous membrane as shown in . The electrode chambers were filled with 350 μl of 25 mM, pH 7.4, HEPES buffer containing 133 mM NaCl. The lower chamber of the diffusion cell contained an electrolyte solution comprising 133 mM NaCl, buffered to pH 7.4 with 25 mM HEPES, and either 5 mM glucose or 10 mM lactate. Each electrode chamber contained a Ag/AgCl electrode. The surface area of the nanoporous membrane exposed to the electrode in each chamber was 0.2 cm2 and the electrode chambers were 11 mm apart. The device was operated at ambient temperature (21–23 °C).
Figure 1 Schematic illustration of the model diffusion cell for in vitro reverse iontophoresis experiments. For the control experiment, the experimental setting was identical except no current flowed through the Ag/AgCl electrodes.
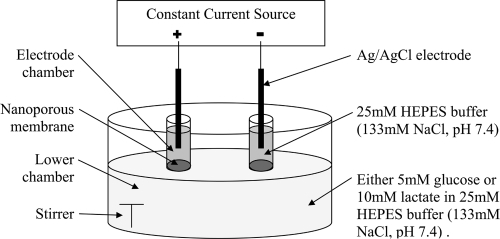
An iontophoretic current of 0.3 mA/cm2 was used for the various applied current waveforms tested in vitro in the cells and each experimental current condition was sustained for 60 minutes with current supplied through the Ag/AgCl wire electrodes.
The applied current waveforms tested in this study were: (i) constant DC current for the entire duration of current flow, (ii) DC current with the polarity of the electrode pair reversed every 5 minutes during the 60 minutes of the current flow, (iii) DC current with the polarity of the electrode pair reversed every 10 minutes during the 60 minutes of the current flow, (iv) DC current with the polarity of the electrode pair reversed every 15 minutes during the 60 minutes of the current flow.
Each applied current waveform was tested in the model iontophoresis cell and the entire contents of the electrode chambers were removed at the end of each experimental procedure and analyzed to quantify the amount of glucose or lactate extracted through the nanoporous membrane. For the control experiments, all the experimental arrangements and procedures were the same as those described for the reverse iontophoresis experiments except that no current was applied in the control experiments, allowing diffusion of lactate and glucose through the nanoporous membrane to be observed in the collection chambers.
A colorimetric clinical chemistry assay method, was adapted for use with a microplate reader (Multiskan Ascent®, Labsystems Oy, Finland), and was used to quantify the amount of glucose (determined by Glucose reagents, GL 26233, Randox Laboratories Limited, UK) or lactate (determined by Lactate reagents, LC 2389, Randox Laboratories Limited, UK) extracted through the nanoporous membrane. For the test, 150 μl of each extracted sample was mixed with 150 μl of the corresponding Glucose/Lactate reagents and dispensed as a sample into a 96-well microplate (Multiskan Ascent, Labsystems Oy, Finland). When loaded with samples the microplate was then immediately placed on the microplate reader. Each plate was incubated for 90 minutes at 37 °C in the microplate reader and absorbance read at either 500 nm (for glucose) or 550 nm (for lactate). Excellent linear relationships (r2 > 0.99 for both cases) between lactate/glucose standard solutions and their relative absorbance were found in the calibration curves for these assays, allowing the lactate/glucose concentrations from the model cells to be calculated by linear regression.
Human reverse iontophoresis experiments
Sodium phosphate monobasic (USP grade) and NaOH were purchased from Sigma-Aldrich. Methylcellulose (MC), Methocel A4M Prem, from the Dow Chemical Company was used to form the electrode contact gels. 0.1 M phosphate buffer solution (PBS) was prepared by dissolving 1.1998 g of sodium phosphate monobasic in 100 ml de-ionized water and adjusting the pH to 7.4 using a 40% w/v solution of NaOH. A 4% w/v MC gel was prepared by mixing 4g of MC with 100 ml of 0.1M PBS. De-ionized water (resistivity ≥ 18M Ωcm) that had been purified by a Millipore System (Milli-Q UFplus; Bedford, MA) was used to prepare all solutions.
