Abstract
Aim
To investigate whether apoM is excreted in urine of children undergoing heart surgery and the potential of apoM as early biomarker of acute kidney injury (AKI).
Materials & methods
Urine was collected in children undergoing heart surgery. ApoM was measured with ELISA. U-apoM was characterized by gel filtration chromatography and western blotting.
Results
ApoM was excreted into the urine 0–4 h postoperatively as the full-length apoM in particles smaller than plasma HDL. At 0 h, U-apoM predicted AKI with an area under the receiver-operating characteristics curve of 0.70 (p < 0.018). Sensitivity was 0.71 and specificity was 0.68 at a cutoff level at 1.45 nmol/l.
Conclusion
ApoM is excreted in the urine of children after cardiac surgery. Its potential as biomarker of AKI deserves exploration.
Apolipoprotein M (apoM) is a lipocalin discovered in 1999 [Citation1,Citation2]. ApoM exerts antiatherogenic and anti-inflammatory effects [Citation3–6] and has recently been identified as plasma carrier of sphingosine-1-phosphate (S1P) [Citation7]. The apoM gene is expressed in the liver and in the kidney proximal tubular epithelium [Citation1,Citation8]. The ApoM protein has an unusual feature of a retained hydrophobic signal peptide which attaches it to HDL [Citation9,Citation10]. The signal peptide sequence likely makes free apoM insoluble in water. Hence, there is no ‘free’ apoM in plasma [Citation1,Citation10]. The large size of HDL (~150–400 kDa [Citation11]) prevents glomerular filtration and loss of plasma apoM in urine.
Urine samples containing 1% plasma were stored at room temperature (RT) or 4°C for 0–5 days. Subsequently, SDS-page gel electrophoresis was performed applying 16 µl of sample per well and 0.5 µl of plasma as positive control. Western blotting was done using a polyclonal antibody against apoM. (B) Recovery of apoM in urine was measured in duplicates in seven different dilutions of plasma corresponding to the dilutions of the standard curve. The dilution buffer contained 50, 25 or 10% urine. (C) Standard curve of the apoM ELISA. A sandwich ELISA using two monoclonal antibodies (M58 and M42) and a horse radish peroxidase detection method measuring within the range of 8.7–66.6 fmol apoM/well.
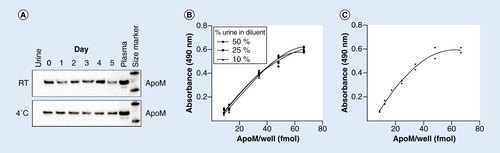
A baseline urine sample was taken before the operation, and postoperative sampling was done every 2 h for the first 12 h and then at 24 h. Patients were assigned to two groups–acute kidney injury (AKI) or no kidney affection (non-AKI) according to the KDIGO classification of AKI. An outlier (patient no. 35) has been omitted. Patients with hemoglobinuria were excluded in C + D. Points represent mean ± SEM. (A) U-apoM (nmol/l), *p = 0.0175. (B) U-apoM/U-creatinine ratio (nmol/mmol), *p = 0.0084, **p = 0.0345. (C) U-apoM (nmol/l). (D) U-apoM/U-creatinine ratio (nmol/mmol), *p = 0.0316, **p = 0.0115.
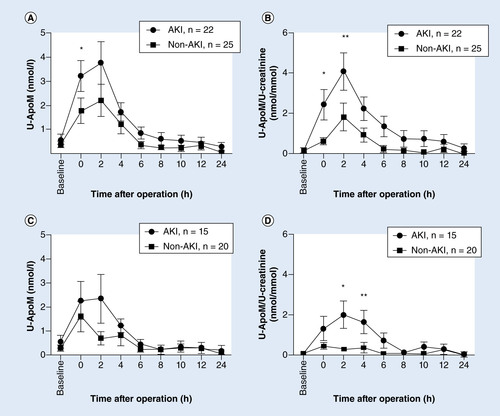
A baseline urine sample was taken before the operation, and postoperative sampling was done every 2 h for the first 12 h and then at 24 h. Patients were assigned to two groups–acute kidney injury (AKI) or no kidney affection (non-AKI) according to the KDIGO classification of AKI. An outlier (patient no. 35) has been omitted. Patients with hemoglobinuria were excluded in C + D. Points represent mean ± SEM. (A) U-NGAL (nmol/l), *p < 0.0001, **p = 0.0059. (B) U-NGAL/U-creatinine ratio (nmol/mmol), *p = 0.0005, **p = 0.0052. (C) U-NGAL (nmol/l), *p = 0.0009, **p = 0.0048. (D) U-NGAL/U-creatinine ratio (nmol/mmol), *p = 0.0027. **p = 0.0059. (E) Correlation between 2 h U-apoM and U-NGAL measurements. Spearman r = 0.8052 (p < 0.0001). Pt no. 35 has been omitted from the regression analysis (included in parenthesis).
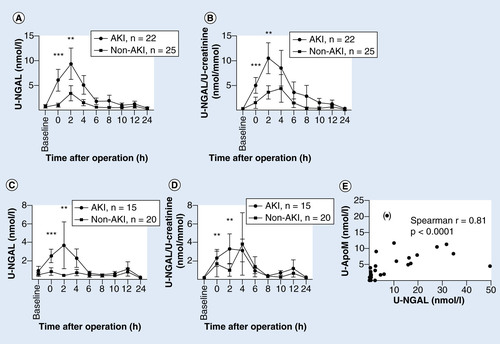
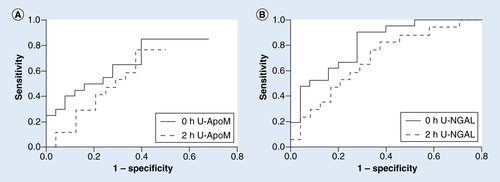
Bars represent medians. (C) Urinary apoA1 (nmol/l) at 2 h postoperatively in children without (n = 25) and with AKI (n = 17) after cardiac surgery and in adult patients with nondiabetic glomerulonephritis and proteinuria (n = 8). Bars represent medians.
