Abstract
Hypertension, atherothrombosis, myocardial infarction, stroke, peripheral vascular disease, and renal failure are the main manifestations of cardiovascular disease (CVD), the leading cause of death and disability in developed countries. Continuing insight into the pathophysiology of CVD can allow identification of effective therapeutic strategies to reduce the occurrence of death and/or severe disabilities. In this context, a healthy endothelium is deemed crucial to proper functioning and maintenance of anatomical integrity of the vascular system in many organs. Of note, epidemiologic studies indicate that the incidence of CVD in women is very low until menopause and increases sharply thereafter. The loss of protection against CVD in post-menopausal women has been chiefly attributed to ovarian steroid deficiency. However, besides steroids, the ovary also produces the peptide hormone relaxin (RLX), which provides potent vasoactive effects which render it the most likely candidate as the elusive physiological shield against CVD in fertile women. In particular, RLX has a specific relaxant effect on peripheral and coronary vasculature, exerted by the stimulation of endogenous nitric oxide (NO) generation by cells of the vascular wall, and can induce angiogenesis. Moreover, RLX inhibits the activation of inflammatory leukocytes and platelets, which play a key role in CVD. Experimental studies performed in vascular and blood cell in vitro and in animal models of vascular dysfunction, as well as pioneer clinical observations, have provided evidence that RLX can prevent and/or improve CVD, thus offering background to clinical trials aimed at exploring the broad therapeutic potential of human recombinant RLX as a new cardiovascular drug.
The endothelium as a key player in vascular health and disease
Hypertension, atherothrombosis, myocardial infarction, stroke, peripheral vascular disease, and renal failure are the main manifestations of cardiovascular disease (CVD), which is the leading cause of death and disability in most developed countries, also due to the progressive increase in people’s life span and expectancy (Wey 1992). Therefore, action should be taken to prevent CVD before its signs and symptoms appear or severe outcome, such as myocardial infarction or stroke, is experienced. In this context, there is a general agreement between basic and clinical scientists that a healthy endothelium is crucial to allow proper functioning and maintenance of anatomical integrity of the vascular system in many organs, including the heart, brain, kidney, lung, and placenta. Conversely, damaged or dysfunctional endothelium substantially contributes to the pathogenesis and complications of CVD (CitationMensah 2007; CitationOsto et al 2007). Public health interventions for health promotion and prevention of CVD and its associated risk factors often target the endothelium, and further understanding of endothelial function can help develop innovative approaches.
Once viewed as a mere cell lining of the blood vessels serving as a barrier between the blood and the tissue extracellular matrix, the endothelium has been more recently recognized to be far more complex and functional (). In fact, endothelial cells play a major role in the regulation of vascular tone, hemostatic/fibrinolytic balance, angiogenesis, and leukocyte trafficking (CitationMensah 2007). Many of the functional properties of the endothelial cells are attributable to their unique ability to synthesize and release a variety of vasoactive mediators, including nitric oxide (NO), a gaseous free radical and key signalling molecule (CitationMoncada et al 1991). Intact endothelial cells constitutively express NO synthase III, which continuously generates nanomolar NO amounts. In turn, endothelium-derived NO accounts for vascular smooth muscle cell relaxation and vasodilatation (CitationPalmer et al 1987; CitationMoncada et al 1991), angiogenic response (CitationZiche et al 1994), and anti-adhesive properties of the inner endothelial surface to platelets (CitationRadomski et al 1987) and leukocytes (CitationKubes et al 1991) by down-regulation of endothelial cell adhesion molecules (CitationSluiter et al 1993). Therefore, failure of endothelial NO pathway and increased oxidative stress with loss of the protective effect of NO tips the endothelial cell balance towards a pro-atherogenic and pro-thrombotic milieu (CitationNedeljkovic et al 2003) and can initiate a vicious cycle of events that result in vasomotor dysfunction and vascular inflammation, which are essential components in the initiation and evolution of atherosclerosis (CitationNapoli et al 2006). On these grounds, it is conceivable that agents that increase NO availability and reduce endothelial dysfunction may decrease susceptibility to CVD.
A typical paradigm of the key importance of endothelial integrity for normal vascular function is represented by the pathogenic cascade initiated and sustained by cigarette smoke (CitationAmbrose and Barua 2004). Smoking is known to increase the incidence of peripheral and coronary artery diseases, myocardial infarction and reduced foeto-placental perfusion and foetal growth restriction (CitationJonas et al 1992; CitationPrice et al 1999; CitationKalinka et al 2005; CitationZdravkovic et al 2005). Experimental and clinical investigations have provided evidence that cigarette smoke causes endothelial cell dysfunction, mainly consistent in decreased generation and bioavailability of NO, which results in defective endothelium-dependent vasodilatation and increased susceptibility to leukocyte and platelet adhesion (CitationBarua et al 2001, Citation2002). Cigarette smoke is an example of the many dietary and lifestyle habits and inherited or acquired diseases predisposing to CVD, which also include obesity, hyperlipemia, diabetes, hypertension, vasculitis, and so on. In all these cases, the dysfunctional endothelium emerges as a crucial pathogenic factor and potential target for early diagnostic, preventative, and curative strategies.
Gender-related differences in CVD and the role of ovarian sex hormones
Clinical and epidemiologic studies have shown that men and women suffering from CVD differ in pathophysiology, time for onset, and prognosis upon treatment. The incidence of CVD in women is very low until menopause and increases sharply thereafter to become similar to men, but with a 10–20-year delay (CitationWenger et al 1993; CitationTunstall et al 1994; CitationHu et al 2000). The epidemiologic findings have been strengthened by the observation that, during fertile life, women have better endothelial function and vascular reactivity and higher NO biosynthesis than age-matched men (CitationForte et al 1998; Sader and Celermajer 2002). Female sex hormones have been obviously implicated in the protective effects of gender on the vasculature (CitationOrshal and Khalil 2004; CitationMendelsohn and Karas 2005). Most of the existing literature is focused on ovarian steroids, estrogen and progesterone, which can influence vascular functions by multiple mechanisms. Estrogen and progesterone receptors are expressed by the vascular endothelium and smooth muscle (CitationKhalil 2005): the interaction of ovarian steroids with cytosolic receptors triggers genomic effects leading to angiogenesis, endothelial NOS III up-regulation and smooth muscle growth inhibition; on the other hand, stimulation of plasma membrane receptors by these hormones initiates non-genomic effects that stimulate endothelium-dependent vasorelaxation via NO-cGMP, prostacyclin-cAMP and hyper-polarization pathways (CitationHayashi et al 1995; CitationKhalil 2005; CitationSiow et al 2007). Moreover, ovarian steroids can also influence vascular function by receptor-independent pathways, including direct antioxidant effects due to the presence of a phenolic group in their molecules (CitationSiow et al 2007), as well as indirect effects mediated by hypothalamic-pituitary feed-back inhibition (Sader and Celermajer 2002).