Circular (11.3 mm diameter) screen-printed Ag/AgCl electrodes (SPE) were fabricated by printing ink through a screen pattern to a thin polyvinyl chloride sheet. Silver paste and Silver-silver chloride (Ag/AgCl) paste for printing the electrodes was purchased from Advanced Conductive Materials (Atascadero, CA). Each SPE was coated with 90 μl of the MC (4%) gel described above within a silicone ring. The MC (4%) gel acts as a conductive media for the transmission of iontophoretic current from the SPE to the human skin and also acts as a collector of the extracted lactate and glucose. The prepared electrodes were used in pairs on the human subjects. A prepared electrode ready for human use is shown in .
Figure 2 The screen-printed electrodes. (a) The front view, with methylcellulose gel (4%) pipetted to this side. (b) The back view, with circular window for crocodile clip connection.
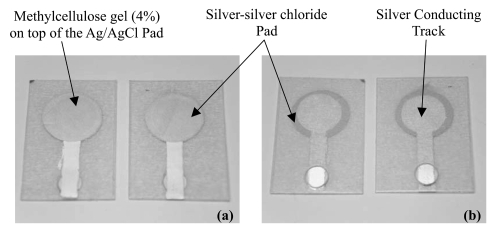
Ten healthy human volunteers (8 men and 2 women), aged between 22 and 35 years (mean = 26.8, SD = 3.7), with no history of dermatological disease participated in this study. The subjects were required to maintain the forearm sites under investigation free from application of any cosmetic topical formulations for at least 5 days before the study. The subjects were not fasted before the study and the measurements were taken during the working day. The study was approved by the University of Strathclyde Ethics Committee and informed consent was obtained from each volunteer.
The areas of skin of each subject’s inner forearm where SPEs were to be located were prepared by briskly rubbing the areas for 6–8 seconds with alcohol soaked pads to remove dry skin, oils and other contaminants. The skin was then allowed to dry thoroughly. Then, four SPEs were positioned on the subject’s inner forearms and fixed in position with surgical tapes. Each pair of SPEs was set with about 23 mm between the electrode centres and the two pairs of SPEs were kept about 50 mm apart. An iontophoretic applied current waveform with a current density of 0.3 mA/cm2, and polarity reversal of the electrodes every 15 minutes during current flow, was applied on one pair of the SPEs on each volunteer’s skin for a period of 60 minutes. The second pair of SPEs was used as a simultaneous control with no current passing through it. At the end of the experiment, the gel sampled from each of the paired SPEs (2 controls and 2 current sources) was mixed thoroughly as it was sampled and 20 μl samples of the MC gel were carefully pipetted from each SPE and stored separately in microcentrifuge tubes at 4 °C for later quantification of lactate and glucose. The subject’s blood glucose and lactate level was also measured before and after experiments by a portable glucose meter (FreeStyle Blood Glucose Monitoring System, TheraSense Ltd., UK) and a portable lactate meter (Accutrend® Lactate, Roche Diagnostics GmbH, Germany), respectively.
For the analysis of the samples 180 μl of 0.1M PBS was pipetted to each microcentrifuge tube containing the extracted 20 μl MC gel. The solutions were then mixed well. These solutions were then subjected to the colorimetric assays described before to determine the concentration of lactate and glucose in each gel sample.
Results
In vitro studies
In vitro diffusion studies were performed using the nanoporous membrane as the skin-like barrier in the iontophoresis cell with and without application of iontophoretic current. It was shown that passage of a current facilitates movement of lactate and glucose across the nanoporous membrane when compared to ordinary diffusion. The applied electrical current waveforms for reverse iontophoresis of lactate and glucose were (i) DC only and (ii) DC with an electrode polarity reversal every 5, 10 or 15 minutes, respectively. The results for glucose and lactate in vitro extraction are shown in .
Figure 3 In vitro reverse iontophoresis extraction of (a) lactate and (b) glucose (mean ± SD; n ≥ 12 for each bar), as a function of applied current waveforms. The iontophoretic current and current application time are 0.3 mA/cm2 and 60 minutes, respectively. The electrolyte in the electrode chambers of the diffusion cell was 25 mM, pH 7.4, HEPES buffer containing 133 mM NaCl. The lower chamber of the diffusion cell were filled with an electrolyte solution comprising 133 mM NaCl, buffered to pH 7.4 with 25 mM HEPES, and either10 mM lactate or 5 mM glucose.