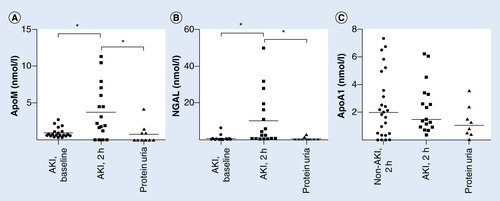
(A) A pool of urine from five patients (400 µl) concentrated sevenfold and sterile filtered was loaded on to a Superose 6 10/300 GL fast protein liquid column. Fractions (~280 µl) were collected, and protein content and elution profile was determined by absorbance at 280 nm. Elution volumes for plasma lipoproteins are shown. From each of five adjacent fractions, 50 µl were pooled to a total of 250 µl and concentrated to 15 µl before electrophoresis in 12% polyacrylamide gels under denaturing and reducing conditions. Subsequently, western blotting was done against apoM (rabbit polyclonal antihuman apoM, EPR2904) followed by stripping and reincubation with anti-apoA1 antibodies. (B) Urine from one patient was run on Superose 6 and 12 in serial connection following the same procedure as in (A). 100 µl from each of seven adjacent fractions were pooled and concentrated 23–28-fold before western blotting against apoM (rabbit polyclonal antihuman apoM). Single fractions within the pools where apoM eluted were concentrated fivefold before repeating the apoM western blot with a monoclonal antihuman apoM antibody (M58). A pool of protein calibrators and plasma was run on the same column set-up to establish the elution volumes of known proteins and lipoproteins.
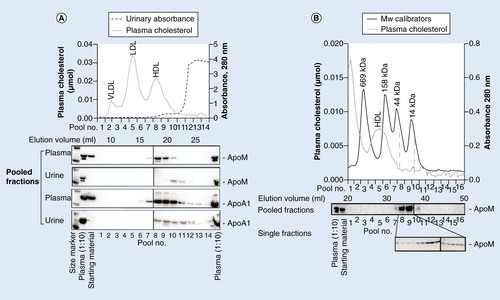
Table 1. Characteristics and clinical outcomes of children developing AKI or no AKI.
Table 2. Characteristics of adult proteinuric patients.
Table 3. ApoM and NGAL test characteristics.
Little is known about the biological function of apoM in the kidney. Megalin is a receptor for apoM and likely contributes to the reuptake of apoM from preurine at the luminal side of the kidney proximal tubule cells [Citation12]. Hence, there is no apoM in the urine under normal circumstances [Citation12]. In contrast, apoM has been shown in robust amounts in the urine of megalin knockout mice [Citation12]. These previous results suggest that apoM both undergoes secretion and megalin-mediated reuptake in the kidney proximal tubule epithelium.
Damage to the kidney proximal tubular epithelium takes part in the pathophysiology of acute kidney injury (AKI). AKI is associated with increased risk of death in hospitalized patients [Citation13] and is a known complication to cardiac surgery [Citation14]. In adults, reported incidences of postoperative AKI are highest in patients with preoperative risk factors such as nephrotoxic medication, insulin-dependent diabetes, peripheral, vascular disease and lung disease [Citation15]. A strong intraoperative risk factor of AKI is cardiopulmonary bypass (CPB) [Citation15]. The risk of AKI increases with longer extra corporeal circulation time [Citation15]. The use of CPB introduces an inflammatory state, which can cause kidney injury. Other factors related to CPB are hemodilution, nonpulsatile flow and possible embolism during aortic cannulation and clamping that can cause ischemic renal damage [Citation15].
CPB is often indispensable in surgical correction of congenital heart disease in children. Accordingly, children undergoing cardiac surgery are in great risk of AKI and reports have shown 28–64% incidence of postoperative AKI [Citation16–20]. The diagnosis of AKI following the RIFLE (Risk, Injury, Failure, Loss, End stage) [Citation21], AKIN (Acute Kidney Injury Network) [Citation22] or pRIFLE (modified pediatric RIFLE) criteria [Citation23] is not possible until 1–3 days after the kidney insult with the currently used markers of glomerular filtration (P-creatinine, estimated glomerular filtration (eGFR) or urinary output). Biomarkers for AKI, which reflect kidney injury rather than glomerular filtration, may reduce the time before diagnosis to hours [Citation24]. This could allow earlier intervention and possibly limit the kidney damage and decrease the mortality of postcardiac surgery AKI. Already, many biomarkers have emerged. With varying sensitivity and specificity in different clinical settings they reflect different parts of the AKI pathology depending on patient population and kidney insult. A panel of biomarkers for AKI to cover the span of the AKI pathology is therefore a probable future scenario, and is why investigation of further biomarkers is relevant.
Disruption of the secretion and reuptake cycle of apoM in the kidney by damage to the proximal tubular cells, particularly in AKI, may result in loss of apoM in the urine. We chose to investigate the AKI biomarker potential of U-apoM to test this idea. We speculated that U-apoM possibly could be more specific than NGAL, since U-apoM is not detectable in urine of healthy subjects and would only originate from the kidney, due to the tight apoM-HDL association in plasma.
