Abstract
Primary open angle glaucoma (OAG) is a multifactorial optic neuropathy characterized by progressive retinal ganglion cell death and associated visual field loss. OAG is an emerging disease with increasing costs and negative outcomes, yet its fundamental pathophysiology remains largely undetermined. A major treatable risk factor for glaucoma is elevated intraocular pressure (IOP). Despite the medical lowering of IOP, however, some glaucoma patients continue to experience disease progression and subsequent irreversible vision loss. The scientific community continues to accrue evidence suggesting that alterations in ocular blood flow play a prominent role in OAG disease processes. This article develops the thesis that dysfunctional regulation of ocular blood flow may contribute to glaucomatous optic neuropathy. Evidence suggests that impaired vascular autoregulation renders the optic nerve head susceptible to decreases in ocular perfusion pressure, increases in IOP, and/or increased local metabolic demands. Ischemic damage, which likely contributes to further impairment in autoregulation, results in changes to the optic nerve head consistent with glaucoma. Included in this review are discussions of conditions thought to contribute to vascular regulatory dysfunction in OAG, including atherosclerosis, vasospasm, and endothelial dysfunction.
Introduction
Primary open angle glaucoma (OAG) is a multifactorial optic neuropathy characterized by progressive retinal ganglion cell death and associated visual field loss. Glaucoma is the second leading cause of blindness worldwide (CitationLeske 2007), yet after years of extensive research the exact cause of glaucomatous optic neuropathy remains elusive. A recent estimate suggests that roughly 80 million people will be diagnosed with glaucoma by 2010, with 4.5 million of those suffering from bilateral blindness (CitationQuigley and Broman 2006). Although elevated intraocular pressure (IOP) currently remains the focus of therapy, some glaucoma patients continue to experience disease progression despite lowering of IOP. The Early Manifest Glaucoma Trial (EMGT) showed that OAG disease progression rate in the treatment group was 45% as compared with 62% in the nontreated control group (CitationHeijl et al 2002). In the Collaborative Initial Glaucoma Treatment Study (CIGTS), substantial visual field loss occurred in 10%–13.5% of participants during 5 years of follow up (CitationLichter et al 2001). Although IOP is still the only treatable OAG risk factor, it is clear that glaucoma is a complex disease that cannot be prevented or cured by IOP reducing therapies in all patients.
Other glaucoma risk factors that may be responsible for disease progression despite lowering of IOP include vascular risk factors, genetics, and other systemic conditions (CitationLeske 2007). Vascular related OAG risk factors have been found in prospective, retrospective, and large population-based studies throughout the world. While not a new concept (Citationvon Graefe 1857; CitationHayreh et al 1970; CitationHayreh 2001; CitationFlammer et al 2001), disturbed ocular blood flow has emerged as an increasingly prevalent glaucoma risk factor in large population-based trials. CitationLeske and colleagues (2007) recently presented new predictors of OAG disease progression in the EMGT trial including lower systolic perfusion pressure, lower systolic blood pressure, and cardiovascular disease history. In the Rotterdam eye study, patients with an ocular perfusion pressure lower than 50 mmHg had a four times greater risk of developing OAG than those with a perfusion pressure of 80 mmHg (CitationHulsman et al 2007). The Egna-Neumarkt study found positive correlations between systemic blood pressure and both the diagnosis of OAG and elevated IOP (CitationBonomi et al 2000). These large scale trials compliment various data from smaller prospective trials which have found aging, systemic blood pressure, nocturnal hypotension, ocular perfusion pressure, migraine, disk hemorrhage, diabetes, and directly assessed reductions of ocular blood flow to be related to OAG. It is currently unclear whether ischemia is secondary to increased IOP through faulty autoregulation of ocular blood flow or if a primary vascular component promotes damage to the optic nerve and retinal ganglion cells in certain OAG patients.
Recent studies have also suggested that perfusion instability, rather than a progressive decline in ocular blood flow, may contribute to OAG. It is well established that circadian rhythms play an important role in maintaining homeostasis in the human body. In that capacity, OAG progression is not necessarily related to the overall level of blood pressure, but an abnormal vascular reaction to a reduction in blood pressure or increase in IOP during nonwaking hours (CitationWerne et al 2008). It has been known for over four decades that IOP varies throughout the day, but not until recently has it been shown that other vascular risk factors such as systemic blood pressure (CitationO’Brien 1991), ocular perfusion pressure (CitationLiu et al 2003), and ocular blood flow (CitationOsusky et al 2000) also follow circadian patterns. Ocular blood flow deficits may therefore represent either a primary insult or are secondary to vascular regulatory dysfunction during diurnal fluctuations in vascular risk factors in certain OAG patients (CitationGrieshaber and Flammer 2005).
Blood flow autoregulation in glaucoma
Autoregulation of blood flow is described as the intrinsic capacity of an organ to maintain its blood flow despite changes in local vascular parameters (CitationHaggendal et al 1969). Within the eye, autoregulation is defined as local vascular constriction or dilation causing vascular resistance to reciprocally increase or decrease, thereby maintaining a constant nutrient supply in response to perfusion pressure changes (CitationHarris et al 1998). Challenges to normal ocular blood flow include increased IOP, fluctuating blood pressure, a resultant decrease in ocular perfusion pressure, and/or a rise in local tissue metabolic demands. Failure of stable blood flow regulation may lead to ischemic damage of the optic nerve and/or retinal ganglion cells, which likely contributes to further impairment in vascular regulation. A cascade of events may occur, which then leads to retinal ganglion cell death and structural changes of the optic nerve head (ONH) (CitationFlammer et al 2001) consistent with OAG pathophysiology ().
Figure 1 A conceptual representation of the contribution of autoregulatory dysfunction to glaucomatous damage of the optic nerve head.
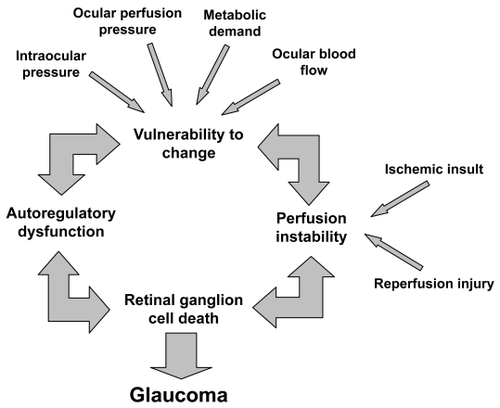
Various underlying medical conditions have been proposed which may contribute to ocular vascular regulatory dysfunction including atherosclerosis, vasospasm, and endothelial dysfunction. This review highlights these and other factors as they relate to glaucomatous optic neuropathy and discusses potential implications in our understanding of OAG disease pathophysiology and management.
Physiology of the optic nerve head blood flow autoregulation
The optic nerve is a continuation of the central nervous system and shares several physiologic similarities with the brain, including blood flow autoregulation (CitationFlammer and Orgul 1998). Despite extensive research, the precise microvascular anatomy and blood flow regulation of the ONH remains difficult to ascertain (CitationHarris et al 2003). Several mechanisms involved in the vascular regulation of ocular blood flow that have been demonstrated include: metabolic, myogenic, neurogenic, humoral, and endothelial-mediated factors.
Autoregulation of the cerebral circulation has been shown to depend largely on local blood gas perturbations (CitationHaggendal et al 1969). Similarly, as ONH blood flow decreases, the local metabolic vascular response is primarily oxygen-dependent (CitationKontos et al 1978). Hyperoxia and hypocapnia mediate arteriolar constriction and a subsequent decrease in ONH blood flow, while hypoxia and hypercapnia result in the opposite response (CitationFlammer and Orgul 1998). Additionally, the concentrations of K+, H+, adenosine, and intercellular osmolarity are known to influence local vascular tone (CitationOrgul et al 1995a).