On the above grounds, the loss of protection against CVD in post-menopausal women has been chiefly attributed to the deficiency of ovarian steroids. Consequently, menopausal replacement therapy has been widely advocated for primary and secondary prevention (CitationGrodstein et al 1996). However, estrogen or progesterone have not been clearly identified as the protective agents in women. In fact, the results of estrogen/progestin replacement therapy are still controversial and under discussion (CitationHerrington et al 2000; CitationRosano et al 2006). The conclusions drawn from randomized studies carried out by the Heart and Estrogen/progestin Replacement Study (HERS) Research Group (CitationHulley et al 1998) and the Women’s Health Initiative Investigators (WHI) (CitationManson et al 2003), enrolling large cohorts of subjects, concur to indicate that estrogen plus progestin does not confer cardiac protection and may even increase the risk of CVD among generally healthy post-menopausal women, especially during the first year after the initiation of hormone use, most likely due to unfavorable effects of ovarian steroids upon platelet activation and coagulation (CitationBonnar 1987). Therefore, it has been recommended that estrogen/progestin replacement should not be prescribed for the prevention of cardiovascular disease (CitationManson et al 2003).
This ostensible paradox can find a logical explanation if bearing in mind that menopause causes the loss of ovarian function, which does not merely imply the cessation of estrogen and progesterone production. In fact, besides steroids, the ovary produces the peptide hormone relaxin (RLX), which also has vasoactive properties. RLX is secreted by the corpus luteum and is secreted at detectable levels in the blood of cycling and pregnant women, being absent – or at least undetectable with the current assay methods – in post-menopausal women and in men (CitationO’Byrne et al 1978; CitationEddie et al 1986; CitationKhan-Dawood et al 1989; CitationStewart et al 1990; CitationWinslow et al 1992). Could RLX be the elusive natural or physiological shield against CVD in fertile women? In the following chapters, the many points in favour of this hypothesis will be summarized and reviewed.
RLX, its cognate molecules, and their receptors
RLX was discovered in 1926 by Frederick Hisaw, who observed that the injection of serum from pregnant guinea pigs or rabbits into virgin guinea pigs induced significant elongation of the interpubic ligament, attributed to stimulation of collagen turn-over (CitationHisaw 1926). Later on, he and his colleagues isolated from pregnant sow corpora lutea a peptide responsible for the observed relaxant effect on pubic symphisis and named it ‘relaxin’ (CitationFevold et al 1930).It is worth noting that the collagen remodeling properties of RLX first highlighted by Hisaw have been confirmed by many subsequent investigations (CitationUnemori and Amento 1990; CitationUnemori et al 1992, Citation1993, Citation1996; CitationGarber et al 2001; CitationWilliams et al 2001; CitationMcDonald et al 2003; CitationSamuel et al 2003) and remain as a hallmark of RLX and a paradigm of its specific biological effects (CitationSherwood 2004).
RLX has been long included in the insulin hormone superfamily because of structural homologies with insulin: both peptides have similar 6 kDa molecular weights and are composed of A and B chains stabilized by inter- and intra-chain disulfide bonds (CitationSchwabe and Büllesbach 1994). More recently, however, it has been definitely ascertained that RLX is a member of a peptide hormone family that diverged from insulin early in vertebrate evolution; therefore, RLX has been assigned to a specific hormone family, termed the RLX peptide family, which includes three different RLXs, H1, H2 and H3, as well as insulin-like peptide (INSL)3, INSL4, INSL5 and INSL6 (CitationBathgate et al 2003; CitationSamuel et al 2006). In humans, 3 separate RLX genes have been found and designated RLN1, RLN2 and RLN3 (CitationBathgate et al 2003; CitationSamuel et al 2006). The peptide encoded by the RLN2 gene, H2 RLX, is the major circulating form and is produced mainly in the corpus luteum (CitationHudson et al 1984). Circulating RLX accounts for most of the known biological effects of the hormone in humans and experimental animals. RLX acts on the female reproductive system as well as on non-reproductive targets, including the cardiovascular system and the connective tissue (CitationBani 1997; CitationConrad and Novak 2004; CitationSherwood 2004; CitationDschietzig et al 2006; CitationSamuel et al 2006; CitationNistri et al 2007).
RLX is the ligand for two leucine-rich repeat-containing G-protein coupled receptors (LGRs), LGR7 and LGR8 (CitationHsu et al 2002), now classified as relaxin family peptide receptors 1 and 2 (RXFP1 and RXFP2), respectively (CitationBathgate et al 2006). RXFP1 is the main and most specific H2 RLX receptor, but it is also able to bind H1 and H3 RLX, although with less affinity. On the other hand, RXFP2 chiefly binds INSL3 and also H1 and H2 RLX, but with less affinity (CitationHsu et al 2002; CitationSudo et al 2003). These receptors have been found on most if not all RLX target tissues and cells, and are abundantly expressed in the reproductive, nervous, renal and cardiovascular systems (CitationHsu et al 2002). More recently, other G protein-coupled receptors for peptides of the relaxin family have been discovered and termed RXFP3 (formerly GPCR135) and RXFP4 (formerly GPCR142) (CitationBathgate et al 2006). RXFP3 binds H3 RLX with high affinity (CitationLiu et al 2003a) while RXFP4 binds H3 RLX (CitationLiu et al 2003b) and also INSL5 (CitationLiu et al 2005). Moreover, RLX has been reported to act as an agonist for cytoplasmic glucocorticoid receptors, thereby exerting possible cortisol-like effects (CitationDschietzig et al 2004). Binding of RLX to its surface receptor results in the activation of multiple intracellular response pathways, which include cAMP and NO as second messengers (CitationHsu et al 2002; CitationNistri and Bani 2003). In particular, the observation that RLX can up-regulate NO biosynthesis in several of its targets underscores its potential as cardiovascular hormone, NO being a key regulator of vascular function (CitationMoncada et al 1991; CitationNathan 1992).
