Abstract
Aim: The goal of this study is to characterize the specific methylation profile triggered by DNMT3B protein isoforms expressed at different levels in breast cell lines. Materials & methods: Microarray DNA methylation data were analyzed and associated with functional genome annotation data. Results: A large spectrum of DNMT3B3/DNMT3B2 expression ratio values was observed in parental breast cell lines. According to their methylation profiles, hierarchical clustering of untransfected cell lines revealed clustering based on their ER/PR status. Overexpression of DNMT3B3 triggered methylation changes of thousands of CpG sites in breast cells. Based on the trend of methylation changes, the results suggest an antiproliferative action of the DNMT3B3 isoform through a dominant negative effect on its wild-type counterpart DNMT3B2. Conclusion: This study revealed specific pathways modulated by DNMT3B isoforms, which could regulate cell proliferation and other biological mechanisms. This illustrates the importance of multiple interactions between isoforms in the complexity of methylation processes.
Keywords::
Relative mRNA expression of human DNMT3B2 (gray bar) and its alternative spliced isoform DNMT3B3 (black bar). Results are expressed in mRNA copy number corrected with the reference gene HPRT1 as mean ± SEM; n = 4.
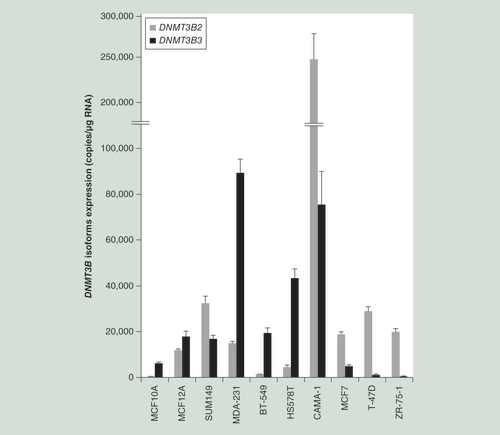
Hierarchical clustering of parental (untransfected) cell lines based on genome-wide methylation data of each cell line. This analysis was performed with GenomeStudio calculating dissimilarities based on absolute correlation using a 1-r distance measure. Rows represent each parental (untransfected) cell line.
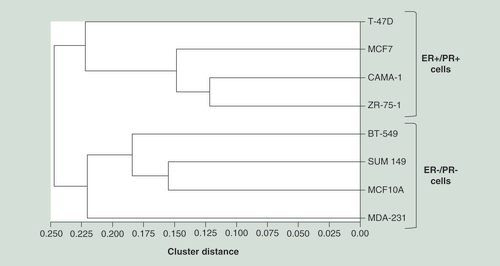
Epigenetics is defined as the inheritance of reversible gene-expression patterns not determined by the nucleotide sequence, and leading to changes in gene expression [Citation1–5]. DNA methylation is one of these epigenetic DNA modifications used by normal cells to regulate gene expression [Citation6].
In cancer, DNA hypermethylation is frequently associated with epigenetic silencing of tumor suppressor genes and genomic instability, while global DNA hypomethylation leads to overexpression of proto-oncogenes, growth factors and genes that are involved in cell proliferation, invasion and metastasis [Citation7–16]. Indeed, the aberrant methylation of CpG island promoters of genes controlling restricted cellular growth is considered important epigenetic hallmarks of cancer [Citation15–19].
On the other hand, as observed for methylation, alternative splicing is another mechanism involved in regulation of gene expression and several other functions, and is also recognized as a key mechanism influencing initiation and development of tumorigenesis [Citation20]. There is also convincing evidence that in several cancers, expression of splice variants is significantly altered and correlates with disease severity [Citation21,Citation22].
Human DNA methylation is introduced into DNA by enzymes of the DNA cytosine methyltransferases family including DNMT1, DNMT3A and DNMT3B [Citation23]. The DNMT3B protein allows a de novo DNA methylation activity in vitro and in vivo [Citation24], which is recognized as a key mechanism involved in the transformation of normal cells into cancer cells [Citation25,Citation26].
DNMT3B expression is elevated in several human cancers [Citation27–30] and its suppression results in tumor cell apoptosis [Citation31]. Moreover, several studies suggest that DNMT3B plays a predominant role over DNMT3A and DNMT1 in breast tumorigenesis, given its overexpression in breast cancer tissues relative to DNMT1/3A [Citation28,Citation32–38].
Among the spliced isoforms generated by the DNMT3B gene, the DNMT3B3 isoform (NM_175849) has been suggested to play a role of dominant negative factor and seems to be involved in the acquisition of anchorage independent growth [Citation39,Citation40].
Despite regions missing from the catalytic domain of DNMT3B3 (motifs VII and VIII as well as the first nine amino acids of catalytic motif IX) [Citation30], in vitro experiments demonstrated that the human DNMT3B3 protein could methylate unmethylated and hemimethylated DNA substrates, thus suggesting that DNMT3B3 was likely contributing to DNA methylation activity in human cells [Citation40,Citation41]. Therefore, this region of the catalytic domain is unlikely required for the in vitro protein activity.
Overexpression of the DNMT3B3 isoform has been observed in cancerous tissues and tumor cell lines [Citation41], while its expression was reported to be essential for cancer cell survival [Citation31]. It has been shown that the antiproliferative effect of DNMT3B depletion observed in A549 lung cells [Citation42], prostate and breast cancer cells [Citation43–45], T24 human bladder cancer cells [Citation46] as well as in HCT116 human colon cancer cell line [Citation47], was rescued in HCT116 cells by exogenous expression of either of the splice variants DNMT3B2 or DNMT3B3 but not DNMT1. These results indicate that DNMT3B has significant site selectivity that is distinct from DNMT1, regulates aberrant gene silencing and is essential for cancer cell survival [Citation31]. This suggests that DNMT3B2 and DNMT3B3 perform similar roles in cancer cells clearly distinct from those of DNMT1. In addition, ovarian steroids have been reported to alter the expression of DNMTs in human endometrium of endometriotic lesions [Citation48].
Given that the DNMT3B3 isoform was found to be highly expressed in individuals of non-BRCA1/2 breast cancer families and that this isoform has been recently shown to interact with and modulate the activity of the other active methylating isoforms [Citation49], we undertook to discriminate the specific methylation profile associated with this DNMT3B3 isoform and its reference counterpart (DNMT3B2) in breast cell lines. This revealed specific pathways targeted by these DNMT3B isoforms, which could be significantly involved in control of carcinogenesis development.
Materials & methods
Cell culture & constructions
The human breast cancer cell lines MCF7, CAMA-1, ZR-75-1, T-47D, MDA-MB-231 as well as the human MCF10A cell line derived from normal breast are all routinely cultured in our laboratory, and the conditions have been optimized as recommended by the American Type Culture Collection (ATCC, VA, USA). The human breast cancer cell lines SUM149, BT-549 and Hs578T were kindly provided by Dr Stéphane Gobeil (CHU de Québec Research Centre). All cells were plated in T75 flasks, maintained at 37°C and 5% CO2 in medium recommended by the ATCC. The SUM149 cells were grown in Ham’s F12 medium supplemented with 5% FBS, 500 ng/ml hydrocortisone and 10 μg/ml insulin. Human Embryonic Kidney 293 (HEK293T) cells were maintained in DMEM medium supplemented with 5% FBS. All media and supplements for cell culture were purchased from Wisent Inc. (St-Bruno, QC, Canada). The hydrocortisone, insulin and EGF were purchased from Sigma-Aldrich (Sigma-Aldrich Canada Co., ON, Canada) and E2 was obtained from Steraloids (Newport, RI, USA).
The human DNMT3B2 (referred to as the reference isoform in this study; NM_175848) and DNMT3B3 (NM_175849) cDNAs were PCR-amplified from a non-BRCA1/2 individual using primers introducing BamHI and XbaI restriction sites as described in Supplementary Table 1A. PCR products were then digested and introduced into the pcDNA3.1(+) vector (ThermoFisher Scientific, Waltham, MA, USA).
