Abstract
Hyponatremia is one of the most common electrolyte abnormalities linked to adverse outcomes and increased mortality in hospitalized patients. While the differential diagnosis for hyponatremia is diverse, most cases stem from arginine vasopressin (AVP) dysregulation, where hypoosmolality fails to suppress AVP synthesis and release. The physiological effects of AVP are currently known to depend on its interaction with any of 3 receptor subtypes V1A, V2, and V1B. Activation of V2 by AVP is the key in renal water regulation and maintenance of total body volume and plasma tonicity. Despite the long-recognized problem with excess AVP in euvolemic and hypervolemic hyponatremia, traditional therapeutic options have relied on nonspecific and potentially problematic strategies. More recently, a new class of drugs, introduced as “aquaretics,” has gained great attention among clinicians because of its ability to correct hyponatremia via direct competitive inhibition of AVP at V2 receptors to induce renal electrolyte-free water excretion. In this paper, we aim to review available clinical data on the only FDA-approved aquaretic, dual V1A/V2 receptor antagonist conivaptan, discuss its clinical indications, efficacy, safety profile, and comment on its clinical limitations.
Hyponatremia is the most frequent fluid and electrolyte abnormality in hospitalized patients, particularly in the intensive care unit setting (CitationDeVita et al 1990; CitationSedlacek et al 2006). In addition to being a potentially life-threatening condition, hyponatremia is an independent predictor of death among intensive care unit and geriatric patients and those with heart failure, acute ST elevation myocardial infarction, and cirrhosis (CitationArieff et al 1976; CitationTerzian et al 1994; CitationBorroni et al 2000; CitationLee et al 2000; CitationBennani et al 2003; CitationGoldberg et al 2004; CitationRuf et al 2005).
The syndrome of inappropriate antidiuretic hormone secretion (SIADH), congestive heart failure (CHF), and cirrhosis are common conditions associated with abnormal water retention mediated by excess antidiuretic hormone (ADH), also known as arginine vasopressin (AVP) irrespective of hypoosmolality. Over the years, conventional therapies for these conditions including water restriction, hypertonic saline, demeclocycline, and lithium have all proved to have significant limitations. The advent of AVP receptor antagonists has therefore sparked great enthusiasm among clinicians as a promising direct therapeutic option for hyponatremia associated with dysregulated excess AVP.
While several AVP receptor antagonists are being investigated in clinical trials, conivaptan is the first, and currently, only vasopressin receptor antagonist approved by United States Federal Drug Administrations (FDA) for the treatment of hyponatremia. In the current manuscript, we aim to review the literature on the clinical efficacy, safety, and therapeutic options for the use of conivaptan.
AVP in water and electrolyte balance
Overview of AVP synthesis and release
AVP is produced in the hypothalamus, predominantly in the paraventricular and supraoptic nuclei by magnocellular neurons. Subsequently, it is transported down axon terminals through the supraoptic hypophyseal tract, and eventually stored in the posterior pituitary as inactive complexes with neurophysins (CitationDavison et al 1981; CitationIshikawa and Schrier 2003). AVP release occurs quantally by exocytosis of AVP-containing secretory granules in response to both osmotic and nonosmotic stimuli (CitationDunn et al 1973).
In the event of an increase in extracellular osmolality, water from osmoreceptor cells within the hypothalamus is shifted extracellularly to achieve osmotic equilibrium. The resultant cell-volume shrinkage or increased intracellular osmolality, or both, in turn initiate a cascade of events that lead to the augmentation of both AVP synthesis and release (CitationRobinson et al 1990). In humans, the osmotic threshold for AVP release is approximately 280–290 mOsm/kg (280–290 mmol/kg) (CitationRobertson 1987). In addition, for every 1% increase in serum osmolality, there may be a 2- to 4-fold increase in AVP level (CitationDunn et al 1973).
The synthesis and release of AVP may also be induced by nonosmotic stimuli including hypovolemia, a fall in arterial blood pressure, neural regulation by various hypothalamic neurotransmitters and neuropeptides, and many pharmacological agents. Endogenous agents including acetylcholine, angiotensin II, histamine, bradykinin, and neuropeptide Y have all been implicated in the stimulation of AVP release (CitationSladek 1983; CitationBaylis 1989). Pharmacological agents such as morphine, nicotine, chemotherapeutic agents, tricyclic antidepressants, anticonvulsants, clofibrate, and chlorpropamide and clinical conditions including nausea, vomiting, pain, and hypoglycemia also may play a role (CitationSladek 1983; CitationBaylis 1989).
AVP receptor subtypes
AVP exerts its actions through three subtypes of G-protein – coupled membrane receptors including V1A (vascular), V2 (renal), and V1B (pituitary), also denoted as V3 in the literature.
V1A receptors are found on vascular smooth muscle, liver, kidneys, reproductive organs, spleen, adrenal cortex, and platelets. The vasoconstrictive, mitogenic, and possibly platelet aggregative and hypercoagulable actions of AVP through V1A have been suggested to potentially contribute to the pathogenesis and progression of arterial hypertension, heart failure, and atherosclerosis (CitationThibonnier et al 2001).
V2 receptors are expressed primarily in basolateral membranes of renal cortical and medullary collecting ducts and, to a lesser extent, distal tubules. Binding of circulating AVP to the G-protein-coupled V2 receptors on the basolateral membranes of principal cells in the collecting ducts activates adenylyl cyclase, thereby enhances the production of cyclic adenosine monophosphate (cAMP) and the subsequent activation of protein kinase A. The down-stream effect is enhanced production and trafficking of the water channels aquaporin-2 to the luminal membranes to facilitate water reabsorption into the hypertonic medullary interstitium. In effect, the physiological roles of AVP via V2 receptors include maintenance of plasma tonicity and blood pressure and volume regulation (CitationThibonnier et al 2001; CitationLee et al 2003).
V1B (or V3) receptors are found predominantly in the anterior pituitary corticotroph cells and, to a lesser extent, the brain, kidney, pancreas, and adrenal medulla. Physiologically, activation of V3 receptors is associated with adrenocorticotropic hormone and β-endorphin release (CitationThibonnier et al 2001).