Myogenic autoregulation involves arteriolar tone changes through smooth muscle cell to cell signal propagation (CitationJohnson 1986). Specifically, transmural pressure sensitive arteriole pacemaker cells initiate the response and although the exact mechanism has not been defined, extracellular calcium concentrations and endothelial factors appear to contribute (CitationDavies and Tripathi 1993). While myogenic autoregulation contributes to a great extent in several organs, most notably the colonic vasculature (CitationAndersson 1984), its role in ocular tissue is still under investigation.
The autonomic nervous system orchestrates a coordinated response to changes in systemic physiology. In the coronary circulation, for example, intrinsic cardiac and extracardiac autonomic neurons influence cardiac output and coronary blood flow, often irrespective of local stimuli (CitationKresh and Armour 1997). In ocular tissue, the autonomic nervous system contributes extensively to the retrobulbar and choroidal circulatory system, but the retinal and ONH vasculature lack direct innervation (CitationFunk 1997). Hence, sympathetic stimulation provides only indirect influence on OHN perfusion as neurogenic contribution to the vascular response is minimal (CitationHarris et al 2003).
In a prototypical vascular bed, vasoactive hormones in the circulating blood act directly on endothelial, pericyte, and smooth muscle cells. The ONH, however, is exceptional in that it contains 1) a blood – brain barrier preventing smooth muscle and pericyte contact with humoral factors and 2) a scleral flange also acting as a barrier to prevent humoral diffusion from the choroid to the ONH (CitationFlammer and Orgul 1998). Hormones believed to exert an autoregulatory effect on the ONH by overcoming these barriers include circulating catecholamines (CitationYu et al 1994), vasopressin, natriuretic peptides, and the renin – angiotensin system (CitationJossi and Anderson 1983).
The vascular endothelium directly regulates permeability, but also indirectly influences blood vessel diameter, coagulation parameters, cellular proliferation, and the inflammatory cascade amongst other pathways through the release of vasoactive susbstances (CitationBehrendt and Ganz 2002). Endothelial mediators are increasingly reported factors in the regulation of ONH blood flow. Studies have demonstrated a basal formation of nitric oxide in the ocular endothelium that leads to active dilatation of the vasculature as well as a dose-dependent response relationship between endothelin-1 and blood flow to the anterior optic nerve, as discussed in detail later (CitationOrgul et al 1999).
Atherosclerosis
Atherosclerosis is a chronic, progressive disease affecting many vascular beds including the ocular circulation (CitationDavies 1990). It results from the deposition of lipids, inflammatory cells, and connective tissue within arterial walls, leading to plaque formation and intimal thickening. Over time this results in obstruction to flow, compromised perfusion, and tissue ischemia (CitationDavies et al 2004; Citationde Voogd et al 2006). Recent research on the topic of atherosclerosis has focused on molecular and cellular mechanisms and led to a shift in the paradigm of disease pathophysiology (CitationDavies et al 2004). Studies have shown endothelial cell dysfunction to be the first detectable change in atherosclerotic vessels (CitationBehrendt and Ganz 2002). This is supported by the observation that risk factors such as aging, smoking, hypertension, elevated low-density lipoprotein (LDL) levels, diabetes, and ischemia have been shown to alter endothelial function (CitationLuscher et al 1993). Both the barrier and secretory function of endothelial cells are perturbed in atherosclerosis, aiding in plaque formation. This also manifests as abnormal vasoreactivity in affected vessels, suggesting a predisposition to ischemic insult (CitationBogaty et al 1994).
The current literature collectively demonstrates various mechanisms leading to autoregulatory dysfunction in atherosclerotic vessels. However, a corollary correlation between atherosclerosis and OAG has yet to be identified. The prospective Rotterdam eye study found neither atherosclerosis nor serum C-reactive protein (a marker of inflammation associated with atherosclerosis) to be important risk factors for the development of OAG (Citationde Voogd et al 2006). This is consistent with previous studies which were unable to find a significant relationship between atherosclerosis and OAG (CitationLevene 1980; CitationHayreh 1993; CitationGasser 1998). Conversely, CitationHayreh (1999) noted that serotonin, a biomarker released during platelet aggregation on an atherosclerotic plaque, produced vasospasm of the central retinal and short posterior ciliary arteries in atherosclerotic monkeys. While lacking a control group and not performed in human subjects, Hayreh cites previous reports suggesting improvement of serotonin induced vasoconstriction after abstaining from an atherogenic diet for several months. Further, a recent study found over-expression of a specific subset of the phospholipase A2 enzyme family, a marker for atherosclerosis, in the ocular tissue of OAG patients (CitationRonkko et al 2007). However, this result may point more to the role of oxidative stress than atherosclerosis in OAG disease pathogenesis.
It is important to note that noninvasive measurements of atherosclerosis are of limited utility. The methods used in previous studies included echography or radiography of the carotid arteries to quantify intimal thickness, abdominal imaging to measure calcification in the aorta and ankle-brachial index (Citationde Voogd et al 2006). These techniques are intended to provide in vivo quantification of systemic atherosclerosis, but measure only isolated vascular beds. Further, no study directly visualized the ocular circulation in humans, indicating a need for additional studies to accurately identify localized atherosclerosis and resulting changes in the vasculature of OAG patients.
Since atherosclerosis has a known association with other vascular risk factors such as aging, cerebrovascular and cardiovascular disease, several studies have attempted to discern a correlation between these factors and glaucoma. A recent report from the EMGT indicated cardiovascular disease history is a newly defined predictor of glaucomatous disease progression (CitationLeske et al 2007). As a systemic process, atherosclerosis plays a causative role in such pathology as myocardial infarction and cerebral vascular accidents. Several studies have reported an increased incidence of silent myocardial ischemia in glaucoma patients (CitationKaiser et al 1993; CitationWaldmann et al 1996), while multiple reports link cerebral ischemia and infarct with glaucoma (CitationStroman et al 1995; CitationOng et al 1995; CitationTutaj et al 2004). Further, the incidence of OAG, atherosclerosis, and ocular ischemia are known to increase with age (CitationHarris et al 2000). With advancing age, there is a decrease in the relative capillary blood flow, velocity, and volume within the neuroretinal rim and lamina cribrosa of the ONH (CitationBoehm et al 2005). Although multiple factors likely contribute to this process, it has been determined that retinal arteriolar and venular diameters narrow during normal aging due to age-associated vascular rigidity and atherosclerosis (CitationLeung et al 2003). This prevents the elderly population from having the same capacity to alter vessel diameter and thus blood flow as a function of autoregulation (CitationWong et al 2003). This may help explain the observation that endothelial function of the retinal vasculature improves with angiotension AT1 receptor blockade in young patients, but shows no response in older individuals (CitationDelles and Schmieder 2004; CitationOehmer et al 2006).
In summary, atherosclerosis has been demonstrated to cause autoregulatory dysfunction in multiple vascular beds resulting in significant vascular disease pathologies. Although a relationship between certain atherosclerotic risk factors and OAG has been reported, the direct influence of atherosclerosis on the progression of glaucoma is undetermined. More research is required to understand how atherosclerosis, cardiovascular disease, age, and blood flow autoregulation are inter-related in OAG disease pathophysiology.
Vasospasm
Vasospasm is characterized as a sharp, exaggerated, and often persistent contraction of a blood vessel, resulting in a reduction in luminal diameter and respective blood flow (CitationMchedlishvili 1981). This vasoconstriction or insufficient dilatation of the microcirculation may lead to inadequate blood flow to the surrounding tissue and consequent ischemia. Simultaneous arterial or venous dilatations in neighboring vascular beds may be a by-product of vascular dysregulation in vasospastic individuals (CitationFlammer 1998). Certain organs, such as the heart, brain, and eye produce clinically apparent spasms more regularly than other organ systems.