To enhance the effect triggered by the DNMT3B3 isoform on DNA methylation in ER+/PR+ cell lines (MCF7, T-47D, ZR-75-1 and CAMA-1), the strategy was to increase the expression of DNMT3B3 combined with a decrease of DNMT3B2 expression. This modulation was achieved by stably transducing a shRNA targeting DNMT3B2 in exon 21 (this exon is missing in DNMT3B3), and by overexpressing the DNMT3B3 isoform through transient transfection. To generate stable cell lines, lentiviruses were produced by cotransfecting the pLKO.1 vector expressing DNMT3B shRNA (Sigma-Aldrich, MISSION shRNA NM_006892.3-2553s1c1, GCCCGTGATAGCATCAAAGAA) or scramble shRNA with lentiviral plasmid pMD2.G and psPAX2 (Addgene, MA, USA) into HEK293T cells. Given its high proliferation and transfectable characteristics, HEK293T cells are widely used in cell biology research and by the biotechnology industry to produce therapeutic proteins and viruses for gene therapy. Fourty-eight hours following transfection, the MCF7, T-47D, CAMA-1 and ZR-75-1 cells lines were transduced with the filtrated viral supernatants supplemented with 4 μg/ml polybrene as previously described [Citation50,Citation51]. The virus was removed 24 h later and cells were selected for resistance to puromycin. The stable cell lines were then transiently transfected for 72 h with the pcDNA3–DNMT3B3 vector using lipofectamine 2000 reagent (ThermoFisher Scientific) in triplicates to produce cells expressing high levels of the alternative spliced isoform DNMT3B3.
Regarding MDA-MB-231, BT-549 and MCF10A cell lines, transient overexpression of DNMT3B2 was sufficient to reverse the expression ratio between both DNMT3B isoforms. It should be noted that it was impossible to specifically reduce the expression of DNMT3B3 in ER-/PR- cells without affecting the subsequent transient overexpression of the DNMT3B2 isoform.
Thus, transient transfection of pcDNA3-DNMT3B2 in BT-549, MDA-MB-231 and MCF10A cells were performed in three biological replicates with lipofectamine 2000 reagent following manufacturer’s recommendations for 72 h.
DNA extraction & DNA methylation analysis
Genomic DNA was isolated using the QIAamp DNA mini kit (QIAGEN Inc., Toronto, ON, Canada) as described by the manufacturer. DNA samples were processed at McGill University and Génome Québec Innovation Centre (Montreal, Canada) to perform quantitative DNA methylation measurement using the Infinium Methylation Assay. Briefly, DNA (1 μg) was treated with bisulfite and converted DNA was then hybridized on the Infinium Human Methylation 450 BeadChip according to manufacturer’s instructions (Illumina Inc., CA, USA). Visualization and analysis of methylation data were conducted using the GenomeStudio software version 2011.1 (Illumina Inc.) and the Methylation Module (1.9.0). Sample signals were normalized with internal control probe pairs and background-subtracted.
The methylation level or β-value is a ratio between methylated probe intensity and total probe intensities (sum of methylated and unmethylated probe intensities) based on the formula: β-value = methylated allele intensity/(unmethylated allele intensity + methylated allele intensity + 100). For quality control (QC), methylation measures with a detection p-value > 0.05 and samples with CpG coverage <95% were removed. In addition, probes corresponding to CpG not annotated in the human genome build 37, located on sex chromosomes (X and Y) or having polymorphism located within or near the probe sequence were filtered out from analysis.
For the MCF7, CAMA-1, ZR-75-1 and T-47D cell lines, differential methylation was computed using the Illumina Custom model between scramble shRNA + empty pcDNA3 vector samples (so-called B2-overexpressing cells; mean β-values) and corresponding cell lines cotransfected with shRNA targeting DNMT3B2 + pcDNA3-DNMT3B3 (so-called B3-overexpressing cells). In MDA-MB-231, MCF10A and BT-549 cells, differential methylation was computed between the empty pcDNA3 vector-transfected cells (so-called B3-overexpressing cells) and DNMT3B2-transfected pcDNA3 cells (so-called B2-overexpressing cells). False-discovery-rate-corrected (FDR-corrected), β-values and DiffScores were then computed. FDR cutoff ≤5% was applied to correct for multiple testing. DiffScore defined as the differential methylation score calculated from β-values and differences in β-values (delta β) between the two groups (B2- and B3-overexpressing cells), was used as the main statistical result for comparison between each subgroup. CpG sites associated with a significant DiffScore value ≥|22| (correlated p-value ≤ 0.01) were considered as significant. It should be noted that p-values below 0.01 or 0.05 have been considered as significant cutoff p-values in several studies [Citation52–56].
Unsupervised hierarchical clustering was performed with Genome Studio software using whole raw methylation data from parental (untransfected) cell lines, and the analysis was carried out using the absolute correlation cluster metric.
Pathway & correlation analysis
Pooled differential methylation analysis combining data from ER+/PR+ (MCF7 and T-47D) cell lines as well as ER-/PR- (MDA-MB-231 and BT-549) cell lines resulted in two distinct lists of genes (ER+/PR+ and ER-/PR-), which were then submitted to functional analysis. The genes associated with a significant differential undermethylation or overmethylation value of a given CpG site were then displayed using Venn diagrams, which allowed identifying common genes between several categories. Venn diagrams were created using the VENNY software [Citation57].
Identification of overrepresented pathways, functions and gene-associated diseases were performed using the Ingenuity Pathway Analysis (IPA, QIAGEN) software with default settings [Citation58,Citation59]. Gene lists were uploaded using gene symbols and submitted for IPA Core Analysis. IPA calculates p-values that reflect the statistical significance of association between the genes and the networks by the Fisher’s exact test. p-value ≤ 0.05 was considered significant.
Prediction of the activation state of genes associated with differentially methylated promoter CpG sites following overexpression of DNMT3B2 and DNMT3B3 isoforms (opposite DNMT3B3/DNMT3B2 expression ratios) has also been performed using IPA. The direction of data methylation change was inverted to mimic a change in expression ratio, and the top methylation value was selected when several promoter CpG sites were affected for the same gene.
RNA extraction, semiquantitative & quantitative real-time PCR
Total RNA was extracted using TRI Reagent (Sigma-Aldrich Canada Co.) and purified using the Total RNA purification kit with DNAse on-column step (Sigma-Aldrich Canada Co.) according to the manufacturer’s instructions. The quality of RNA was analyzed on an Agilent 2100 Bioanalyzer (Agilent Technologies Inc, CA, USA). cDNA synthesis and purification were carried out as reported previously [Citation50–51,Citation60–61]. cDNA was then used to perform fluorescent-based Realtime PCR quantification using the LightCycler Realtime PCR apparatus (Roche Inc., NJ, USA). PCR amplification and reading of the fluorescence signal was performed as previously described [Citation62–64]. Four biological replicates (n = 4) were performed for each sample, mRNA expression levels are expressed as number of copies/μg total RNA using a standard curve of Cp versus logarithm of the quantity. Data calculation and normalization were performed with second derivative and double correction method using the housekeeping gene Hprt1 as described previously [Citation64]. The list or primers used for amplification of DNMT3B isoforms can be found in Supplementary Table 1B.
Results
Using quantitative PCR and primers designed to specifically amplify DNMT3B2 and DNMT3B3 transcripts, the relative expression of both the DNMT3B2 and DNMT3B3 isoforms were established in different breast cancer cell lines and the noncancerous breast cell lines MCF10A and MCF12A. The DNMT3B3 isoform lacks 63 amino acid residues (exons 20-21 of the DNMT3B2 isoform). As illustrated in , the cell lines analyzed displayed a large spectrum of DNMT3B3/DNMT3B2 expression values ranging from very weak expression of DNMT3B3 in T-47D and ZR-75-1 cell lines, to the highest expression observed in cancerous MDA-231 cells. Of interest, the ER and PR status in cells seems to be associated with the expression level of DNMT3B isoforms. The DNMT3B2 expression is higher than DNMT3B3 in ER+/PR+ cell lines, while the expression of the reference isoform (DNMT3B2) is lower in most of ER-/PR- cell lines, with the exception of SUM149 cells.