AVP: V2 interaction and water homeostasis
Regulated state
While water homeostasis is dependent on osmotically active solutes at the cellular level, total body water balance is regulated by the thirst center and renal water excretion (CitationVerbalis 2003).
Renal free water excretion is dependent on the integrity of nephron-diluting segments, a hypertonic medullary interstitium, and intact AVP activity at the collecting ducts (). For renal water excretion to occur, free water is first produced in the nephron-diluting segment, the thick ascending loop of Henle, by salt extractions from the lumen through the sodium potassium-2 chloride (NKCC) cotransporter. Intraluminal free water is produced with this process because water cannot diffuse across the luminal surface in parallel with salt uptake due to the unique water-impermeable characteristic of this diluting segment. Reabsorption of salt without water continues to occur in the distal convoluted tubule, where dilution of urine is maximized. While free water is produced within the lumen, salt uptake by previous nephron segments concomitantly creates and maintains the high tonicity within the medullary interstitium. Subsequent delivery of the intraluminal free water to the collecting ducts may be either reabsorbed or excreted into urine. In the presence of AVP and an intact hypertonic medullary interstitium, AVP, mediated by V2 receptors, upregulates the expression and trafficking of aquaporin-2 to the luminal side of the collecting tubules and allows water to be reabsorbed efficiently into a more tonic medullary milieu. AVP-facilitated free-water reabsorption at the collecting tubules reduces and concentrates the final urine volume (CitationVerbalis 2003). In the absence of AVP, the free water produced from the diluting segment is excreted and is reflected clinically as a high urine output with low osmolality.
Figure 1 Renal-free water metabolism. The presence of AVP favors renal free water reabsorption whereas its absence favors water excretion.
Abbreviations: PT, proximal tubule; TAH, thick ascending limb loop of Henle; DCT, distal convoluted tubule; CT, collecting tubule; NCCK, sodium potassium-2 chloride cotransporter; AVP, arginine vasopressin.
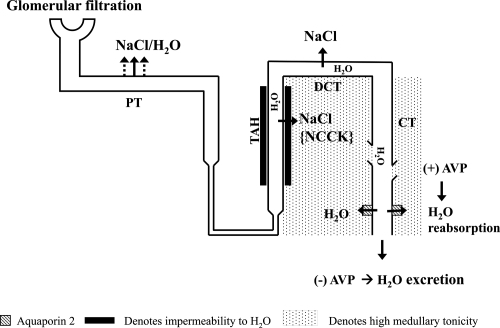
Dysregulated state: AVP excess and hyponatremia
In the dysregulated state where AVP is excessively produced and released irrespective of hypoosmolality, profound water retention and hyponatremia may occur (CitationBaylis 2003).
In the setting of hypovolemia where there is great volume loss or fall in arterial blood pressure, AVP is appropriately upregulated to enhance both thirst and renal water retention in an effort to restore volume and arterial blood pressure. Excessive free water uptake in this case, however, can easily lead to hyponatremia (CitationHew-Butler et al 2005).
In cases of CHF, where the reduced cardiac function fails to effectively drive the blood volume from the venous to arterial circulation to maintain the effective arterial volume, AVP is upregulated to induce cardiomyocyte hypertrophy and vasoconstriction via V1A and enhance renal water retention via V2, as compensatory mechanisms to increase the effective arterial volume. With further deterioration in heart failure, however, the successive and cumulative compensatory upregulation of AVP paired with unrestricted free water intake can lead to the development of hypervolemia and hyponatremia (CitationBartter and Schwartz 1967). Similarly, patients with cirrhosis or nephrosis who have poor arterial pressure because of peripheral vasodilation, relatively low intravascular oncotic pressure, or both, may also progressively develop an upregulated AVP state irrespective of osmolality. In the setting of unrestricted free water intake, hypervolemia and hyponatremia develop as a result of the excessive AVP-driven renal free-water reabsorption (CitationAndreoli 2000; CitationCardenas and Arroyo 2003).
Euvolemic hyponatremia accounts for 60% of all forms of chronic hyponatremia, of which SIADH, a disorder in which AVP release is independent of volume, blood pressure, and osmotic control, predominates (CitationZerbe et al 1980; CitationBaylis 2003). The major categories of illnesses associated with SIADH include neoplasm, neurological disease or trauma, pulmonary disorders, and various physiologically stressful states such as nausea, vomiting, pain, and anxiety. In addition, SIADH may be induced by various pharmacological agents including tricyclic antidepressants and anticonvulsants (CitationZerbe et al 1980; CitationFraser and Arieff 1997; CitationAdrogué and Madias 2000; CitationBaylis 2003).
Traditional therapeutic options for hyponatremia
Ethanol was the first agent recognized to inhibit ADH secretion. The therapeutic potential of ethanol in the management of hyponatremia, however, is unacceptable because of the multiple medical complications associated with its use (CitationBerl 2007).
Prior to 2005, common treatment options for patients with hyponatremia, included sodium supplementation, water restriction, and in some cases, pharmacological agents such as demeclocycline, lithium, and loop diuretic therapy. Although these therapeutic options may be generally effective, they are not without limitations ().
Table 1 Traditional therapeutic options for hyponatremia
For hypovolemic hyponatremia, normal saline infusion is most effective and is undoubtedly the therapy of choice. Normal saline infusion, however, may not be effective or even detrimental in severe cases of SIADH (ie, urine osmolality much greater than 300 mOsm/kg), where avid renal free water reabsorption can, in essence, extract free water from the normal saline infused for reabsorption at the collecting tubules, a phenomenon known as “desalination.” The excess free water reabsorbed can exacerbate the existing hyponatremia and induce serious neurological sequelae.
In patients with hypervolemic hyponatremia due to CHF, cirrhosis, or nephrosis, saline infusion may worsen the existing hypervolemia. Water restriction with or without concomitant diuretic administration in patients with euvolemic and hypervolemic hyponatremia, on the other hand, may lead to a slow response, less predictable outcomes, and occasionally, serious hypovolemic complications.