The aims of the present study were to examine the stability of apoM in urine and whether apoM is excreted in the urine of children undergoing heart surgery. Second, to test the ability of U-apoM in predicting AKI and compare it to NGAL. Third, to do molecular characterization of U-apoM and to investigate the pathophysiology of apoM secretion by measuring U-apoM in another patient cohort with glomerular disease without AKI.
Patients & methods
Patients
Fifty children (median age: 0.5 years [0.01–14.9 years]) undergoing operation for congenital heart malformation were prospectively recruited from November 2009 to January 2011 at Rigshospitalet, Copenhagen, Denmark. With no prior reports of human U-apoM secretion, lack of expected U-apoM levels precluded power calculations. Instead, a sample size of N = 50 was chosen to obtain N ≥ 10 of patients with AKI, by assuming minimum 20% incidence of AKI in our patient cohort. Exclusion criteria were diabetes mellitus, chronic kidney disease, incorrect sample handling or pre- or per-operative use of medication with a reported incidence of nephrotoxic side effects of >1%. Two patients were excluded from analyses due to wrong sample handling and preoperative treatment with gentamicin, respectively. One patient without AKI (no. 35) was excluded from statistical analyses due to an outlier apoM-concentration. Patient flow is described in . The study is reported according to the STROBE recommendations.
Patients were monitored with P-creatinine and diuresis as part of the standard procedure after surgery. AKI was defined according to the newly recommended KDIGO staging of AKI, which incorporates the RIFLE, pRIFLE and AKIN criteria [Citation25]. Twenty-two of 48 patients developed AKI. AKI diagnoses were based on a >1.5-fold increase or >26.5 µM increase in P-creatinine or eGFR <35 ml/min per 1.73 m2. None of the patients met the criteria for oliguria/anuria in AKI.
Before enrollment, informed written consent was obtained from parents or legal guardians of the children. The study was approved by the Danish Ethics Committee for Region Hovedstaden (H-D-2008-049) and registered with The Danish Data Protection Agency (2008-41-2222).
Eight adult patients with nondiabetic nephropathy enrolled in a multicenter trial by the Holland Nephrology Study (HONEST) Group were included to study apoM in the urine of patients with severe proteinuria without AKI. At time of inclusion, all patients had blood pressure >125/75 mmHg and proteinuria >1.0 g/day despite treatment with maximal dose of ACE inhibitors. The study is registered with the Netherlands Trial Register (NTR675) [Citation26].
Patient data
For the children in this study, P-creatinine was obtained from the patient electronic biochemical charts. Age, weight, height, sex, extra corporeal circulation time, operation time and aortic clamp time were noted from the patients’ anesthetic and cardio-pulmonary perfusion charts. Diagnosis, intervention procedure and number of prior operations were obtained from the surgical procedure registry database ‘PATS’. Length of stay in the intensive care unit was extracted from IntelliVue Clinical Information Portfolio (ICIP, Phillips). Diuresis was noted from the anesthetic charts and drawn from ICIP. Medication prior to and during the study period was obtained from the electronic patient medication charts ‘EPM’.
Urine sampling
A baseline urine sample was taken from the children preoperatively at the time of bladder catheter insertion. Collection of postoperative urine was initiated at time 0, in other words, the time of closure of the sternum, and was performed every 2 h for the first 12 h and at 24 h. In two cases, the sternum was not sutured immediately after the operation due to bleeding. The sampling of urine was instead initiated at the time of transfer to the intensive care unit. All postoperative urine sampling was done from the collecting bag, which was emptied at all sampling time points. Urine was stored at 4°C for up to 48 h before centrifugation at 2000 × g for 10 min. Supernatants were isolated and subsequently stored at -80°C.
Baseline 24-h urine samples were collected by the adult, proteinuric patients before the interventions of the multicenter trial started. Samples were stored at -80°C [Citation26].
Plasma & urinary biochemistry
Plasma (P-) and urinary (U-) creatinine in children was measured with an enzymatic method on the Modular P Hitachi Automatic Analyzer (Roche, Hvidovre, Denmark). Urinary hemoglobin was measured on Unicam UV2 UV/Vis Spectrophotometer (ATI Unicam, Cambridge, UK). U-protein and U-creatinine concentrations in proteinuric patients were measured with a turbidimetric assay using benzethonium chloride and an automated multianalyser, respectively (Modular, Roche Diagnostics, Mannheim, Germany) [Citation26].
ApoM in urine was measured with enzyme-linked immunosorbent assay (ELISA) using the two monoclonal antibodies M58 and M42 described earlier [Citation4,Citation27]. Interference of urine with the apoM measurements was estimated by measuring the recovery of human apoM in plasma diluted with a buffer containing 0, 10, 25 or 50% urine from a healthy individual. The stability of apoM in urine was examined by adding plasma to urine (1 µl plasma per 100 µl urine). The mixture was left at room temperature or at 4°C for 0–5 days before examination of apoM by western blotting. ApoM in all urine samples was initially measured in a 1:10 dilution and repeated at higher dilutions if necessary.
