Abstract
Aim: Fulminant myocarditis (FM) has neither validated biomarkers nor well-established therapy. Roles of tRNA-derived small RNAs (tsRNAs) in FM remain unknown. Materials & methods: Small RNA sequencing was conducted in plasma from children with FM during acute and convalescent phase and matched healthy volunteers. Data were validated by quantitative real-time PCR in larger sample-sized groups and in vitro. Functional analysis was performed to explore the roles. Results: tiRNA-Gln-TTG-001 was overexpressed in children with FM during acute phase, and the generation and extracellular release of tiRNA-Gln-TTG-001 were higher after myocarditis-mimicked activity in vitro. Several pathways might participate in the pathogenesis of FM. Conclusion: tsRNAs may play an important role in FM, and tiRNA-Gln-TTG-001 might represent a novel and promising biomarker and therapeutic target.
Fulminant myocarditis (FM) is a rare form of sudden and severe myocarditis that often affects previously healthy individuals, especially children and women [Citation1]. It is characterized by a rapid onset of severe hemodynamic collapse, resulting in a high risk of death due to cardiogenic shock and electrical instability [Citation1–3]. The incidence rate of myocarditis was reported to be 19.1 cases per 100,000 patients [Citation4], which may be underestimated as FM was almost exclusively diagnosed at autopsy in the past [Citation1]. A delay in diagnosis and treatment leads to significant morbidity and mortality [Citation5]. Currently, there is still a lack of validated and rapid biomarkers with predictive prognostic value. To make matters worse, treatment options for FM are currently limited to optimal care of arrhythmia, heart failure (HF) and etiology-targeted therapy [Citation6]. So far, there have been no strategies to tailor individualized therapies to improve the outcome of patients with FM [Citation7].
In recent years, extensive efforts have been devoted to the putative functions of tRNA-derived small RNAs (tsRNAs; also called tRNA-derived fragments) in human diseases. tsRNAs, which were first considered to be random cleavage byproducts of tRNA, are a novel class of small noncoding RNAs and produced through cleavage of mature and precursor tRNAs at various positions [Citation8]. A growing body of evidence has shown that tsRNAs play important roles in cellular homeostasis, adaptation of cellular functions to changing environments [Citation8,Citation9] and have a critical modulatory function in human diseases such as inflammation, infection, immunity, metabolism and tumors [Citation10–14]. They have also been identified in plasma [Citation13,Citation15], which indicates that tsRNAs are stable and may directly affect miscellaneous physiological and pathological processes. Therefore, they stand as promising candidates for investigation as biomarkers and therapeutic targets. However, the expression profiles and their potential roles in FM remain elusive.
Here, we assessed the expression profiles of circulating tsRNAs in plasma separated from peripheral blood samples of three children with FM during both the acute and convalescent phases and three age- and sex-matched healthy volunteers by small RNA sequencing (sRNA-seq), and confirmed by quantitative real-time PCR (qRT-PCR) that plasma tiRNA-Gln-TTG-001 (MINTbase ID: tRF-34-V29K9UV36562FQ) was significantly elevated in 12 acute-phase samples compared with 12 convalescent-phase samples, 12 healthy control samples and 12 HF control samples. In vitro studies evidenced that the generation and extracellular release of tiRNA-Gln-TTG-001 were increased after myocarditis-mimicked activity in human cardiomyocytes (HCM). Finally, we analyzed its possible biological functions in the pathogenesis of FM using bioinformatic tools and revealed that the predicted target genes of tiRNA-Gln-TTG-001 are involved in myotube differentiation and metabolism, and Ras, MAPK and PI3K-Akt signaling pathways may exert crucial influence on the initiation and progression of FM. Taken together, this study provides comprehensive expression profiles of tsRNAs in FM for the first time and our data indicated that tsRNAs may play an important role in FM, and tiRNA-Gln-TTG-001 might represent a novel and promising biomarker and therapeutic target.
Materials & methods
Patient demographics & sample collection
Study participants were recruited between September 2018 and September 2020. These included 12 children (3–13.2 years old, three females) with FM during the acute phase, the same 12 children with FM during the convalescent phase, 12 age- and sex-matched healthy children and 12 age- and sex-matched children with dilated cardiomyopathy (DCM).
