*Optional to children.
‡Inhaled LABA optional.
IC: Inhaled corticosteroids; LABA: Long action β2-agonist; LTR: Leukotriene receptor; LTRA: Leukotriene receptor antagonists.
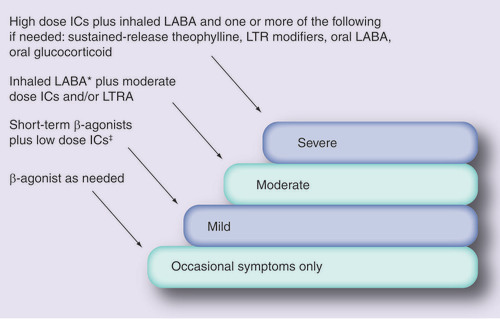
Asthma is a complex clinical syndrome with multiple genetic and environmental factors contributing to its phenotypic expression Citation[1]. Multiple epidemiologic and experimental studies indicate that asthma risk may be modified by epigenetic changes, such as DNA methylation, covalent histone modifications, microRNA changes and chromatin alterations, after early or later environmental exposures Citation[2]. This etiological heterogeneity adds to the complexity when addressing variation in the response to anti-asthma treatment. The relevant clinical variability in response to asthma medications may be due to genetic determinants and refers to the polymorphisms in the genes associated with asthma susceptibility, genes encoding either the drug targets or molecular components of the downstream signal transduction pathways responsible for drug action Citation[3].
Clinically the spectrum of asthma severity has three distinct levels: mild, moderate and severe. At each level, the clinical characteristics and the mode of treatment of an individual differs . It is critical to determine the level of severity to better understand how to treat and control the asthma. Therapies typically used for the treatment of asthma include: β2-adrenergic agonists, for bronchodilation; glucorticosteroids, for their potent anti-inflammatory action; theophylline and its derivatives, for bronchospasm and inflammation; and leukotriene inhibitors and antagonists as bronchodilators and anti-inflammatory drugs. Anticholinergics may also be used as bronchodilators, although they are less potent than β2-agonists. Short-acting β2-agonists (e.g., salbutamol, terbutaline, isoprenaline, fenoterol and so on) are the most effective medications used for the treatment of mild asthma, whereas long-acting β2-agonists (e.g., salmeterol, formoterol and so on), inhaled corticosteroids (ICs) and leukotriene inhibitors may be used independently or in combination for the treatment of mild-to-moderate asthma. Severe or ‘refractory‘ asthma can be treated either by oral corticosteroids, by very high dose of inhaled corticosteroids or in combination with theophylline. The degree of responsiveness to these medications varies from person to person. Variability in an individual‘s response to the prescribed asthma treatment may be due to several other factors, including treatment compliance, severity, intercurrent illness, environmental exposure, age and drug interactions with other medication being taken at the same time Citation[4]. Although heterogeneity in treatment responses are likely to arise in part by environmental factors, it is thought that up to 60–80% of this variability may be due to genetic factors Citation[5]. This indicates the need for finding genes responsible for clinically relevant variability in response to the main classes of asthma drugs. Many genetic variants often consist of just a single base pair substitution resulting in alterations of protein function or expression Citation[6]. These SNPs, as well as their variable linear combinations (haplotypes), may be major determinants of the variability in therapeutic response.
