Abstract
The preparation, properties, and application in adriamycin delivery of biocompatible and biodegradable poly(lactide-co-glycolide)-polyethylene glycol (PLGA-PEG) nanoparticles are discussed. PLGA-PEG copolymers were synthesized by ring opening polymerization of the dl-lactide and glycolide in the presence of PEG1000.1H-NMR and FT-IR spectrum were consistent with the structure of PLGA-PEG copolymers. The adriamycin-loaded nanoparticles could be prepared using a precipitation-solvent evaporation technique. The nanoparticles have been produced by a precipitation-solvent evaporation technique. The physical characteristics and drug loading efficiency of the PLGA-PEG nanoparticles were influenced by the composition of the PLGA-PEG copolymers used to prepare the nanoparticles. Particle sizes were between 65 and 100 nm for different compositions of PLGA-PEG copolymers. PLGA-PEG nanoparticles prepared from copolymers having relatively high PLGA/PEG ratios were smaller. Entrapment efficiency was 25%–33%. Adriamycin release from the nanoparticles at pH 7.4 showed an initial burst release and then sustained release phase. These results showed that PLGA-PEG nanoparticles could be an effective carrier for cancer therapy.
Introduction
The development of drug delivery systems has improved the therapeutic and toxicological properties of existing chemotherapies and facilitated the implementation of new ones. By including the drug in technologically optimized drug delivery systems or conjugating the drugs with different polymers, it is possible to modify the pharmacokinetics and biodistribution of the drugs improving the efficacy and security of the therapy (CitationPeppas and Blanchette 2004).
A critical advantage in treating cancer with advanced, non-solution based therapies is the inherent leaky vasculature present serving cancerous tissues. The defective vascular architecture, created due to the rapid vascularization necessary to serve fast-growing cancers, coupled with poor lymphatic drainage allows an enhanced permeation and retention effect (EPR effect) (CitationTeicher 2000; CitationSledge and Miller 2003).
Targeting the tumor vasculature is a strategy that can allow targeted delivery to a wide range of tumor types (CitationEatock et al 2000; CitationReynolds et al 2003).Tremendous opportunities exist for using nanoparticles as controlled drug delivery systems for cancer treatment (CitationPanyam and Labhasetwar 2003; CitationBirnham and Branno-Peppas 2004). Natural and synthetic polymers including albumin, fibrinogen, alginate, chitosan, and collagen have been used for the fabrication of nanoparticles. However, among all of these, lactic-glycolic acid copolymers are the most frequently employed materials due to their biocompatibility and biodegradability (CitationOrive et al 2005). Nanoparticulate drug carriers must show persistence in systemic circulation after intravenous (i.v.) administration in order to be useful for controlled drug delivery and/or targeting applications. The biodistribution properties of polylactide-co-glycolide-co-polyethylene glycol (PLGA-PEG) nanoparticles have been studied in experimental animals after labeling them with radioactive agents. The results showed a character of extending half-life and ability to control the release of the encapsulated compounds (CitationLi et al 2001). PLGA-PEG nanoparticles were found to exhibit linear, dose-independent pharmacokinetics for a dose range of 150–1050 μg per mouse. The dosage-independence of the PLGA-PEG nanoparticles would provide further advantages for their application in controlled drug delivery and targeting (CitationBeletsi et al 2005).
One of the most potent and widely used anticancer drugs is an adriamycin (doxorubicin), which works by inhibiting the synthesis of nucleic acids within cancer cells (CitationYoo and Park 2000). Adriamycin has a number of undesirable side-effects such as cardiotoxicity and myelosupression, which leads to a very narrow therapeutic index. Various researchers have studied ways to target adriamycin delivery to cancer tissues or at least to diminish its side effects (CitationKwon et al 1995; CitationMitra et al 2001; CitationNa et al 2003; CitationShikata et al 2002).
The aim of present work was to assess the merits of PEG-PLGA nanoparticles as an adriamycin carrier. For this purpose, the copolymer PLGA-PEG was synthesized and characterized. Polymeric nanoparticles were prepared using a precipitation-solvent evaporation technique. The loading efficiency of the nanoparticles and in-vitro drug release from the nanoparticles was investigated.