Vasospasm has been associated as a secondary finding in multiple disease states including multiple sclerosis, lupus erythematosus, antiphospholipid syndrome, rheumatoid arthritis, giant cell arthritis, Behcet’s disease, Crohn’s disease, Buerger’s disease, Raynaud’s disease, prinzmetal’s angina, preeclampsia, and AIDS (CitationGasser 1991; CitationFlammer et al 2001). However, a primary vasospastic syndrome has also been discovered wherein patients without an underlying disease develop vasospasm in response to stimuli such as cold or emotional stress (CitationGasser et al 1990). This disorder is of particular interest since the most commonly reported organ involving vasospasm is the eye (CitationGasser 1991). This syndrome is found more frequently in women than men (CitationFlammer and Prunte 1991), which possibly correlates with the observation that normal tension glaucoma (NTG) is twice as common in women. Furthermore, the female gender is considered an independent risk factor for visual field loss progression in NTG (CitationOrgul et al 1994; CitationDrance et al 2001). The increased susceptibility in females may be due to falling estrogen levels, which are known to have a vasoprotective effect and are reduced post-menopause (CitationSiesky et al 2008). Similarly, vasospasm is also observed more commonly in Japanese than Caucasian patients and correspondingly, NTG is found more commonly in Japan than most other countries (CitationBeltrame et al 1999; CitationFlammer et al 2001). These and various other studies suggest that vasospasm may exemplify a specific mechanism by which glaucomatous changes can occur (CitationKaiser et al 1993a; CitationNicolela et al 1996; CitationGasser 1998; CitationAnderson et al 1999; CitationFlammer et al 2001). Vasospasm can create an environment of blood flow dysregulation which increases the vulnerability of the ONH to vascular challenges, leading to perfusion instability, ischemic changes, axonal loss, and changes of the ONH.
The most prevalent clinical signs and symptoms of vasospasm are cold hands and feet, low blood pressure, and migraine (CitationGasser 1989, Citation1998; CitationGasser et al 1990; CitationOrgul et al 1995b; CitationFlammer et al 2001). A systematic diagnosis of vasospasm syndrome has yet to be defined, but it has been suggested that finger or nail blood flow response to cold provocation can be diagnostic (Drance et al 1998; CitationGasser and Flammer 1991). It is important to note that patients with glaucoma who have been shown to have evidence of vasospasm were more likely to demonstrate worsening of visual fields following cooling than nonvasospastic patients (CitationNicolela et al 2003). This suggests that vasospasm may manifest itself in clinically relevant glaucomatous deterioration in certain individuals. Migraine, often characterized as a vasospastic disorder, is more commonly found in females and has a well documented association with NTG (CitationCursiefen et al 2000). It has been demonstrated as a risk factor for the progression of visual field loss, suggesting a common underlying vascular pathology for both conditions (CitationMcKendrick et al 2000). Also frequently associated with vasospasm, low blood pressure is a reported risk factor for glaucoma, but if systemic hypotension is secondary to vasospasm or an unrelated phenomenon remains uncertain (CitationKaiser et al 1993b; CitationGass et al 1997; CitationGasser 1998; CitationPache et al 2003). It has been shown that blood pressure variations away from normal physiologic nocturnal dips are correlated with vasospasm, OAG, and NTG (CitationWerne et al 2008). The failure of ocular blood flow to remain constant during physiological challenges may manifest itself as ocular vasospasm or represent primary vascular dysregulation during vasospastic episodes.
Vasospasm is a transient phenomenon that is potentially reversible, allowing the restoration of steady-state perfusion. Due to its time-dependent nature, baseline blood flow measurements may not demonstrate any abnormalities compared with controls (CitationGugleta et al 2003). Therefore, the use of a provocation test to unmask underlying vascular dysregulation has been proposed in ocular hemodynamic measurements (CitationGugleta et al 2005). Studies have been conducted to demonstrate this reversibility. Increased visual field deficits have been demonstrated after cold water provocation in vasospastic patients that decreased with the administration of calcium channel blockers or magnesium (CitationGasser et al 1990; CitationGasser and Flammer 1990; CitationGaspar et al 1995). Likewise, treatment with calcium channel blockers has been shown to improve retrobulbar hemodynamics in some patients with NTG (CitationHarris et al 1997). In addition to calcium channel blockers, hyperoxia and elevated carbon dioxide (CO2) breathing are sometimes utilized to assess vascular reactivity. While studies investigating the retinal circulatory response to blood gas perturbations report conflicting results (CitationGugleta et al 2003), reports consistently indicate CO2 inhalation provides a reversible improvement in visual field defects and a decrease in the resistive indices of orbital vessels (CitationWilson et al 1997; CitationNiwa et al 2000). Specifically, CitationHarris and colleagues (1994) showed that NTG patients had significantly lower ophthalmic artery blood flow velocities and higher vascular resistance than controls. The differences between NTG and controls were reversed during elevated CO2 breathing, suggesting the presence of a reversible vasospasm in the NTG cohort (CitationHarris et al 1994). A study by CitationGugleta and colleagues (2005) found women with vasospasm demonstrated an inverse response pattern of choroidal and ONH blood gas perturbations compared with women without vasospasm. The authors suggest that vasospastic patients may have diminished autoregulatory capacity or increased responsiveness to CO2 in certain vascular beds. A differentiation of normal and abnormal blood flow regulation during blood gas perturbations and calcium channel blockers may demonstrate which persons are at greatest risk for vasospasm, impaired ocular blood flow and possibly OAG.
The current body of literature suggests that vasospasm may play a more significant role in OAG ONH damage than atherosclerosis. Although both conditions have been shown to contribute to systemic autoregulatory dysfunction, it is hypothesized that most patients with increased vascular resistance secondary to atherosclerotic change compensate with proficient autoregulation in small arterioles and capillaries (CitationFlammer et al 1999). Intracranial vessels seem more resistant to the development of atherosclerosis in comparison to other vascular beds (CitationFaraci and Heistadt 1998). While effects of atherosclerosis are readily apparent on fundoscopic exam, relying on these changes for measure of systemic and retinal disease may be of limited utility (CitationHelvaci et al 2007). It is believed that perfusion fluctuates more dramatically in vasospastic patients, which can additionally lead to reperfusion damage (CitationSchempp and Elstner 1998).
The concept of vasospasm-induced reperfusion injury is heavily emphasized in other fields of research including vascular and transplant surgery (CitationJokuszies et al 2006), neurology (CitationReid et al 1995), and cardiology (CitationLaude et al 2004). During myocardial ischemia and infarction, for example, reperfusion is characterized by the overproduction of oxygen-derived free radicals that spurn an acute inflammatory response and tissue degradation. It has also been argued that reperfusion injury alters the endothelium architecture, allowing for such adverse conditions as vasospasm and atherosclerosis to develop more readily (CitationLaude et al 2004).
In summary, vasospasm is associated with multiple disease states including OAG with NTG patients and women appearing to be the most at risk for vasopastic contributions to disease processes. Vasospasm may result in fluctuations in ocular perfusion, ischemia, and/or reperfusion injury to the ocular tissues. Importantly, vasospasm may be reversible (treatable). The capacity of diagnostic testing for OAG risk using blood gas perturbations should be further explored, especially in patients that demonstrate a vasospastic propensity.
Endothelial dysfunction
Endothelial dysfunction has been increasingly implicated in OAG disease pathophysiology. Disruption of the homeostatic factors maintaining physiologic vessel caliber may shift the balance toward an excess of vasoconstriction over vasodilation resulting in vasospasm (CitationDelaney et al 2006). Similarly, atherosclerosis changes endothelial architecture and disrupts mechanisms contributing to endothelium-derived vascular relaxation (CitationFaraci and Heistadt 1998). A causative and/or synergistic role involving atherosclerosis, vasospasm, and endothelial dysfunction may result in dysfunctional regulation of the ocular vasculature potentially contributing to OAG pathophysiology.