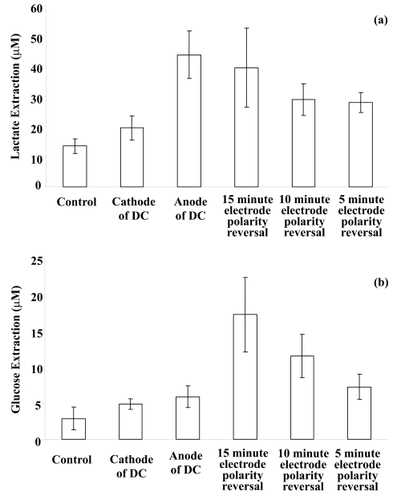
In the in vitro lactate diffusion studies, it was found that there is a significant difference between the four applied current waveforms (one-way ANOVA: p < 0.001). Lactate extraction at the anode during continuous current passage (without a polarity reversal) was, as might be expected for a negatively charged ion, found to be significantly higher than that at all other applied current waveforms (LSD post-hoc multiple comparisons: p < 0.001 in all cases), except the 15 minute electrode polarity reversal waveform. Lactate extraction using the 15 minute electrode polarity reversal condition was found to be significantly higher (LSD post-hoc multiple comparisons: p < 0.001 in both cases) than that of protocols utilizing 5 and 10 minutes electrode polarity reversal conditions. It was also found that there was a significant higher lactate extraction for 5, 10 and 15 minutes electrode polarity reversal protocols than that in the control sample which utilized diffusion-only extraction (LSD post-hoc multiple comparisons: p < 0.05 in all cases).
In the in vitro glucose diffusion studies, a significant difference was found between the four applied current waveforms (one-way ANOVA: p < 0.001). It was found that glucose extraction at 15 minute electrode polarity reversal was significantly higher than that at all other applied current waveforms (LSD post-hoc multiple comparisons: p < 0.001 in all cases). A significantly higher glucose extraction was also found in 5, 10 and 15 minutes electrode polarity reversal than the control (LSD post-hoc multiple comparisons: p < 0.001 in all cases). It can be seen in that anodal extraction of glucose was greater than cathodal extraction but it was not a significant difference.
Transdermal extraction of glucose and lactate from human subjects
During the human studies, lactate and glucose were successfully extracted simultaneously from the subjects’ skin using reverse iontophoresis.
Only one subject (Subject D) reported any discomfort, mainly the experience of a very weak tingling sensation as the current was brought to 0.3 mA at the start of the experiment and when the electrode polarity reversed. At time zero (the start of current flow), she experienced a tingling sensation which lasted no longer than two seconds. At time = 15 minutes of current passage (ie, the moment of electrode polarity reversal), she again experienced a tingling sensation which also lasted no longer than two seconds. After that, she did not experience any other further discomfort or sensations. Four subjects had very mild erythema at the reverse iontophoresis site after the experiment and this lasted for 15–30 minutes after termination of current flow.
The results of analysis of the human studies are summarized in and and , and . Transdermal diffusion of both lactate and glucose occurred for each subject, as observed in the control electrodes that were not subjected to iontophoretic current. However, reverse iontophoresis was found to significantly promote more glucose extraction (around 4 times greater) and lactate extraction (around 2.5 times greater) than diffusion alone (independent t-test: p < 0.001 in both cases) as shown in .
Table 1 Measured blood lactate level and transdermally extracted lactate level for the 10 healthy volunteers
Table 2 Measured blood glucose level and transdermally extracted lactate level for the 10 healthy volunteers
Figure 4 Average results of long duration bipolar direct current application (current density of 0.3 mA/cm2, polarity of electrodes reversed at intervals of 15 minutes, experimental time of 60 minutes) on human transdermal extraction of (a) lactate and (b) glucose (mean ± SD; n = 10). Extraction of lactate or glucose by reverse iontophoresis was significantly higher (p < 0.001 for both cases) than that of the control sample.
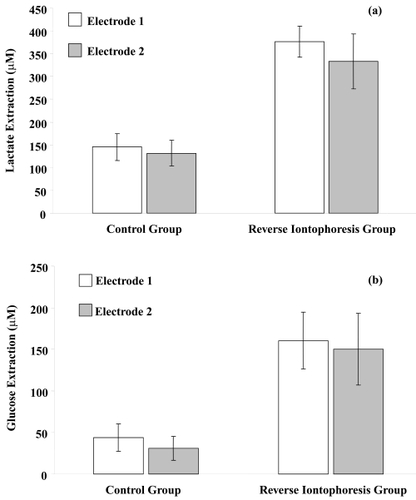
Figure 5 Graphical comparison of real blood glucose levels of healthy subjects and glucose levels in the collection methylcellulose gel after reverse iontophoresis.