Patients with FM were clinically diagnosed according to the position statement of the European Society of Cardiology Working Group on myocardial and pericardial diseases [Citation6]. Reconfirmation was carried out when these patients were stable enough to undergo cardiovascular magnetic resonance (CMR) according to the JACC white paper [Citation16]. The inclusion criteria included the following: younger than 18 years; rapid onset of symptoms of acute HF, life-threatening arrhythmias, aborted sudden death, cardiogenic shock or severely impaired left ventricular function within less than 2 weeks in the absence of coronary artery diseases and other known causes; severe hemodynamic compromise requiring high doses of vasopressors (≥5 μg of dopamine or dobutamine per kilogram of bodyweight per minute) or use of mechanical circulatory support such as temporary pacemaker and extracorporeal membrane oxygenation; positive necrosis biomarkers (highly sensitive cardiac troponin T) combined with two of the three CMR criteria for acute myocarditis (edema at the T2-weighted imaging, myocardial early gadolinium enhancement and myocardial late gadolinium enhancement) [Citation16]. Exclusion criteria were as follows: life-threatening conditions not caused by FM; known pre-existing cardiovascular disease or extra-cardiac causes that could explain the syndrome (such as valve disease, congenital heart disease, hyperthyroidism); treated with immunomodulators before the first sample collection; died or forego treatment before clinical stabilization.
Patients that met the criteria were numbered as FM-01 to FM-12 according to patients’ admission time. Peripheral blood samples (3–5 ml) taken from FM-01 to FM-12 just before treatment initiation and upon arrival at the department of pediatric cardiology, Shandong Provincial hospital, were assigned to the acute-phase group (FM-A group) and numbered from FM-A-01 to FM-A-12. A second blood sample was collected from FM-01 to FM-12 when their clinical symptoms disappeared or significantly improved, with decreased levels of hs-cTnT and NT-proBNP and improved levels of LVEF, and could be discharged. These samples were enrolled into the convalescent-phase group (FM-C group) and numbered from FM-C-01 to FM-C-12. Clinical characteristics of these patients are provided in , and the serological and imaging examinations are provided in and . Healthy volunteers were age- and sex-matched children termed as the CON group, and their samples were coded from CON-01 to CON-12. Patients with DCM were age- and sex-matched children enrolled as an HF control group (HF-CON group), and their samples were coded from HF-CON-01 to HF-CON-12. Basic features of these two control groups are provided in and . In addition, the patients who did not undergo CMR but met the other inclusion and the fourth exclusion criteria for FM were numbered as FM-a to FM-e, whose peripheral blood samples (3–5 ml) were also taken just before treatment initiation and upon arrival at the department of pediatric cardiology, Shandong Provincial hospital. Clinical characteristics and the serological examinations of these patients are provided in Supplementary Tables 1 and 2.
Table 1. Clinical characteristics of patients with fulminant myocarditis.
Table 2. Serological and imaging examinations of patients with fulminant myocarditis during acute phase.
Table 3. Serological and imaging examinations of patients with fulminant myocarditis during convalescent phase.
Table 4. Volunteers demographics.
Table 5. Dilated cardiomyopathy patient demographics.
Nine samples (FM-A-01/02/03 vs FM-C-01/02/03 vs CON-01/02/03) were subjected to sRNA-seq, and all 48 samples were used for subsequent qRT-PCR validation. This study was approved by the institutional Ethics Committee of the Shandong Provincial Hospital (NSFC: NO. 2018-115). Informed written consent was obtained from the legal guardians of each participant according to the Declaration of Helsinki principles.
Cell lines & culture conditions
Cell lines for HCM were purchased from Fenghuishengwu Company (Hunan, China) and routinely maintained in Dulbecco’s Modified Eagle’s Medium (DMEM) (HyClone, Beijing, China) supplemented with 10% fetal bovine serum (Biological Industries, CT, USA) in a humidified incubator at 37°C and 5% CO2. Cells were conventionally cultured for 24 h, then, were incubated in a serum-free medium consisting of DMEM as the sole substrate for 12 h and later incubated with 10 μg/ml lipopolysaccharides (LPS). After 24 h, HCM and cell culture supernatant (CCS) were collected.
Pretreatment of clinical specimens & CCS
Blood samples were stored at 4°C and processed within 1 h after venipuncture. They were first centrifuged for 10 min at 1900 × g and 4°C. Then the upper plasma phase was transferred into a 2-ml conical tube and centrifuged for a second time for 10 min at 16,000 × g and 4°C. The clear supernatant was transferred to an empty tube and stored in aliquots at -80°C and used for RNA extraction.
CCS were collected and centrifuged immediately in conical tubes for 15 min at 3000 × g and 4°C. The cleared supernatant was carefully transferred to a new tube and stored in aliquots at -80°C and used for RNA extraction.
RNA extraction
Total RNA of plasma samples subjected to sRNA-seq was extracted using TRIzol (Invitrogen, CA, USA), following the manufacturer’s instructions. The integrity and quantity of each RNA sample were assessed by agarose gel electrophoresis and NanoDrop ND-1000 instrument (Thermo Fisher Scientific, DE, USA).