The vascular endothelium regulates the microcirculation through release of vasoactive factors, the most potent of which are the vasodilator nitric oxide (NO) and the vasoconstrictor endothelin-1 (ET-1) (CitationAdams 2006). Additional endothelium-derived activating compounds include prostacyclins, acetylcholine, bradykinin, and histamine (CitationFlammer and Orgul 1998; CitationAdams 2006). In endothelial cells, NO synthase (NOS) catalyzes the conversion of L-arginine to NO and L-citrulline. NO released from endothelial cells directly stimulates the surrounding vascular smooth muscle to promote vasodilation (CitationToda and Nakanishi-Toda 2007). Systemic factors such as hyperlipidemia, atherosclerosis, and hyperglycemia impair endothelial NO signaling through oxidative stress damage (CitationNapoli et al 2001). Plasma levels of asymmetric dimethylarginine, an endogenous inhibitor of NOS, are disproportionately elevated in hyperlipidemia, hyperhomocystinemia, atherosclerosis, coronary artery disease, hyperglycemia, and other disorders affected by endothelial dysfunction (CitationMiyazaki et al 1999; CitationDelaney et al 2006). Conversely, infusions of L-arginine, a precursor molecule to NO, limit injury from ischemia, and reperfusion-induced endothelial dysfunction (CitationPernow et al 2003).
It is known that NO activity contributes to ocular auto-regulation and can protect the endothelium and nerve fiber layer against pathologic stressors implicated in glaucoma, ischemia, and diabetes (CitationToda and Nakanishi-Toda 2007). A recent report by CitationPolak and colleagues (2007) presents the first in vivo evidence for altered ocular L-arginine/NO pathway in glaucoma patients. Polymorphisms in the endothelium specific NOS (eNOS) have been found in familial patients with glaucoma (CitationTunny et al 1998). A preliminary study by Wiggs and colleagues also suggests the presence of a polymorphism in the eNOS gene in patients with high and NTG that predisposes these patients to superior paracentral field loss (CitationDelaney et al 2006). Decreased levels of cyclic GMP, an indicator of NO activity, have been demonstrated in the plasma and aqueous humor of glaucoma patients, with corresponding lower systolic and diastolic ophthalmic artery blood flow velocities of NTG patients (CitationLaude et al 2004).
Opposing the vasodilation properties of NO is ET-1, the most important and potent vascular constricting factor. The peptide is synthesized by endothelial cells and either binds to the ETA receptor on smooth muscle cells to mediate vasoconstriction or binds to the ETB receptor on endothelial cells to mediate vasodilation via the autocrine release of NO, prostacyclin and by increasing removal of endothelin from the circulation. () Although this indicates ET-1 can stimulate either vasoconstriction or vasodilation, the overall response in vivo is sustained and powerful vasoconstriction (CitationRubanyi and Polokoff 1994). There is evidence that enhanced ET-1 vasoconstriction is involved in the pathology of such conditions as hypertension, heart failure, myocardial infarction, renal failure, and secondary vasospastic disorders (CitationOrtega and de Artinano 1997).
Figure 2 A schematic diagram of the known effects of endothelin-1 on the endothelium and smooth muscle. The dashed line indicates an inhibitory effect. Abbreviations: ET-1, endothelin-1; NO, nitric oxide; PC, prostacyclin; ETA, endothelin-A receptor, ETB, endothelin-B receptor.
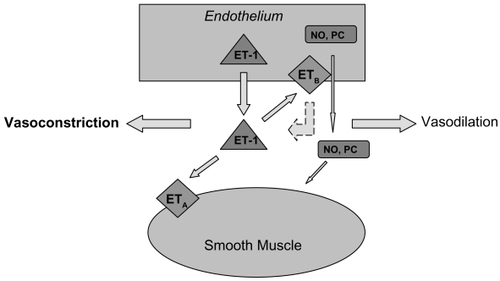
A number of studies have investigated the role of ET-1 in glaucoma. ET-1 has been shown to decrease blood flow to the anterior optic nerve when directly applied to this region (CitationOrgul et al 1996). It has been determined that ET-1 provokes forceful contractions of isolated ophthalmic arteries with smaller vessels expressing greater ET-1 sensitivity (CitationHaefliger et al 1992; CitationPolak et al 2003). Utilizing arteries dissected from gluteal fat biopsies, CitationBuckley and colleagues (2002) identified dysfunction of the systemic vascular endothelial cell in patients with NTG by demonstrating decreased responsiveness to ET-1 stimulation. Alterations in the vascular responsiveness to ET-1 or changes in synthesis, activation, and removal of the molecule may therefore result in impaired regulation of ocular blood flow. Several studies have demonstrated increased plasma ET-1 levels in NTG patients (CitationSugiyama et al 1995; CitationKaiser et al 1995), but these results have not been reproduced in patients with high tension glaucoma (HTG) (CitationTezel et al 1997). Aqueous humor levels of ET-1 are reportedly elevated in HTG (CitationNoske et al 1997) and one study demonstrated a significant elevation of plasma ET-1 in HTG when the subjects were moved from the supine to upright position (Kaiser et al 1997). To further support a role for vasospasm in endothelial dysfunction, CitationNicolela and colleagues (2003) reported an abnormal increase in plasma ET-1 in response to body cooling in OAG patients. This suggests a hyperactive production and/or release of ET-1 in response to vasospastic stimuli in predisposed patients (CitationNicolela et al 2003).
Several recent studies have shifted the focus from solely examining ET-1 expression to investigating the role of ETA and ETB receptors in ocular blood flow. In studies of porcine eyes, ET-1 increases ocular blood flow at negligible doses and reduces it at higher ones, which is thought to occur secondary to activation of ETB and ETA receptors, respectively (CitationYao et al 1991). This suggests that low ET-1 doses have a greater affinity for ETB receptors to cause vasodilation, but increased levels of ET-1 leads to vasoconstriction by binding to ETA receptors. Blocking ETA receptors to the exogenous administration of ET-1 in humans has been shown to reverse the observed reduction in retinal blood flow, further indicating that ETA receptors mediate the vasoconstrictive effects of ET-1 in the retina (CitationSugiyama et al 1995). In patients with NTG, Henry et al reported normal vasoreactivity to ET-1, but significantly decreased vasodilation in response to ETA receptor antagonism. The authors suggest this response may be due to a combination of diminished ETA receptor-mediated tone, increased ETB receptor mediated contraction, or impaired release of NO (CitationHenry et al 2006). Correspondingly, polymorphisms of the ETA receptor have been associated with NTG (CitationKim et al 2006).
ETB receptor polymorphisms, however, have yet to show any significant association with systemic or ocular disease (CitationDelaney et al 2006). The role of ETB in vascular physiology is shown to be selectively dependent on the tissue bed. It has been demonstrated in both rat and monkey models of sub-arachnoid hemorrhage that increased smooth muscle cell ETB receptor expression induces vasospasm of cerebral vessels (CitationHino et al 1996; CitationHansen-Schwartz et al 2003). Conversely, the function of ETB in human skin seems insignificant (CitationLipa et al 1999). The role of ETB in ocular hemodynamics is yet to be determined, but similar to other vascular beds in the body, it is likely influenced by the proportion of endothelial to smooth muscle cells and corresponding ETA/ETB ratio (CitationDelaney et al 2006).
In addition to directly reducing ocular blood flow, ET-1 increases local vascular sensitivity to other vasoconstrictive elements such as angiotensin II and catecholamines (CitationSchmetterer 1997). CitationGrieshaber and Flammer (2005) suggest that the elevation of ET-1 in glaucoma represents an epiphenomenon rather than a distinctive mechanism. They point to the observation of significantly elevated ET-1 levels in vasospastic and systemic diseases such as multiple sclerosis, rheumatoid arthritis, fibromyalgia, and Susac syndrome. Although a pale ONH is often associated with these diseases, glaucoma does not occur more frequently in these patients than in the average population. This suggests that ET-1 upregulation may be secondary to repetitive perfusion insufficiency and damage occurring not only in the ONH, but also subclinically in multiple organs (CitationWaldmann et al 1996; CitationGrieshaber and Flammer 2005). Although animal models have suggested that increased levels of ET-1 by itself can lead to glaucoma via ischemia (CitationCioffi et al 2004), these same results have not been demonstrated in human population studies.
In summary endothelial dysfunction is likely related to glaucoma, but the exact role of mediators such as NO, NOS, ET-1, and ETA/ETB in OAG pathophysiology has yet to be fully appreciated. Endothelial dysfunction may therefore be primary or secondary to vascular diseases including atherosclerosis and vasospasm in its contribution to OAG pathology.