Depending on the DNMT3B3/DNMT3B2 expression ratio found in each cell line, different combinations of transfections/transductions were performed as described in the ‘Materials and Methods’ section in order to generate the highest and lowest DNMT3B3/DNMT3B2 expression ratio for all cell lines. The DNMT3B3/DNMT3B2 expression ratio obtained in each untransfected and modified cell line is indicated in .
DNA methylation levels of individual CpG sites in modified and parental (untransfected) breast cell lines were measured using the HumanMethylation450 BeadChip. Differential methylation analysis of all CpGs sites was performed between the so-called DNMT3B2-overexpressing and DNMT3B3-overexpressing cells.
Unsupervised hierarchical clustering analysis using the genome-wide methylation profile of untransfected cell lines revealed clustering of cells based on their ER/PR status (). Indeed, the MDA-231, MCF10A, SUM149 and BT-549 clustered together, while the ER+/PR+ cell lines namely ZR-75-1, CAMA-1, MCF7 and T-47D distinctly gathered with each other in a separate cluster. Thus, the unsupervised cluster analysis did not group cells based on their expressing levels of DNMT3B2, but rather on their ER/PR expression status.
The differential methylation analyses between the DNMT3B2-overexpressing and DNMT3B3-overexpressing modified cell lines were performed for each strain of cells analyzed in this study. Significant variations of gene methylation levels associated with the action of the DNMT3B3 isoform are displayed in . It should be noted that the SUM149 cell line has been excluded from further analysis given the low yield of transfection/transduction levels observed.
In ER+/PR+ cells (), overexpression of DNMT3B3 triggered methylation changes of 36,856 CpG sites (10,530 genes) in MCF7, 6013 CpG sites (3 152 genes) in T-47D, 446 CpG sites (307 genes) in ZR-75-1 and 82 CpG sites (53 genes) in CAMA-1 cells. Considering exclusively CpG sites located in gene promoters, 11,793 (7 040 genes), 1973 (1686 genes), 178 (161 genes) and 18 (17 genes) CpG sites have been significantly affected in MCF7, T-47D, ZR-75-1 and CAMA-1 cell lines, respectively.
Overlap of common CpG sites and genes between cell lines were illustrated by Venn diagrams in Supplementary Figure 1A & B. The overlap between MCF7 and T-47D cells showed the highest number of CpG sites (400), which are commonly identified in both cell lines. However, taking into account the gene ID only, without regard for gene location of a given CpG, 1204 genes (850 undermethylated and 354 overmethylated) were commonly and differentially methylated following overexpression of the DNMT3B3 transcript in both cell lines. It should be noted that several CpGs (not necessarily differentially methylated in both cell lines) can be associated to a given gene, which explains the higher number of genes than CpG sites commonly identified in both cell lines. Therefore given that only a few CpG sites (46 undermethylated and 29 overmethylated sites) were commonly affected between CAMA-1/ZR-75-1 and MCF7/T-47D cells, we excluded CAMA-1 and ZR-75-1 cell lines from further pooling analyses.
Hence, using pooled methylation data (β-values) from MCF7 and T-47D sample cells (), 14,619 CpG sites (6454 genes) were found to be differentially methylated by the overexpression of DNMT3B3, which included 4510 CpG sites (3464 genes) located in gene promoters. Combination of MCF7 and T-47D methylation data revealed that 74% of CpG sites (2431 genes) were undermethylated, while 26% (1033 genes) were overmethylated by the DNMT3B3 isoform.
The same analysis was performed in ER-/PR- cell lines (MDA-231, BT-549 and MCF10A). As presented in , overexpression of DNMT3B3 triggered significant methylation changes of 1,527 CpG sites (1094 genes) in MDA-231 with the vast majority being undermethylated, 50 CpG sites (39 genes) in BT-549 and 739 CpG sites (496 genes) in noncancerous MCF10A cells. Considering exclusively CpG sites located in gene promoters, 1035 (858 genes), 16 (16 genes) and 248 (212 genes) CpG sites have been significantly affected in MDA-231, BT-549 and MCF10A cell lines, respectively. Using exclusively pooled methylation data (β-values) from cancerous MDA-231 and BT-549 sample cells, 1461 CpG sites (1059 genes) were found to be differentially methylated by the overexpression of DNMT3B3, which included 996 CpG sites (847 genes) located in gene promoters (). Combination of methylation data from these ER-/PR- cell lines revealed that more than 99% of CpG sites were undermethylated by the DNMT3B3 isoform.
As displayed in Supplementary Figure 1C & D, comparison of common and differentially methylated CpG sites and genes between cell lines revealed that 14 CpG sites (13 undermethylated and one overmethylated) were commonly affected in all three ER-/PR- cell lines analyzed. Without regard for the CpG site location, 16 genes were found to be commonly and differentially methylated in all three cell lines.
Common CpGs and genes identified using pooled methylation data from ER+/PR+ as well as ER-/PR- cell lines were displayed in Supplementary Figure 2. Of interest, 254 genes (253 undermethylated and one overmethylated) have been pinpointed to be commonly affected by the overexpression of DNMT3B3.
The distribution of gene location of CpG sites commonly affected in cancerous ER+/PR+ cell lines (MCF7 and T-47D) as well as in ER-/PR- cell lines (MDA-231 and BT-549) was described in & Supplementary Figure 3 (MCF7 and T-47D) and Supplementary Figure 4 (MDA-231 and BT-549).
Approximately 3% of all CpG probes interrogated on the BeadChip were significantly affected by the overexpression of DNMT3B3 in MCF7/T-47D cell lines and 26.2% of these loci were overmethylated, while the remaining 73.8% was undermethylated (Supplementary Figure 3A & B). In ER-/PR- cell lines (MDA-231 and BT-549), 0.63% of all probes were significantly affected and the large majority of them was undermethylated (99.7%) (Supplementary Figure 4A & B).
A global overview of all differentially methylated CpG sites in ER+/PR+ and ER-/PR- cell lines revealed that these methylated loci were distributed in similar proportion over the 22 chromosomes (X and Y chromosome were excluded) (Supplementary Figures 3C & 4C).
Exhaustive analysis of the distribution of these loci in the genome is found in , while the percentage values of this distribution are indicated in Supplementary Figures 3D (ER+/PR+ cell lines) and 4D (ER-/PR- cell lines).
The CpG sites can be located in different parts of the genome such as in the promoter region (TSS1500: 1500 bp of the transcription start site and TSS200: 200 bp of the transcription start site), the 5′ or 3′ untranslated (UTR) region, the first exon, the gene body or in intragenic regions. As seen in , most of the CpG sites were situated in promoter regions (including the 5′-UTR) and gene body regions in ER+/PR+ and ER-/PR- cell lines. However, a significant number of CpG sites in ER+/PR+ (4755) and ER-/PR- cell lines (175) were also located in intergenic regions.
In addition to the genome location, the exact location of these CpG sites relative to CpG islands is described in Supplementary Figure 3E (ER+/PR+ cell lines) and 4E (ER-/PR- cell lines). Indeed, CpG sites could be located upstream (N_Shelf: 2-4 kb and N_Shore: <2 kb) or downstream of CpG islands (S_Shelf and S_Shore) as indicated in these Supplementary Figures (Supplementary Figures 3E & 4E). While the distribution of CpG sites seemed to be relatively equally distributed in ER+/PR+ cells, the large majority of CpG sites (81%) in ER-/PR- cell lines were located in CpG islands. Similar observations were seen when considering only the CpG sites located in promoter regions (Supplementary Figures 3F & 4F).
All genes associated with differentially methylated sites (p < 0.01) were then submitted for pathway, molecular function and disease-associated analyses. Several canonical pathways and functions using Ingenuity Pathway analysis were identified following overexpression of the DNMT3B3 isoform.