Total RNA used for qRT-PCR validation was extracted from 200 μl of the pretreated plasma and CCS using miRNeasy Serum/Plasma Kit (Qiagen, Hilden, Germany), according to the manufacturer’s instructions. The concentration and quality of RNA were assessed via absorbance spectrometry on an Agilent 2100 Bioanalyzer (Agilent Technologies Inc., CA, USA).
Small RNA of HCM was extracted using the RNAiso for small RNA (TaKaRa, Dalian, China) according to the manufacturer’s instructions. The concentration and quality of RNA were assessed via NanoDrop™ 2000 (Thermo Fisher Scientific, FL, USA).
Library preparation & sequencing
RNA modifications interfering with the library construction were removed from total RNA samples by pretreatment with rtStar™ tRF tiRNA Pretreatment Kit (Arraystar Inc., MD, USA) before the library preparation as follows: 3′-aminoacyl (charged) deacylation, 3′-cP (2′,3′-cyclic phosphate) removal, 5′-OH (hydroxyl group) phosphorylation and m1A and m3C demethylation for efficient reverse transcription. cDNA was then synthesized and amplified using Illumina’s proprietary RT and amplification primers (Illumina, DE, USA). Subsequently, approximately 134–160 base pair (bp) PCR-amplified fragments (corresponding to 14–40 nt small RNA size range) were extracted and purified from the PAGE gel. The libraries were qualified and quantified using Agilent BioAnalyzer 2100 and were then denatured and diluted to a loading volume of 1.3 ml and concentration of 1.8 pM. Diluted libraries were loaded onto the reagent cartridge and forwarded to the sequencing run on an Illumina NextSeq 500 system (Illumina, DE, USA) using the NextSeq 500/550 V2 kit (Illumina), following the manufacturer’s instructions. The custom tRNA library used to quantify reads aligning to tRNAs was summarized in Supplementary Table 3. The sequence data have been submitted to the GEO database (accession no.: GEO:GSE163944).
Sequencing data analysis
Image analysis and base calling were performed using the Solexa pipeline v1.8 (Off-Line Base Caller software, v1.8). Sequencing quality was examined by FastQC and trimmed reads (pass Illumina quality filter, trimmed 5′,3′-adaptor bases by ‘cutadapt’) were aligned allowing for only one mismatch with the mature tRNA sequences. Readings that were not mapping were aligned allowing for only one mismatch with the precursor tRNA sequences using the ‘bowtie’ software. The abundance of tsRNAs was evaluated using their sequencing counts and normalized as counts per million (CPM) of total aligned reads (CPM <20 is the abundance thresholding criterion). The tsRNAs that were differentially expressed were screened based on the count value with the R package edgeR. Hierarchical cluster analysis, principal component analysis and correlation analysis were performed in R or Perl environment for statistical computing, and graphics of the expressed tsRNAs were drawn to perform a visual identification.
qRT-PCR validation
To validate the changes of tsRNAs detected by sRNA-seq, all samples were assessed by qRT-PCR on a LightCycler480 system (Roche, Basel, Switzerland). No existing assays were available to quantify tsRNAs, therefore, we designed small RNA TaqMan assays as described previously [Citation13]. These assays adopted standard qRT-PCR techniques to quantify small RNA fragments specifically, rapidly and sensitively. As these assays required sequences of at least 17 bases in length, the downregulated tRF-Gln-CTG-011 with 14 bases had to be excluded from this investigation. First, reverse transcription was performed to convert RNA to cDNA using a Taqman miRNA cDNA synthesis Kit (Serum/Plasma; Haigene, Ha’erbin, China) for plasma and CCS, and a Taqman miRNA cDNA synthesis Kit (Haigene, Ha’erbin, China) for HCM. Then, cDNA was quantified by qRT-PCR with the Taqman qRT-PCR mix (Haigene, Ha’erbin, China) as instructed by the manufacturer. qRT-PCR was performed in 20 μl reaction volume, which included 2 μl cDNA (cDNA from HCM should be diluted ten-times), 4 μl 5 × golden HS Taqman qRT-PCR mix, 1 μl 20 × miRNA Taqman assay and 13 μl double-distilled water. The reaction was pre-denatured at 95°C for 15 min, followed by 45 amplification cycles at 95°C for 10 s and 60°C for 60 s. For plasma and CCS samples, 3 μl of cel-miR-39-3p (20 fmol/μl; Ribobio, Guangzhou, China) was used as a spike-in control for normalization to obtain relative tsRNA expression, while for HCM, U6 was used. The relative expression levels were determined by the 2-ΔCT & 2-ΔΔCt method and the sequential tiRNA-Gln-TTG-001 levels expressed as 2-ΔCT in each group were provided in Supplementary Table 4.