Blood pressure changes and blood flow
Fluctuations in IOP, blood pressure, ocular perfusion pressure, and thus blood flow are implicated in OAG. A patient with vasospastic syndrome may not be able to compensate for elevations of IOP and/or blood pressure dips, while a healthy patient can autoregulate to maintain consistent ocular perfusion. While not detailed in this review, several studies have found a modest association between OAG and hypertension (CitationTielsch et al 1995; CitationBonomi et al 2000; CitationMitchel 2004), while others found no significant relationships (CitationLeske et al 1996, Citation2008). CitationChoi and colleagues (2007) investigated 24-hour IOP, blood pressure, and perfusion pressure changes associated with clinically relevant visual field outcomes. The study determined that both anatomic (retinal nerve fiber layer thickness) and functional (visual field) outcome variables were significantly worse in glaucoma patients with wider circadian perfusion pressure fluctuations. The authors attributed glaucoma progression to daily repetitive ischemic insults followed by reperfusion injury in eyes through dysfunctional regulation mechanisms that could not maintain consistent perfusion pressure (CitationChoi et al 2007). In the Thessaloniki Eye Study, patients without glaucoma having a diastolic blood pressure of <90 mm Hg with antihypertensive treatment was associated with increased cupping and decreased rim area of the optic disk (CitationTopouzis et al 2006). A recent report from the European Glaucoma Prevention Study (EGPS) indicates that systemic diuretic use was associated with the development of OAG (CitationMiglior et al 2007). This may be due to the inability of the eye to maintain consistent, adequate perfusion during pharmacologically lowered blood pressure in susceptible individuals. A detailed review of fluctuations in blood pressure, IOP, ocular perfusion pressure and blood flow are covered in great detail elsewhere (CitationWerne et al 2008).
Diabetes and glaucoma
Other vascular diseases, such as diabetes, have conflicting reports about a potential role in OAG pathology (CitationLeske et al 2007), although a significant correlation with diabetes was determined by a meta-analysis (CitationBonovas et al 2004). Diabetes, a disease with many vascular complications, has been reported to be related to glaucoma, however the evidence for diabetes as a contributing factor in OAG pathology is currently lacking. Population-based studies, such as the Baltimore eye survey, the Barbados eye study, and the Rotterdam study failed to support an association between diabetes and glaucoma. The relationship with other vascular risk factors are even less well defined, with a previous review indicating no consensus between OAG and smoking, hypercholesterolemia, or body mass index (CitationFlammer et al 1999).
The role of other vascular diseases in glaucoma remains unclear. How each disease is related to ocular vascular regulation, or lack thereof, is uncertain. It is possible that understanding how OAG patients react differently to physiological challenges than healthy controls may reveal the differences reported in the literature. It is also possible that diabetes and hypercholesterolemia, while vascular in nature, do not directly influence ocular vascular regulation in a capacity that contributes to OAG.
Clinical and therapeutic implications
With evidence supporting vascular risk factors in glaucomatous optic neuropathy, the medical community may choose to modify the focus of OAG disease characterization and management. Certain patients with glaucoma demonstrate vascular regulatory dysfunction. Physiologic phenomenons, such as circadian variations of IOP, ocular perfusion pressure, and ocular blood flow are generating increasing evidence for their role in ocular ischemia and OAG disease processes (CitationWerne et al 2008). Systemic medications such as antihypertensives have been shown to exacerbate OAG changes, indicating a need for heightened awareness of medication regimens in the glaucoma patient population (CitationNetland et al 1997).
To screen for a primary vasospastic disorder, a recent review recommends practitioners include questions regarding a history of cold hands/feet, migraine or hypotension in patients (CitationDelaney et al 2006). A thorough assessment of vascular risk factors including a history of cardiovascular disease may assist in predicting disease progression and in improving management in identified patients (CitationLeske et al 2007).
Several vascular pharmacological targets have been identified in preliminary therapeutic trials for vascular dysfunctional regulation. Calcium channel blockers have been shown to improve outcomes in vasospastic-induced secondary ischemia in patients with subarachnoid hemorrhage (CitationMees et al 2007) and intractable migraine (CitationNg et al 2000). Authors have demonstrated calcium channel blocker-induced relief of coronary spasm following coronary artery bypass grafting (CitationCaputo et al 1999), with one study suggesting injection of calcium blockers whenever there is reason to suspect vasospasm in arterial grafts (CitationHe et al 2000). In ocular ischemia, calcium channel antagonists can be indicated in the treatment of the ocular ischemia syndrome and amaurosis fugax (CitationWinterkorn et al 1993; CitationWinterkorn and Beckman 1995). Similarly, calcium channel blockers may reduce ocular vasospasm in certain glaucoma patients (CitationHarris et al 1997; CitationFlammer et al 1999). Several studies show that calcium channel antagonists reduce the effect of increased levels of ET-1 on ocular perfusion and improve visual field defects through induced vasodilation (CitationFlammer et al 2001). Magnesium also reduces ET-1-induced vasospasm and although it is actively being pursued in the management of subarachnoid hemorrhage, its usefulness in glaucoma is currently unknown (CitationDettmann et al 1998; CitationMees et al 2007). Blood gas changes, including elevated CO2 breathing may also be used to test for vasospastic tendency and/or reversibility (CitationHarris et al 1994).
The contribution of NO in the treatment of vascular dysfunctional regulation is becoming increasingly apparent. Investigations into cerebral vasospasm following cerebral vascular insults have demonstrated reduced vasospasm and ischemia-reperfusion injury with nitrate infusion (CitationPluta et al 2005; CitationJung et al 2006). The stroke literature has recently highlighted a role for initiating or continuing statin therapy in patients with vasospasm and ischemia-reperfusion injury (CitationEndres and Laufs 2006; CitationTrimble and Kockler 2007). These medications are thought to produce a local effect through upregulation and activation of eNOS to increase NO production (CitationTseng et al 2005). NO has also been shown to protect the liver and heart against reperfusion injury, with one report suggesting a role for daily dietary nitrite and nitrate therapy for optimal cardiovascular health (CitationBryan et al 2007). Studies concerning ocular health have demonstrated that systemic nitroglycerin preparations have a protective effect against optic neuropathy in glaucoma patients taking the medication for nonophthalmic reasons (CitationZurakowski et al 1998). Acute intravenous and oral administration of nitroglycerine lowers IOP in both glaucoma and healthy patients, with a transient increase in ocular blood flow (CitationWizemann and Wizemann 1980; CitationSinclair et al 1993). The L-arginine/NO/cyclic GMP pathway has also been implicated in the IOP decrease (CitationKanno et al 2000). Chronic exposure to NO may actually contribute to glaucomatous progression (CitationToda and Nakanishi-Toda 2007), indicating a potential role for NOS inhibition or a potential for downregulation or insensitivity. For example, inducible NOS (iNOS) is expressed only during inflammation and other pathological responses resulting in abundant NO production and eventual retinal ganglion cell loss (CitationToda and Nakanishi-Toda 2007). Animal trials of iNOS selective inhibition have demonstrated significantly lower retinal ganglion cell loss in treated versus untreated subjects (CitationNeufeld et al 1999). It is therefore recommended that future efforts continue to elucidate the role of NO and its use in potential therapeutic interventions.
In a similar fashion, the known effect of endothelin on systemic and ocular circulation has led to a number of studies investigating potential therapeutic strategies. The pharmaceutical industry has recognized the importance of this biomolecule and an increasing number of ETA specific antagonists are undergoing trials for the management of hypertension (CitationD’Orleans-Juste et al 2002). Preliminary studies also suggest this selective antagonism maintains endothelin’s role in vascular relaxation (CitationTakase et al 1995). Endothelin mediated pathology has been demonstrated in the ocular circulation, although application of this knowledge to disease therapy is currently limited.