RLX and the vascular system
Peculiarly, the first reports on the ability of RLX to influence blood vessels came from clinical rather than basic research. During the late 1950s and early 1960s, the Warner-Chilcott Laboratories provided an impure preparation of porcine RLX, commercially available as Releasin, and supported studies in humans that examined the use of relaxin as a therapeutic agent for scleroderma, cervical ripening and premature labor, based on its collagen-remodeling properties. Releasin, injected intramuscularly to patients suffering for peripheral vascular diseases and Raynaud’s syndrome, caused a dramatic, albeit transient, amelioration of symptoms and signs of ischemia (CitationCasten and Boucek 1958; CitationCasten et al 1960). These effects were attributed to increased compliance of the blood vessels due to loosening of their adventitial extracellular matrix. However, Casten and colleagues also reported that, in some patients who also suffered for ischemic heart disease, Releasin treatment led to the reduction of the daily glyceryl trinitrate requirements. Retrospectively, this important observation clearly suggests that RLX does have a direct, dilatory effect on peripheral and coronary vasculature. Subsequent studies in monkeys by the Hisaw’s group described an enlargement of endometrial arterioles and capillaries (CitationDallenbach-Hellweg et al 1966) and provided first evidence for an angiogenic effect of RLX on endothelial cells of the endometrial blood vessels (CitationHisaw 1967). Definite recognition of the blood vessels as specific RLX targets came from the identification of RLX binding sites/receptors on vascular cells in reproductive and non-reproductive organs (CitationMin and Sherwood 1996; CitationKohsaka et al 1998; CitationHsu et al 2002).
Vasodilatation may be regarded as an additional, specific hallmark of RLX, as it has been observed in many target organs and tissues, regardless of gender. RLX-induced vasodilatation mainly involves the distal segments of the vascular tree, namely arterioles provided with a smooth muscle coat as well as capillaries and post-capillary venules, which lack smooth muscle in their wall. Of note, RLX promotes vasodilatation in reproductive organs, such as the uterus (CitationVasilenko et al 1986; CitationBani G et al 1995) and the mammary gland (CitationBani et al 1988), as well as in non-reproductive targets, including mesocaecum (CitationBigazzi et al 1986), kidney (CitationDanielson et al 1999, Citation2000; CitationNovak et al 2001), liver (CitationBani et al 2001), lung (CitationBani et al 1997), and heart (CitationBani Sacchi et al 1995; CitationMasini et al 1997). Vasodilatation appears as a physiologic effect of RLX since it is fully manifest at hormone concentrations which are in the nanomolar range, similar to the RLX blood levels of normal human pregnancy (CitationO’Byrne et al 1978; CitationEddie et al 1986). RLX is extremely potent as a vasorelaxant: in the isolated, perfused rat and guinea pig heart, the dose-dependent increase in coronary flow induced by RLX is significantly higher than that obtained with similar doses of typical vasodilatatory agents such as acetylcholine or sodium nitroprusside (CitationBani Sacchi et al 1995); in the mesocaecum, RLX counteracted similar concentrations of norepinephine (CitationBigazzi et al 1986). Vasodilatation also emerges as a primary effect of RLX and is not secondary to tissue growth stimulation, as it could be appreciated even in the absence of any growth response.
Of note, the vasodilatatory effects of RLX are most prominent in vasoconstricted blood vessels, rather than in normal blood vessels, suggesting that this hormone could act as a natural regulator of vascular tone and a shield against the derangements of vascular reactivity. In the rat kidney, RLX reduces the myogenic activity of small arteries and blunts the vasoconstrictive response to angiotensin II, in both genders (CitationDanielson et al 1999, Citation2000; CitationNovak et al 2001). In a rat model of renal artery ligation, RLX counteracts the increase in systemic blood pressure due to activation of the renin-angiotensin system (CitationGarber et al 2003). In spontaneously hypertensive rats (SHR), but not normotensive rats, RLX reduces blood pressure and blunts the response of mesenteric vessels to vasoconstrictors (CitationSt Louis et al 1985).
Besides vasodilatation, RLX can also induce angiogenesis in some target organs, as suggested by the pioneer findings by CitationHisaw (1967), using partially purified RLX. In human endometrial cells in vitro, RLX induces the production of potent angiogenic molecules such as basic fibroblast growth factor (bFGF) and vascular endothelial growth factor (VEGF) (CitationUnemori et al 1999). In a rat model of chronic myocardial infarction, systemic infusion of RLX potentates bFGF mRNA expression in the peri-infarct region by both cardiomyocytes and fibroblasts (CitationLewis et al 2001). Similarly, in a swine model of post-infarcted heart, locally produced RLX by RLN2 gene-transfected myoblasts grafted into the post-ischemic myocardium, causes a significant increase in microvessel density and over-expression of VEGF mRNA by the host cardiac cells (CitationFormigli et al 2007). Thus, RLX, by promoting vasodilation and, at least in some conditions, angiogenesis could influence the perfusion of many target organs.