As displayed in , pathway analysis in ER+/PR+ cell lines (MCF7 and T-47D) identified the G-protein coupled receptor signaling (p = 1.03 × 10-10) as the most overrepresented pathway based on genes associated with undermethylated CpGs. The same observation was observed when all genes (undermethylated and overmethylated) were submitted to IPA analysis. In this study, overmethylated CpG sites and their associated genes could be considered as being specifically methylated by the DNMT3B3 isoform. Therefore it was very interesting to find that overmethylated genes in ER+/PR+ cell lines were likely involved in molecular mechanisms of cancer (p = 8.67 × 10-5) as well as regulation of the epithelial-mesenchymal transition (p = 1.25 × 10-4), two groups of genes intimately involved in carcinogenesis development. Moreover, cellular development and cell death and survival (p = 6.8 × 10-17 and 2.4 × 10-16) represent the most overrepresented functions associated with the overmethylated genes, while cellular movement and cell-to-cell signaling and interaction were identified using all (over- and undermethylated) or only undermethylated genes. In addition, genes identified in ER+/PR+ cell lines were also associated with disease such as cancer and organismal injury and abnormalities ().
The same analysis performed with genes identified in ER-/PR- cell lines is found in . Given the low number of genes associated with overmethylated CpG, which have been identified in ER-/PR- cells, overrepresented pathways were only identified using undermethylated genes. Thus, genes associated with undermethylated CpGs were mostly associated with Rho family GTPases signaling (p-value ranging from 1.1 × 10-4 to 1.3 × 10-3) involved in coordination of the actin cytoskeleton, modulation of the formation of signaling reactive oxidant species and control of gene transcription. Of great interest, a significant portion of these undermethylated genes were also implicated in breast cancer through the regulation of Stathmin1 (p = 1.6 × 10-3). As also described in , IPA analysis revealed cellular growth and proliferation as well as cell death and survival (p = 2.9 × 10-8 and 8.3 × 10-7, respectively) as enriched molecular and cellular functions in ER-/PR- cells. Again, genes associated with cancer and endocrine disorders were significantly overrepresented with 820 and 205 genes, respectively.
In addition, this IPA analysis was carried out using genes (n = 254) commonly identified in ER+/PR+ and ER-/PR- cell lines (see Supplementary Figure 2B). As seen in , Axonal guidance signaling (p = 5.3 × 10-4) and breast cancer regulation by Stathmin1 (p = 1.7 × 10-3) were two biological processes which are overrepresented. Cellular assembly (60 genes), cellular function and maintenance (80 genes) and growth and proliferation (88 genes) represent the molecular functions enriched by these genes. As observed in ER+/PR+ cell lines, cancer (210 genes) and organismal injury and abnormalities (211 genes) were the major diseases associated with these genes.
Therefore, altogether these analyses revealed enrichment of several pathways and functions involved in carcinogenesis, particularly in ER+/PR+ cell lines. Moreover, genes which were likely affected by the expression of DNMT3B3 (overmethylated genes) in ER+/PR+ cell lines were more associated with carcinogenesis than undermethylated genes, which could result from a lower activity of the DNMT3B2 isoform disturbed by the high expression of the DNMT3B3 isoform.
However, the involvement of genes in specific pathways or functions does not indicate whether the gene activates or represses these biological processes. Therefore, an additional analysis aiming to anticipate the action of each isoform on the activation or repression of specific biological and molecular processes was also performed for all promoter CpG sites using the sense of methylation change. This analysis was carried out according to the well-known concept associating the increase of methylation levels of promoter CpG sites and repression of gene expression and vice-versa. These projections are found in . It should be noted that for several CpG sites being simultaneously affected in the same promoter region, with the exception of only a few genes where one CpG site showed inverse methylation change compared with other CpG sites, all promoters CpG sites of a given gene were affected in a same way for more than 95% of genes analyzed (data not shown).
Based on predicted gene expression behavior, in ER+/PR+ cell lines, the DNMT3B3 protein seems to activate general functions related to organism survival, developmental disorders and inflammation (). On the other hand, the DNMT3B2 protein could potentially activate proliferation, migration, differentiation of cells as well as transport, organization and angiogenesis. Although the significativity of results was much lower than that found in ER+/PR+ cells, the same analysis performed in ER-/PR- cells and displayed in , revealed that the DNMT3B3 isoform could activate various processes involved in organism survival, dermatological diseases as well as endocrine and reproductive system disorders, while the DNMT3B2 isoform, as found in ER+/PR+ cell lines, could activate all functions related to cell proliferation and migration of tumor cells.
Discussion
DNMT3B has been proposed as a candidate target for drug development in oncology [Citation65]. However, given the high diversity of splicing variants encoded by the DNMT3B gene and the large variation of expression of DNMT3B2 and DNMT3B3 isoforms in human and cancer cells, we undertook to discriminate the targets of methylation of both proteins and thus enhance knowledge on DNMT3B splicing variants in the regulation of gene expression through DNA methylation mechanisms.
The DNMT3B gene has been shown to be overexpressed in several types of tumors [Citation27,Citation66–67], while antisense-mediated depletion of DNMT3B-triggered apoptosis and demethylation of tumor suppressor genes in cancer cells [Citation49]. Indeed, as shown in colon cancer mouse model, overexpression of Dnmt3b contributed directly to aberrant methylation in cancer [Citation68]. Unlike DNMT1 and DNMT3A genes, which encode only a few splicing variants [Citation69,Citation70], more than 30 different DNMT3B isoforms ensuing from alternative splicing involving alternative sequence or promoter usage have been reported to be expressed in mouse and human cells [Citation30,Citation70–75].
Although the DNMT3B3 isoform was initially considered as catalytically inactive [Citation39,Citation76–77], this transcript has been shown to either contribute to DNA methylation activity or to be associated with the ability to maintain aberrant DNA methylation patterns in cancer [Citation31,Citation40–41]. Indeed, as demonstrated for numerous spliced gene isoforms [Citation78–83], the DNMT3B3 isoform has been recently shown to interact with and modulate the activity of the active methylating DNMT isoforms through a dominant negative effect [Citation39,Citation49]. The human DNMT3B3 isoform was considered inactive based on the activity associated with the corresponding murine isoform, which is unable to methylate the usual DNMT3B targets, SAT2 [Citation39]. Weisenberg et al. concluded that DNMT3B3 is likely involved in methylation profile of normal cells, while additional trans-acting factors are likely involved for modulating DNMT3B activity in cancer cells [Citation41]. The incomplete and partial depletion of DNMT3B3 triggered by a 5-Aza-CdR treatment supports the interaction of DNMT3B3 with DNA [Citation41]. It has been also proposed that DNMT3B3 may interact with other DNMTs upon complex formation and modulate their catalytic activity to regulate DNA methylation [Citation41,Citation49]. In addition, the plant homeodomain (PHD) and PWWP domains required for targeting DNMT3A and DNMT3B to pericentromeric heterochromatin contribute also to protein–protein interactions by recognition of histone modifications [Citation84–86]. Indeed, the PWWP domain induces recruitment of DNMT3B proteins to pericentromeric heterochromatin, while the PHD domain is involved in protein-protein interactions and the ability of DNMT3B to repress transcription in a histone-deacetylase (HDAC)-dependent manner [Citation85,Citation87–88]. Although DNMT3B3 could stimulate de novo methylation activity of DNMT3A, in vitro assays demonstrated that the addition of DNMT3B3 led to a progressive decrease in DNA methylation activity of DNMT3A2 in the presence of DNMT3L [Citation49]. This illustrates the complex/intricate interactions occurring between DNMT proteins.
The DNMT3B3 isoform is ubiquitously expressed in normal human tissues [Citation49]. As described in this study, this isoform is highly expressed in non-BRCA1/2 high-risk breast cancer individuals (data not shown) and ER/PR-negative breast cancer cells. Both the DNMT3B2 and DNMT3B3 isoforms were reported to be the major mRNA isoforms expressed in most of mouse and human normal and cancerous cells analyzed, with DNMT3B3 being highly expressed in all cell lines [Citation41]. A switch of DNMT3B1/2 to DNMT3B3 expression has been shown to correlate with acquisition of anchorage-independent growth and differentiation of human embryonic stem cells [Citation40,Citation89].