Materials and methods
Materials
DL-Lactide (LA) and glycolide (GA) were purchased from Boehringer Ingelheim (Germany). They were recrystallized twice from ethyl acetate and dried under high vacuum at room temperature before use. Polyethylene glycol (PEG, molecular weight: 1000) was obtained from Sigma Chemical Co. (St. Louis, MO, USA) and dried under high vacuum at room temperature. Stannous octoate was also obtained from Sigma.
Synthesis and characterization of PLGA-PEG copolymers
PLGA-PEG copolymers of different composition were prepared by a melt polymerization process under nitrogen, using stannous octoate as catalyst (CitationBeletsi et al 1999). They were characterized by FT-IR (Shimadzu 8400) and 1H-NMR (Bruker AC-80) and their molecular weight and molecular weight distribution (polydispersity index, Mw/Mn) was determinedly gel permeation chromatography (GPC, Walters, 515 HPMC pump).
Preparation of nanoparticles
PLGA-PEG nanoparticles were prepared using a precipitation-solvent evaporation technique (CitationAvgoustakis et al 2003). Briefly, a solution of the polymer in acetone was transferred drop wise to a stirred aqueous solution of poly vinyl alcohol (PVA, molecular weight: 10000, 0.5% w/v). The mixture was kept under stirring until acetone had been evaporated, and the nanoparticle dispersion formed was condensed in a rotary evaporator and filtered through a 1.2 μm filter (Millex AP, Millipore). Finally, the nanoparticles were collected by centrifugation at 25000×g for 25 min and washed twice with water before lyophilization (Christ Alpha 1–4).
FT-IR (cm−1): 3010, 2955, 2880, 1765, 1185-1070.
H-NMR (ppm); δ=3.7 (m, 4H, methylene groups of PEG), δ=1.6 (d, 3H, methyl group of the lactide), δ=4.8 (d, 2H, methylene group of glycolide), δ= 5.2 (m, 1H, CH of lactide).
Observation of transmission electron microscope (TEM)
The morphology of the polymeric nanoparticles was observed using a TEM (LEO 960). A drop of drug loaded nanoparticle suspension in aqueous solution was placed on a carbon film coated on a copper grid for TEM and freez-dried. Observation was done at 80 kV.
Entrapment efficiency
Loaded drug quantity was determined according to the following procedure: after nanoparticles were formed, unbound adriamycin was separated by centrifugation at 30000×g at 25º C for 10 min and the precipitate was discarded. The precipitate was then lyophilized and resulting powder containing the loaded nanoparticles was dissolved in ethanol to obtain a clear solution and analyzed UV spectrophotometrically (Shimadzu UV-160) at 479 nm. Loading capacity was expressed in terms of entrapped drug quantity, and entrapment efficiency.
In vitro drug release
The in vitro release experiments were carried out as follows: 5g of adriamycin-loaded polymeric nanoparticles and 1 ml of phosphate buffer saline (PBS 0.1 M, pH 7.4) were put into a dialysis tube and then was introduced into a vial with 10 mL of PBS. At specific time interval, the whole medium was taken and replaced with fresh PBS. The concentration of the released adriamycin was determined by UV spectrophotometer at 479 nm.
Results and discussion
Synthesis and characterization of PLGA-PEG copolymers
PLGA-PEG copolymers of different composition were prepared by a melt polymerization process. They were characterized with regard to their composition by 1H-NMR and their molecular weight and molecular weight distribution The results are given in .
Table 1 Characterization of PLGA-PEG copolymers of with different composition
Physicochemical characterization of nanoparticles
In order to investigate the physicochemical characteristics of nanoparticles, they were observed by TEM (). From these micrographs, nanoparticles prepared with PLGA-PEG were spherical in shape and uniform with size about 60–100 nm.
Figure 1 Transmission electron microscopy (TEM) photographs of adriamycin-loaded PLGA-PEG nanoparticles (A: sample PI, B: sample PII, C: sample PIII).
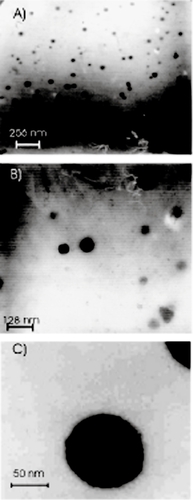
The encapsulation efficiency values achieved for adriamycin were influenced by the presence of PEG in PLGA-PEG chains ().