Bioinformatic analysis
Databases Diana Tools (http://diana.imis.athena-innovation.gr/DianaTools/index.php), TargetScan (www.targetscan.org/vert_72/) and Miranda (www.microrna.org/microrna/) were used to predict target genes of the differently expressed tsRNAs. Gene Ontology (GO) (www.geneontology.org) was used to determine the possible biological functions of genes with regard to their biological process, cellular component and molecular function. Kyoto Encyclopedia of Genes and Genomes (KEGG) database (www.kegg.jp/) was used to analyze signaling pathways.
Statistical analysis
Paired-samples t-test, independent-samples t-test, Kruskal–Wallis H-test were used for group differences, and the rank correlation test was conducted to evaluate the relationships between expression levels of candidate tsRNAs (expressed as 2-ΔCT) and the serological or imaging parameters of FM during acute phase using R programming language (version 3.5.3). A p-value <0.05 was considered statistically significant.
Results
Expression profiles of plasma tsRNAs in FM-A, FM-C & CON groups
To identify the expression profiles of tsRNAs in the plasma of patients with FM and explore whether the levels of differentially expressed tsRNAs were associated with the initiation and progression of FM, we detected the expression profiles of plasma tsRNAs by sRNA-seq in FM-A group (FM-A-01/02/03), FM-C group (FM-C-01/02/03) and CON group (CON-01/02/03). The variation of plasma tsRNAs expression among every sample is shown by heatmaps (), and the variation between every two groups is shown by heatmaps, volcano and scatterplots to provide a quick visualization (A–I). The CPM of tiRNA-Gln-TTG-001 and tRF-Gln-CTG-011 were, respectively, the highest and lowest in the FM-A group, while the FM-C group had values similar to those of the CON group (Supplementary Table 5). Under the condition of FC ≥1.5, a total of 557 tsRNAs were differentially expressed in FM-A and CON groups, of which 257 tsRNAs were upregulated and 300 were downregulated, with150 tsRNAs excluded (A); 535 tsRNAs were differentially expressed in paired FM-A and FM-C groups, of which 230 tsRNAs were upregulated and 305 were downregulated, with 157 tsRNAs excluded (B); 536 tsRNAs were differentially expressed in paired FM-C and CON groups, of which 212 tsRNAs were upregulated and 324 were downregulated, and 175 tsRNAs showed no significant trends (C). Furthermore, under the condition of FC ≥1.5 and p < 0.05, a total of 13 tsRNAs were differentially expressed in FM-A and CON groups, of which 11 tsRNAs were upregulated and two were downregulated (D & G); seven tsRNAs were differentially expressed in paired FM-A and FM-C groups, of which two tsRNAs were upregulated and five were downregulated (E & H); 703 tsRNAs showed no significant trends in paired FM-C and CON groups (F & I). The counts and length of each unique read in nine samples are shown in the bar chart ().
Hierarchical clustering heatmap analysis of tsRNAs expression data of nine samples. Each row represents one tsRNA and each column represents one sample.
CON: Control; FM: Fulminant myocarditis.
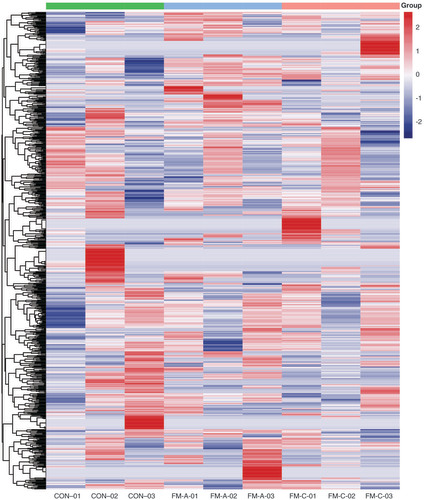
The scatter plot of tsRNAs in three pairwise that is FM-A versus CON (A), FM-A versus FM-C (B) and FM-C versus CON (C). The values of x- and y-axes in the scatter plot are the averaged CPM of each group (log2 scaled). Red/green dots indicate statistically significant differentially expressed tsRNAs with FC ≥1.5. Gray dots indicate nondifferentially expressed tsRNAs. Heatmaps show the hierarchical cluster analysis of differentially expressed tsRNAs in three pairwise between the three groups: FM-A versus CON (D), FM-A versus FM-C (E) and FM-C versus CON (F). Each row represents one tsRNAs and each column represents one sample. Color brightness reflects the degree of expression increase or decrease: red represents high expression, and blue represents low expression. The volcano plot of tsRNAs in three pairwise between the three groups: FM-A versus CON (G), FM-A versus FM-C (H) and FM-C versus CON (I). The values of x- and y-axes in the volcano plot are log2 transformed fold change and -log10 transformed p-values between the two groups, respectively. Red/green circles indicate statistically significant differentially expressed tsRNAs with FC ≥1.5 and p < 0.05. Gray circles indicate nondifferentially expressed tsRNAs.