Correlation observed between methylation profile and expression of DNMT3B3 in LD419 fibroblasts and T24 bladder cells strongly suggests an important role for this transcript in methylation [Citation41]. However, its expression alone could not explain the differential methylation of DNMT3B3 target sequences, thus other factors related to gene transcription or chromatin remodeling are likely involved in specific gene methylation.
Based on their genome-wide methylation profile, our clustering analyses of parental breast cell lines led to two distinct subgroups of hormone receptor (ER and PR)-positive and -negative cell lines. This clustering is in agreement with the genome-wide methylation analysis performed by Sun et al., where breast cancer cells displayed differential CpG island methylation depending on their ER status [Citation90]. These results were also similar to the 64 methylation-sensitive genes expression reported by Roll et al., where ZR-75-1 and MCF7 cells clustered together as low-frequency methylators, while MDA-231 and BT-549 cell lines were considered as hypermethylators [Citation38]. In the latter study, hypermethylation was defined as a six-gene hypermethylator signature (CDH1, CEACAM6, CST6, ESR1, LCN2 and SCNN1A), whereas the low-frequency methylator cell lines did not methylate these genes [Citation38]. In addition, Han and colleagues established the genome-wide methylation profile in 40 breast cancer cell lines, which revealed gene methylation signatures discriminating ER- and ER+ cell lines [Citation56]. Distinct clusters between ER- and ER+ cells have been identified using 345 significant genes with p-value < 0.05.
In our study, the overexpression of DNMT3B3 triggered a decrease in global methylation of most cell lines analyzed. An increased global methylation was observed only in ZR-75-1 and T-47D cell lines. Analysis of combined methylation data of two ER+/PR+ (MCF7 and T-47D) as well as two ER-/PR- (MDA-MB-231 and BT-549) cell lines revealed a decrease in methylation of 75 and 99.6% of all CpG sites differentially methylated in hormone receptor-positive and -negative cell lines, respectively.
Hence, genomic hypomethylation can potentially lead to chromosomal instability, aberrant activation of endogenous retroviral elements and oncogenes as well as loss of imprinting in genes such as SNCG, which is demethylated and leads in a more aggressive breast cancer phenotype [Citation91,Citation92]. Therefore, as observed in ICF syndrome caused by germline DNMT3B mutations, the associated decrease in Sat2 methylation renders DNMT3B likely involved in hypomethylation [Citation93].
Mutant isoforms of the DNMT3B gene showed decreased activity (when compared with the wild type isoform) or can disrupt their interactions with DNMT3L [Citation94,Citation95]. However, the DNMT3B3 protein showed also a direct methylation effect on a significant number of genes through its catalytic activity, while the global undermethylation observed could result from interactions with other DNMTs, which inhibit their function. A decrease in DNMT3B2 activity could be envisaged to explain this undermethylation caused by the overexpression of the DNMT3B3 protein.
Of interest, depletion of DNMT1 gene in human cancer cells resulted in a 20% decrease in overall genomic methylation [Citation96], while silencing of DNMT3B in HCT116 colorectal cancer cell line reduced global DNA methylation by less than 3% [Citation97]. This is in agreement with our global methylation variation of approximately 2.8% observed in DNMT3B3-transfected T-47D and MCF7 cell lines. However, gene disruption of both the DNMT1 and DNMT3B reduced genomic DNA methylation by greater than 95%, which illustrates the cooperation of both proteins in regulation of methyltransferase activity [Citation97].
The slight variation in methylation level under the direct action of DNMT3B has also been shown by Hagemann’s study, where siRNA knockdown of DNMT3B led to significant methylation changes of a negligible fraction of the genome in HCT-116 cells of less than 1% using 450K arrays [Citation65]. On the other hand, this latter study demonstrated that DNMT3B was required for maintaining proliferation, preventing apoptosis and supporting survival of colon cancer cells.
Dnmt3b has recently been identified as the major de novo methyltransferase methylating body regions of transcribed genes in mouse [Citation98]. Challen et al. reported an increased hypomethylation of about 20% in all gene locations (genic, ext gene, 5′ and 3′UTR and intergenic) following DNMT3B knockout in hematopoietic stem cell [Citation99]. This supports our results which identify similar differential methylation in all genomic locations (Supplementary Figures 3 & 4). Indeed, SETD2-mediated methylation of lysine 36 on histone H3 and a functional PWWP domain of catalytically active DNMT3B isoforms are required for selective binding of the mouse dnmt3b gene [Citation98]. De novo methylation of the mouse dnmt3b1 isoform is preferentially targeted to genomic regions with elevated H3K36me3 levels reflecting the recruitment of dnmt3b1 to regions of active transcription [Citation98]. This also supports our results demonstrating high levels of differential methylation found in gene body regions and CpG islands.
In our study, several genes targeted by the DNMT3B3 isoform activity were specifically involved in inflammation, immunity and cell proliferation processes. Although specific pathways have been identified to be potentially regulated by DNMT3B2 and DNMT3B3 proteins, we should keep in mind that the effects reported in this study are more likely related to extreme changes in DNMT3B3/DNMT3B2 expression ratio than individual expression of both isoforms. Therefore as described in , based on predicted up- or downregulation of gene expression established upon methylation level variations triggered by both DNMT3B isoforms, a high expression of DNMT3B3 (or high DNMT3B3/DNMT3B2 expression ratio) could repress cell growth and proliferation, while the DNMT3B2 protein (low DNMT3B3/DNMT3B2 expression ratio) could promote these cancer-related processes in all cell lines analyzed. Based on these results, the reported anti-proliferative effect of DNMT3B [Citation65] could therefore involve high expression of DNMT3B3, high DNMT3B3/DNMT3B2 expression ratio or low expression of DNMT3B2. It has been reported that the antiproliferative effect of DNMT3B was not associated with direct DNA methylation [Citation65], thus other roles or protein activities and interactions of the alternative DNMT3B variants remain to be elucidated.
Conclusion
To our knowledge, this is the first publication describing the specific pathways and methylation spectrum affected by DNMT3B splicing variants. Therefore, given the key functions played by DNMT3B, it will be of great interest to further investigate the roles of alternative DNMT3B isoforms in methylation, regulation of gene expression as well as key biological mechanisms regulating cancer development and treatment. Given aberrant methylation in cancer cells, establishment of the exact functions of each protein variant could help in targeting the most appropriate approach in drug development for cancer treatment and control of cell proliferation.
Table 1. DNMT3B3 and DNMT3B2 expression levels in untransfected and transfected/transduced breast cell lines.
Table 2. Number of CpG sites and associated genes identified as differentially methylated in ER+/PR+ breast cell lines.
Table 3. Number of differentially methylated CpG sites according to their gene location.
Table 4. Over-represented pathways, functions and diseases triggered by the overexpression of the DNMT3B3 isoform in ER+/PR+ cell lines.
Table 5. Overrepresented pathways, functions and diseases triggered by the overexpression of the DNMT3B3 isoform in ER-/PR- cell lines.
Table 6. Over-represented pathways, functions and diseases triggered by the overexpression of the DNMT3B3 isoform using affected genes commonly identified in ER+/PR+ and ER-/PR- cell lines.
Table 7. Predicted effect of DNMT3B isoform expression on relevant biological processes in ER+/PR+ cell lines.
The DNMT3B3 isoform lacks motifs VII and VIII as well as the first nine amino acids of catalytic motif IX.
A large spectrum of DNMT3B3/DNMT3B2 expression ratio values was observed in cancer and noncancerous cell lines and this expression ratio is likely related to ER/PR expression status in most of breast cell lines examined.
Based on their methylation profiles, hierarchical clustering of parental breast cell lines revealed clustering of cells based on their ER/PR expression status.
Overexpression of DNMT3B3 triggered methylation changes of thousands of CpG sites in MCF7, T-47D, MDA-231 and MCF10A cells.
Based on the number of undermethylated and overmethylated sites triggered by the overexpression of DNMT3B3, this isoform could act as a dominant negative factor on DNMT3B2 protein activity.
Based on the trend of methylation changes, the results suggest an antiproliferative effect of the DNMT3B3 isoform, while the DNMT3B2 isoform could promote cancer-related processes in all cell lines analyzed.