In chronic hyponatremia where fluid restriction is not tolerated, demeclocycline, may be used to induce free water excretion via the reduction of renal medullary adenylate cyclase activity, the mediator for a full vasopressin effect on the collecting system (CitationSinger and Rotenberg 1973). The use of demeclocycline, however, is limited by inconsistent results and adverse effects including azotemia, photosensitivity, and nausea. In addition, its use is contraindicated in patients with cirrhosis and renal failure (CitationMiller et al 1980; CitationFried and Palevsky 1997).
Lithium, an agent commonly prescribed for patients with manic depressive patients, has also been previously suggested to be a possible therapeutic agent in the management of chronic hyponatremia. The mechanism of action is thought to be via the downregulation aquaporin 2 in collecting tubules (CitationRobbenet al 2006). The chronic use of lithium, however, is limited by nephrotoxicity (CitationMarkowitz et al 2000).
Loop diuretics are effective in inhibiting renal free water production and reabsorption and thus potentially beneficial in the management of euvolemic and hypervolemic hyponatremia. Unfortunately, excessive volume depletion and poor response predictability may be problematic.
Given the potential adverse effects with existing therapeutic options for hyponatremia, the introduction of vasopressin receptor antagonists as a class of drug that can directly induce electrolyte-free water excretion to correct hyponatremia has gained great interests among clinicians. Indeed, the advent of vasopressin receptor antagonists has given rise to the term, “aquaretics,” as opposed to “diuretics,” to denote agents that can induce “electrolyte-free” water excretion.
AVP receptor antagonists
The safety and efficacy of AVP receptor antagonists in the treatment of both euvolemic and hypervolemic hyponatremia have been evaluated in multiple clinical trials. AVP receptor antagonists directed at V2 receptors (lixivaptan, tolvaptan and satavaptan) are currently being investigated for clinical use in patients with euvolemic and hypervolemic hyponatremia. Lixivaptan, an oral V2 receptor antagonist, has been shown to be effective in raising serum sodium concentrations in patients with CHF, SIADH, and cirrhosis with ascites. Tolvaptan, another oral V2 receptor antagonist, has also been studied extensively and shown to be effective in inducing aquaresis and improving serum sodium concentrations in patients with CHF (reviewed in CitationPham et al 2006). Satavaptan, is the latest oral V2 receptor antagonist introduced into clinical trials for the treatment of hyponatremia in SIADH patients (CitationSoupart et al 2006) (). To date, all of these agents have been shown to effectively promote “aquaresis,” the electrolyte-sparing excretion of free water and thereby improve hyponatremia (CitationSerradeil-Le Gal et al 2002).
Table 2 Overview of AVP receptor antagonists
Similar to all V2 receptor antagonists, conivaptan, a dual V1A/V2 receptor antagonist, has also been studied extensively in both euvolemic and hypervolemic patients. Currently, however, conivaptan is the only FDA approved aquaretic in the United States for use in hospitalized patients with euvolemic and hypervolemic hyponatremia.
Conivaptan
Chemical structure and molecular mechanism of action
Conivaptan hydrochloride (Vaprisol®, Astellas Pharma US, Inc.), is chemically [1,1•biphenyl]-2-carboxamide, N-[4-[(4,5-dihydro-2-methylimidazo[4,5-d][1]benzazepin-6(1H)-yl)carbonyl]phenyl]-,monohydrochloride (). Conivaptan inhibits AVP by competitively and reversibly binding to selected AVP receptors without interacting with the receptors’s active sites. Although conivaptan has high affinities for both V1A and V2 receptors, radioligand competition binding assays revealed a tenfold higher affinity for V2 than that for V1A receptors (CitationBurnier et al 1999). Because of the higher affinity for V2, conivaptan is predominantly used for its V2-associated aquaretic effect. Nonetheless, conivaptan inhibition of V1A receptors can theoretically result in clinically significant effects that must be considered among a subset of hyponatremic patients. As V1A stimulation is associated with vasoconstrictive, mitogenic, and possibly platelet aggregative effects, its inhibition can be potentially beneficial for patients with CHF with concomitant hypertension and atherosclerotic disease. In contrast, the very same effects derived from V1A inhibition can be harmful for hypotensive patients or cirrhotic patients with dilated splanchnic beds and variceal bleeds. Further dilatation of the splanchnic bed and interference with platelet aggregation by V1A inhibition could exacerbate variceal bleeding complications. Hypothermia due to skin vasodilatation is another theoretical concern among those at risk.
Pharmacokinetics and metabolism
The pharmacokinetics of conivaptan have been evaluated in both oral and intravenous forms in healthy individuals and patients with various euvolemic and hypervolemic conditions including CHF. In healthy individuals, oral conivaptan at 60 mg has been demonstrated to have an absolute bioavailability of 44%, Cmax of 664 ng/dL, and t ½ of 5.6 hours (h) (CitationBurnier et al 1999). In healthy males, intravenous conivaptan administered at a 20 mg loading dose over 30 minutes resulted in a mean Cmax of 619 ng/mL at the end of the loading dose and Cmin at 12 hours. With a continuous infusion of 40 mg/d over 3 days following the loading dose, a mean concentration of 188 ng/mL over the infusion duration was achieved. The mean terminal elimination half-life following the infusion was 5.0 hours with a mean clearance of 15.2 L/h (46). High intersubject variability was noted. Patients with hypervolemia may achieve a lower Cmax compared to euvolemic patients when given the same loading dose. Of note, the pharmacokinetics of conivaptan following either route of administration were non-linear, a phenomenon suggested to reflect conivaptan’s inhibition of its own metabolism (CitationAstellas Pharma US, Inc. 2007).