Conclusion
Glaucoma is a comprehensive term for a heterogeneous disease comprising multiple etiologies. Alterations in ocular blood flow have become increasingly implicated in OAG disease pathology. The current body of literature suggests several possible mechanisms may be involved in the vascular regulatory dysfunction of OAG patients, although an inclusive appreciation from the molecular level to the clinically apparent is incomplete. Generalized vascular diseases, including endothelial dysfunction, atherosclerosis, and vasospasm may contribute to OAG disease pathophysiology. Patients suffering from vascular impairment, and therefore, faulty regulation of blood flow may be unable to compensate for physiologic fluctuations in IOP and blood pressure to maintain ocular perfusion pressures. This may result in chronic, intermittent ischemic and reperfusion damage to the ONH, which contributes to glaucomatous optic neuropathy. Continuing research is needed to discern the significance of the vascular factors presented in this text, along with others not mentioned or unknown. Long-term multicenter clinical trials investigating the relationship between vascular dysregulation in glaucoma patients and nonclinical outcomes such as visual function and optic nerve changes are necessary.
Acknowledgments
This project was supported in part by an unrestricted research grant from Research to Prevent Blindness, New York NY. The authors report no other conflicts of interest.
References
- AdamsJA2006ndothelium and cardiopulmonary resuscitationCrit Care Med34Suppl 12S4586517114978
- AnderssonPO1984Vascular control in the colon and rectumScand J Gastroenterol Suppl9365786374876
- AndersonDR1999Introductory comments on blood flow autoregulation in the optic nerve head and vascular risk factors in glaucomaSurv Ophthalmol43Suppl 1S5910416742
- BehrendtDGanzP2002Endothelial function: from vascular biology to clinical applicationsAm J Cardiol9040L48L
- BeltrameJFSasayamaSMaseriA1999Racial heterogeneity in coronary artery vasomotor reactivity: differences between Japanese and Caucasian patientsJ Am Coll Cardiol3314425210334407
- BoehmAGKoellerAUPillunatLE2005The effect of age on optic nerve head blood flowInvest Ophthalmol Vis Sci461291515790893
- BogatyPHackettDDaviesG1994Vasoreactivity of the culprit lesion in unstable anginaCirculation905118026037
- BonomiLMarchiniGMarraffaM2000Vascular risk factors for primary open angle glaucoma: The Egna-Neumarkt StudyOphthalmology10712879310889099
- BonovasSPeponisVFilioussiK2004Diabetes mellitus as a risk factor for primary open-angle glaucoma: a meta-analysisDiabet Med216091415154948
- BryanNSCalvertJWElrodJW2007Dietary nitrite supplementation protects against myocardial ischemia-reperfusion injuryPNAS10419144918025468
- BuckleyCHadokePWHenryE2002Systemic vascular endothelial cell dysfunction in normal pressure glaucomaBr J Ophthalmol862273211815352
- CaputoMNicoliniFFranciosiG1999Coronary artery spasm after coronary artery bypass graftingEur J Cardiothorac Surg15545810371140
- ChoiJKimKHJeongJ2007Circadian fluctuation of mean ocular perfusion pressure is a consistent risk factor for normal-tension glaucomaInvest Ophthalmol Vis Sci481041117197523
- CioffiGAWangLFortuneB2004Chronic ischemia induces regional axonal damage in experimental primate optic neuropathyArch Ophthalmol12215172515477464
- CursiefenCWisseMCursiefenS2000Migraine and tension headache in high-pressure and normal-pressure glaucomaAm J Ophthalmol129102410653426
- DaviesMJ1990A macro and micro view of coronary vascular insult in ischemic heart diseaseCirculation82Suppl 3II38462203563
- DaviesPFTripathiSC1993Mechanical stress mechanisms and the cell: an endothelial paradigmCirc Res72239458418981
- DaviesJRRuddJHWeissbergPL2004Molecular and metabolic imaging of atherosclerosisJ Nucl Med45189890715534061
- DelaneyVWalsheTEO’BrienC2006Vasospasm in glaucoma: clinical and laboratory aspectsOptom Vis Sci834061416840865
- DellesCSchmiederRE2004Renal endothelial effects of antihypertensive therapyCurr Opin Nephrol Hypertens134899315300153
- DettmannESLuscherTFFlammerJ1998Modulation of endothelin-1-induced contractions by magnesium/calcium in porcine ciliary arteriesGraefes Arch Clin Ophthalmol2364751
- de VoogdSWolfsRCJansoniusNM2006Atherosclerosis, c-reactive protein, and risk for open-angle glaucoma: the Rotterdam studyInvest Ophthalmol Vis Sci473772616936085
- D’Orleans-JustePLabonteJBkailyG2002Function of the endothelin (B) receptor in cardiovascular physiology and pathophysiologyPharmacol Ther952213812243796
- DranceSMDouglasGRWijsmanK1988Response of blood flow to warm and cold in normal and low-tension glaucoma patientsAm J Ophthalmol1053593337192
- DranceSAndersonDRSchulzerM2001Risk factors for progression of visual field abnormalities in normal-tension glaucomaAm J Ophthalmol13169970811384564
- EndresMLaufsU2006Discontinuation of statin treatment in stroke patientsStroke372640316946153
- FaraciFMHeistadtDD1998Regulation of the cerebral circulation: role of endothelium and potassium channelsPhysiol Rev7853979457169
- FlammerJPrunteC1991Ocular vasospasm. I: Functional circulatory disorders in the visual system, a working hypothesisKlin Monatsbl Augenheilkd198411121886371
- FlammerJHaefligerIOFlammerJ1998The concept of vascular dysregulation in glaucomaNitric oxide and endothelin in the pathogenesis of glaucomaPhiladelphiaLippincott-Raven1421
- FlammerJOrgulS1998Optic nerve blood-flow abnormalities in glaucomaProg Retin Eye Res17267899695795
- FlammerJHaefligerIOOrgulS1999Vascular dysregulation: a principal risk factor for glaucomatous damage?J Glaucoma82121910376264
- FlammerJPacheMResinkT2001Vasospasm, its role in the pathogenesis of diseases with particular reference to the eyeProg Retin Eye Res203194911286896
- FunkRH1997Blood supply of the retinaOphthalmic Res2932059323723
- GalassiFRenieriGSodiA2004Nitric oxide proxies and ocular perfusion pressure in primary open angle glaucomaBr J Ophthalmol887576015148207
- GasparAZGasserPFlammerJ1995The influence of magnesium on visual field and peripheral vasospasm in glaucomaOphthalmologica20911137715920
- GassAFlammerJLinderL1997Inverse correlation between endothelin-1-induced peripheral microvascular vasoconstriction and blood pressure in glaucoma patientsGraefes Arch Clin Exp Ophthalmol23563489349947
- GasserP1989Ocular vasospasm: a risk factor in the pathogenesis of low-tension glaucomaInt Ophthalmol13281902693385
- GasserPFlammerJGuthauserU1990Do vasospasms provoke ocular diseases?Angiography4121320
- GasserPFlammerJ1990Short-and long-term effect of nifedipine on the visual field in patients with presumed vasospasmJ Int Med Res1833492227081
- GasserP1991Clinical syndromes with vasoconstrictor responseWien Klin Wochenschr103217211858387
- GasserPFlammerJ1991Blood-cell velocity in the nailfold capillaries of patients with normal-tension and high-tension glaucomaAm J Ophthalmol11158582021167
- GasserP1998Why study vascular factors in glaucoma?Int Ophthalmol22221510674866
- GrieshaberMCFlammerJ2005Blood flow in glaucomaCurr Opin Ophthalmol16798315744136
- GugletaKOrgulSHaslerPW2003Choroidal vascular reaction to hand-grip stress in subjects with vasospasm and its relevance in glaucomaInvest Ophthalmol Vis Sci4415738012657594