Based on Ingenuity Pathway Analysis, cancer is the major disease associated with genes affected by the overexpression of DNMT3B3 in ER+/PR+ and ER-/PR- breast cell lines.
Data deposition
The genome-wide methylation data have been deposited in NCBI’s Gene Expression Omnibus and are accessible through GEO Series accession number GSE77348.
Supplemental Figure 1
Download MS Power Point (510.8 KB)Supplemental Figure 2
Download MS Power Point (255.7 KB)Supplemental Figure 3
Download MS Power Point (1.9 MB)Supplemental Figure 4
Download MS Power Point (2 MB)Supplemental Tables
Download MS Word (15.7 KB)Acknowledgements
The authors would like to thank N Paquet involved in qRT-PCR experiments and S Gobeil for his precious help with retroviruse-related experiments. The authors also thank F Guénard at the Institut sur la Nutrition et les Aliments Fonctionnels (Laval University) for his precious help regarding genome-wide methylation statistical analyses.
Supplementary data
To view the supplementary data that accompany this paper please visit the journal website at: www.tandfonline.com/doi/full/10.2217/epi-2016-0013
Financial & competing interests disclosure
This work was supported by the Canadian Cancer Society through its Innovation grant program. The authors have no other relevant affiliations or financial involvement with any organization or entity with a financial interest in or financial conflict with the subject matter or materials discussed in the manuscript apart from those disclosed.
No writing assistance was utilized in the production of this manuscript.
Additional information
Funding
References
- Esteller M . Epigenetics in cancer . N. Engl. J. Med.358 ( 11 ), 1148 – 1159 ( 2008 ).
- Miozzo M , VairaV , SirchiaSM . Epigenetic alterations in cancer and personalized cancer treatment . Future Oncol.11 ( 2 ), 333 – 348 ( 2015 ).
- Chen X , JinP . Current status of epigenetics and anticancer drug discovery . Anticancer Agents Med. Chem.16 ( 6 ), 699 – 712 ( 2016 ).
- Kumar R , LiDQ , MüllerS , KnappS . Epigenomic regulation of oncogenesis by chromatin remodeling . Oncogene ( 2016 ) ( Epub ahead of print ).
- Ning B , LiW , ZhaoW , WangR . Targeting epigenetic regulations in cancer . Acta Biochim. Biophys. Sin. (Shanghai)48 ( 1 ), 97 – 109 ( 2016 ).
- Kanwal R , GuptaS . Epigenetic modifications in cancer . Clin. Genet.81 ( 4 ), 303 – 311 ( 2012 ).
- Jones PA , BaylinSB . The epigenomics of cancer . Cell128 ( 4 ), 683 – 692 ( 2007 ).
- Stearns V , ZhouQ , DavidsonNE . Epigenetic regulation as a new target for breast cancer therapy . Cancer Invest.25 ( 8 ), 659 – 665 ( 2007 ).
- Pathiraja TN , StearnsV , OesterreichS . Epigenetic regulation in estrogen receptor positive breast cancer – role in treatment response . J. Mammary Gland Biol. Neoplasia15 ( 1 ), 35 – 47 ( 2010 ).
- Holm K , HegardtC , StaafJet al. Molecular subtypes of breast cancer are associated with characteristic DNA methylation patterns . Breast Cancer Res.12 ( 3 ), R36 ( 2010 ).
- Ordway JM , BudimanMA , KorshunovaYet al. Identification of novel high-frequency DNA methylation changes in breast cancer . PLoS ONE2 ( 12 ), e1314 ( 2007 ).
- Ruike Y , ImanakaY , SatoF , ShimizuK , TsujimotoG . Genome-wide analysis of aberrant methylation in human breast cancer cells using methyl-DNA immunoprecipitation combined with high-throughput sequencing . BMC Genomics11 , 137 – 148 ( 2010 ).
- Fang F , TurcanS , RimnerAet al. Breast cancer methylomes establish an epigenomic foundation for metastasis . Sci. Transl. Med.3 ( 75 ), 75ra25 ( 2011 ).
- Sproul D , NestorC , CulleyJet al. Transcriptionally repressed genes become aberrantly methylated and distinguish tumors of different lineages in breast cancer . Proc. Natl Acad. Sci. USA108 ( 11 ), 4364 – 4369 ( 2011 ).
- Sandoval J , EstellerM . Cancer epigenomics: beyond genomics . Curr. Opin. Genet. Dev.22 ( 1 ), 50 – 55 ( 2012 ).
- Locke WJ , ClarkSJ . Epigenome remodelling in breast cancer: insights from an early in vitro model of carcinogenesis . Breast Cancer Res.14 ( 6 ), 215 ( 2012 ).
- Mair B , KubicekS , NijmanSMB . Exploiting epigenetic vulnerabilities for cancer therapeutics . Trends Pharmacol. Sci.35 ( 3 ), 136 – 145 ( 2014 ).
- Schnekenburger M , DicatoM , DiederichM . Epigenetic modulators from “The Big Blue”: a treasure to fight against cancer . Cancer Lett.351 ( 2 ), 182 – 197 ( 2014 ).
- Bird A . DNA methylation patterns and epigenetic memory . Genes Dev.16 ( 1 ), 6 – 21 ( 2002 ).
- Kelemen O , ConvertiniP , ZhangZet al. Function of alternative splicing . Gene514 ( 1 ), 1 – 30 ( 2013 ).
- Tubsuwan A , MunkongdeeT , JearawiriyapaisarnNet al. Molecular analysis of globin gene expression in different thalassaemia disorders: individual variation of β(E) pre-mRNA splicing determine disease severity . Br. J. Haematol.154 ( 5 ), 635 – 643 ( 2011 ).
- Nissim-Rafinia M , KeremB . The splicing machinery is a genetic modifier of disease severity . Trends Genet.21 ( 9 ), 480 – 483 ( 2005 ).
- Leppert S , MatarazzoMR . De novo DNMTs and DNA methylation: novel insights into disease pathogenesis and therapy from epigenomics . Curr. Pharm. Des.20 ( 11 ), 1812 – 1818 ( 2014 ).
- Chédin F . The DNMT3 family of mammalian de novo DNA methyltransferases . Prog. Mol. Biol. Transl. Sci.101 , 255 – 285 ( 2011 ).
- Ding J , WuJ . Epigenetic regulation of hepatic tumor-initiating cells . Front. Biosci. (Landmark Ed.)20 , 946 – 963 ( 2015 ).
- Portela A , EstellerM . Epigenetic modifications and human disease . Nat Biotechnol.28 ( 10 ), 1057 – 1068 ( 2010 ).
- Saito Y , KanaiY , SakamotoM , SaitoH , IshiiH , HirohashiS . Expression of mRNA for DNA methyltransferases and methyl-CpG-binding proteins and DNA methylation status on CpG islands and pericentromeric satellite regions during human hepatocarcinogenesis . Hepatology33 ( 3 ), 561 – 568 ( 2001 ).
- Jin F , DowdySC , XiongY , EberhardtNL , PodratzKC , JiangSW . Up-regulation of DNA methyltransferase 3B expression in endometrial cancers . Gynecol. Oncol.96 ( 2 ), 531 – 538 ( 2005 ).
- Chen WC , ChenMF , LinPY . Significance of DNMT3b in oral cancer . PLoS ONE9 , e89956 ( 2014 ).
- Robertson KD , UzvolgyiE , LiangGet al. The human DNA methyltransferases (DNMTs) 1, 3a and 3b: coordinate mRNA expression in normal tissues and overexpression in tumors . Nucleic Acids Res.27 ( 11 ), 2291 – 2298 ( 1999 ).
- Beaulieu N , MorinS , ChuteIC , RobertMF , NguyenH , MacLeodAR . An essential role for DNA methyltransferase DNMT3B in cancer cell survival . J. Biol. Chem.277 ( 31 ), 28176 – 28181 ( 2002 ).
- Girault I , TozluS , LidereauR , BiècheI . Expression analysis of DNA methyltransferases 1, 3A, and 3B in sporadic breast carcinomas . Clin. Cancer Res.9 ( 12 ), 4415 – 4422 ( 2003 ).