At therapeutic range, conivaptan is highly protein-bound (99%). It is both a substrate and potent inhibitor of the liver cytochrome P450 isozyme CYP3A4. The metabolites of conivaptan have been shown to retain some pharmacological effect (3%–50% V1A and 50%–100% V2 inhibition). With rapid drug elimination in most cases, however, the clinical contributions from the metabolites have been shown to be small (CitationBurnier et al 1999). Conivaptan elimination has been shown to occur predominantly via the gastrointestinal tract, and to a lesser extent, urinary route. Special caution must be exercised in treating geriatric patients and individuals with renal and hepatic impairment because of decreased drug clearance.
Because conivaptan significantly interacts with the cytochrome P450 isozyme CYP3A4, great caution must be given when conivaptan is co-administered with medications that can affect or are affected by this system. Co-administration of conivaptan can increase plasma concentrations of midazolam, simvastatin, digoxin and amlodipine. Co-administration of conivaptan with potent inhibitors of CYP3A4, such as ketoconazole, itraconazole, clarithromycin, ritanovir, and indinavir is contraindicated. Due to concerns for potentially serious drug-drug interactions with chronic use, conivaptan is only available clinically in the intravenous form for short-term in-hospital use.
Clinical efficacy ()
Conivaptan in heart failure
As AVP has been shown to be elevated in most patients with heart failure and suggested to adversely affect cardiovascular outcomes (CitationRouleau et al 1994), the potential benefits and hemodynamic effects of conivaptan in patients with advanced heart failure have been examined in several trials (CitationUdelson et al 2001; CitationGross et al 2004; CitationVerbalis et al 2004).
Table 3 Clinical use of conivaptan
In a large multicenter, randomized, double-blind, placebo-controlled trial, CitationUdelson et al (2001) examined the acute effects of conivaptan as a dual V1A/V2 inhibitor on commonly measured hemodynamic parameters among patients with advanced heart failure. The study involved patients with symptomatic heart failure (New York Heart Association Class III and IV) of at least 3 months duration due to left ventricular systolic dysfunction. Eligible patients had to be on a standard background therapy for heart failure, including at least 1 month of therapy with a loop diuretic and an angiotensin converting enzyme inhibitor (unless intolerance was previously demonstrated). At study entry, patients were randomized to receive a single dose of placebo, 10, 20, or 40 mg of conivaptan intravenously. Randomization was stratified as to whether patients were receiving background therapy with β-adrenergic blockers. Hemodynamic and renal parameters and vital signs were measured at multiple time points (1- to 2-hour intervals) over a 12-hour study period. Background diuretics and other medications were held during the study period, and fluid intake was “restricted” to 250 mL every 2 hours.
A total of 142 patients were included in the study. The patient sample had an average age of 58.3 ± 2 and consisted of 76.1% males, 59.2% Caucasians, 35.9% African Americans, and 4.9% Hispanics. Eighty-nine percent of the patients were classified as having CHF class III and 11% class IV. Significant beneficial alterations in hemodynamics were observed to peak during 3–6 hours and maintained, although at a decreasing level, over the 12-hour study period. Compared to placebo, conivaptan at 20 and 40 mg significantly reduced pulmonary capillary wedge pressure (peak effect: −2.6 ± 0.7, −5.4 ± 0.7, and −4.6 ± 0.7 mmHg for the placebo, 20, and 40 mg groups, respectively, p < 0.05) and right atrial pressure (peak effect: −2.0 ± 0.4, −3.7 ± 0.4, and −3.5 ± 0.4 mmHg for the placebo, 20, and 40 mg groups, respectively, p < 0.05). The fall in pulmonary capillary wedge pressure and right atrial pressure was associated with a significant increase in urine output which peaked during the first four hours following the administration of conivaptan (−11 ± 17, 152 ± 19, and 176 ± 18 mL/h for the placebo, 20, and 40 mg groups, respectively, p < 0.001). The higher urine output in both conivaptan groups was associated with markedly lower osmolality compared to that of placebo. With the exception of a slight increase in vasopressin level in the 40 mg/d treatment group, vasopressin levels in general, did not change significantly over the 12 hour study period. Other hemodynamic parameters including cardiac index, systemic and pulmonary vascular resistance, blood pressure, and heart rate did not differ significantly from placebo. The investigators, thus concluded that the use of conivaptan in advanced heart failure resulted in favorable hemodynamic changes, presumably via enhancing aquaresis without affecting blood pressure and heart rate. It must be noted, however, that the study only examined the acute alterations in hemodynamics following a single intravenous dose of conivaptan.
Despite Udelson et al’s encouraging results, 10 Phase 2/pilot heart failure studies failed to show that the use of conivaptan significantly improved heart failure outcomes including length of hospital stay, categorized physical findings of heart failure, ejection fraction, exercise tolerance, functional status, or heart failure symptoms compared to placebo (CitationAstellas Pharma US, Inc. 2007). The inconsistencies between Udelson’s study and all other subsequent studies may have reflected the very loose fluid “restriction” of 250 mL every 2 hours used in Udelson’s study. In the routine management of CHF, fluid restriction is typically 50–75 mL/h. The high fluid intake allowed may have exaggerated the short-term beneficial aquaretic effects of conivaptan. That is, conivaptan may have predominantly helped remove the excess fluid intake during the study, and not the volume overload from the underlying CHF per se. Moreover, the higher fluid intake allowed may have masked conivaptan-induced adverse effects on the various hemodynamic parameters. Net fluid balance was not reported in the study.
Due to the lack of data documenting positive outcomes with the use of conivaptan in CHF patients and, in part, concerns for unknown effects of conivaptan on endothelin production and neurohormonal systems including the renin angiotensin aldosterone system and sympathetic nervous system, conivaptan is currently not recommended for CHF patients, unless the benefit of correcting the associated hyponatremia outweighs the potential risk of adverse events.
Conivaptan in euvolemic and hypervolemic hyponatremia
The efficacy of conivaptan as an aquaretic agent to correct hyponatremia has been evaluated in several multicenter-randomized, double-blind, placebo-controlled trials. In 2004, several such studies were presented at the American Heart Association Scientific Sessions (CitationGhali et al 2004; CitationGross et al 2004; CitationVerbalis et al 2004).