CON: Control; FM: Fulminant myocarditis.
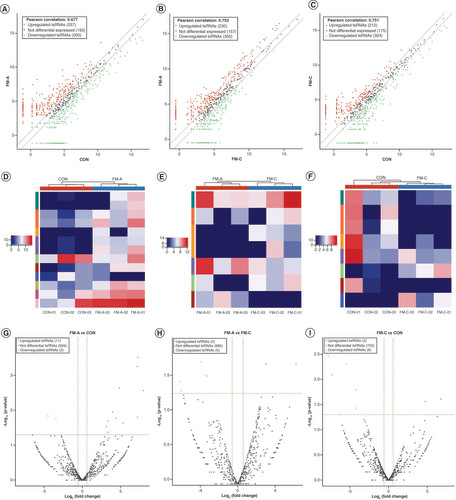
Bar chart shows the total read counts against the lengths for the complete adapter-trimmed read set in the nine samples from FM-A, FM-C and CON groups, respectively. (A) FM-A-01, (B) FM-A-02, (C) FM-A-03, (D) FM-C-01, (E) FM-C-02, (F) FM-C-03, (G) CON-01, (H) CON-02, (I) CON-03.
FM: Fulminant myocarditis.
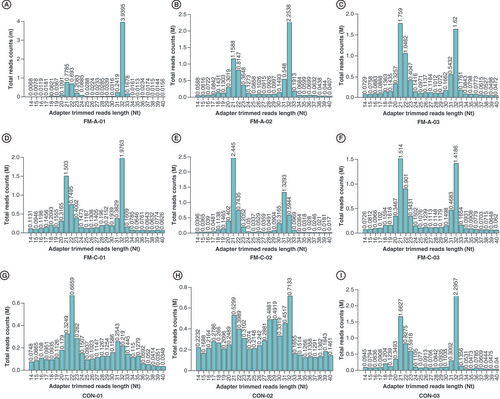
Validation for the differential expression level of plasma tsRNAs
To further validate these sRNA-seq data, qRT-PCR was performed and differentially expressed tsRNAs between FM-A and FM-C groups (FC ≥1.5, p < 0.05) were selected. Five of these were downregulated: tRF-Glu-TTC-003, tRF-Glu-TTC-004, tRF-Lys-TTT-006, tRF-Gln-CTG-011 and tRF-Leu-AAG-017; while two were upregulated: tiRNA-Gln-TTG-001 and tRF-Pro-TGG-005. Unfortunately, the downregulated tRF-Gln-CTG-011 was excluded from the investigation in the process of qRT-PCR validation, because small RNA TaqMan assays require a minimum sequence of at least 17 bases, and the sequence of tRF-Gln-CTG-011 is only 14 bases. TRF-Glu-TTC-003 and tRF-Pro-TGG-005 were excluded from further analysis, because their plasma concentrations were too low to be detected. No significant differences were found for tRF-Glu-TTC-004 (p = 0.32, A), tRF-Lys-TTT-006 (p = 0.33, B) and tRF-Leu-AAG-017 (p = 0.15, C) between the FM-A and FM-C groups; therefore, these tsRNAs were then excluded from further analyses. Only tiRNA-Gln-TTG-001 was found to be overexpressed in the FM-A group with a 2.72-fold increase when compared with FM-C (p = 0.00052, D). In line with the sRNA-seq data, tiRNA-Gln-TTG-001 was found to be overexpressed in FM-A group, with a 2.29-fold increase when compared with FM-C group and a 3.49-fold increase when compared with CON group (p = 0.0000048434, 0.00000053493, respectively; ). There was no statistical difference of tiRNA-Gln-TTG-001 between FM-C and CON groups (p = 0.7335; ). Since all patients in FM-A group also presented with the symptoms of HF, we further compared the expression levels of plasma tiRNA-Gln-TTG-001 in patients with DCM who also presented with HF to evaluate whether the differently expressed tsRNAs were specific responsible for the onset of FM. tiRNA-Gln-TTG-001 was found to be overexpressed in FM-A group, with a 5.38-fold increase when compared with HF-CON group (p = 0.000000019589; ). There was no statistical difference of tiRNA-Gln-TTG-001 between HF-CON and CON groups (p = 0.234; ). It is worth mentioning that the levels of tiRNA-Gln-TTG-001 were downregulated, instead of upregulated, in FM-a/b/c who died within 24 h of admission (data not shown, as the sample size was too small). As shown in , levels of tiRNA-Gln-TTG-001 were positively associated with the values of hs-cTnT (r = 0.7622, p = 0.0059), C-reactive protein (CRP; r = 0.6725, p = 0.0166) and procalcitonin (PCT; r = 0.669, p = 0.0174) and negatively associated with the values of neutrophil proportion (N%; r = -0.6434, p = 0.028). The correlation coefficient between levels of tiRNA-Gln-TTG-001 and NT-proBNP (r = 0.1611, p = 0.6169)/white blood cell counts (WBC; r = 0.2028, p = 0.5281)/erythrocyte sedimentation rate (ESR; r = 0.0286, p = 1)/LVEF (r = -0.0701, p = 0.8287)/early gadolinium enhancement ratio (EGEr; r = 0.3579, p = 0.2534) was not statistically significant.