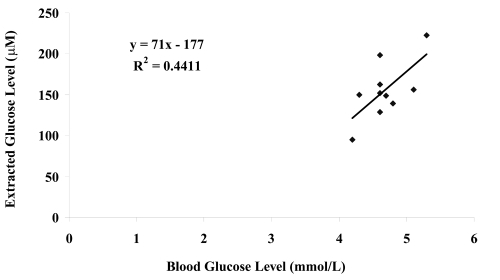
Figure 6 Graphical comparison of real blood lactate levels of healthy subjects and lactate levels in the collection methylcellulose gel after reverse iontophoresis.
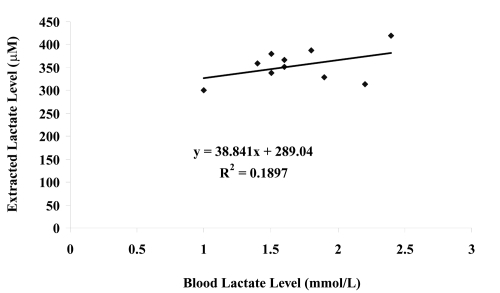
The blood glucose levels of the ten healthy volunteers at the start of the experiment and the extracted glucose levels (averaged glucose found in both gel electrodes) after reverse iontophoresis were compared (see ) and poor correlation was found between extracted and blood glucose across the subject group (r2 = 0.44). It was found that there was no significant different in the volunteers’ blood glucose levels before and after experiment (paired t-test: p = 0.227). The poor correlation across the group when comparing internal versus extracted glucose is due to skin permeability differences between human subjects and an individual calibration of the device for each subject would be required for practical, time dependent monitoring.
Similarly, the blood lactate levels of the ten healthy volunteers at the start of the experiment and the extracted lactate levels (averaged lactate extraction across both electrodes) were also compared (see ) and no correlation was found (r2 = 0.19). It was found that there was no significant different on the volunteers’ blood lactate level before and after experiment (paired t-test: p = 0.116). Again, the lack of correlation across the group in internal versus extracted lactate is most likely to be due to skin permeability differences between human subjects and individual calibration of the device for each subject would be required for any practical application of the technique.
Discussion and conclusions
For the in vitro diffusion studies, cellulose ester nanoporous membrane was used to substitute for human or animal skin samples in order to avoid the large sample-to-sample differences in human skin. This allowed the electrical properties of the electrodes and the effects of the electrical waveforms on reverse iontophoresis of lactate and glucose to be studied without the nonuniformities introduced by large sample-to-sample variation in animal tissue. Moreover, the availability of excised human or animal skin samples is becoming limited for legal, ethical and safety reasons. Though excised human skin samples can be obtained, they may have a wide range of physical parameters that differ and therefore introduce a significant scatter into any skin transport experiments. Thus basic electrical characterisation studies of the electrodes and instrumentation for iontophoresis are preferably conducted in as controlled an environment as possible before skin studies commence.
In the in vitro glucose diffusion studies, glucose is an uncharged molecule and therefore electroosmosis is the main mechanism for glucose extraction during reverse iontophoresis. Because the efficiency of electroosmotic flow is weakly dependent upon current density in the range of 0.14–0.55 mA/cm2 (CitationDelgado-Charro and Guy 1994), a current density for iontophoresis of 0.3 mA/cm2, close to the value recommended as an upper limit by other workers (CitationLedger 1992), was used.