In the study led by Verbalis in North America, 84 patients involving men and women of 18 years of age or greater with “euvolemic and hypervolemic hyponatremia” including patients with CHF with serum sodium ([Na+]) 115 to less than 130 mEq/L were recruited. Subjects were randomized to receive placebo or 20 mg of conivaptan as a bolus intravenous dose, followed by a continuous infusion of 40 or 80 mg per day for 4 days (CitationVerbalis et al 2004). At baseline, serum [Na+] were 124.3 ± 4.9, 123.3 ± 4.7, and 124.8 ± 3.4 mEq/L for the placebo, 40 mg/day, and 80 mg/day groups respectively, p = NS. The least square mean changes in serum [Na+] at day 4 for the 3 respective groups were 2.0 ± 0.8, 6.8 ± 0.8, and 9.0 ± 0.8 mEq/L. Accordingly, the number (%) of patients achieving 6 mEq/L or more increase in serum [Na+] from baseline or normalization of [Na+] (defined as ≥ 135 mEq/L) were 6 (20.7%), 20 (69%); p < 0.01, and 23 (88.5%); p < 0.001 for the 3 respective groups. The median time from the first dose to 4 mEq/L increase in serum [Na+] was 23.7 hours and 23.4 hours for the conivaptan 40 mg/day and 80 mg/d groups, respectively. In addition, the mean changes in effective water clearance from baseline on day 1 were −332.3 ± 434.1, 1984 ± 1559.4; p < 0.05, and 1759.4 ± 1748.3 ml; p < 0.05 for the 3 respective groups. Based on the data, CitationVerbalis et al (2004) concluded that during the short-term study, conivaptan significantly improved serum [Na+] and water clearance within 24 hours in their patients with euvolemic and hypervolemic hyponatremia including those with CHF.
In a different study conducted across 17 centers in Europe, CitationGross et al (2004) evaluated the efficacy of oral conivaptan in the treatment of euvolemic and hypervolemic hyponatremia including patients with CHF. Recruited patients were 18 years of age or greater with serum [Na+] of 115 to <130 mEq/L and plasma osmolality <290 mOsm/kg H2O. At study entry, patients were randomized to receive placebo, 40, or 80 mg/day orally in 2 divided doses for 5 days. The study sample involved 55 men and 28 women, 52 of whom had euvolemic and 31 hypervolemic hyponatremia. At baseline, serum [Na+] were 125.6 ± 3.9, 125.1 ± 5.1, and 125.6 ± 3.6 mEq/L for the placebo, conivaptan 40 mg/d, and 80 mg/d groups, respectively, p = NS. The least squares mean change in serum [Na+] on day 5 for the 3 respective groups were 1.0 ± 0.9, 6.8 ± 0.9, and 8.8 ± 0.9 mEq/L. The number (%) of patients achieving 6 mEq/L or greater increase in serum [Na+] or normalization of [Na+] (defined as [Na+] ≥ 135 mEq/L) during the treatment period was 6 (20%), 18 (67%); p < 0.001, and 23 (89%); p =0.0001, for the 3 respective groups. Most importantly, the increase in serum [Na+] among the conivaptan treated groups appeared to be dose-dependent. The median time from the first dose to 4 mEq/L increase in serum [Na+] was 23.5 hours (95% CI 12–24 hours) for the 40 mg/d group and 8.7 hours (95% CI 4–24 hours) for the 80 mg/d group. The improvement in serum [Na+] was reflected in significantly better effective water clearance (−12 ± 464, 974 ± 1233, and 1342 ± 1143 mL for the placebo, conivaptan 40 mg/d, and 80 mg/d groups, respectively). The investigators thus concluded that during the 5-day study period, oral conivaptan resulted in significant increases in serum [Na+] and effective water clearance within the first day of treatment.
Most recently, CitationGhali et al (2006) published the results from their randomized, double-blind, placebo-controlled trial conducted across 21 sites in the United States, Canada, and Israel, involving the efficacy of oral conivaptan in the treatment of patients with euvolemic and hypervolemic hyponatremia. The study involved hospitalized patients of at least 18 years of age who had a serum [Na+] of 115 to less than 130 mEq/L, plasma osmolality less than 290 mosmol/kg H2O, and no evidence of extracellular volume depletion. Recruited patients were randomized to receive placebo, 40, or 80 mg/d of conivaptan orally in 2 divided doses for 5 days. Close monitoring of clinical laboratory and safety profile parameters occurred throughout the study period and at 6–9 days following the conclusion of the treatment period. Fluid intake was limited to 2.0 L/d. The main outcome measure was the change in serum [Na+] area under the curve from baseline.
The study involved a sample size of 74 patients who had an average age of 69.3 years (range 35–94). The underlying conditions thought to be associated with hyponatremia included chronic obstructive pulmonary disease (4.2%), malignancy (11.3%), idiopathic (21.0%), CHF III and IV (45.0%), and other (22.5%). The randomization process resulted in 23, 24, and 27 patients in the placebo, 40, and 80 mg/d groups, respectively. The baseline serum [Na+] were 123.4 ± f4.1, 125.3 ± 3.5, and 125.4 ± 4.0 mEq/L in the 3 respective groups, p = NS.
The changes from baseline in serum [Na+] AUC [least-squares mean (SE) mEq*h/L] were 309.2 (94.8), 621.3 (89.0), and 836.2 (87.8) in the 3 respective groups, p-values for the treated groups compared to placebo were 0.03 and <0.001 for the 40 and 80 mg/d groups, respectively. The least-squares mean total time for serum [Na+] to increase ≥ 4 mEq/L above baseline were 46.5 (9.0), 69.8 (8.5), and 88.8 (8.4) for the 3 respective groups, with p-values of 0.103 and 0.001 for the 40 and 80 mg/d groups compared to placebo, respectively. The percentage of patients with confirmed normalization of serum [Na+] (defined as ≥ 135 mEq/L) or increase of ≥ 6 mEq/L from baseline was 48, 71, and 82% in the 3 respective groups, with p = 0.014 for the 80 mg/d group compared to placebo. In addition, the effective water clearance on day 1 of the study as well as its cumulative change over the 5-day treatment period were significantly higher in both conivaptan groups compared to placebo. The increase in aquaresis by both conivaptan treatment groups was in accordance with the significant fall in urine osmolality in both groups compared to that of placebo.