(A) No significant difference was found in tRF-Glu-TTC-004 between the FM-A and FM-C groups (p = 0.32). (B) No significant difference was found in tRF-Lys-TTT-006 between the FM-A and FM-C groups (p = 0.33). (C) No significant difference was found in tRF-Leu-AAG-017 between the FM-A and FM-C groups (p = 0.15). (D) tiRNA-Gln-TTG-001 was 2.72-fold change upregulated in FM-A compared with FM-C (p = 0.00052).
FM: Fulminant myocarditis.
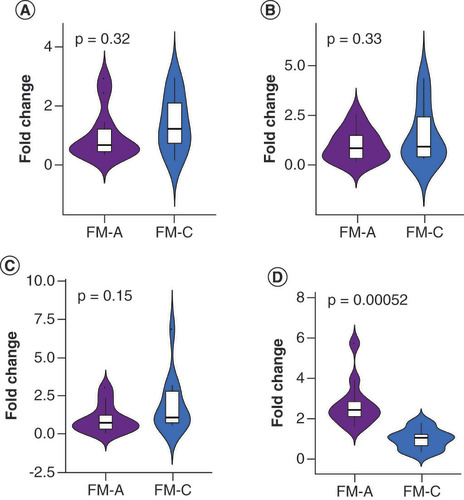
tiRNA-Gln-TTG-001 was found to be overexpressed in FM-A group, with a 2.29-fold increase when compared with FM-C group (p = 0.0000048434), and a 3.49-fold increase when compared with CON group (p = 0.00000053493). There was no statistical difference of tiRNA-Gln-TTG-001 between FM-C and CON groups (p = 0.7335).
CON: Control; FM: Fulminant myocarditis.
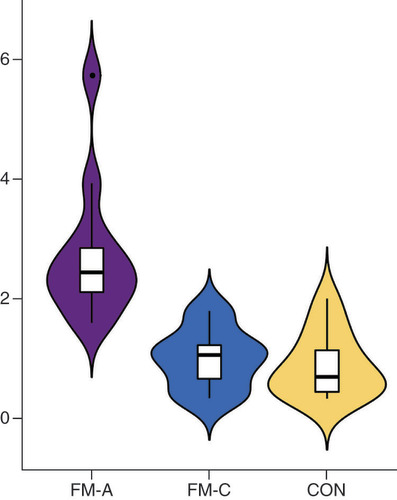
tiRNA-Gln-TTG-001 was found to be overexpressed in FM-A group, with a 5.38-fold increase when compared with HF-CON group (p = 0.000000019589). There was no statistical difference of tiRNA-Gln-TTG-001 between HF-CON and CON groups (p = 0.234).
CON: Control; FM: Fulminant myocarditis.