Electroosmotic flow is always in the same direction as the flow of counter ions. Since human skin is negatively charged under physiological conditions (CitationBurnette 1987), the counter ions are cations and the electroosmotic flow is thus from anode to cathode (CitationGuy et al 2000; CitationPikal 2001). The nanoporous membrane used in the diffusion cell is negatively charged. Therefore, electroosmotic flow in this model system should be from anode to cathode during direct current reverse iontophoresis and thus more glucose should be found at cathode rather than anode. However, in this model system, extracted level of glucose at the anode was slightly greater than that found at the cathode during direct current reverse iontophoresis but this was not significant. This result was not in agreement with other researchers (CitationRao et al 1993, Citation1995). The reason that the anodal extraction is greater than the cathodal extraction may be due to neutral or positively charged pores existing in the membrane (CitationPikal and Shah 1990). It is also possible that some masking of negative charge in the nanoporous membrane or some membrane polarization occurred which reduced the effect of cathodal electroosmosis.
As can be seen in , the amount of glucose extracted in the model system at either 10 or 15 minutes switching time was significantly more than that obtained by continuous current passage without switching of the electrode polarity (ie, cathode and anode held constant) but this was inconsistent with the results reported by CitationSanti and Guy (1996). They found that total mannitol extractions were statistically equivalent for the continuous current passage with or without switching, albeit that a different sugar molecule was studied. The increased glucose extracted in this study with electrode polarity reversal may be due to effective depolarization of the membrane (Banga and Chien 1988).
Based on the findings, it is postulated that a 15 minute time interval for electrode polarity reversal is optimum in an applied current waveform as it extracts significantly more glucose than found in the control gel (at least 3.9 times greater) and more than DC current passage alone (at least 3.1 times greater) within the present study.
In the in vitro lactate diffusion studies, results showed that reverse iontophoresis could facilitate lactate extraction and applied current waveforms governed the amount of lactate to be extracted. Lactate, a negatively charged ion, is mainly extracted by electromigration during reverse iontophoresis. Based on a general principle of electricity, with like charges repelling each other and opposite charges attracting each other, lactate passed easily across the nanoporous membrane to the anode during continuous current passage. Therefore, more lactate should be detected at the anode than at the cathode.
Clearly, the time of the application of positive polarity to the electrode in any chamber during periodic electrode polarity reversal is half of that of the continuous current conditions. However, the lactate found in either chamber in the polarity reversal mode tended to be a function of the overall positive and negative current cycles in each chamber and as such the 15 min switching period extracted a good level of lactate. It was deemed worthwhile to try and extract both lactate and glucose from the human subjects utilizing the 15 minute switching interval.
In the human studies, 40% of the subjects still displayed very mild erythema even when bipolar current was employed for reverse iontophoresis despite the polarity reversal of the electrodes.
Relatively few researchers have investigated glucose extraction by reverse iontophoresis in human subjects (see for example: CitationRao et al 1995; CitationPotts et al 2002; CitationSieg et al 2004a, Citation2004b) and no researchers have studied the effects of reverse iontophoresis on lactate extraction both in human subjects and in vitro. CitationRao and colleagues (1995) passed a direct current (0.25 mA/cm2) across the human skin (ventral forearm) of subjects for a period of 60 minutes to extract glucose out of the skin and they found that around 5.83 nmol of glucose could be extracted at the cathode, somewhat less than found in this study. In the present study around 14 nmol of glucose was extracted by the application of bipolar direct current for 60 minutes. The possible explanation might be that we used a slightly higher current density (0.3 mA/cm2) compared with the study by CitationRao and colleagues (1995) More importantly, we used a bipolar direct current rather than a direct current. Since glucose is mainly extracted out of the skin by electroosmosis and bipolar direct current can prevent the reduction in the level of electroosmosis, bipolar direct current can promote more glucose extraction than direct current.
In this study reverse iontophoresis significantly promoted transdermal lactate extraction, around 2.5 times greater than diffusion alone, but there is no other human study for data comparison.
The concentration levels of glucose and lactate found in the gel electrodes had average values >150 μM for glucose and >330 μM for lactate. These concentration levels would be eminently suitable for biosensor detection directly in the gel electrodes allowing continuous monitoring of both.