Based on currently available studies, conivaptan appears to be effective in inducing aquaresis to correct hyponatremia with short-term use in both hypovolemic and hypervolemic hyponatremic patients including those with CHF. Nevertheless, as previously discussed, the use of conivaptan in CHF is not routinely recommended due to inadequate data and unclear safety issues.
Conivaptan in cirrhosis
As previously discussed, it is not advisable to use conivaptan in patients with cirrhosis because V1A inhibition could exacerbate splanchnic vasodilatation and interfere with platelet aggregation, thus promote variceal bleeds. Whether the use of pure or relatively pure V2 receptor antagonists is safe in these conditions is not known.
Other potential uses of conivaptan: PCKD, nephrogenic DI
Polycystic kidney disease (PCKD) is one of the most recognized inherited kidney diseases characterized by the formation of renal cysts from tubular epithelial cells of predominantly collecting duct origin. The underlying genetic defects have been identified to involve the polycystin-encoding PKD1 and PKD2 for autosomal dominant and the fibrocystin-encoding PKHD1 for autosomal recessive polycystic kidney disease. Defects of the involved proteins in tubular epithelia are thought to result in cellular de-differentiation, excessive fluid secretion, and proliferation, hence, cyst formation. While alterations in many cellular components and processes associated with the primary protein defects have been implicated in the pathogenesis of PCKD, renal accumulation of cAMP has been observed as a common factor in most models of PCKD (reviewed in CitationTorres and Harris 2006). In the principal cells of collecting ducts, it is known that vasopressin stimulates adenylyl cyclase via V2 receptors to form cAMP. The introduction of V2 receptor antagonists has therefore led to ongoing investigations into the role of these agents in slowing the progression of human PCKD. Preliminary data in animal models of PKD have revealed reduction in kidney weight, cyst volume, and renal fibrosis in association of reductions in renal cAMP levels with the use of V2 receptor antagonists (CitationGattone et al 2003; CitationTorres et al 2004; CitationTorres and Harris 2006).
Congenital nephrogenic diabetes insipidus (NDI) is a condition characterized by the loss of AVP-mediated renal free water reabsorption. The pathogenic mechanisms may involve mutations leading to reduced AVP binding to V2 receptors (type 1), misfolding hence reduced trafficking of V2 receptors from the endoplasmic reticulum to cell surface (type 2), or direct defect in the transcription of V2 receptors (type 3) (CitationFujiwara and Bichet 2005). In a recent study, Bernier et al provided data to suggest that the use of conivaptan may be beneficial in the treatment of NDI in patients with type 2 mutations. The investigators demonstrated that conivaptan can improve cell surface expression of the mutated misfolded V2 receptors in cultured cell systems. It was suggested that the agent acts as a pharmacologic chaperone to improve trafficking of the mutated misfolded V2 receptors to the cell surface where they can interact with AVP to enhance free water retention (CitationBernier et al 2006). Further confirmatory studies are warranted.
Clinical safety ()
Minor adverse effects
The most common adverse effects reported for the short-term use of conivaptan include headaches, increased thirst, pyrexia, constipation, nausea, and phlebitis at the site of drug infusion (CitationAstellas Pharma US, Inc. 2007).
Table 4 Limitations to the use of conivaptan
Serious adverse effects
Minimal to clinically significant fall in blood pressure to the extent of postural hypotension have been reported (CitationUdelson et al 2001; CitationGhali et al 2006). In a study involving euvolemic and hypervolemic hyponatremic patients, CitationGhali et al (2006) reported significant hypotension in two patients with severe cardiomyopathy, one of whom had concomitant conventional diuretic therapy, and another patient with presumed polydipsia-induced hyponatremia. In our opinion, the use of combined conventional diuretics and V2 receptor antagonists must be carefully evaluated, especially in the setting involving patients with tenuous cardiac function and blood pressure. In addition, the use of V2 receptor antagonists should be contraindicated in fluid-restricted patients with polydipsia-induced hyponatremia.
One of the most concerning issues with the use of any V2 receptor antagonist in the treatment of hyponatremia is the rate of correction of serum [Na+]. While most patients were reported to do well with conivaptan, it is concerning to note that 5 out of 51 patients who received conivaptan 40–80 mg/d from CitationGhali et al’s (2006) study had a rapid serum [Na+] correction, apparently as fast as 14 mEq/L within 4 hours of treatment. While no serious neurological sequelae has been reported from the rapid rate of correction to date, the development of osmotic demyelination syndrome remains a possibility with post-marketing use.
Hypokalemia, reported to occur in up to 22% of patients receiving conivaptan, is another concerning side effect that must be recognized (CitationVHA 2006; CitationLandwehr and Huling 2007). Particular caution must be given to patients with CHF and those with underlying total body potassium depletion to avoid potentially life-threatening arrhythmias, rhabdomyolysis, or other hypokalemic-related metabolic complications. We speculate that conivaptan induces renal potassium loss via enhanced urinary flow and facilitated potassium secretion at the collecting tubules.
Unknown potential adverse effects
Rebound hyponatremia may occur following withdrawal of conivaptan therapy because of a compensatory rise in plasma AVP levels during the treatment period. With the upregulated AVP, both renal water retention and thirst may, once again, be excessively stimulated to result in hyponatremia. Indeed, CitationBurnier et al (1999) previously reported that the aquaretic effect of conivaptan led to a transient negative fluid balance when fluid restriction was enforced, but rapidly became positive when the restriction was lifted. The requirement for water restriction during and following conivaptan treatment must be further examined.
In addition to rebound hyponatremia, the long-term effects of the compensatory rise in plasma AVP levels on V1B, and to a lesser extent, V1A receptors are unknown.