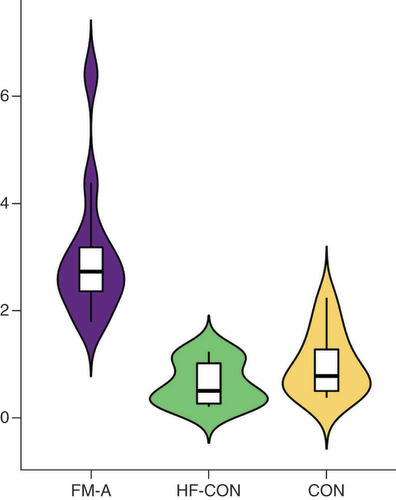
(A) Expression levels of tiRNA-Gln-TTG-001 were positively associated with the values of hs-cTnT (r = 0.7622, p = 0.0059). (B) Expression levels of tiRNA-Gln-TTG-001 were positively associated with the values of CRP (r = 0.6725, p = 0.0166). (C) Expression levels of tiRNA-Gln-TTG-001 were positively associated with the values of PCT (r = 0.669, p = 0.0174). (D) Expression levels of tiRNA-Gln-TTG-001 were negatively associated with the values of N% (r = -0.6434, p = 0.028). (E) The correlation coefficient between levels of tiRNA-Gln-TTG-001 and NT-proBNP (r = 0.1611, p = 0.6169) was not statistically significant. (F) The correlation coefficient between levels of tiRNA-Gln-TTG-001 and WBC counts (r = 0.2028, p = 0.5281) was not statistically significant. (G) The correlation coefficient between levels of tiRNA-Gln-TTG-001 and ESR (r = 0.0286, p = 1) was not statistically significant. (H) The correlation coefficient between levels of tiRNA-Gln-TTG-001 and LVEF (r = -0.0701, p = 0.8287) was not statistically significant. (I) The correlation coefficient between levels of tiRNA-Gln-TTG-001 and EGEr (r = 0.3579, p = 0.2534) was not statistically significant.
EGEr: Early gadolinium enhancement ratio = enhancement myocardium/enhancement skeletal muscle; ESR: Erythrocyte sedimentation rate; FM: Fulminant myocarditis; hs-cTnT: Highly sensitive cardiac troponin T; LVEF: Left ventricular ejection fraction; N%: Neutrophil proportion; NT-proBNP: N-terminal pro-brain natriuretic peptide; PCT: Procalcitonin; WBC: White blood cell.
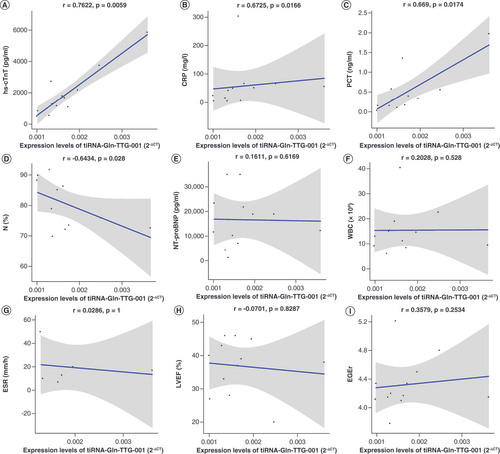
Validation for the production & extracellular release of tsRNAs in vitro
To experimentally model the observation that production and extracellular release of tsRNAs increased after myocarditis-mimicked activity in HCM, we collected HCM and CCS and performed qRT-PCR to test for expression changes. Intracellular tiRNA-Gln-TTG-001 levels were significantly increased following exposure to LPS (p = 0.0029, A). Since tsRNAs may be secreted from HCM, we next quantified tsRNAs in CCS, and found that tiRNA-Gln-TTG-001 was significantly increased following LPS induction (p = 0.0056, B).
(A) Intracellular tiRNA-Gln-TTG-001 levels were significantly increased following exposure (p = 0.0029). (B) tiRNA-Gln-TTG-001 were significantly increased in the CCS, following exposure (p = 0.0056).
CCS: Cell culture supernatant; HCM: Human cardiomyocyte; LPS: Lipopolysaccharide.
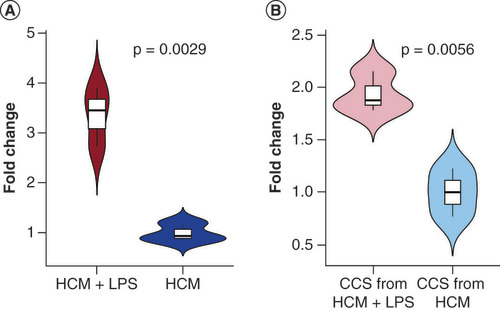
GO enrichment analysis & KEGG pathway analysis
To explore the functions of tiRNA-Gln-TTG-001 and analyze its possible biological functions in the pathogenesis of FM, Diana Tools, Target Scan and Miranda algorithms were used to predict target genes. Putative genes are displayed in . Then, GO enrichment analysis was performed. The potential target genes of tiRNA-Gln-TTG-001 were found to be related to myotube differentiation and metabolism (). KEGG pathway analysis showed that 17 pathways may be involved and the ten most significant pathways were displayed (). It was speculated that the top Ras, MAPK, PI3K-Akt signaling pathways may exert crucial influence on the pathogenesis and progression of FM.
GO: Gene ontology.