The pharmacodynamics and molecular basis of the action of β2-agonists have been extensively reviewed Citation[7]. A series of SNPs in the β2-AR gene have been identified Citation[8,9]. A total of four of these were found to cause changes in the encoded amino acids at residue 16, 27, 34 and 164, and few were found to have particular relevance to pharmacogenetics Citation[10–13]. In a recent placebo-controlled, double-blind study (β-Agonist Response by Genotype; BARGE) Citation[14] suggested that the 16th residue of the β2-adrenergic receptor (β2AR) has a pharmacogenetic relevance despite being neither in the drug-binding domain nor in the intracellular coupling domain of the trimeric Gs protein. Further Drysdale et al. indicated that the unique interaction of multiple SNPs within a haplotype may affect biologic and therapeutic phenotypes and may also have a good predictive power as a pharmacogenetic marker Citation[11]. ICs are the preferred first-line choice for the management of mild-to-moderate persistent asthma Citation[15]. They are considered to be the most effective and potent anti-inflammatory medication currently available for asthma treatment. Despite the proven efficacy of ICs in asthma, their precise mechanism of action is still incompletely characterized. They exert their pharmacological action through activation of the cytosolic glucocorticoid receptors. Recently, it has been hypothesized that sequence variants in the genes in the glucocorticosteroid pathway contribute to the variability in inhaled steroid treatment response Citation[16]. Polymorphisms in corticotropin realeasing hormone receptor 1 (CRHR1) were found to be associated with significantly improved lung function (a difference of 8–10% forced expiratory volume in 1 second [FEV1]) after 8 weeks of IC therapy. Interestingly, Tantisira et al. also recently reported a functional variant in another gene that may be able to predict responsiveness to ICs Citation[17]. They demonstrated that the nonsynonymous variation coding for replacement of histidine 33 with glutamine in the T-box 21 (TBX21) gene, which codes for the transcription factor T-bet (T-box expressed in T cells), is associated with significant improvement in the PC20 (a measure of airway responsiveness) of asthmatic children in a large clinical trial spanning 4 years. Studies of leukotrienes and their role in asthma have prompted great interest in agents that affect leukotriene activity Citation[18–20]. Although antileukotriene agents have an anti-inflammatory function, they are not as potent as corticosteroids and are generally restricted to mild asthma as a substitute for ICs, or in severe asthma as an add-on therapy. Two essential pharmacologic approaches have been taken in an attempt to reduce the effects of leukotrienes: interruption of leukotriene biosynthesis (leukotriene inhibitors) and blockage of the leukotriene receptor (leukotriene antagonists). In the leukotriene biosynthetic pathway, some key enzymes are encoded by polymorphic genes that can affect the therapeutic response of leukotriene therapy.
Theophylline has been widely used as a bronchodilator for the treatment of bronchial asthma and has been suggested to modulate immune response. The molecular mechanism for the anti-inflammatory action of theophylline is currently unknown, but low-dose theophylline is known to control asthma through increasing activation of histone deacetylase which is subsequently recruited by corticosteroids to suppress inflammatory genes Citation[21]. Other actions such as adenosine receptor antagonism and phosphodiesterase inhibition have also been suggested. Anticholinergic agents are used as bronchodilators in asthma and other airway diseases. In asthma, airways function and response to bronchospastic stimuli (e.g., allergens, irritants and inflammatory mediators) is controlled in part by cholinergic muscarinic receptors, which regulate smooth muscle contraction. Out of the five known muscarinic receptors, airway smooth muscle contains M2 and M3 receptors Citation[22] and the bronchoconstriction of the airway smooth muscles is mainly mediated by M3 receptors Citation[23]. The genetic variations in these receptors could potentially affect treatment responses to anticholinergic agents Citation[24–26], but the few studies conducted so far have failed to support a pharmacogenetic association. Possible reasons for lack of association might be an inadequate sample size and underpowered study design. However, the possibility of involvement of unknown variations of these genes for drug response still needs to be elucidated.
Owing to the complexity of drug action and its therapeutic response, a broader genomics approach will provide an edge in finding new drug targets from categories such as the protein receptor at which the drug directly binds, signal transduction cascades or downstream proteins, and proteins involved in disease pathogenesis, and optimizing therapy for the individual patient Citation[27,28]. The pharmacogenetic literature explicates that the consideration of only one protein or receptor as an efficient drug target will not be of much clinical relevance to predict the therapeutic response. Specific problems to date include the inability of a single polymorphism to be highly predictive of response, and inconsistencies across studies of the same polymorphism. It seems likely that an important factor in the above limitations is the approach of focusing on a single polymorphism in a single gene. Considering that a drug response may involve a large number of proteins, it seems unlikely that a single polymorphism in a single gene would explain a high degree of drug response variability in a consistent fashion. Thus, it shows that a polygenic or genomic approach will be more appropriate. The attempts in this direction will provide a new dimension in personalized medicine, drug efficacy and drug development in the future.