- Issa JP , VertinoPM , WuJet al. Increased cytosine DNA-methyltransferase activity during colon cancer progression . J. Natl Cancer Inst.85 ( 15 ), 1235 – 1240 ( 1993 ).
- Mizuno S , ChijiwaT , OkamuraTet al. Expression of DNA methyltransferases DNMT1, 3A, and 3B in normal hematopoiesis and in acute and chronic myelogenous leukemia . Blood97 ( 5 ), 1172 – 1179 ( 2001 ).
- Eads CA , DanenbergKD , KawakamiK , SaltzLB , DanenbergPV , LairdPW . CpG island hypermethylation in human colorectal tumors is not associated with DNA methyltransferase overexpression . Cancer Res.59 ( 10 ), 2302 – 2306 ( 1999 ).
- Patra SK , PatraA , ZhaoH , DahiyaR . DNA methyltransferase and demethylase in human prostate cancer . Mol. Carcinog.33 ( 3 ), 163 – 171 ( 2002 ).
- Ahluwalia A , HurteauJA , BigsbyRM , NephewKP . DNA methylation in ovarian cancer. II. Expression of DNA methyltransferases in ovarian cancer cell lines and normal ovarian epithelial cells . Gynecol. Oncol.82 ( 2 ), 299 – 304 ( 2001 ).
- Roll JD , RivenbarkAG , JonesWD , ColemanWB . DNMT3b overexpression contributes to a hypermethylator phenotype in human breast cancer cell lines . Mol. Cancer7 , 15 ( 2008 ).
- Aoki A , SuetakeI , MiyagawaJet al. Enzymatic properties of de novo-type mouse DNA (cytosine-5) methyltransferases . Nucleic Acids Res.29 ( 17 ), 3506 – 3512 ( 2001 ).
- Soejima K , FangW , RollinsBJ . DNA methyltransferase 3b contributes to oncogenic transformation induced by SV40T antigen and activated Ras . Oncogene22 ( 30 ), 4723 – 4733 ( 2003 ).
- Weisenberger DJ , VelicescuM , ChengJCet al. Role of the DNA methyltransferase variant DNMT3b3 in DNA methylation . Mol. Cancer Res.2 ( 1 ), 62 – 72 ( 2004 ).
- Kassis ES , ZhaoM , HongJA , ChenGA , NguyenDM , SchrumpDS . Depletion of DNA methyltransferase 1 and/or DNA methyltransferase 3b mediates growth arrest and apoptosis in lung and esophageal cancer and malignant pleural mesothelioma cells . J. Thorac. Cardiovasc. Surg.131 ( 2 ), 298 – 306 ( 2006 ).
- Starlard-Davenport A , KutanziK , TryndyakV , WordB , Lyn-CookB . Restoration of the methylation status of hypermethylated gene promoters by microRNA-29b in human breast cancer: a novel epigenetic therapeutic approach . J. Carcinog.12 , 15 ( 2013 ).
- Yaqinuddin A , QureshiSA , QaziR , AbbasF . Down-regulation of DNMT3b in PC3 cells effects locus-specific DNA methylation, and represses cellular growth and migration . Cancer Cell Int.8 , 13 ( 2008 ).
- Chik F , SzyfM . Effects of specific DNMT gene depletion on cancer cell transformation and breast cancer cell invasion; toward selective DNMT inhibitors . Carcinogenesis32 ( 2 ), 224 – 232 ( 2011 ).
- Kawasaki T , TomitaY , BilimV , TakedaM , TakahashiK , KumanishiT . Abrogation of apoptosis induced by DNA-damaging agents in human bladder-cancer cell lines with p21/WAF1/CIP1 and/or p53 gene alterations . Int. J. Cancer68 ( 4 ), 501 – 505 ( 1996 ).
- Chang BD , XuanY , BroudeEVet al. Role of p53 and p21waf1/cip1 in senescence-like terminal proliferation arrest induced in human tumor cells by chemotherapeutic drugs . Oncogene18 ( 34 ), 4808 – 4818 ( 1999 ).
- Wu Y , StrawnE , BasirZ , HalversonG , GuoSW . Aberrant expression of deoxyribonucleic acid methyltransferases DNMT1, DNMT3A, and DNMT3B in women with endometriosis . Fertil Steril.87 ( 1 ), 24 – 32 ( 2007 ).
- Gordon CA , HartonoSR , ChédinF . Inactive DNMT3B splice variants modulate de novo DNA methylation . PLoS ONE8 ( 7 ), e69486 ( 2013 ).
- St-Laurent Pedneault C , PlourdeK , BélangerSet al. Regulated expression of a FANCL splicing variant as a potential modifier of DNA repair activity . J. Genetic Syndr. Gene Ther.4 , 143 ( 2013 ).
- Bélanger S , PlourdeKV , Joly BeauparlantCet al. Impaired effects of a FANCC splicing isoform in the FANC–BRCA DNA repair pathway . J. Genetic Syndr. Gene Ther.4 , 144 ( 2013 ).
- Joubert BR , HåbergSE , NilsenRMet al. 450K epigenome-wide scan identifies differential DNA methylation in newborns related to maternal smoking during pregnancy . Environ. Health Perspect.120 ( 10 ), 1425 – 31 ( 2012 ).
- Martino D , JooJE , Sexton-OatesAet al. Epigenome-wide association study reveals longitudinally stable DNA methylation differences in CD4+ T cells from children with IgE-mediated food allergy . Epigenetics9 ( 7 ), 998 – 1006 ( 2014 ).
- Huang RC , GarrattES , PanHet al. Genome-wide methylation analysis identifies differentially methylated CpG loci associated with severe obesity in childhood . Epigenetics10 ( 11 ), 995 – 1005 ( 2015 ).
- Anton L , BrownAG , BartolomeiMS , ElovitzMA . Differential methylation of genes associated with cell adhesion in preeclamptic placentas . PLoS ONE9 ( 6 ), e100148 ( 2014 ).
- Han L , ZhengS , SunSet al. Genome-wide methylation profiling in 40 breast cancer cell lines . Lecture Notes in Computer Science6215 , 277 – 284 ( 2010 ).
- Olivero JC . VENNY. An interactive tool for comparing lists with Venn Diagrams . http://bioinfogp.cnb.csic.es/tools/venny/index.html .
- Calvano SE , XiaoW , RichardsDRet al. A network-based analysis of systemic inflammation in humans . Nature437 ( 7061 ), 1032 – 1037 ( 2005 ).
- Ingenuity . www.ingenuity.com/ .
- Litim N , LabrieY , DesjardinsSet al. Polymorphic variations in the FANCA gene in high-risk non-BRCA1/2 breast cancer individuals from the French Canadian population . Mol. Oncol.7 ( 1 ), 85 – 100 ( 2013 ).
- Desjardins S , BelleauP , LabrieYet al. Genetic variants and haplotype analyses of the ZBRK1/ZNF350 gene in high-risk non BRCA1/2 French Canadian breast and ovarian cancer families . Int. J. Cancer122 ( 1 ), 108 – 116 ( 2008 ).
- Ivanga M , LabrieY , CalvoEet al. Temporal analysis of E2 transcriptional induction of PTP and MKP and downregulation of IGF-I pathway key components in the mouse uterus . Physiol. Genomics29 ( 1 ), 13 – 23 ( 2007 ).
- Ivanga M , LabrieY , CalvoEet al. Fine temporal analysis of DHT transcriptional modulation of the ATM/Gadd45g signaling pathways in the mouse uterus . Mol. Reprod. Dev.76 ( 3 ), 278 – 288 ( 2009 ).
- Luu-The V , PaquetN , CalvoE , CumpsJ . Improved real-time RT-PCR method for high-throughput measurements using second derivative calculation and double correction . BioTechniques38 ( 2 ), 287 – 293 ( 2005 ).
- Hagemann S , KuckD , StresemannCet al. Antiproliferative effects of DNA methyltransferase 3B depletion are not associated with DNA demethylation . PLoS ONE7 ( 5 ), e36125 ( 2012 ).