Sound experimental evidence exists that the vasoactive action of RLX can be chiefly ascribed to the prompt synthesis and release of the potent vasodilator NO (CitationMoncada et al 1991; CitationNathan 1992) by blood vessel cells. In vitro studies have shown that addition of RLX to the culture medium of endothelial cells from rat coronaries and human umbilical vein and of bovine vascular smooth muscle cells causes a dose-dependent increase in the production of NO and in the intracellular levels of cGMP (CitationBani et al 1998a; CitationFailli et al 2001; CitationQuattrone et al 2004), the mediator of the cell response to NO (CitationIgnarro 1991). This is accompanied by a concurrent decrease in cytosolic Ca2+ concentration and by morphological changes in cell shape and cytoskeletal contractile microfilaments consistent with cell relaxation (CitationBani et al 1998a). RLX activates endogenous NO biosynthesis by increasing the expression and activity of inducible NO synthase (NOS II) in both cell types (CitationBani et al 1998a; CitationFailli et al 2001; CitationQuattrone et al 2004). In keeping with these findings, RLX has been found to up-regulate endothelin type-B (ETB) receptors and the release of NO on the renal vascular endothelium in the rat in vivo (CitationConrad et al 1999). Based on the current literature, there are multiple pathways by which the G protein-coupled RLX receptors can stimulate NO generation (CitationNistri and Bani 2003). In endothelial cells, constitutive NO synthase (NOS III) activity is stimulated by vasoactive agonists and hormones, such as acetylcholine, bradykinin and estrogens, which also act by specific membrane-associated G protein-coupled receptors (CitationLiu et al 1995; CitationVanhoutte 1997; CitationWyckoff et al 2001). The classical signaling pathway involves G protein βγ subunits that, through phosphoinositide 3-kinase (PI3-K), switch on protein kinase B (Akt), which in turn activates NOS III by phosphorylation at Ser-1179 (CitationIgarashi and Michel 2001). Another mechanism, which may either coexist in the same cell or be alternatively operating in different cell types, could involve cAMP and the inducible NOS II isoform. In rat vascular smooth muscle cells, G protein-coupled receptor-activated adenylate cyclase and the consequent rise in cAMP upregulates protein kinase A (PKA) activity (CitationKim et al 2002). In turn, PKA is able to phosphorylate and inactivate IkB-α, the inhibitor subunit of the transcription factor NF-κB, thus allowing NF-κB to translocate into the nucleus and to promote the expression of NOS II (CitationGrimm and Baeuerle 1993). This latter mechanism may be also operating in the noted RLX vascular target cells, in which induction of NOS II expression and high-output, sustained NO generation have been observed (CitationBani et al 1998a; CitationFailli et al 2001). It has been also suggested that RLX may indirectly activate the NO pathway through the stimulation of extracellular matrix-remodeling. In fact, RLX-induced collagenase activation could convert big endothelin (ET)-1 into bioactive ET1–32, which in turn binds to and activates ETB receptors, thereby inducing NOS III activation and NO release (CitationConrad and Novak 2004).
Whichever intracellular mechanism is involved in the up-regulation of endogenous NO generation by vascular cells, RLX clearly emerges from the above studies as a major vasoactive hormone and potential drug able to influence vascular tone and, consequently, organ and tissue perfusion.
Relaxin and vascular dysfunction
As summarized in the introduction, endothelial dysfunction is a crucial event in the initiation and evolution of atherosclerosis and CVD (CitationNapoli et al 2006). The damaged endothelium becomes unable to generate vasoactive autacoids, chiefly NO and prostaglandins, and loses its physiological function to control vasomotion and blood cell adhesion (CitationNedeljkovic et al 2003). Thence, vasospasm and ischemia on one hand, and recruitment and activation of inflammatory leukocytes and platelets on the other hand, can occur and cause overall vascular and organ injury and degeneration. Multiple lines of evidence, both experimental and clinical, converge to suggest that RLX may beneficially interfere with this pathogenic cascade ().
Figure 2 Schematic diagram highlighting the role of the endothelium in the pathophysiology of vascular dysfunction and the targets for RLX action, which include i) up-regulation of NOS II and endogenous NO production in endothelial and vascular smooth muscle cells, leading to vascular relaxation; reduced expression of endothelial cell adhesion molecules; reduced adhesion and activation of neutrophils, leading to diminished oxidative stress and tissue injury; reduced platelet activation; angiogenesis.
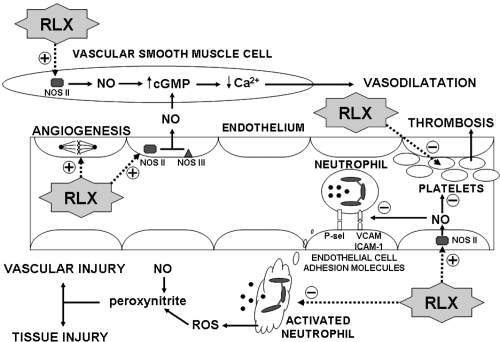
Our previous in vitro studies on vascular endothelial cells suggest that RLX could sustain endogenous NO generation by an alternate pathway to the constitutive NOS III, ie, by the expression of inducible NOS II (CitationFailli et al 2001; CitationQuattrone et al 2004). This mechanism may likely attain biological and pharmacological relevance when RLX is administered to functional endothelium to prevent the adverse effects of the different causes of endothelial failure, but it cannot be ruled out that it could also be operating on early damaged endothelium, thereby reverting initial NO failure and interrupting the vicious cycle that would further worsen endothelial injury and dysfunction. Sustained endothelial NO generation by RLX can counteract vasoconstriction as well as leukocyte adhesion and vascular inflammation. We have recently demonstrated that RLX can reduce the endothelial adhesiveness to neutrophils in pro-inflammatory conditions in vitro by down-regulating early- and late-phase endothelial cell adhesion molecules (CitationNistri et al 2003). Moreover, besides the endothelium, RLX can also influence the other aspect of vascular inflammation, ie, the leukocytes. In fact, RLX has been shown to strongly inhibit the activation of neutrophils challenged in vitro with inflammatory mediators, by reducing oxidative burst, reactive oxygen species (ROS) generation and chemotaxis (CitationMasini et al 2004). Finally, RLX has been reported to reduce markedly, and in a dose-dependent fashion, the aggregation of platelets (CitationBani et al 1995, Citation2007), which represents another pathogenic pillar of atherosclerosis. These multiple anti-inflammatory, anti-thrombotic effects of RLX rely on the stimulation of endogenous NO generation, but other concurrent mechanisms are likely to be involved. As cited in a earlier, it has been recently demonstrated that RLX may act as a glucocorticoid receptor agonist by a RXFP receptor-independent pathway, thereby mimicking some of the anti-inflammatory effects of cortisol (CitationDschietzig et al 2004).