Pharmacogenetic studies of asthma have not yet been adequately replicated in different populations; hence they cannot be adopted as the standard-of-care as a diagnostic. Furthermore, due to the influence of environmental factors in asthma, it is worth to speculating that the direct association of any sequence variation with the variability of drug response cannot be obtained. So, the frequency and penetrance of sequence variants (such as SNPs, microsatellites and so on) affecting responsiveness to a particular drug and potential interactions with other genetic and environmental factors should be assessed in multiple population-based samples Citation[29,30]. Therefore, there is a pressing need for numerous large-scale prospective studies in order to further elucidate gene–environment interaction in asthma pharmacogenomics. Such studies have to be carried out in different ethnic groups, as genetic association findings of one population will not necessarily be valid for other populations. Apart from what we have already achieved in asthma pharmacogenetics, there is still a long way to go before the pharmacotyping concept and its knowledge are translated into clinical practice. In conclusion, despite of new developments and recent studies in asthma pharmacogenetics, significant gaps in knowledge still remain and we need several replicative studies in different populations to derive firm conclusions, which may help to bring pharmacotyping into clinical practice.
Acknowledgments
The authors thank Prof. SK Brahmachari, Dr Balram Ghosh and Dr Anurag Agarwal for their invaluable scientific suggestions. The authors also acknowledge Pallav Bhatnagar for helpful discussions.
Financial & competing interests disclosure
The authors have no relevant affiliations or financial involvement with any organization or entity with a financial interest in or financial conflict with the subject matter or materials discussed in the manuscript. This includes employment, consultancies, honoraria, stock ownership or options, expert testimony, grants or patents received or pending, or royalties.
No writing assistance was utilized in the production of this manuscript.
Bibliography
- Global Initiative for Asthma. Global strategy for asthma management and prevention, MD, USA: National Institute of Health; Publication No. 02–3659 (2002).
- Miller RL , HoSM: Environmental epigenetics and asthma: current concepts and call for studies.Am. J. Respir. Crit. Care Med.177 , 567–573 (2008).
- Guidelines for management of asthma at primary and secondary levels of health care in India: WHO – Government of India Collaborative Programme. 1–43 (2005).
- Hall IP : Pharmacogenetics of asthma.Eur. Respir. J.15 , 449–451 (2000).
- Weiss ST , LitonjuaAA, LangeC et al.: Overview of the pharmacogenetics of asthma treatment.Pharmacogenomics J.6(5) , 311–326 (2006).
- Pastinen T , SladekR, GurdS et al.: A survey of genetic and epigenetic variation affecting human gene expression.Physiol. Genomics16(2) , 184–193 (2004).
- Silverman R : Treatment of acute asthma: a new look at the old and at the new.Clin. Chest Med.21 , 361–379 (2000).
- Reihsaus E , InnisM, MacIntyreN, LiggettSB: Mutations in the gene encoding for the β2-adrenergic receptor in normal and asthmatic subjects.Am. J. Respir. Cell Mol. Biol.8(3) , 334–339 (1993).
- Liggett SB : Polymorphisms of the β2-adrenergic receptor and asthma.Am. J. Respir. Crit. Care Med.156 , S156–S162 (1997).
- Martinez FD , GravesPE, BaldiniM, SolomonS, EricksonR: Association between genetic polymorphisms of the β2-adrenoceptor and response to albuterol in children with and without a history of wheezing.J. Clin. Invest.100(12) , 3184–3188 (1997).