Urinary NGAL was measured with a sandwich ELISA using two monoclonal antibodies according to the manufacturer’s instructions (KIT 036, BioPorto, Gentofte, Denmark). Results were converted into molar concentrations using a molecular weight of 22,664 g/mol calculated from the amino acid sequence of NGAL at [Citation28]. Urinary apoA1 measurements were done in 1:10 dilutions with a sandwich ELISA using a polyclonal capture antibody against ApoA1 (Q0496; DAKO, Glostrup, Denmark) and a monoclonal detection antibody (M25, developed in B. Dahlbäck’s laboratory) [Citation29]. Potential interference of urine was estimated as for the apoM ELISA with the recovery of plasma in a dilution buffer with 10% urine.
Gel filtration
Prior to gel filtration, urine (5 ml) was reduced in a vacuum centrifuge at 30°C (Eppendorf Concentrator 5301, Hoersholm, Denmark), passed through a sterile filter (0.45 µm Millex filters, Millipore, MA, USA). The volume was adjusted with H2O to achieve a sevenfold concentration. Concentrated urine (400 µl) was loaded on to a Superose 6 or serially connected Superose 6 and 12 10/300 GL fast protein liquid columns (GE Healthcare Bio-Sciences, Hiller⊘d, Denmark). Elution was done with phosphate buffered saline with EDTA (0.1 g/l) at a flow rate of 0.2 ml/min. Aliquots of 70 µl from single fractions and pools containing 50 or 100 µl from each of respectively 5 or 7 adjacent fractions were concentrated in a vacuum centrifuge. With H2O volume adjustments to get loadable sample viscosity, the single fractions were fivefold and the pools 17–28-fold concentrated before polyacrylamide electrophoresis and western blot against apoM.
Western blotting
Aliquots of sterile filtered urine and concentrated single or pooled gel filtration fractions were denatured and reduced prior to electrophoresis in 12% polyacrylamide gels (NuPAGE BisTris Mini Gel, Invitrogen). Upon transfer to a polyvinylidene fluoride membrane, apoM western blotting was performed with one of two rabbit polyclonal antibodies against human apoM (either EPR2904, from Genetex, CA, USA or an in-house antibody from B. Dahlbäck’s laboratory) [Citation4] or a mouse monoclonal antihuman apoM antibody (M58 from B. Dahlbäck’s laboratory) [Citation4]. For the ApoA1 western blots, the polyvinylidene fluoride membranes were stripped with 0.4 M NaOH for 15 min followed by five washes (in 10 mM Tris, 0.1M NaCl, 0.25% Tween 20) for a total of 30 min. Subsequent western blotting was done using a rabbit polyclonal antihuman apoA1 antibody (Q0496, DAKO, Glostrup, Denmark). The secondary antibodies were goat antirabbit or goat antimouse polyclonal antibodies linked to horse radish peroxidase (p0448, DAKO, Glostrup, Denmark and M15345, BD Biosciences, Albertslund, Denmark).
Statistics
Data were analyzed in GraphPad Prism (CA, USA) using Spearman correlation, nonparametric Mann–Whitney, unpaired t, and Fischer’s exact tests as indicated. p < 0.05 was considered statistically significant.
We used the statistical software package Stata (version 12.0; STATA Corp., College Station, TX, USA) to evaluate the biomarker potential of apoM. The predictive probability for AKI was calculated as the area under the receiver-operating characteristics (AU-ROC) curve. We tested whether the AU-ROC curves for NGAL and apoM were equally good and evaluated whether the predicted probability from a logistic model including both urinary NGAL and urinary apoM differed significantly from a logistic model including urinary NGAL alone.
Results
Patients
Twenty-two of 48 children developed postoperative AKI. The children with AKI compared with the non-AKI group were not different with regards to age, gender, number of previous heart surgery and body surface area. The children with AKI had longer surgery time, longer extra corporeal circulation time, longer aortic clamp time, and spent more postoperative time in the intensive care unit. Tetralogy of Fallot was associated with a significantly increased risk of AKI (Fisher’s exact test, p = 0.0059) ().
The basic characteristics of adult patients with proteinuria are shown in .
Urinary apoM ELISA
There was no degradation of apoM in urine for up to 5 days as judged by western blotting (). The putative interference of urine on apoM ELISA measurements was studied by adding exogenous apoM to urine. The recovery of apoM in urine diluted 1:2, 1:4 and 1:10 with ELISA dilution buffer was 86% (95% CI: 72–99%), 84% (95% CI: 75–92%) and 79% (95% CI: 70–87%), respectively (). The detection limit of the urine apoM ELISA assay was 0.87 nmol/l as defined by the concentration of the lowest standard in the calibration curve. The coefficient of variation was 11%. These results indicated that apoM could be measured in urine with the apoM ELISA employed.
Urinary ApoM excretion is temporarily induced after cardiac surgery in children
Urinary apoM was measured prior to and every 2 h after surgery. In accordance with the absence of apoM in the urine of normal mice, rats and humans in western blotting analyses [Citation12], the baseline concentration was less than the detection limit of the apoM ELISA (). Notably, there was a pronounced temporary increase in the urinary apoM concentration from 0 to 4 h postoperatively in 40 of 48 children to a mean peak concentration of approximately 3 nmol/l (). The urinary apoM excretion had returned to baseline values 24 h after the operation in 46 of the children ().
AKI was associated with an increased concentration of apoM in the urine at t = 0 h postoperatively (). When the apoM concentration was normalized with U-creatinine, the difference between AKI and non-AKI children became even more apparent (). Notably, there was no statistically significant difference in the U-creatinine concentrations between the AKI and non-AKI children (data not shown).