The recent literature reports several experimental studies on animal models of disease whose results validate the above theoretical concept that RLX may be beneficial in CVD. In particular, we have provided evidence that RLX, administered to isolated, perfused guinea pig hearts or to intact rats in vivo, protects the heart against myocardial injury caused by ischemia and reperfusion (IR) (CitationMasini et al 1997; CitationBani et al 1998b). During IR, there is a primary damage of the coronary endothelium leading to impaired production of NO. This triggers a cascade of events, such as vasoconstriction, leukocyte and platelet recruitment, ROS generation and oxidative stress, mast cell activation and histamine release and so on, which result in myocardial contractile dysfunction and, eventually, irreversible myocardial injury (CitationLefer et al 1991). RLX, given preventatively before ischemia, caused a significant reduction of the myocardial tissue area damaged by post-ischemic reperfusion, as well as reducing the occurrence of severe ventricular arrhythmias. RLX also reduced myocardial neutrophil accumulation, platelet and mast cell activation, ROS-induced tissue injury and histopathological signs of irreversible cardiomyocyte and endothelial injury (CitationMasini et al 1997; CitationBani et al 1998b). The cardioprotective action of RLX can go beyond the prevention of IR-induced myocardial damage and may find a therapeutic application in myocardial infarction. Recently, we used a swine model of cardiac IR in which RLX was administered at reperfusion to reproduce an adjunctive medical therapy to primary coronary angioplasty in patients with acute myocardial infarction. In these studies, RLX, given at reperfusion upon a 30-min ischemia at doses yielding physiological or juxta-physiological plasma concentrations, caused a marked, dose-related reduction of the main serum markers of myocardial damage as well as of the metabolic and histopathological parameters of cardiomyocyte injury and apoptosis and myocardial inflammation. These effects of RLX resulted in increased myocardial salvage and improved ventricular performance (CitationBani et al 2005; CitationPerna et al 2005). These findings provide support to the above concept that RLX may be also effective on the endothelium damaged by ischemia, likely counteracting the NO failure and preventing severe endothelial injury and dysfunction. Our pioneering studies on RLX-induced protection in cardiac IR have been confirmed and extended by a recent study on rats with isoproterenol-induced cardiac ischemia, in which the assayed parameters of myocardial damage and dysfunction improved markedly upon treatment with exogenous RLX (CitationZhang et al 2005). Of note, in this study, the injured heart also appears to up-regulate the production and release of endogenous RLX, providing support to the view that RLX is a cardiac hormone (CitationTaylor and Clark 1994) and may have paracrine effects against cardiac ischemic injury and dysfunction (CitationDschietzig et al 2001). As occurs in the heart, RLX has been shown to protect against IR-induced injury in other organs, such as the liver (CitationBoehnert et al 2005), the small bowel (CitationMasini et al 2006), the pancreas (CitationCosen-Binker et al 2006) and the brain (CitationWilson et al 2006). In all these studies, direct or indirect evidence is provided that the protection afforded by RLX relies on its effects on the endothelium and often involve endogenous NO generation.
From a clinical perspective, evidence for the efficacy of RLX as a vascular protector emerges from the early studies of CitationCasten and colleagues (1960), who successfully used RLX to treat patients with peripheral vascular diseases and obtained impressive amelioration of peripheral arteriopathy and ischemic ulcers. Moreover, in subjects also suffering from ischemic heart disease, RLX reduced the need for daily nitrovasodilatator therapy. Possible criticisms raised by the use of an impure RLX preparation are overridden by a more recent observation that clinical grade human recombinant RLX, given as merciful therapy to a patient with severe idiopathic occlusive peripheral arterial disease of the lower limbs, caused a marked, long-lasting amelioration of both subjective symptoms and objective clinical and instrumental signs (CitationBani and Bigazzi 2005). This clinical experience, albeit limited to a single patient, confirms the findings of Casten and colleagues and gives further momentum to future clinical studies in this direction.
Another clinical issue highlighting the vasoprotective role of RLX and its possible therapeutical use is pre-eclampsia, a disease of late pregnancy characterized by de novo occurrence of hypertension and proteinuria and by histopathological signs of peripheral and placental endothelial injury. Its occurrence is the leading cause of pre-term delivery and fetal growth restriction and predisposes both the offspring and the mother to subsequent cardiovascular risk (CitationSmith et al 2001). Recently, compelling evidence has been brought forth suggesting a major role for soluble growth factor receptor fms-like tyrosine kinase 1, asymmetric dimethyl arginine and autoantibodies in the pathophysiology of pre-eclampsia. These molecules are present at abnormally high levels in the blood of pre-eclamptic women and lead to inactivation of circulating pro-angiogenic factors, inhibition of endothelial NO biosynthesis and stimulation of angiotensin receptors, thereby causing pre-eclampsia (CitationDavison et al 2004). As RLX can increase renal vasodilation and hyperfiltration, reduce myogenic reactivity of small renal arteries through activation of the endothelin B receptor-NO pathway (CitationDanielson et al 1999, Citation2000; CitationNovak et al 2001) and stimulate endothelial NO generation (CitationBani et al 1998a; CitationFailli et al 2001; CitationQuattrone et al 2004), it appears the perfect candidate to counteract the pathogenic events of pre-eclampsia. To test this hypothesis a randomized, double-blind, placebo controlled clinical trial is currently in progress in the United States (http://www.clinicaltrials.gov; No NCT00333307) sponsored by BAS Medical, owner of the patent for human recombinant RLX. Hopefully, this study could provide exciting new suggestions for the possible clinical use of RLX in pre-eclamptic patients.
Concluding remarks
The studies that I have briefly summarized in the above paragraphs offer clear-cut evidence that RLX has specific effects on the vascular and blood cells which may help counteract the different pathogenic aspects of CVD. Many clues also suggest that RLX could be the elusive ovarian hormone involved in the protection of fertile women from CVD. The experimental studies, especially those performed in animal models of vascular dysfunction, provide background to current and future clinical trials aimed at exploring the broad therapeutic potential of human recombinant RLX as a new cardiovascular drug.