Although dual V1A/V2 receptor antagonism by conivaptan is potentially valuable in the management of congestive heart failure, data on its long-term effects on cardiovascular morbidity and mortality and overall improvement in quality of life and survival are lacking. Of interest, there is evidence to suggest that compared to placebo, intravenous administration of conivaptan to hypervolemic hyponatremic patients with underlying heart failure may be associated with a higher frequency of adverse cardiac events, atrial dysrhythmias, and sepsis (CitationAstellas Pharma US, Inc. 2007). The use of conivaptan in CHF patients must therefore be carefully weighed and should be limited to those with the greatest benefits to risks ratios.
Due to relatively limited data, another concern that remains unanswered, but must be examined, is the long-term effect of conivaptan on renal function. It is conceivable that excessive aquaresis with the use of conivaptan in some patients may lead to clinically significant hypovolemia, hypotension, and subsequent acute tubular necrosis and kidney injury.
Limitations
It must be noted that current data have only included patients with chronic conditions associated with euvolemic and hypervolemic hyponatremia including patients with congestive heart failure. No data on the use of conivaptan in acute or severe hyponatremia (ie, [Na+] <115 mEq/L or hyponatremia with associated serious neurological sequelae) are available. A major concern for the use of conivaptan in critical conditions or severe symptomatic hyponatremia is the potential for inaccurate predictions for the correction rate of serum [Na+] where prompt and precise corrections are key factors in assuring positive outcomes.
Conclusions
To date, V2 antagonism to induce aquaresis appears to be the most physiological approach to correct hyponatremia associated with dysregulated AVP. Intravenous conivaptan is the first dual V1A/V2 receptor antagonist approved by the FDA for the treatment of euvolemic and hypervolemic hyponatremia in hospitalized patients. However, the use of conivaptan cannot be routinely recommended for CHF patients due to insufficient efficacy and safety data.
Although conivaptan has been shown to be an effective aquaretic with short-term use, its use is not without limitations. Adverse effects reported with short-term use are typically minimal, but may include serious effects including hypokalemia, orthostatic hypotension, and unexpectedly rapid serum [Na+] correction. Data to support the chronic use of conivaptan are currently lacking. Nevertheless, long-term use of conivaptan may eventually prove to be problematic due to its multiple drug-drug interactions. Finally, it must be noted that the aquaretic effect induced by conivaptan is not prolonged when the medication is discontinued.
Careful patient selection, avoidance of combined use with conventional diuretics, and close monitoring may reduce complication rates with the use of conivaptan. The decision to use conivaptan over traditional therapeutic options for euvolemic and hypervolemic hyponatremic patients must be individualized while our current understanding of the systemic and long-term effects of dual V1A/V2 receptor antagonism is limited.
References
- AdroguéHJMadiasNEHyponatremiaN Engl J Med20003421581910824078
- AndreoliTEWater: Normal balance, hyponatremia, and hypernatremiaRen Fail2000227113511104160
- ArieffAILlachFMassrySGNeurological manifestations and morbidity of hyponatremia: Correlation with brain water and electrolytesMedicine (Baltimore)19765512191256311
- Astellas Pharma US, IncVaprisol (conivaptan hydrochloride injection) product monograph2007Deerfield, IL
- BartterFCSchwartzWBThe syndrome of inappropriate secretion of antidiuretic hormoneAm J Med1967427908065337379
- BaylisPHRegulation of vasopressin secretionBaillieres Clin Endocrinol Metab19893313302698140
- BaylisPHThe syndrome of inappropriate antidiuretic hormone secretionInt J Biochem Cell Biol2003351495912824060
- BennaniSLAbouqalRZeggwaghAAIncidence, causes and prognostic factors of hyponatremia in intensive careRev Med Interne200324224912706778
- BerlTTreatment of the syndrome of inappropriate antidiuretic hormone secretion and the emergence of vasopressin antagonists for hyponatremic disorders2007May 2007 [online] URL: http://www.nephrologyrounds.org
- BernierVMorelloJPZarrukAPharmacologic Chaperones as a Potential Treatment for X-Linked Nephrogenic Diabetes InsipidusJ Am Soc Nephrol2006172324316319185
- BorroniGMaggiASangiovanniAClinical relevance of hyponatraemia for the hospital outcome of cirrhotic patientsDig Liver Dis2000326051011142560
- BurnierMFrickerAFHayozDPharmacokinetic and pharmacodynamic effects of YM087, a combined V1/V2 vasopressin receptor antagonist in normal subjectsEur J Clin Pharmacol199955633710638391
- CardenasAArroyoVMechanisms of water and sodium retention in cirrhosis and the pathogenesis of ascitesBest Pract Res Clin Endocrinol Metab2003176072214687592
- DavisonJMVallottonMBLindheimerMDPlasma osmolality and urinary concentration and dilution during and after pregnancy: Evidence that lateral recumbency inhibits maximal urinary concentrating abilityBr J Obstet Gynaecol19818847297236550
- DeVitaMVGardenswartzMHKoneckyAIncidence and etiology of hyponatremia in an intensive care unitClin Nephrol19903416362257702
- DunnFLBrennanTJNelsonAEThe role of blood osmolality and volume in regulating vasopressin secretion in the ratJ Clin Invest197352321294750450
- FraserCLArieffAIEpidemiology, pathophysiology, and management of hyponatremic encephalopathyAm J Med199710267779209203
- FriedLFPalevskyPMHyponatremia and hypernatremiaMed Clin North Am1997815856099167647
- FujiwaraTMBichetDGMolecular biology of hereditary diabetes insipidusJ Am Soc Nephrol20051628364616093448