Since the peak concentration of apoM in urine was only approximately 1/300 of the mean plasma apoM concentration (i.e., ~0.9 µmol/l in adults [Citation27]), we wondered whether the urinary apoM could be derived from blood contamination during the bladder catheterization procedure. Therefore, we re-examined the U-apoM after excluding 12 children with biochemically detectable hemoglobinuria associated with the peak U-apoM concentration (). Even though the U-apoM concentration was slightly lower after excluding patients with hemoglobinuria, there was still a higher U-apoM in the AKI than in the non-AKI group when the U-apoM was normalized with U-creatinine ().
U-apoM correlates with U-NGAL
As for apoM, the urinary excretion of NGAL was essentially absent at baseline, increased temporarily at 0–4 h after surgery, and was increased in the AKI compared with the non-AKI group irrespectively of excluding children with hemoglobinuria (). The similarity of the excretion pattern of the two urinary proteins was in accordance with a close positive correlation between U-apoM and U-NGAL (Spearman’s r = 0.8052, p < 0.0001, ).
The ability of urinary NGAL to predict AKI at 0 h was significant with an area under the ROC curve of 0.85 (SE 0.056; p < 0.0001; ). Sensitivity was 0.90 and specificity was 0.72 for a cutoff level at 0.48 nmol/l (). ApoM was also a significant predictor of AKI at 0 h. The area under the ROC curve was 0.70 (SE 0.079; p < 0.01779; ). Sensitivity was 0.71 and specificity 0.68 when cutoff was 1.45 nmol/l (). U-NGAL was better at predicting AKI than U-apoM (p < 0.0187). To test if U-apoM would add to the predictive value of U-NGAL, we compared the area under the ROC curve at 0 h between NGAL and NGAL adjusted for apoM. However, apoM did not increase the predictive ability of NGAL (data not shown).
Proteinuria is not accompanied by urinary excretion of apoM
To assess whether apoM excretion in the urine also occurs in patients with an impaired glomerular filtration barrier, we measured U-apoM in adult patients with severe proteinuria in the setting of nondiabetic nephropathy and found levels that were on average similar to the baseline values in children prior to heart surgery (). U-NGAL in the proteinuric patients was also low and at the level of the children’s baseline values ().
Urinary apoM in AKI is not associated with HDL
We characterized apoM in the urine to determine whether the U-apoM excretion in children originates from the plasma or is derived from the kidney. In plasma, apoM associates with large apoA1-containing HDL particles. The ratio between the measured concentrations of apoA1 in urine and the normal ApoA1 plasma concentration was 1:10,000–1:100,000, that is, much lower than that for apoM (~1:300). There was no significant difference in U-apoA1 levels between AKI versus non-AKI or proteinuria (), and, moreover, U-apoA1 did not correlate with U-NGAL or U-apoM and (data not shown).
To further characterize U-apoM, we performed gel filtration chromatography of urine from children with AKI (). The apparent size of plasma HDL is approximately 160–410 kDa [Citation11]. As opposed to plasma apoM, U-apoM co-eluted with smaller particles (14–44 kDa) than HDL (). Upon delipidation and SDS-PAGE, the size of urinary apoM protein was approximately 25 kDa, which is similar to that of the plasma apoM protein, indicating that apoM is predominantly excreted from the kidney as the glycosylated full length protein [Citation1]. ApoA1 western blots revealed U-apoA1 in larger particles than U-apoM. This implies that the sparse amounts of apoA1 in urine are mainly associated with other particles than apoM ().
Discussion
This study demonstrates that apoM is excreted into the urine after cardiac surgery in children at 0–4 h postoperatively. The excretion peaks at 2 h. Forty-six percent of the children developed AKI. They had significantly higher U-apoM at the time of closure of sternum (t = 0) compared with the non-AKI patients. Increased U-apoM/creatinine ratio predicted AKI at 0 and 2 h postoperatively. The higher U-apoM/creatinine ratio in the AKI group was not a result of decreased glomerular filtration and thereby lower U-creatinine. U-creatinine decreased from baseline until 2 h postoperatively in both groups, and there was no significant difference between the AKI and the non-AKI group.
NGAL is a promising biomarker of AKI. It has been suggested that a multimarker approach with several biomarkers including NGAL may eventually allow rapid biochemical diagnosis of AKI [Citation30]. NGAL is synthesized by myeloid cells in the bone marrow [Citation31] and stored in the specific granules of neutrophils [Citation32], but is also expressed in other tissues including kidney proximal tubule epithelium [Citation33,Citation34]. NGAL has bacteriostatic effects by binding of bacterial ferric siderophores and depleting bacteria of iron [Citation35]. Furthermore, protective effects of NGAL in the kidney have been demonstrated with intravenous administration of NGAL in ischemia-reperfusion mouse models [Citation36,Citation37]. NGAL expression in the kidney is upregulated after ischemia [Citation38] and U-NGAL has been proposed as an early biomarker of AKI [Citation39,Citation40]. Interpretation of U-NGAL can, however, be complicated [Citation41]. NGAL is mainly secreted as a 25 kDa monomer from the kidney proximal tubule cells. However, other molecular forms of NGAL originating from blood neutrophils and without relation to AKI can also be found in the urine [Citation41,Citation42]. Therefore, a higher specificity of U-apoM than U-NGAL for AKI could be speculated in clinical settings where P- and U-NGAL levels are elevated due to other reasons than AKI.
We compared U-apoM with U-NGAL to further examine the potential of apoM to diagnose AKI in children undergoing heart surgery. U-NGAL and U-apoM measurements were highly correlated, suggesting that the pathophysiology causing apoM and NGAL secretion is interconnected in the present group of patients. Accordingly, we could not see a beneficial effect of measuring both U-NGAL and U-apoM in the prediction of AKI.