References
- AmbroseJABaruaRSThe pathophysiology of cigarette smoking and cardiovascular diseaseJ Am Coll Cardiol2004431731715145091
- BaniDBigazziMMasiniERelaxin depresses platelet aggregation: in vitro studies on isolated human and rabbit plateletsLab Invest199573709167474945
- BaniDRelaxin, a pleiotropic hormoneGen Pharmacol19972813229112071
- BaniDBallatiLMasiniERelaxin counteracts asthma-like reaction induced by inhaled antigen in sensitized guinea pigsEndocrinology19971381909159112386
- BaniDFailliPBelloMGRelaxin activates the L-arginine-nitric oxide pathway in vascular smooth muscle cells in cultureHypertension1998a31124079622136
- BaniDMasiniEBelloMGRelaxin protects against myocardial injury caused by ischemia and reperfusion in rat heartAm J Pathol1998b1521367769588905
- BaniDNistriSQuattroneSThe vasorelaxant hormone relaxin induces changes in liver sinusoid microcirculation: a morphologic study in the ratJ Endocrinol2001171541911739020
- BaniDBigazziMClinical aspects and therapeutic perspectives of relaxinCurr Med Chem – Immunology, Endocrine and Metabolic Agents (CMC-IEMA)2005540310
- BaniDNistriSBani SacchiTBasic progress and future therapeutic perspectives of relaxin in ischemic heart diseaseAnn N Y Acad Sci200510414233015956740
- BaniDNistriSCinciLA novel, simple bioactivity assay for relaxin based on inhibition of platelet aggregationRegul Peptides2007in press
- BaniGBani SacchiTBigazziMEffects of relaxin on the microvasculature of mouse mammary glandHistol Histopath1988333743
- BaniGMauriziMBigazziMEffects of relaxin on the endometrial stroma. Studies in miceBiol Reprod199553253627492676
- Bani SacchiTBigazziMBaniDRelaxin-induced increased coronary flow through stimulation of nitric oxide productionBrit J Pharmacol19951161589948564223
- BaruaRSAmbroseJAEales-ReynoldsLJDysfunctional endothelial nitric oxide biosynthesis in healthy smokers with impaired endothelium-dependent vasodilatationCirculation200110419051011602492
- BaruaRSAmbroseJASahaDCSmoking is associated with altered endothelial-derived fibrinolytic and antithrombotic factors: an in vitro demonstrationCirculation2002106905812186791
- BathgateRASamuelCSBurazinTCRelaxin: new peptides receptors and novel actionsTrends Endocrinol Metab2003142071312826326
- BathgateRAIvellRSanbornBMInternational Union of Pharmacology LVII: Recommendations for the nomenclature of receptors for relaxin family peptidesPharmacol Rev20065873116507880
- BigazziMDel MeseAPetrucciFThe local administration of relaxin induces changes in the microcirculation of the rat mesocaecumActa Endocrinol198611229693739555
- BoehnertMUHilbigHArmbrusterFPRelaxin as an additional protective substance in preserving and reperfusion solution for liver transplantation shown in a model of isolated perfused rat liverAnn N Y Acad Sci200510414344015956742
- BonnarJCoagulation effects of oral contraceptionAm J Obstet Gynecol1987157104282960241
- CastenGGBoucekRJUse of relaxin in the treatment of sclerodermaJAMA195816631924
- CastenGGGilmoreHRHoughtonFEA new approach to the management of obliterative peripheral arterial diseaseAngiology1960114081413691417
- ConradKPGandleyREOgawaTEndothelin mediates renal vasodilation and hyperfiltration during pregnancy in chronically instrumented conscious ratsAm J Physiol Renal Physiol1999276F76776
- ConradKPNovakJEmerging role of relaxin in renal and cardiovascular functionAm J Physiol Regul Integr Comp Physiol200428725061
- Cosen-BinkerLIBinkerMGCosenRRelaxin prevents the development of severe acute pancreatitisWorld J Gastroenterol20061215586816570348
- Dallenbach-HellwegGDawsonABHisawFLThe effect of relaxin on the endometrium of monkeys-histological and histochemical studiesAm J Anat19661196178
- DanielsonLASherwoodODConradKPRelaxin is a potent renal vasodilator in conscious ratsJ Clin Invest19991035253310021461
- DanielsonLAKercherLJConradKPImpact of gender and endothelin on renal vasodilation and hyperfiltration induced by relaxin in conscious ratsAm J Physiol Regul Integr Comp Physiol2000279R129830411003996
- DavisonJMHomuthVJeyabalanANew aspects in the pathophysiology of preeclampsiaJ Am Soc Nephrol2004152440815339993
- DschietzigTRitcherCBartschCThe pregnancy hormone relaxin is a player in human heart failureFASEB J200116521879511641245
- DschietzigTBartschCStanglVIdentification of the pregnancy hormone relaxin as glucocorticoid receptor agonistFASEB J2004131536815289446
- DschietzigTBartschCBaumannGRelaxin – a pleiotropic hormone and its emerging role for experimental and clinical therapeuticsPharm Ther20061123856
- EddieLWBellRJLesterARadioimmunoassay of relaxin in pregnancy with an analogue of human relaxinLancet19868494134462872469
- FailliPNistriSQuattroneSRelaxin up-regulates inducible nitric oxide synthase expression and nitric oxide generation in rat coronary endothelial cellsFASEB J200116252411744624
- FevoldHLHisawFLMeyerRKThe relaxative hormone of the corpus luteum. Its purification and concentrationJ Am Chem Soc19305233408
- FormigliLPernaAMMeacciEParacrine effects of transplanted myoblasts and relaxin on post-infarction heart remodelingJ Cell Mol Med2007111101417979885
- FortePKnealeBJMilneEEvidence for a difference in nitric oxide biosynthesis between healthy women and menHypertension19983273049774371
- GarberSLMirochnikYBrecklinCSRelaxin decreases renal interstitial fibrosis and slows progression of renal diseaseKidney Int2001598768211231342
- GarberSLMirochnikYBrecklinCEffect of relaxin in two models of renal mass reductionAm J Nephrol20032381212373075
- GrimmSBaeuerlePAReview article: the inducible transcription factor NF–κB: structure-function relationship of its protein subunitsBiochem J19932902973088452515
- GrodsteinFStampferMJMansonJEPostmenopausal estrogen and progestin use and the risk of cardiovascular diseaseN Engl J Med1996335453618672166
- HayashiSYamadaKEsakiTestrogen increases endothelial nitric oxide by a receptor-mediated systemBiochim Biophys Res Commun199521484755