- GugletaKOrgulSHaslerP2005Circulatory response to blood gas pertubations in vasospasmInvest Ophthalmol Vis Sci4632889416123431
- HaefligerIOFlammerJLuscherTF1992Nitric oxide and endothelin-1 are important regulators of human ophthalmic arteryInvest Ophthalmol Vis Sci33234031607246
- HaggendalENilssonNJNorbackB1969Aspects of the autoregulation of cerebral blood flowInt Anesthesiol Clin7353674911052
- Hansen-SchwartzJHoelNLZhouM2003Subarachnoid hemorrhage enhances endothelin receptor expression and function in rat cerebral arteriesNeurosurgery521188941194512699564
- HarrisASergottRCSpaethGL1994Color Doppler analysis of ocular vessel blood velocity in normal tension glaucomaAm J Ophthalmol11864297977577
- HarrisAEvansDWCantorLB1997Hemodynamic and visual field function effects of oral nifedipine in patients with normal-tension glaucomaAm J Ophthalmol1242963029439355
- HarrisACiullaTAChungHS1998Regulation of retinal and optic nerve blood flowArch Ophthalmolol11614915
- HarrisAHarrisMBillerJ2000Aging affects the retrobulbar circulation differently in females and malesArch Ophthalmol11810768010922201
- HarrisAJonescu-CuypersCPKagemannL2003Atlas of Ocular Blood Flow: Vascular anatomy, pathophysiology, and metabolismPhiladelphiaButterworth Heinemann
- HayrehSSRevieIHEdwardsJ1970Vasogenic origin of visual field defects and optic nerve changes in glaucomaBr J Ophthalmol5446725417654
- HayrehSS1993The role of age and cardiovascular disease in glaucomatous optic neuropathySurv Ophthalmol43Suppl 1S27S4210416745
- HayrehSS1999Retinal and optic nerve head ischemic disorders and atherosclerosis: role of serotoninProg Retin Eye Res181912219932283
- HayrehSS2001Blood flow in the optic nerve head and factors that may influence itProg Retin Eye Res2059562411470452
- HeGWFanKYChiuSW2000Injection of vasodilators into arterial grafts through cardiac catheter to relieve spasmAnn Thorac Surg69625810735717
- HeijlALeskeMCBengtssonB2002Early Manifest Glaucoma Trial Group. Reduction of intraocular pressure and glaucoma progression: results from the Early Manifest Glaucoma TrialArch Ophthalmol12012687912365904
- HelvaciMROzcuraFKayaH2007Funduscopic examination has limited benefit for management of hypertensionInt Heart J481879417409584
- HenryENewbyDEWebbDJ2006Altered endothelin-1 vasore-activity in patients with untreated normal-pressure glaucomaInvest Ophthalmol Vis Sci4725283216723466
- HinoATokuyamaYKobayashiM1996Increased expression of endothelin B receptor mRNA following subarachnoid hemorrhage in monkeysJ Cereb Blood Flow Metab16688978964809
- HulsmanCAVingerlingJRHofmanA2007Blood pressure, arterial stiffness and open angle glaucoma. The Rotterdam studyArch Ophthalmol1258051217562992
- JohnsonPC1986Autoregulation of blood flowCirc Resc5948395
- JokusziesANiederbichlerAMeyer-MarcottyM2006Influence of transendothelial mechanisms on microcirculation: consequences for reperfusion injury after free flap transfer. Previous, current, and future aspectsJ Reconstr Microsurg225131817048133
- JossiNAndersonDR1983Blockage of axonal transport in optic nerve induced by elevation of intraocular pressure: effect of arterial hypertension induced by angiotensin-IArch Ophthalmol1019476185108
- JungKHChuKKoSY2006Early intravenous infusion of sodium nitrite protects brain against in vivo ischemia-reperfusion injuryStroke3727445017008610
- KaiserHJFlammerJBurckhardtD1993aSilent myocardial ischemia in glaucoma patientsOphthalmologica207678278174
- KaiserHJFlammerJGrafT1993bSystemic blood pressure in glaucoma patientsGraefes Arch Clin Exp Ophthalmol231677808299973
- KaiserHJFlammerJWenkM1995Endothelin-1 plasma levels in normal-tension glaucoma: abnormal response to postural changesGraefes Arch Clin Exp Ophthalmol23348488537023
- KannoMAraieMKoibuchiH2000Effects of topical nipradilol, a beta-blocking agent with alpha-blocking and nitroglycerin-like activities, on intraocular pressure and aqueous dynamics in humansBr J Ophthalmol84293910684841
- KimSHKimJYKimDM2006Investigations on the association between normal tension glaucoma and single nucleotide polymorphisms of the endothelin-1 and endothelin receptor genesMol Vis1210162116971893
- KontosHAWeiEPRaperAJ1978Role of tissue hypoxia in local regulation of cerebral microcirculationAm J Physiol234H58291645924
- KreshJYArmourJA1997The heart as a self-regulating system: integration of homeodynamic mechanismsTechnol Health Care5159699134627
- LaudeKRichardVThuillezC2004Coronary endothelial cells: a target of ischemia reperfusion and its treatment?Arch Mal Coeur Vaiss97250415106748
- LeskeMCWarheit-RobertsLWuSY1996Open-angle glaucoma and ocular hypertension: The Long Island Glaucoma Case-Control StudyOphthalmic Epidemiol385968841060
- LeskeMC2007Open-angle glaucoma: an epidemiologic overviewOphthalmic Epidemiol141667217896292
- LeskeMCHeijlAHymanL2007Predictors of long-term progression in the early manifest glaucoma trialOphthalmology1119657217628686
- LeskeMCWuSYHennisA2008Risk factors for incident open-angle glaucoma: the Barbados Eye StudiesOphthalmology115859317629563
- LeungHWangJJRochtchinaE2003Relationships between age, blood pressure, and retinal vessel diameters in an older populationInvest Ophthalmol Vis Sci442900412824229
- LeveneRZ1980Low tension glaucoma: a critical review and new materialSurv Ophthalmol24621647414505
- LichterPRMuschDCGillespieBW2001Interin clinical outcome in the collaborative initial glaucoma treatment study comparing initial treatment randomized to medications or surgeryOphthalmology10819435311713061
- LipaJENeliganPCPerreaultTM1999Vasoconstriction effect of endothelin-1 in human skin: role of ETA and ETB receptorsAm J Physiol276H359679950834
- LiuJHZhangXKripkeDF2003Twenty-four-hour intraocular pressure pattern associated with early glaucomatous changesInvest Ophthalmol Vis Sci4415869012657596
- LuscherTFTannerFCTschudiMR1993Endothelial dysfunction in coronary artery diseaseAnn Rev Med443954188476260
- MchedlishviliG1981The nature of cerebral vasospasmBlood Vessels18311207034819
- McKendrickAMVingrysAJBadcockDR2000Visual field losses in subjects with migraine headachesInvest Ophthalmol Vis Sci4112394710752965
- MeesDSMRinkelGJFeiginVL2007Calcium antagonists for anerysmal subarachnoid haemorrhageCochrane Database Syst Rev3CD00027717636626
- MigliorSTorriVZeyenT2007Intercurrent factors associated with the development of open-angle glaucoma in the European glaucoma prevention studyAm J Ophthalmol22667517543874
- MitchelPLeeAJRochtchinaE2004Open-angle glaucoma and systemic hypertension: The Blue Mountains Eye StudyJ Glaucoma133192615226661
- MiyazakiHMatsuokaHCookeJP1999Endogenous nitric oxide synthase inhibitor: a novel marker of atherosclerosisCirculation991141610069780
- NapoliCde NigrisFPalinskiW2001Multiple roles of reactive oxygen species in the arterial wallJ Cell Biochem826748211500945
- NetlandPAWeissHSStewartWC1997Cardiovascular effects of topical carteolol hydrochloride and timolol maleate in patients with ocular hypertension and primary open-angle glaucoma. Night Study GroupAm J Ophthalmol123465779124243
- NeufeldAHSawadaABeckerB1999Inhibition of nitric-oxide synthase 2 by aminoguanidine provides neuroprotection of retinal ganglion cells in a rat model of chronic glaucomaProc Natl Acad Sci USA969944810449799