- Drysdale CM , McGrawDW, StackCB et al.: Complex promoter and coding region β2-adrenergic receptor haplotypes alter receptor expression and predict in vivo responsiveness.Proc. Natl Acad. Sci. USA97(19) , 10483–10488 (2000).
- Choudhry S , UngN, AvilaPC et al.: Pharmacogenetic differences in response to albuterol between Puerto Ricans and Mexicans with asthma.Am. J. Respir. Crit. Care Med.171(6) , 563–570 (2005).
- Kukreti R , BhatnagarP, B-RaoC et al.: β2-adrenergic receptor polymorphisms and response to salbutamol among Indian asthmatics.Pharmacogenomics6(4) , 399–410 (2005).
- Israel E , ChinchilliVM, FordJG et al: Use of regularly scheduled albuterol treatment in asthma: genotype-stratified, randomized, placebo-controlled cross-over trial. Lancet (364) , 1505–1512 (2004).
- British Thoracic Society. The British guidelines on asthma management: Review and position statement. Thorax52 (Suppl. 1) , 1–21 (1997).
- Weiss ST , LakeSL, SilvermanES et al.: Asthma steroid pharmacogenetics: a study strategy to identify replicated treatment responses.Proc. Am. Thorac. Soc.1(4) , 364–367 (2004).
- Tantisira KG , HwangES, RabyBA et al.: TBX21: a functional variant predicts improvement in asthma with the use of inhaled corticosteroids.Proc. Natl Acad. Sci. USA101(52) , 18099–18104 (2004).
- Barnes N : New drugs for asthma.Practitioner237 , 870–873 (1993).
- Barnes NC , KuitertLM: Drugs affecting the leukotriene pathway in asthma.Br. J. Clin. Pract.49 , 262–266 (1995).
- Barnes N : Leukotriene receptor antagonists: clinical effects.J. R. Soc. Med.90 , 200–204 (1997).
- Ito K , LimS, CaramoriG et al.: A molecular mechanism of action of theophylline: induction of histone deacetylase activity to decrease inflammatory gene expression.Proc. Natl Acad. Sci. USA99(13) , 8921–8926 (2002).
- Mak JC , BaraniukJN, BarnesPJ: Localization of muscarinic receptor subtype mRNAs in human lung.Am. J. Respir. Cell Mol. Biol.7(3) , 344–348 (1992).
- Roffel AF , ElzingaCRS, ZaagsmaJ: Muscarinic M3 receptor mediates contraction of human central and peripheral airway smooth muscle.Pulmon. Pharmacol.3 , 47–51 (1990).
- Fenech AG , EbejerMJ, FeliceAE, Ellul-MicallefR, HallIP: Mutation screening of the muscarinic M(2) and M(3) receptor genes in normal and asthmatic subjects.Br. J. Pharmacol.133(1) , 43–48 (2001).
- Yamamoto T , YamashitaN, KuwabaraM et al.: Mutation screening of the muscarinic M2 and M3 receptor genes in asthmatics, outgrow subjects, and normal controls.Ann. Genet.45(3) , 109–113 (2002).
- Fenech AG , BillingtonCK, SwanC et al.: Novel polymorphisms influencing transcription of the human CHRM2 gene in airway smooth muscle.Am. J. Respir. Cell Mol. Biol.30(5) , 678–686 (2004).
- Lima JJ , BlakeKV, TantisiraKG, WeissST: Pharmacogenetics of asthma.Curr. Opin. Pulm. Med.15(1) , 57–62 (2009)
- Bhatnagar P , GuleriaR, KukretiR: Variable therapeutic response in asthma: a genetic perspective.Personalized Medicine3(1) , 61–78 (2006).
- Brahmachari SK , MajumderPP, MukerjiM et al.; Indian Genome Variation Consortium: Genetic landscape of the people of India: a canvas for disease gene exploration. J. Genet.87 , 3–20 (2008).
- Séguin B , HardyBJ, SingerPA, DaarAS: Genomic medicine and developing countries: creating a room of their own.Nature Rev. Genet.9 , 487–494 (2008).