- HerringtonDMReboussinDMBrosnihanKBEffects of estrogen replacement on the progression of coronary-artery atherosclerosisN Engl J Med2000343522910954759
- HisawFLExperimental relaxation of the pubic ligament of the guinea pigProc Soc Exp Biol Med1926236613
- HisawFLHisawFLJrDawsonABEffect of relaxin on the endothelium of endometrial blood vessels in monkey (Macaca mulatta)Endocrinology196781375854952013
- HsuSYNakabayashiKNishiSActivation of orphan receptors by the hormone relaxinScience2002295671411809971
- HuFBStampferMJMansonJETrends in the incidence of coronary heart disease and changes in diet and lifestyle in womenN Engl J Med2000343530710954760
- HudsonPJohnMCrawfordRRelaxin gene expression in human ovaries and the predicted structure of a human preprorelaxin by analysis of cDNA clonesEMBO J19843233396548702
- HulleySGradyDBushTRandomized trial of estrogen plus progestin for secondary prevention of coronary heart disease in postmenopausal women. Heart and Estrogen/progestin Replacement Study (HERS) Research GroupJAMA1998280605139718051
- IgarashiJMichelTSphingosine 1-phosphate and isoform-specific activation of phosphoinositide 3-kinase beta. Evidence for divergence and convergence of receptor-regulated endothelial nitric-oxide synthase signaling pathwaysJ Biol Chem200127636281811470796
- IgnarroLJSignal transduction mechanisms involving nitric oxideBiochem Pharmacol199141485901847633
- JonasMAOatesJAOckeneJKStatement on smoking and cardiovascular disease for health care professionals. American Heart AssociationCirculation199286166491423984
- KalinkaJHankeWSobalaWImpact of prenatal tobacco smoke exposure, as measured by midgestation serum cotinine levels, on fetal biometry and umbilical flow velocity waveformsAm J Perinatol20052241715668843
- KhalilRASex hormones as potential modulators of vascular function in hypertensionHypertension2005462495415983238
- Khan-DawoodFSGoldsmithLTWeissGHuman corpus luteum secretion of relaxin oxytocin and progesteroneJ Clin Endocrinol Metab198968627312918060
- KimNYPaeHOKimYCPentoxifylline potentiates nitric oxide production in interleukin-1beta-stimulated vascular smooth muscle cells through cyclic AMP-dependent protein kinase A pathwayGen Pharmacol2002352051111827727
- KohsakaTMinGLukasGIdentification of specific relaxin-binding cells in the human femaleBiol Reprod19985999199746753
- KubesPSuzukiMGrangerDNNitric oxide: an endogenous modulator of leukocyte adhesionProc Natl Acad Sci USA199188465151675786
- LeferAMTsaoPSLeferDJRole of endothelial dysfunction in the pathogenesis of reperfusion injury after myocardial ischemiaFASEB J199152029342010056
- LewisMDeshpandeUGuzmanLTregearGWIvellRBathgateRAWadeDJSystemic relaxin administration stimulates angiogenic cytokine expression and vessel formation in a rat myocardial infarct modelDordrecht2001Relaxin 2001: Proceedings of the 3rd international conference on relaxin and related peptidesThe Netherlands: Kluwer15967
- LiuJConklinBRBlinNIdentification of a receptor/G-protein contact site critical for signaling specificity and G-protein activationProc Natl Acad Sci USA1995921164268524820
- LiuCChenJSuttonSIdentification of relaxin-3/INSL7 as a ligand for GPCR142J Biol Chem2003a278507657014522967
- LiuCEristeESuttonSIdentification of relaxin-3/INSL7 as an endogenous ligand for the orphan G-protein-coupled receptor GPCR135J Biol Chem2003b278507546414522968
- LiuCKueiCSuttonSINSL5 is a high affinity specific agonist for GPCR142 (GPR100)J Biol Chem200528029230015525639
- MansonJEHsiaJJohnsonKCWomen’s Health Initiative InvestigatorsEstrogen plus progestin and the risk of coronary heart diseaseN Engl J Med20033495233412904517
- MasiniEBaniDBelloMGRelaxin counteracts myocardial damage induced by ischemia-reperfusion in isolated guinea pig hearts: evidence for an involvement of nitric oxideEndocrinology19971384713209348198
- MasiniENistriSVannacciARelaxin inhibits the activation of human neutrophils: involvement of the nitric oxide pathwayEndocrinology200414511061214630720
- MasiniECuzzocreaSMazzonEProtective effects of relaxin in ischemia/reperfusion-induced intestinal injury due to splanchnic artery occlusionBrit J Pharmacol200614811243216847443
- McDonaldGASarkarPRennkeHRelaxin increases ubiquitin-dependent degradation of fibronectin in vitro and ameliorates renal fibrosis in vivoAm J Physiol Renal Physiol2003285F59F6712820641
- MendelsohnMEKarasRHMolecular and cellular basis of cardiovascular gender differencesScience20053081583715947175
- MensahGAHealthy endothelium: the scientific basis for cardiovascular health promotion and chronic disease preventionVasc Pharmacol2007463104
- MinGSherwoodODIdentification of specific relaxin-binding sites in the cervix mammary glands nipples, small intestine and skin of pregnant pigsBiol Reprod1996551243528949880
- MoncadaSPalmerRMJHiggsEANitric oxide physiology pathophysiology, and pharmacologyPharmacol Rev199143109421852778
- NapoliCde NigrisFWilliams-IgnarroSNitric oxide and atherosclerosis: an updateNitric Oxide2006152657916684613
- NathanCNitric oxide as a secretory product of mammalian cellsFASEB J199263051641381691
- NedeljkovicZSGokceNLoscalzoJMechanisms of oxidative stress and vascular dysfunctionPostgrad Med, J200379195912743334
- NistriSBaniDRelaxin receptors and nitric oxide synthases. Search for the missing linkReprod Biol Endocrinol20031512646076
- NistriSChiappiniLSassoliCRelaxin inhibits lipopolysaccharide-induced adhesion of neutrophils to coronary endothelial cells by a nitric oxide-mediated mechanismFASEB J20031721091114500542
- NistriSBigazziMBaniDRelaxin as a cardiovascular hormone. Physiology, pathophysiology and therapeutic promisesCardiovasc Hematol Agents Med Chem (CHA-MC)200751018
- NovakJDanielsonLAKerchnerLJRelaxin is essential for renal vasodilation during pregnancy in conscious ratsJ Clin Invest200110714697511390429