In summary, both glucose and lactate can be effectively and noninvasively extracted from human subjects simultaneously by reverse iontophoresis. This illustrates the potential for the development of a continuous and noninvasive system to concurrently monitor blood glucose and lactate levels. To arrive at this a calibration of internal versus external glucose and lactate will be required for each individual subject. We have only been able to study this in a limited manner in the current work. As shown in and , there was a poor correlation across the subject group between blood glucose and extracted glucose levels and blood lactate and extracted lactate levels. However, this is to be expected because of the known skin to skin permeability variations between human subjects. This was overcome in the GlucoWatch® and a calibration was possible for each individual user. Each user was able to calibrate the device by utilising a single finger stick internal blood glucose sample which the device correlated with the reverse iontophoresis glucose level of the wearer at a single point in time (CitationTamada et al 1999). This was then used in an algorithm to link reverse iontophoresis glucose to internal glucose without the need for any further blood tests for a period of up to 12 hours. It is hoped that long term temporal studies on individual subjects will allow similar calibration procedures to be developed for lactate and glucose in simultaneous monitoring.
Acknowledgements
We would like to thank Brian Cartlidge for his technical help, Canniesburn Research Trust for funding part of this work and John Swire and Sons Ltd. (HK)for the support of the studies of Congo TS CHING with a James Henry Scott Scholarship. There are no conflicts of interest to report.
References
- AbramsonDScaleaTMHitchcockRLactate clearance and survival following injuryJ Trauma19933558498411283
- AmosAFMcCartyDJZimmetPThe rising global burden of diabetes and its complications: estimates and projections to the year 2010Diabet Med199714Suppl 5S1S859450510
- ArtruFJourdanCPerret-LiaudetALow brain tissue oxygen pressure: incidence and corrective therapiesNeurol Res199820Suppl 1S48S519584925
- BakkerJCoffernilsMLeonMBlood lactate levels are superior to oxygen-derived variables in predicting outcome in human septic shockChest199199956622009802
- BangaAKCheinYWIontophoretic delivery of drugs: Fundamentals, developments and biomedical applicationsJ Control Release19987124
- BellDSHStroke in the diabetic patientDiabetes Care199417213198174450
- de BoerJPotthoffHMulderPOLactate monitoring with subcutaneous microdialysis in patients with shock: a pilot studyCirc Shock19944357637834821
- BrancatiFLWheltonPLRandallBLRisk of end-stage renal disease in diabetes mellitus: a prospective cohort study of men screened for MRFIT. Multiple Risk Factor Intervention TrialJAMA19972782069749403420
- BurnetteRROngpipattanakulBCharacterization of the perm selective properties of excised human skin during iontophoresisJ Pharm Sci US19877676573
- CairnsCBMooreFAHaenelJBEvidence for early supply independent mitochondrial dysfunction in patients developing multiple organ failure after traumaJ Trauma19974253269095123
- CarrierMTrudelleSThaiPIschemic threshold during cold blood cardioplegic arrest: monitoring with tissue pH and pO2J Cardiovasc Surg (Torino)1998395937
- ChienYWSiddiquiOSunYTransdermal iontophoretic delivery of therapeutic peptides/proteins. I: InsulinAnn N Y Acad Sci198750732513327418
- ChienYWLelawongsPSiddiquiOFacilitated transdermal delivery of therapeutic peptides and proteins by iontophoretic delivery devicesJ Control Release1990132–326378
- ChingCTSCamilleriIConnollyPA low-cost, programmable device for versatile current delivery in iontophoresis applicationsSensor Actuat B-Chem200510653440
- ConnollyPCottonCMorinFOpportunities at the skin interface for continuous patient monitoring: A reverse iontophoresis model tested on lactate and glucoseIEEE Trans Nanobioscience20021374116689220
- DavisBLKuznickiJPraveenSSLower-extremity amputations in patients with diabetes: pre- and post-surgical decisions related to successful rehabilitationDiabetes Metab Res Rev200420Suppl 1S45S5015150814
- DegimITIlbasmisSDundarozRReverse iontophoresis: a non-invasive technique for measuring blood urea levelPediatr Nephrol2003181032712898373
- Delgado-CharroMBGuyRHCharacterization of convective solvent flow during iontophoresisPharm Res199411929357937551
- EliaSLiuPHilgenbergACoronary haemodynamics and myocardial metabolism during weaning from mechanical ventilation in cardiac surgical patientsCan J Anaesth199138564711934203
- Evans J. 1995. Causes of blindness and partial sight in England and Wales 1990–1991. HMSO.