- NgTMKohliAFaganSC2000The effect of intravenous verapamil on cerebral hemodynamics in a migraine patient with hemiplegiaAnn Pharmacother34394310669185
- NicolelaMTWalmanBEBuckleyAR1996Various glaucomatous optic nerve appearances. A color Doppler imaging study of retrobulbar circulationOphthalmology103167098874441
- NicolelaMTFerrierSNMorrisonCA2003Effects of cold-induced vasospasm in glaucoma: the role of endothelin-1Invest Ophthalmol Vis Sci4425657212766058
- NiwaYYamamotoTHarrisA2000Relationship between the effect of carbon dioxide inhalation or nilvadipine on orbital blood flow in normal-tension glaucomaJ Glaucoma9262710877378
- NoskeWHensenJWiederholtM1997Endothelin-like immunoreactivity in aqueous humor of patients with primary open-angle glaucoma and cataractGraefes Arch Clin Exp Ophthalmol23555129342603
- O’BrienEMurphyJTyndallA1991Twenty-four-hour ambulatory blood pressure in men and women aged 17 to 80 years: the allied Irish Bank StudyJ Hypertens9355601646262
- OehmerSHaraznyJDellesC2006Valsartan and retinal endothelial function in elderly hypertensive patientsBlood Press151859116864162
- OngKFarinelliABillsonF1995Comparative study of brain magnetic resonance imaging findings in patients with low-tension glaucoma and control subjectsOphthalmology02163289098255
- OrgulSGasparAZHendricksonP1994Comparison of the severity of normal-tension glaucoma in men and womenOphthalmologica20814248065698
- OrgulSMeyerPCoiffiGA1995aPhysiology of blood flow regulation and mechanisms involved in optic nerve perfusionJ Glaucoma442743
- OrgulSKaiserHJFlammerJ1995bSystemic blood pressure and capillary blood-cell velocity in glaucoma patients: a preliminary studyEur J Ophthalmol588917549448
- OrgulSCioffiGAWilsonDJ1996An endothelin-1 induced model of chronic optic nerve ischemia in the rabbitInvest Ophthalmol Vis Sci37186098759355
- OrgulSGugletaKFlammerJ1999Physiology of perfusion as it relates to the optic nerve headSurv Ophthalmol43Suppl 1S172610416744
- OrtegaMAde ArtinanoAA1997Highlights on endothelins: a reviewPharmacol Res36339519441724
- OsuskyRRohrPSchotzauA2000Nocturnal dip in the optic nerve head perfusionJpn J Ophthalmol441283110715378
- PacheMDublerBFlammerJ2003Peripheral vasospasm and nocturnal blood pressure dipping – two distinct risk factors for glaucomatous damage?Eur J Ophthalmol13260512747647
- PernowJBohmFBeltranE2003L-arginine protects from ischemia-reperfusion-induced endothelial dysfunction in humans in vivoJ Appl Physiol9522182212937030
- PlutaRMDejamAGrimesG2005Nitrite infusions to prevent delayed cerebral vasospasm in a primate model of subarachnoid hemorrhageJAMA29314778415784871
- PolakKLukschAFrankB2003Regulation of human retinal blood flow by endothelin-1Exp Eye Res766334012697427
- PolakKLukschABerishaF2007Altered nitric oxide system in patients with open-angle glaucomaArch Ophthalmol1253938
- QuigleyHABromanAT2006The number of people with glaucoma worldwide in 2010 and 2020Br J Ophthalmol90262716488940
- ReidJLDawsonDMacraeIM1995Endothelin, cerebral ischaemia and infarctionClin Exp Hypertens173994077735285
- RonkkoSRekonenPKaarnirantaK2007Phospholipase A2 in chamber angle of normal eyes and patients with primary open angle glaucoma and exfoliation glaucomaMol Vis134081717417602
- RubanyiGMPolokoffMA1994Endothelins: molecular biology, biochemistry, pharmacology, physiology, and pathophysiologyPharmacol Rev463254157831383
- SchemppHElstnerEFHaefligerIOFlammerJ1998Free radicals in the pathogenesis of ocular diseasesNitric oxide and endothelin in the pathogenesis of glaucomaPhiladelphiaLippincott-Raven11235
- SchmettererLFindlOStrennK1997Effects of endothelin-1 (ET-1) on ocular hemodynamicsCurr Eye Res16687929222086
- SieskyBHarrisAKlaasCL2008Comparison of visual function and ocular hemodynamics between pre- and post-menopausal womenEur J Ophthalmol18320318320533
- SinclairAMHughesADSeverPS1993Effect of nifedipine and glyceryl trinitrate on retinal blood flow in normal subjectsJ Hum Hypertens73994018410935
- StromanGAStewartWCGolnikKC1995Magnetic resonance imaging in patients with low-tension glaucomaSurv Ophthalmol11316872
- SugiyamaTMoriyaSOkuH1995Association of endothelin-1 with normal tension glaucoma: clinical and fundamental studiesSurv Ophthalmol39suppl 1S49567660312
- TakaseHMoreauPLuscherTF1995Endothelin receptor subtypes in small arteries. Studies with FR139317 and bosentanHypertension25739437721425
- TezelGKassMAKolkerAE1997Plasma and aqueous humor endothelin levels in primary open-angle glaucomaJ Glaucoma68399098815
- TielschJMKatzJSommerA1995Hypertension, perfusion pressure, and primary open-angle glaucoma. A population-based assessmentArch Ophthalmol113216217864755
- TodaNNakanishi-TodaM2007Nitric oxide: Ocular blood flow, glaucoma, and diabetic retinopathyProg Retin Eye Res262053817337232
- TopouzisFColemanALHarrisA2006Association of blood pressure status with the optic disk structure in non-glaucoma subjects: the Thessaloniki eye studyAm J Ophthalmol14260716815251
- TrimbleJLKocklerDR2007Statin treatment of cerebral vasospasm after aneurismal subarachnoid hemorrhageAnn Pharmacother4120192317986515
- TsengMYCzosnykaMRichardsH2005Effects of acute treatment with pravastatin on cerebral vasospasm, autoregulation, and delayed ischemic deficits after aneurismal subarachnoid hemorrhageStroke3616273216049199
- TunnyTJRichardsonKAClarkCV1998Association study of the 5′ flanking regions of endothelial-nitric oxide synthase and endothelin-1 genes in familial primary open-angle glaucomaClin Exp Pharmacol Physiol252699493554
- TutajMBrownCMBrysM2004Dynamic cerebral autoregulation is impaired in glaucomaJ Neurol Sci220495415140605
- Von GraefeA1857Uber die iridectomie bei glaukom und uber den glaucomatosen prozessVon Graefe’s Arch Ophthalmol3456555
- WaldmannEGasserPDublerB1996Silent myocardial ischemic in glaucoma and cataract patientsGraefes Arch Clin Exp Ophthalmology103101424
- WerneAHarrisAMooreD2008The circadian variations in systemic blood pressure, ocular perfusion pressure and ocular blood flow: risk factors for glaucoma?Surv Ophthalmol
- WilsonRPChangWJSergottRC1997A color Doppler analysis of nifedipine-induced posterior ocular blood flow changes in open-angle glaucomaJ Glaucoma623169264302
- WinterkornJMJupersmithMJWirtschafterJD1993Brief report: treatment of vasospastic amaurosis fugax with calcium-channel blockersN Engl J Med32939688326973
- WinterkornJMBechmanRL1995Recovery from ocular ischemic syndrome after treatment with verapamilJ Neuroophthalmol15209118748556
- WizemannAWizemannV1980The use of organic nitrates to lower intraocular pressure in outpatient and surgical treatmentKlin Monatsbl Augenheilkd17729256779050
- WongTYKleinRKleinBEK2003Retinal vessel diameters and their associations with age and blood pressureInvest Ophthalmol Vis Sci4446445014578380
- YaoKTschudiMFlammerJ1991Endothelium-dependent regulation of vascular tone of the porcine ophthalmic arteryInvest Ophthalmol Vis Sci32179182032802
- YuDYAlderVACringleSJ1994Vasoactivity of intraluminal and extraluminal agonists in perfused retinal arteriesInvest Ophthalmol Vis Sci354087997960591
- ZurakowskiDVorwerkCKGorlaM1998Nitrate therapy may retard glaucomatous optic neuropathy, perhaps through modulation of glutamate receptorsVision Res381489949667013