The fact, that U-NGAL was better than apoM in predicting AKI, is possibly dominated by two reasons. First, U-NGAL baseline levels were low meaning the measured increase in NGAL excretion was a specific effect of cardiac surgery. Second, the U-apoM levels probably had two sources: ApoM from the kidney, reflecting tubular damage, and apoM from plasma due to hematuria. The U-apoM concentration being approximately 1/300 of that in plasma can be highly affected by plasma ‘contamination’. Some children had macroscopic hematuria. When omitting the children (25% of the study population) who had biochemically detectable U-hemoglobin, the U-apoM/creatinine remained significantly elevated in the AKI group at 2 and 2 h. A reduction of statistical power can be the attributable factor to the lack of difference at 0 h. The relatively low AUC value and lack of dose-effect relationship of U-apoM in the whole cohort is possibly a reflection of the confounding hematuria. The ELISA employed cannot distinguish between apoM derived from the kidney and plasma. Therefore, U-apoM as a predictive biomarker of AKI is most likely not a suitable biomarker in the present clinical setting.
The biochemical characterization supports that U-apoM is derived from the kidney rather than plasma HDL. In plasma, apoM is tightly associated with large lipoproteins (>160 kDa). In urine, apoM co-eluted with small (14–44 kDa) proteins, indicating no lipoprotein association. U-apoM was absent in patients with severe proteinuria, suggesting that the HDL-associated plasma apoM is not filtered into the urine to a significant extent, even when the glomerular barrier is defect. Moreover, the relative concentration of apoA1 in urine compared with plasma was much lower than that of apoM. This further implies that the increase in U-apoM in children after heart surgery is not due to loss of plasma HDL to the urine, since there is no concurrent increase in U-apoA1, which is a surrogate marker of HDL. The idea that U-apoM is derived from the kidney is consistent with our previous observation in mice, that is, that U-apoM secretion in mice with a defective megalin receptor mediated uptake of apoM in the proximal tubules results in loss of kidney-derived apoM [Citation12].
Elevated levels of U-NGAL have been reported in proteinuric patients with positive correlation to the severity of proteinuria [Citation43]. The low U-NGAL level of the proteinuric patients in the present study compared with a report by Bolignano et al. [Citation44] might partly be due to approximately four-times lower levels of proteinuria. However, the presence of different types of glomerular disease and angiotensin receptor blockade at maximum dose in our proteinuric patients are possibly contributing causes to the lower levels of U-NGAL.
The reason why apoM under normal conditions likely undergoes secretion and reabsorption in the kidney is yet to be found. Plasma apoM is a carrier of S1P which is a bioactive lipid. Notably, S1P has vasoprotective effects and prevents AKI in an ischemia-reperfusion mouse model [Citation45,Citation46]. Four S1P receptors (S1P1–4) are expressed in the kidney of mice with S1P1 and S1P3 being upregulated after ischemia-reperfusion injury [Citation45]. Hence, apoM could work as a kidney-protective co-factor for delivery of S1P to the tubular epithelium.
Limitations of the present study include that it is a small population, single-center analysis. Furthermore we only investigated patients in one specific clinical setting, which does not reflect the multifactorial etiology of AKI. As already discussed, possible blood contamination of urine makes U-apoM measurements hard to interprete, since our current ELISA cannot distinguish between apoM derived from plasma and apoM from the proximal tubules reflecting AKI. Finally, a great disadvantage of urinary diagnostics is the scarcity of urine in oliguric patients which can make it difficult to obtain the required amount of urine necessary for analysis.
Conclusion
ApoM, which is normally essentially absent in urine, is secreted into the urine of children after cardiac surgery. The U-apoM concentration was highest in the children who developed AKI, implying that the degree of kidney affection during surgery determines apoM secretion. U-apoM and U-NGAL are highly correlated. U-NGAL is better in predicting AKI than U-apoM and U-apoM does not substantially add to the diagnostic power of U-NGAL. ApoM is not secreted in the urine of severely proteinuric patients, which supports the possibility that U-apoM could be a specific marker of damage to the proximal tubules. However, in the present clinical setting, apoM is likely not a suitable biomarker of AKI, as reflected in the relatively low AUC.
Future perspective
The present study has added U-apoM to the list of early biomarkers of AKI. Measurements of U-apoM in future studies will cast light upon the diagnostic value of apoM in different AKI patient cohorts. With the recent discovery that apoM carries S1P, which mediates endothelial barrier protective functions, it would be of interest to determine whether human apoM has renoprotective effects during AKI.
Urinary ApoM excretion is temporarily induced after cardiac surgery in children
ApoM was essentially undetectable in preoperative urine samples, but was excreted into the urine 0–4 h postoperatively
At 0 h, in other words, at time of closure of the sternum, U-apoM predicted AKI with an area under the ROC curve of 0.70 (p < 0.018). Sensitivity was 0.71 and specificity was 0.68 at a cutoff level at 1.45 nmol/l.
U-apoM correlates with U-NGAL
U-apoM correlated with (r = 0.8052; p < 0.0001) but did not increase the predictive ability of U-NGAL.
Proteinuria is not accompanied by urinary excretion of apoM
U-apoM was absent in adult patients with proteinuria.
Urinary apoM in AKI is not associated with HDL
Urinary apoM was secreted as the full-length apoM in particles smaller than plasma HDL.