- GattoneVHWangXHarrisPCInhibition of renal cystic disease development and progression by a vasopressin V2 receptor antagonistNat Med200391323614502283
- GerbesALGulbergVGinesPTherapy of hyponatremia in cirrhosis with a vasopressin receptor antagonist: Arandomized double-blind multicenter trialGastroenterology2003124933912671890
- GhaliJKKorenMJTaylorJFEfficacy and safety of oral conivaptan: a V1A/V2 vasopressin receptor antagonist, assessed in a randomized, placebo-controlled trial in patients with euvolemic or hypervolemic hyponatremiaJ Clin Endocrinol Metab20069121455216522696
- GhaliJKSmithNEfficacy of the vasopressin V1A/V2 antagonist conivaptan in patients with euvolemic or hypervolemic hyponatremiaJ Am Soc Nephrol200415355A abstr SA-P0251
- GheorghiadeMGattisWAO’ConnorCMEffects of tolvaptan, a vasopressin antagonist, in patients hospitalized with worsening heart failure: A randomized controlled trialJAMA200429119637115113814
- GheorghiadeMNiaziIOuyangJVasopressin V2-receptor blockade with tolvaptan in patients with chronic heart failure: Results from a double-blind, randomized trialCirculation20031072690612742979
- GoldbergAHammermanHPetcherskiSPrognostic importance of hyponatremia in acute ST-elevation myocardial infarctionAm J Med2004117242815308433
- GrossPBisahaJGSmithNConivaptan, a novel V1A and V2 antagonist, increased serum sodium and effective water clearance in patients with euvolemic or hypervolemic hyponatremiaJ Am Soc Nephrol200415355A abstr SA-P0243
- Hew-ButlerTAlmondCAyusCExercise-Associated Hyponatremia (EAH) Consensus PanelClin J Sport Med2005152081316003032
- IshikawaSESchrierRWPathophysiological roles of arginine vasopressin and aquaporin-2 in impaired water excretionClin Endocrinol200358117
- LandwehrMHulingUStudy shows vaprisol® is effective in increasing serum sodium levels and well-tolerated in patients with euvolemic or hypervolemic hyponatremia2007 Astella News Release, http://www.astellas.us/press_room/docs
- LeeCRWatkinsMLPattersonJHVasopressin: A new target for the treatment of heart failureAm Heart J200314691812851603
- LeeCTGuoHRChenJBHyponatremia in the emergency departmentAm J Emerg Med200018264810830680
- MarkowitzGSRadhakrishnanJKambhamNLithium nephrotoxicity: a progressive combined glomerular and tubulointerstitial nephropathyJ Am Soc Nephrol20001114394810906157
- MillerPDLinasSLSchrierRWPlasma demeclocycline levels and nephrotoxicity. Correlation in hyponatremic cirrhotic patientsJAMA1980243251356770106
- PhamPCPhamPMPhamPTVasopressin excess and hyponatremiaAm J Kidney Dis2006477273716632011
- RobbenJHKnoersNVDeenPMCell biological aspects of the vasopressin type-2 receptor and aquaporin 2 water channel in nephrogenic diabetes insipidusAm J Physiol Renal Physiol2006291F2577016825342
- RobertsonGLPhysiology of ADH secretionKidney Int, Suppl198721S2063476800
- RobinsonAGRobertsMMEvronWAHyponatremia in rats induces downregulation of vasopressin synthesisJ Clin Invest199086102392211999
- RouleauJ-LPackerMMoyeLPrognostic value of neurohumoral activation in patients with an acute myocardial infarction: effect of captoprilJ Am Coll Cardiol199424583917915733
- RufAEKremersWKChavezLLAddition of serum sodium into the MELD score predicts waiting list mortality better than MELD aloneLiver Transpl2005113364315719386
- SedlacekMSchoolwerthACRemillardBDElectrolyte disturbances in the intensive care unitSemin Dial20061949650117150050
- SerradeilLe GalCWagnonJValetteGNonpeptide vasopressin receptor antagonists: Development of selective and orally active V1A, V2 and V1B receptor ligandsProg Brain Res200213919721012436936
- SingerIRotenbergDDemeclocycline-induced nephrogenic disbetes insipidus. In vivo and in vitro studiesAnn Intern Med197379679834356453
- SladekCDRegulation of vasopressin release by neurotransmitters, neuropeptides and osmotic stimuliProg Brain Res19836071906198689
- SoupartAGrossPLegrosJ-JSuccessful long-term treatment of hyponatremia in syndrome of inappropriate antidiuretic hormone secretion with satavaptan (SR121463B), an orally active nonpeptide vasopressin V2- receptor antagonistClin J Am Soc Nephrol2006111546017699341
- TerzianCFryeEBPiotrowskiZHAdmission hyponatremia in the elderly: Factors influencing prognosisJ Gen Intern Med1994989918164083
- ThibonnierMColesPThibonnierAThe basic and clinical pharmacology of nonpeptide vasopressin receptor antagonistsAnnu Rev Pharmacol Toxicol20014117520211264455
- TorresVEHarrisPCMechanisms of Disease: autosomal dominant and recessive polycystic kidney diseasesNat Clin Pract Nephrol20062405516932388
- TorresVEWangXQianQEffective treatment of an orthologous model of autosomal dominant polycystic kidney diseaseNat Med200410363414991049
- UdelsonJESmithWBHendrixGHAcute hemodynamic effects of conivaptan, a dual V1A and V2 vasopressin receptor antagonist, in patients with advanced heart failureCirculation200110424172311705818
- VerbalisJGBisahaJGSmithNNovel vasopressin V1A and V2 antagonist conivaptan increases serum sodium concentration and effective water clearance in hyponatremiaJ Am Soc Nephrol200415356A abstr SA-P0254
- VerbalisJGDisorders of body water homeostasisBest Pract Res Clin Endocrinol Metab20031747150314687585
- [VHA] VHA Pharmacy Benefits Management Strategic Healthcare Group and the Medical Advisory PanelNational PBM Drug Monograph: Conivaptan Hydrochloride Injection (Vaprisol®)2006
- WongFBleiATBlendisLMA vasopressin receptor antagonist (VPA-985) improves serum sodium concentration in patients with hyponatremia: A multicenter, randomized, placebo-controlled trialHepatology2003371829112500203
- ZerbeRStropesLRobertsonGVasopressin function in the syndrome of inappropriate antidiuresisAnnu Rev Med198031315276772090