Table 2 Physicochemical characteristics and encapsulation efficiency of adriamycin-loaded PLGA-PEG nanoparticlesTable Footnote*
From the values in , the particle sizes were dependent on the PEG percent of the copolymer i.e., the higher the PEG percent, the larger the particle size.
Drug loading and release study in vitro
The adriamycin loading contents and loading efficiency onto the PLGA-PEG nanoparticles are shown in . It was found that drug loading contents and loading efficiency increased with a decrease of PEG percent in the copolymer.
Total release of adriamycin from PLGA-PEG nanoparticles is shown in .
Figure 2 Release of adriamycin (ADR) from PLGA-PEG nanoparticles at pH 7.4, 37ºC (average ±SD, n=3).
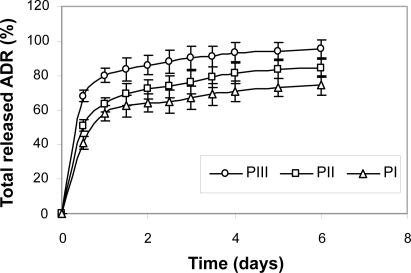
These results indicate that the release of adriamycin from the nanoparticles decreased with an increase of PEG content in PLGA-PEG nanoparticles. The slower release in the nanoparticles with higher PEG content can be attributed to the stronger hydrophobic interaction between hydrophobic domain (PLGA) and drug. It was also found that the greater the drug content, the slower the drug release.
In the release pattern of the samples a pseudo zero-order release after an initial burst release effect was observed. It is generally assumed that a drug is released by several processes such as diffusion through the polymer matrix, release by polymer degradation and solubilization and diffusion through microchannels that exist in the polymer matrix or are formed by erosion (CitationJeong et al 1999).In this system, polymer degradation occurred after about 21–25 days; accordingly, we can consider that the drug is released from the nanoparticles through the diffusion mechanism in vitro.
The measured acidic pH of extracellular fluid of solid tumors has extensively been documented for a few decades, combined with biological backgrounds of this observation. The acidic pH is now regarded as a phenotype of solid tumors for their growth and invasiveness (CitationTannock and Rotin 1989; CitationStubbs et al 2000). This finding has prompted investigators to fabricate pH-sensitive carriers by decorating the surface with carboxylic groups. Based on their findings, at pH 6.8 and 6.4 the pH-sensitive nanoparticles aggressively bounded to tumor cells.
The adriamycin release rate from the PLGA-PEG nanoparticles was also pH-dependent and enhanced at pH 6.4, as presented in .It is generally assumed that a drug is released by several process (CitationGref et al 1994) such as diffusion through the polymer matrix, release by polymer degradation and solubilization and diffusion through microchannels that exist in the polymer matrix or are formed by erosion. The copolymers prepared in the present work are ABA triblock copolymers composed of hydrophobic A-blocks (lactide-co-glycolide) and hydrophilic B blocks (central PEG). These copolymers are not soluble in water but exhibit reverse thermal and pH-dependent gelation properties. Hydrolysis of the ester linkage in these polymers will cause the swelling to increase with time as hydrolysis proceeds. The gel becomes increasingly pH-sensitive as hydrolysis proceeds and carboxylic acid groups are generated in the structure. In within a few days (6 days) we can consider that the drug is released from the PLGA-PEG nanoparticles through the diffusion mechanism in vitro. The swelling of the particles increased in acidic buffered solutions due to the protonation of PEG central groups and formation of positively charged chains in the polymer structure.
Conclusion
To reduce or minimize undesired interactions or undesired uptake into normal sites, a biodegradable nanocarrier has been developed for adriamycin wherein the amount and site of drug release is controlled by the structure of the copolymer and pH. This nanoparticle was designed and prepared, so that the carrier can be used for targeting a broad range of solid tumors. For this purpose, ABA triblock copolymers of PLGA-PEG were synthesized by ring opening polymerization of the lactide and glycolide in the presence of PEG 1000. 1H-NMR and FT-IR spectrum were consistent with the structure of PLGA-PEG copolymer. The adriamycin-loaded nanoparticles could be prepared using a precipitation-solvent evaporation technique. The entrapment efficiency was 25%–33%, and particle size about 65–95 nm. Adriamycin release from the nanoparticles at pH 7.4 showed an initial burst release and then sustained release phase.