- GershBJAndersonJLThrombolysis and myocardial salvage. Results of clinical trials and the animal paradigm – paradoxic or predictable?Circulation1993882963068319343
- GuyRHKaliaYNDelgado-CharroMBIontophoresis: electrorepulsion and electroosmosisJ Control Release2000641–31293210640651
- HabichtJMWolffTLangemannHIntraoperative and postoperative microdialysis measurement of the human heart – feasibility and initial resultsSwiss Surg1998Suppl 226309757802
- HildingssonUSelldenHUngerstedtUMicrodialysis for metabolic monitoring in neonates after surgeryActa Paediatr199685589948827104
- HirvonenJHueberFGuyRHCurrent profile regulates iontophoretic delivery of amino acids across the skinJ Control Release19953723949
- HowardJPDrakeTRKelloggDLEffects of alternating current iontophoresis on drug deliveryArch Phys Med Rehabil19957646367741619
- [IDF] International Diabetes FederationDiabetes Atlas 200320032BrusselsInternational Diabetes Federation
- KingHRewersMGlobal estimates for prevalence of diabetes mellitus and impaired glucose tolerance in adults. WHO Ad Hoc Diabetes Reporting GroupDiabetes Care199316157778123057
- LaingSPSwerdlowAJSlaterSDThe British Diabetic Association Cohort Study, I: all-cause mortality in patients with insulin-treated diabetes mellitusDiabet Med1999a164596510391392
- LaingSPSwerdlowAJSlaterSDThe British Diabetic Association Cohort Study, II: cause-specific mortality in patients with insulin-treated diabetes mellitusDiabet Med1999b164667110391393
- LedgerPWSkin biological issues in electrically enhanced transdermal deliveryAdv Drug Deliver Rev199292–3289307
- LevrautJCiebieraJPChaveSMild hyperlactatemia in stable septic patients is due to impaired lactate clearance rather than overproductionAm J Respir Crit Care Med19981574 Pt 1102169563714
- MarecauxGPinskyMRDupontEBlood lactate levels are better prognostic indictors than TNF and IL-6 levels in patients with septic shockIntens Care Med1996224048
- MendelowitschAMergnerGWShuaibACortical brain microdialysis and temperature monitoring during hypothermic circulatory arrest in humansJ Neurol Neurosurg Psychiatry199864611189598676
- MenzelMDoppenbergEMZaunerAIncreased inspired oxygen concentration as a factor in improved brain tissue oxygenation and tissue lactate levels after severe human head injuryJ Neurosurg19999111010389873
- MerinoVKaliaYNGuyRHTransdermal therapy and diagnosis by iontophoresisTrends Biotechnol1997152882909263476
- MerinoVLopezAHochstrasserDNoninvasive sampling of phenylalanine by reverse iontophoresisJ Control Release1999611–265910469903
- MizeNKButteryMDaddonaPReverse iontophoresis: monitoring prostaglandin E2 associated with cutaneous inflammation in vivoExp Dermatol199762983029412817
- MoomeyCBJrMeltonSMCroceMAPrognostic value of blood lactate, base deficit, and oxygenderived variables in an LD50 model of penetrating traumaCrit Care Med199927154619934910
- MurrayMJGonzeMDNowakLRSerum D(-)-lactate levels as an aid to diagnosing acute intestinal ischemiaAm J Surg199416757588209931
- NumajiriSSugibayashiKMorimotoYNon-invasive sampling of lactic acid ions by iontophoresis using chloride ion in the body as an internal standardJ Pharm Biomed Anal19931190398305594
- PickupJPickupJCWilliamsGDiabetic control and its measurementsTextbook of Diabetes20033OxfordBlackwell34.134.17
- PikalMJShahSTransport mechanisms in iontophoresis. III. An experimental study of the contributions of electroosmotic flow and permeability change in transport of low and high molecular weight solutesPharm Res1990722292339093
- PikalMJThe role of electroosmotic flow in transdermal iontophoresisAdv Drug Deliv Rev2001461–328130511259844
- PitzerKRDesaiSDunnTDetection of hypoglycaemia with the GlucoWatch biographerDiabetes Care200124881511347748
- PottsROTamadaJATierneyMJGlucose monitoring by reverse iontophoresisDiabetes Metab Res Rev200218Suppl 1S49S5311921430
- RaoGGlikfeldPGuyRHReverse iontophoresis: Development of a noninvasive approach for glucose monitoringPharm Res199310175158302761