- O’ByrneEMCarriereBTSorensenLPlasma immunoreactive relaxin levels in pregnant and nonpregnant womenJ Clin Endocrinol Metab197847110610263340
- OrshalJMKhalilRAGender, sex hormones and vascular toneAm J Physiol Regul Integr Comp Physiol2004286R2334914707008
- OstoECoppolinoGVolpeMRestoring the dysfunctional endotheliumCurr Pharm Des20071310536817430168
- PalmerRMJFerrigeAMoncadaSNitric oxide release accounts for the biological activity of endothelium-derived relaxing factorNature198732752463495737
- PernaAMMasiniENistriSNovel drug development opportunity for relaxin in acute myocardial infarction. evidences from a swine modelFASEB J2005191525716009702
- PriceJFMowbrayPILeeAJRelationship between smoking and cardiovascular risk factors in the development of peripheral arterial disease and coronary artery disease: Edinburgh Artery StudyEur Heart J1999203445310206381
- QuattroneSChiappiniLScapagniniGRelaxin potentiates the expression of inducible nitric oxide synthase by endothelial cells from human umbilical vein in in vitro cultureMol Hum Reprod2004103253015026539
- RadomskiMVPalmerRMJMoncadaSThe role of nitric oxide and cGMP in platelet adhesion to vascular endotheliumBiochem Biophys Res Commun1987148148292825688
- RosanoGMVitaleCFiniMhormone replacement therapy and cardioprotection: what is good and what is bad for the cardiovascular system?Ann N Y Acad Sci20061092341817308159
- SaderMACelermajerDSEndothelial function, vascular reactivity and gender differences in the cardiovascular systemCardiovasc Res5359760411861030
- SamuelCSUnemoriENMookerjeeIRelaxin modulates cardiac fibroblast proliferation differentiation and collagen production and reverses cardiac fibrosis in vivoEndocrinology200314541253315155573
- SamuelCSDuXJBathgateRADRelaxin’ the stiffened heart and arteries: the therapeutic potential for relaxin in the treatment of cardiovascular diseasePharm Ther200611252952
- SchwabeCBüllesbachEERelaxin: structure, functions, promises and nonevolutionFASEB J199481152607958621
- SherwoodODRelaxin’s physiological roles and other diverse actionsEndocr Rev2004252053415082520
- SiowRCMLiFYLRowlandsDJCardiovascular targets for estrogens and phytoestrogens: transcriptional regulation of nitric oxide synthase and antioxidant defense genesFree Rad Bio Med2007429092517349919
- SluiterWPietersmaALamersJMJLeukocyte adhesion molecules on the vascular endothelium: their role in the pathogenesis of cardiovascular disease and the mechanism underlying their expressionJ Cardiovasc Pharmacol199322Suppl 4S37447523771
- SmithGCPellJPWalshDPregnancy complications and maternal risk of ischaemic heart disease: A retrospective cohort study of 129,290 birthsLancet20013572002611438131
- StewartDRCelnikerACTaylorCAJrRelaxin in the peri-implantation periodJ Clin Endocrinol Metab199070177132347909
- St-LouisJMassicotteGChronic decrease of blood pressure by rat relaxin in spontaneously hypertensive ratsLife Sci198537135174046737
- SudoSKumagaiJNishiSH3 Relaxin is a specific ligand for LGR7 and activates the receptor by interacting with both the ectodomain and the exoloop 2J Biol Chem200327878556212506116
- TaylorMJClarkCLEvidence for a novel source of relaxin: atrial cardiocytesJ Endocrinol1994143R587829984
- TunstallPHKuulasmaaKPAmouyelPMyocardial infarction and coronary deaths in the World Health Organization MONICA Project. Registration procedures event rates and case-fatality rates in 38 populations from 21 countries in four continentsCirculation1994905836128026046
- UnemoriENAmentoEPRelaxin modulates synthesis and secretion of procollagenase and collagen by human dermal fibroblastsJ Biol Chem19902651068152162358
- UnemoriENBauerEAAmentoEPRelaxin alone and in conjunction with interferon-γ decreases collagen synthesis by cultured human scleroderma fibroblastsJ Invest Dermatol199299337421512471
- UnemoriENBeckLSLeeWPHuman relaxin decreases collagen accumulation in vivo in two rodent models of fibrosisJ Invest Dermatol199310128058370965
- UnemoriENPickfordLBSallesALRelaxin induces an extracellular matrix-degrading phenotype in human lung fibroblasts in vitro and inhibits lung fibrosis in a murine model in vivoJ Clin Invest1996982739458981919
- UnemoriENEriksonMERoccoSERelaxin stimulates expression on vascular endothelial growth factor in normal human endometrial cells in vitro and is associated with menometrorrhagia in womenHum Reprod199914800610221717
- VanhouttePMEndothelial dysfunction and atherosclerosisEur Heart J199718E19299402468
- VasilenkoPMeadJPWeidmannJEUterine growth-promoting effects of relaxin: A morphometric and histological analysisBiol Reprod198635987953814710
- WeiJYAge and the cardiovascular systemN Engl J Med1992327173591304738
- WengerNKSperoffLPackardBCardiovascular health and disease in womenN Engl J Med1993329247568316269
- WilliamsEJBenyonRCTrimNRelaxin inhibits effective collagen deposition by cultured hepatic stellate cells and decreases rat liver fibrosis in vivoGut2001495778311559657
- WilsonBCConnellBSalehTMRelaxin-induced reduction of infarct size in male rats receiving MCAO is dependent on nitric oxide synthesis and not estrogenic mechanismsNeurosci Lett2006393160416233954
- WinslowJWShihABourellJHHuman seminal relaxin is a product of the same gene as human luteal relaxinEndocrinology19921302660881572287
- WyckoffMHChamblissKLMineoCPlasma membrane estrogen receptors are coupled to endothelial nitric oxide synthase through Gαi J Biol Chem200127627071611369763
- ZdravkovicTGenbacevOMcMasterMTThe adverse effects of maternal smoking on the human placenta: a reviewPlacenta200526Suppl AS81615837073
- ZhangJQiYFGengBEffect of relaxin on myocardial ischemia injury induced by isoproterenolPeptides2005261632916112403
- ZicheMMorbidelliLMasiniENitric oxide mediates angiogenesis in vivo and endothelial cell growth and migration in vitro promoted by substance PJ Clin Invest1994942036447525653