The results demonstrated in-vitro that the adriamycin loaded PLGA-PEG nanoparticles show pH sensitivity and can be applied for targeting extracellular pH (pHc) and could be an effective carrier for cancer therapy. It is expected that at tumor pH the adriamycin loaded nanoparticles made of PLGA-PEG triblock copolymers can show enhanced cytotoxicity compared to that at normal pH.
References
- AvgoustakisKBeletsiAPanagiZ2003Effect of copolymer composition on the physicochemical characteristics, in vitro stability, and biodistribution of PLGA-mPEG nanoparticlesInt J Pharm2591152712787641
- BeletsiALeondiadisLKlepetsanisP1999Effect of preparative variables on the properties of PLGA-mPEG copolymers related to their applications in controlled drug deliveryInt J Pharm1821879710341308
- BeletsiAPanagiZAvgoustakisK2005Biodistribution properties of nanoparticles based on mixtures of PLGA with PLGA-PEG diblock copolymersInt J Pharm2982334115936907
- BirnbaumDTBrannon-PeppasL2004Microparticle drug delivery systemsBrownDMDrug delivery systems in cancer therapyHumana Press11735
- EatockMMSchätzlainAKayeSB2000Tumor vasculature as a target for anticancer therapyCancer Treat Rev2619120410814561
- GrefRMinamitakeYPeracchiaMT1994Biodegradable long circulating polymeric nanosphersScience2631600308128245
- JeongYNahJWLeeHC1999Adriamycin release from flower-type polymeric micells based on star-block copolymer composed of poly (γ- Benzyl L-glutamate) as the hydrophobic part and poly(ethylene oxide) as the hydrophilic partInt J Pharm188495810528082
- KwonGSNaitoMYokoyamaM1995Physical entrapment of adriamycin in AB block copolymer micellePharm Res1219257784332
- LiYPPeiYYZhangXY2001PEGylated PLGA nanoparticles as protein carriers: synthesis, preparation and biodistribution in ratsJ Control Release712031111274752
- MitraSGaurUGhoshPC2001Tumor targeted delivery of encapsulated dextran-doxorubicin conjugate using chitosane nanoparticles as carrierJ Control Release743172311489513
- NaKLeeESBaeYH2003Adriamycin loaded pulluan acetate/sulfonamide conjugate nanoparticles responding to tumor pH: pH-dependant cell interaction, internalization and cytotoxicityJ Control Release8731312618018
- OriveGHernándezRMGascAR2005Micro and nano drug delivery systems in cancer therapyCancer Ther31318
- PanyamJLabhasetwarV2003Biodegradable nanoparticles for drug and gene delivery to cells and tissueAdv Drug Del Rev5532947
- PeppasLBBlanchetteJO2004Nanoparticle and targeted systems for cancer therapyAdv Drug Del Rev56164959
- ReynoldsARMoghimiSMHodivala-DilkK2003Nanoparticle-mediated gene delivery to tumor vasculatureTrends Mol Med92412524203
- ShikataFTokumitsoHIchikavaH2002In vitro cellular accumulation of gadolinium incorporated into chitosane nanoparticles designed for neutron-capture therapy of cancerEur J Pharm Biopharm53576311777753
- SledgeGMillerK2003Exploiting the hallmarks of cancer: the future conquest of breast cancerEur J Cancer3916687512888360
- StubbsMMcSheelyPMJGriffithsJR2000Causes and consequence of tumor acidity and implications for treatmentMol Med Today6151910637570
- TannockIFRotinD1989Acid pH in tumors and its potential for therapeutic exploitationCancer Res494373842545340
- TeicherBA2000Molecular targets and cancer therapeutics: discovery, development and clinical validationDrug Resist Updat3677311498368
- YooHSParkTG2000In vitro and in vivo anti-tumor activities of nanoparticles based on doxorubicin-PLGA conjugatesPolymer Prepr419923