- RaoGGuyRHGlikfeldPReverse iontophoresis: Noninvasive glucose monitoring in vivo in humanPharm Res1995121869738786958
- RasmussenIHilleredLUngerstedtUDetection of liver ischemia using microdialysis during experimental peritonitis in pigsShock199416067743330
- RojdmarkJBlomqvistLMalmMMetabolism in myocutaneous flaps studied by in situ microdialysisScand J Plast Reconstr Surg Hand Surg19983227349556818
- SantiPGuyRHReverse iontophoresis – parameters determining electroosmotic flow. I. PH and ionic strengthJ Control Release1996382–315965
- SiegAGuyRHDelgado-CharroMBSimultaneous extraction of urea and glucose by reverse iontophoresis in vivoPharm Res20042118051015553226
- SiegAGuyRHDelgado-CharroMBNoninvasive glucose monitoring by reverse iontophoresis in vivo: Application of the internal standard conceptClin Chem2004b5013839015155544
- SlomovitzBMLaveryRFTortellaBJValidation of a handheld lactate device in determination of blood lactate in critically injured patientsCrit Care Med199826152389751588
- StephensonJMKennySStevensLKProteinuria and mortality in diabetes: the WHO Multinational Study of Vascular Disease in DiabetesDiabet Med199512149557743762
- TamadaJAGargSJovanovicLNoninvasive glucose monitoring: Comprehensive clinical resultsJAMA199928218394410573275
- TierneyMJTamadaJAPottsROCygnus Research TeamClinical evaluation of the GlucoWatch® biographer: a continual, non-invasive glucose monitor for patients with diabetesBiosens Bioelectron2001169–12621911679237
- The Diabetes Research in Children Network (DirecNet) Study GroupAccuracy of the GlucoWatch G2 Biographer and the continuous glucose monitoring system during hypoglycemia: Experience of the Diabetes Research in Children NetworkDiabetes Care200427722614988292
- The UK Prospective Diabetes Study Group (UKPDS) GroupIntensive blood-glucose control with sulphonylureas or insulin compared with conventional treatment and risk of complications in patients with type 2 diabetes (UKPDS 33)Lancet1998a35283753
- The UK Prospective Diabetes Study Group (UKPDS) GroupEffect of intensive blood-glucose control with metformin on complications in overweight patients with type 2 diabetes (UKPDS 34)Lancet1998b35285465
- The UK Prospective Diabetes Study GroupEfficacy of atenolol and captopril in reducing risk of macrovascular and microvascular complications in type 2 diabetes: UKPDS 39BMJ1998c31771320
- The UK Prospective Diabetes Study GroupTight blood pressure control and risk of macrovascular and microvascular complications in type 2 diabetes: UKPDS 38BMJ1998d31770313
- TomohiraYMachidaYOnishiHIontophoretic transdermal absorption of insulin and calcitonin in rats with newly-devised switching technique and addition of ureaInt J Pharm19971552319
- ValadkaABGoodmanJCGopinathSPComparison of brain tissue oxygen tension to microdialysis-based measures of cerebral ischemia in fatally head-injured humansJ Neurotrauma199815509199674554
- VincentJLEnd-points of resuscitation: arterial blood pressure, oxygen delivery, blood lactate, or…?Intensive Care Med199622358857431
- VincentJLBlood lactate levels: index of tissue oxygenationInt J Intensive Care199854853
- VolpeGMosconeDCompagnoneDIn vivo continuous monitoring of L-lactate coupling subcutaneous microdialysis and an electrochemical biocellSensor Actuat B-Chem1995241–313841
- WangSLHeadJStevensLExcess mortality and its relation to hypertension and proteinuria in diabetic patients. The World Health Organization Multinational Study of Vascular Disease in DiabetesDiabetes Care199619305128729151
- WestgrenMKrugerKEkSLactate compared with pH analysis at fetal scalp blood sampling: a prospective randomised studyBr J Obstet Gynaecol199810529339442158
- WestgrenMKublickasMKrugerKRole of lactate measurements during laborObstet Gynecol Surv1999544389891299
- WildSRoglicGGreenAGlobal Prevalence of Diabetes: Estimates for the year 2000 and projections for 2030Diabetes Care20042710475315111519
- ZaunerADoppenbergESoukupJExtended neuromonitoring: new therapeutic opportunities?Neurol Res199820Suppl 1S85S909584932