- Robertson KD , UzvolgyiE , LiangGet al. The human DNA methyltransferases (DNMTs) 1, 3a, and 3b: coordinate mRNA expression in normal tissues and overexpression in tumors . Nucleic Acids Res.27 ( 11 ), 2291 – 2298 ( 1999 ).
- Kanai Y , UshijimaS , NakanishiY , HirohashiS . DNA methyltransferase expression and DNA methylation of CpG islands and peri-centromeric satellite regions in human colorectal and stomach cancers . Int. J. Cancer91 ( 2 ), 205 – 212 ( 2001 ).
- Linhart HG , LinH , YamadaYet al. Dnmt3b promotes tumorigenesis in vivo by gene-specific de novo methylation and transcriptional silencing . Genes Dev.21 ( 23 ), 3110 – 3122 ( 2007 ).
- Bonfils C , BeaulieuN , ChanE , Cotton-MontpetitJ , MacLeodAR . Characterization of the human DNA methyltransferase splice variant Dnmt1b . J. Biol. Chem.275 ( 15 ), 10754 – 10760 ( 2000 ).
- Weisenberger DJ , VelicescuM , Preciado-LopezMAet al. Identification and characterization of alternatively spliced variants of DNA methyltransferase 3a in mammalian cells . Gene298 ( 1 ), 91 – 99 ( 2002 ).
- Xie S , WangZ , OkanoMet al. Cloning, expression and chromosome locations of the human DNMT3 gene family . Gene236 ( 1 ), 87 – 95 ( 1999 ).
- Wang J , WalshG , LiuDD , LeeJJ , MaoL . Expression of {Delta}DNMT3B variants and its association with promoter methylation of p16 and RASSF1A in primary non-small cell lung cancer . Cancer Res.66 ( 17 ), 8361 – 8366 ( 2006 ).
- Ostler KR , DavisEM , PayneSLet al. Cancer cells express aberrant DNMT3B transcripts encoding truncated proteins . Oncogene26 ( 38 ), 5553 – 5563 ( 2007 ).
- Gopalakrishnan S , Van EmburghBO , ShanJet al. A novel DNMT3B splice variant expressed in tumor and pluripotent cells modulates genomic DNA methylation patterns and displays altered DNA binding . Mol. Cancer Res.7 ( 10 ), 1622 – 1634 ( 2009 ).
- Okano M , XieS , LiE . Cloning and characterization of a family of novel mammalian DNA (cytosine-5) methyltransferases . Nat. Genet.19 ( 3 ), 219 – 220 ( 1998 ).
- Chen ZX , MannJR , HsiehCL , RiggsAD , ChedinF . Physical and functional interactions between the human DNMT3L protein and members of the de novo methyltransferase family . J. Cell Biochem.95 ( 5 ), 902 – 917 ( 2005 ).
- Chen T , UedaY , DodgeJE , WangZ , LiE . Establishment and maintenance of genomic methylation patterns in mouse embryonic stem cells by Dnmt3a and Dnmt3b . Mol. Cell. Biol.23 ( 16 ), 5594 – 5605 ( 2003 ).
- Wu JY , TangH , HavliogluN . Alternative pre-mRNA splicing and regulation of programmed cell death . Prog. Mol. Subcell. Biol.31 , 153 – 85 ( 2003 ).
- Zhang J , ZhaoD , ParkHKet al. FAVL elevation in human tumors disrupts Fanconi anemia pathway signaling and promotes genomic instability and tumor growth . J. Clin. Invest.120 ( 5 ), 1524 – 1534 ( 2010 ).
- Kern A , HubbardD , AmanoA , Bryant-GreenwoodGD . Cloning, expression, and functional characterization of relaxin receptor (leucine-rich repeat-containing g protein-coupled receptor 7) splice variants from human fetal membranes . Endocrinology149 ( 3 ), 1277 – 1294 ( 2008 ).
- Sanchez C , EscrieutC , ClercPet al. Characterization of a novel five-transmembrane domain cholecystokinin-2 receptor splice variant identified in human tumors . Mol. Cell Endocrinol.349 ( 2 ), 170 – 179 ( 2012 ).
- van der Vaart M , SchaafMJ . Naturally occurring C-terminal splice variants of nuclear receptors . Nucl. Recept. Signal.7 , e007 ( 2009 ).
- Wu JL , LinYS , YangCCet al. MCRS2 represses the transactivation activities of Nrf1 . BMC Cell Biol.10 , 9 ( 2009 ).
- Bienz M . The PHD finger, a nuclear protein-interaction domain . Trends Biochem. Sci.31 ( 1 ), 35 – 40 ( 2006 ).
- Chen T , TsujimotoN , LiE . The PWWP domain of Dnmt3a and Dnmt3b is required for directing DNA methylation to the major satellite repeats at pericentric heterochromatin . Mol. Cell. Biol.24 ( 20 ), 9048 – 9058 ( 2004 ).
- Ge YZ , PuMT , GowherHet al. Chromatin targeting of de novo DNA methyltransferases by the PWWP domain . J. Biol. Chem.279 ( 24 ), 25447 – 25454 ( 2004 ).
- Bachman KE , RountreeMR , BaylinSB . Dnmt3a and Dnmt3b are transcriptional repressors that exhibit unique localization properties to heterochromatin . J. Biol. Chem.276 ( 34 ), 32282 – 32287 ( 2001 ).
- Qiu C , SawadaK , ZhangX , ChengX . The PWWP domain of mammalian DNA methyltransferase Dnmt3b defines a new family of DNA-binding folds . Nat. Struct. Biol.9 ( 3 ), 217 – 224 ( 2002 ).
- Huntriss J , HinkinsM , OliverBet al. Expression of mRNAs for DNA methyltransferases and methyl-CpG-binding proteins in the human female germ line, preimplantation embryos, and embryonic stem cells . Mol. Reprod. Dev.67 ( 3 ), 323 – 336 ( 2004 ).
- Sun Z , AsmannYW , KalariKRet al. Integrated analysis of gene expression, CpG island methylation, and gene copy number in breast cancer cells by deep sequencing . PLoS ONE6 ( 2 ), e17490 ( 2011 ).
- Wilson AS , PowerBE , MolloyPL . DNA hypomethylation and human diseases . Biochim. Biophys. Acta1775 ( 1 ), 138 – 162 ( 2007 ).
- Jia T , LiuYE , LiuJ , ShiYE . Stimulation of breast cancer invasion and metastasis by synuclein gamma . Cancer Res.59 ( 3 ), 742 – 747 ( 1999 ).
- Ehrlich M , JacksonK , WeemaesC . Immunodeficiency, centromeric region instability, facial anomalies syndrome (ICF) . Orphanet J. Rare Dis.1 , 2 ( 2006 ).
- Gowher H , JeltschA . Molecular enzymology of the catalytic domains of the Dnmt3a and Dnmt3b DNA methyltransferases . J. Biol. Chem.277 ( 23 ), 20409 – 20414 ( 2002 ).
- Xie ZH , HuangYN , ChenZX . Mutations in DNA methyltransferase DNMT3B in ICF syndrome affect its regulation by DNMT3L . Hum. Mol. Genet.15 ( 9 ), 1375 – 1385 ( 2006 ).
- Rhee I , JairKW , YenRWet al. CpG methylation is maintained in human cancer cells lacking DNMT1 . Nature404 ( 6781 ), 1003 – 1007 ( 2000 ).
- Rhee I , BachmanKE , ParkBHet al. DNMT1 and DNMT3b cooperate to silence genes in human cancer cells . Nature416 ( 6880 ), 552 – 6 ( 2002 ).
- Baubec T , ColomboDF , WirbelauerCet al. Genomic profiling of DNA methyltransferases reveals a role for DNMT3B in genic methylation . Nature520 ( 7546 ), 243 – 7 ( 2015 ).
- Challen GA , SunD , MayleAet al. Dnmt3a and Dnmt3b have overlapping and distinct functions in hematopoietic stem cells . Cell Stem Cell15 ( 3 ), 350 – 64 ( 2014 ).