ApoA1 in urine is mainly associated with other particles than apoM
Conclusion
Kidney-derived U-apoM is secreted into the urine of children after cardiac-induced AKI.
The potential use of U-apoM as biomarker of AKI deserves exploration.
Financial & competing interests disclosure
The authors have no relevant affiliations or financial involvement with any organization or entity with a financial interest in or financial conflict with the subject matter or materials discussed in the manuscript. This includes employment, consultancies, honoraria, stock ownership or options, expert testimony, grants or patents received or pending, or royalties.
No writing assistance was utilized in the production of this manuscript.
References
- Xu N , DahlbackB. A novel human apolipoprotein (apoM). J. Biol. Chem.274(44), 31286–31290 (1999).
- Duan J , DahlbackB, VilloutreixBO. Proposed lipocalin fold for apolipoprotein M based on bioinformatics and site-directed mutagenesis. FEBS Lett.499(1–2), 127–132 (2001).
- Wolfrum C , PoyMN, StoffelM. Apolipoprotein M is required for prebeta-HDL formation and cholesterol efflux to HDL and protects against atherosclerosis. Nat. Med.11(4), 418–422 (2005).
- Christoffersen C , NielsenLB, AxlerOet al. Isolation and characterization of human apolipoprotein M-containing lipoproteins. J. Lipid Res.47(8), 1833–1843 (2006).
- Christoffersen C , JauhiainenM, MoserMet al. Effect of apolipoprotein M on high density lipoprotein metabolism and atherosclerosis in low density lipoprotein receptor knock-out mice. J. Biol. Chem.283(4), 1839–1847 (2008).
- Feingold KR , ShigenagaJK, ChuiLGet al. Infection and inflammation decrease apolipoprotein M expression. Atherosclerosis199(1), 19–26 (2008).
- Christoffersen C , ObinataH, KumaraswamySBet al. Endothelium-protective sphingosine-1-phosphate provided by HDL-associated apolipoprotein M. Proc. Natl Acad. Sci. USA108(23), 9613–9618 (2011).
- Zhang XY , DongX, ZhengLet al. Specific tissue expression and cellular localization of human apolipoprotein M as determined by in situ hybridization. Acta Histochem.105(1), 67–72 (2003).
- Axler O , AhnstromJ, DahlbackB. Apolipoprotein M associates to lipoproteins through its retained signal peptide. FEBS Lett.582(5), 826–828 (2008).
- Christoffersen C , AhnstromJ, AxlerOet al. The signal peptide anchors apolipoprotein M in plasma lipoproteins and prevents rapid clearance of apolipoprotein M from plasma. J. Biol. Chem.283(27), 18765–18772 (2008).
- Kontush A , ChapmanMJ. Functionally defective high-density lipoprotein: a new therapeutic target at the crossroads of dyslipidemia, inflammation, and atherosclerosis. Pharmacol. Rev.58(3), 342–374 (2006).
- Faber K , HvidbergV, MoestrupSK, DahlbackB, NielsenLB. Megalin is a receptor for apolipoprotein M, and kidney-specific megalin-deficiency confers urinary excretion of apolipoprotein M. Mol. Endocrinol.20(1), 212–218 (2006).
- Uchino S , BellomoR, BagshawSM, GoldsmithD. Transient azotaemia is associated with a high risk of death in hospitalized patients. Nephrol. Dial. Transplant.25(6), 1833–1839 (2010).
- Rosner MH , OkusaMD. A cute kidney injury associated with cardiac surgery. Clin. J. Am. Soc. Nephrol.1(1), 19–32 (2006).
- Coppolino G , PrestaP, SaturnoL, FuianoG. Acute kidney injury in patients undergoing cardiac surgery. J. Nephrol.26(1), 32–40 (2013).
- Pedersen KR , RavnHB, PovlsenJVet al. Failure of remote ischemic preconditioning to reduce the risk of postoperative acute kidney injury in children undergoing operation for complex congenital heart disease: a randomized single-center study. J. Thorac. Cardiovasc. Surg.143(3), 576–583 (2012).
- Toth R , BreuerT, CserepZet al. Acute kidney injury is associated with higher morbidity and resource utilization in pediatric patients undergoing heart surgery. Ann. Thorac. Surg.93(6), 1984–1990 (2012).
- Hassinger AB , BackerCL, LaneJCet al. Predictive power of serum cystatin C to detect acute kidney injury and pediatric-modified RIFLE class in children undergoing cardiac surgery*. Pediatr. Crit Care Med.13(4), 435–440 (2012).
- Morgan CJ , ZappitelliM, RobertsonCMet al. Risk factors for and outcomes of acute kidney injury in neonates undergoing complex cardiac surgery. J. Pediatr.162(1), 120–127 (2013).
- Ricci Z , NettoR, GaristoCet al. Whole blood assessment of neutrophil gelatinase-associated lipocalin versus pediatricRIFLE for acute kidney injury diagnosis and prognosis after pediatric cardiac surgery: cross-sectional study. Pediatr. Crit Care Med.2012(13), 667–670 (2012).
- Bellomo R , RoncoC, KellumJA, MehtaRL, PalevskyP. Acute renal failure - definition, outcome measures, animal models, fluid therapy and information technology needs: the Second International Consensus Conference of the Acute Dialysis Quality Initiative (ADQI) Group. Crit. Care8(4), R204–R212 (2004).
- Mehta RL , KellumJA, ShahSVet al. Acute Kidney Injury Network: report of an initiative to improve outcomes in acute kidney injury. Crit. Care11(2), R31 (2007).
- Akcan-Arikan A , ZappitelliM, LoftisLLet al. Modified RIFLE criteria in critically ill children with acute kidney injury. Kidney Int.71(10), 1028–1035 (2007).
- de Geus HR , BetjesMG, BakkerJ. Biomarkers for the prediction of acute kidney injury: a narrative review on current status and future challenges. Clin. Kidney J.5(2), 102–108 (2012).
- KDIGO (Kidney Disease Improving Global Outcome) . Section 2: AKI Definition. Kidney Int. Suppl.2, 19–36 (2012).
- Slagman MC , WaandersF, HemmelderMHet al. Moderate dietary sodium restriction added to angiotensin converting enzyme inhibition compared with dual blockade in lowering proteinuria and blood pressure: randomised controlled trial. BMJ343, d4366 (2011).
- Axler O , AhnstromJ, DahlbackB. An ELISA for apolipoprotein M reveals a strong correlation to total cholesterol in human plasma. J. Lipid Res.48(8), 1772–1780 (2007).
- ExPASy Bioinformatics Resource Panel. Compute pI/Mw tool. http://web.expasy.org/compute_pi/.
- Kumaraswamy SB , LinderA, AkessonP, DahlbackB. Decreased plasma concentrations of apolipoprotein M in sepsis and systemic inflammatory response syndromes. Crit. Care16(2), R60 (2012).
- Soni SS , RoncoC, KatzN, CruzDN. Early diagnosis of acute kidney injury: the promise of novel biomarkers. Blood Purif.28(3), 165–174 (2009).
- Borregaard N , SehestedM, NielsenBS, SengelovH, KjeldsenL. Biosynthesis of granule proteins in normal human bone marrow cells. Gelatinase is a marker of terminal neutrophil differentiation. Blood85(3), 812–817 (1995).
- Seveus L , AminK, PetersonCG, RoomansGM, VengeP. Human neutrophil lipocalin (HNL) is a specific granule constituent of the neutrophil granulocyte. Studies in bronchial and lung parenchymal tissue and peripheral blood cells. Histochem. Cell Biol.107(5), 423–432 (1997).
- Friedl A , StoeszSP, BuckleyP, GouldMN. Neutrophil gelatinase-associated lipocalin in normal and neoplastic human tissues. Cell type-specific pattern of expression. Histochem. J.31(7), 433–441 (1999).
- Cowland JB , BorregaardN. Molecular characterization and pattern of tissue expression of the gene for neutrophil gelatinase-associated lipocalin from humans. Genomics45(1), 17–23 (1997).
- Goetz DH , HolmesMA, BorregaardNet al. The neutrophil lipocalin NGAL is a bacteriostatic agent that interferes with siderophore-mediated iron acquisition. Mol. Cell10(5), 1033–1043 (2002).
- Mori K , LeeHT, RapoportDet al. Endocytic delivery of lipocalin-siderophore-iron complex rescues the kidney from ischemia-reperfusion injury. J. Clin. Invest115(3), 610–621 (2005).
- Mishra J , MoriK, MaQet al. Amelioration of ischemic acute renal injury by neutrophil gelatinase-associated lipocalin. J. Am. Soc. Nephrol.15(12), 3073–3082 (2004).
- Mishra J , MaQ, PradaAet al. Identification of neutrophil gelatinase-associated lipocalin as a novel early urinary biomarker for ischemic renal injury. J. Am. Soc. Nephrol.14(10), 2534–2543 (2003).
- Haase M , BellomoR, DevarajanP, SchlattmannP, Haase-FielitzA. Accuracy of neutrophil gelatinase-associated lipocalin (NGAL) in diagnosis and prognosis in acute kidney injury: a systematic review and meta-analysis. Am. J. Kidney Dis.54(6), 1012–1024 (2009).
- Mishra J , DentC, TarabishiRet al. Neutrophil gelatinase-associated lipocalin (NGAL) as a biomarker for acute renal injury after cardiac surgery. Lancet365(9466), 1231–1238 (2005).
- Cai L , RubinJ, HanW, VengeP, XuS. The origin of multiple molecular forms in urine of HNL/NGAL. Clin. J. Am. Soc. Nephrol.5(12), 2229–2235 (2010).
- Martensson J , XuS, BellM, MartlingCR, VengeP. Immunoassays distinguishing between HNL/NGAL released in urine from kidney epithelial cells and neutrophils. Clin. Chim. Acta413(19–20), 1661–1667 (2012).
- Bolignano D , CoppolinoG, CampoSet al. Urinary neutrophil gelatinase-associated lipocalin (NGAL) is associated with severity of renal disease in proteinuric patients. Nephrol. Dial. Transplant.23(1), 414–416 (2008).
- Bolignano D , CoppolinoG, AloisiCet al. Effect of a single intravenous immunoglobulin infusion on neutrophil gelatinase-associated lipocalin levels in proteinuric patients with normal renal function. J. Investig. Med.56(8), 997–1003 (2008).
- Awad AS , YeH, HuangLet al. Selective sphingosine 1-phosphate 1 receptor activation reduces ischemia-reperfusion injury in mouse kidney. Am. J. Physiol Renal Physiol290(6), F1516–F1524 (2006).
- Lien YH , YongKC, ChoC, IgarashiS, LaiLW. S1P(1)-selective agonist, SEW2871, ameliorates ischemic acute renal failure. Kidney Int.69(9), 1601–1608 (2006).