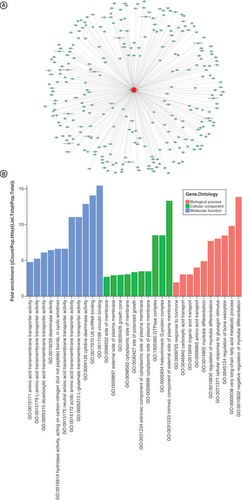
The vertical axis shows the annotated functions of the target genes. The horizontal axis shows the enrichment score (-log10 transformed p-value) and the gene number of each cluster, respectively. Yellow marked nodes are putative molecule in relation to the dysregulated tsRNAs in fulminant myocarditis.
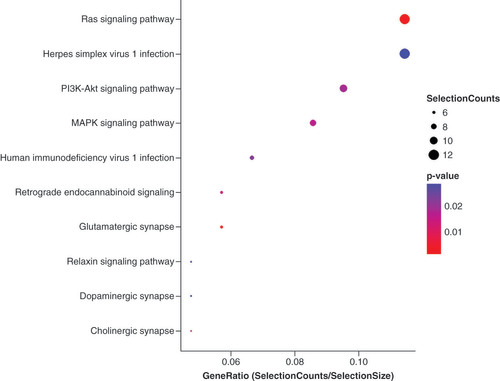
Discussion
Myocarditis is a polymorphic disease further complicated with indeterminate etiology and pathogenesis. Despite its undetermined etiology, myocarditis can be of infectious or noninfectious origins. Pathogens, immunological factors, toxins, hormones, physical agents and miscellaneous factors like animal bites can cause myocarditis [Citation6]. The pathogenesis mainly involves viral and autoimmune mechanisms, with or without a genetic predisposition [Citation6,Citation17–20]. FM is a rare, distinct form of myocarditis that has a difficult diagnosis and treatment scheme. The current diagnostic gold standard is the endomyocardial biopsy (EMB), which gives a histological, immunological and molecular characterization of the disease, and there is consensus that EMB should be performed in all clinically suspected acute myocarditis [Citation1,Citation6]. Nevertheless, EMB is invasive, and the delay in obtaining results as well as the high false-negative rates limit its use in the acute phase of FM [Citation5]. Only 3.4, 8 and 12.6% of patients with acute myocarditis performed EMB, respectively, in Japan [Citation21], the United States [Citation22] and Italy [Citation23]. In recent years, CMR has become the key noninvasive assessment tool to identify patients with suspected myocarditis as it offers a unique combination of safety, clarity of anatomical visualization, interobserver consistency and quantitative accuracy [Citation16]. And if two of the three CMR criteria for acute myocarditis (which include edema at the T2-weighted imaging, myocardial early gadolinium enhancement and myocardial late gadolinium enhancement) are positive, myocarditis can be predicted with a diagnostic accuracy of 78% [Citation16]. Therefore, in this study, we first made the clinical diagnosis of FM mainly based on the clinical syndrome and positive necrosis biomarkers, and then, we reconfirmed the diagnosis with the aid of CMR. However, performing CMR is often not feasible in the acute phase of FM and is frequently delayed up to patient’s stabilization [Citation24]. Currently, there is still a lack of validated and rapid biomarkers.
Recent developments in the field of noncoding RNAs in myocarditis have advanced our knowledge and show great promise as reliable candidate biomarkers. Indeed, several studies have reported dysregulated miRNAs in myocarditis as promising diagnostic markers and therapeutic targets summarized in the review [Citation25]. For example, the levels of plasma miR-208b and miR-499-5p from patients with acute myocarditis were positively correlated with disease severity [Citation26]. In another study, miR-21 was found to be upregulated in patients with acute viral myocarditis and mice with myocarditis induced by coxsackievirus B3 (CVB3) [Citation27,Citation28]. Consistent with this, intravenous injection of miR-21 attenuated cardiac inflammation and myocardial damage in a murine model of viral myocarditis [Citation29]. However, the results from different observers have been conflicting, and the related studies have rather low reproducibility [Citation25]. In recent years, the advent of tsRNAs, which have been shown to play key regulatory roles similar to miRNAs, holds much promise for new diagnostic and therapeutic strategies. However, the expression profiles and functions of tsRNAs in FM remain elusive.
In this study, we first hypothesized that tsRNAs are involved in FM, and selected circulating blood-based tsRNAs for further analysis based on the fact that blood samples are easy and fast to collect and it would also be feasible to develop agonist/antagonist agents to modulate disease progression. Moreover, we used strict inclusion and exclusion criteria to ensure that patients treated with immunomodulators before the first sample collection were excluded with the goal of minimizing any impact from therapeutic interventions.
Then, we set out to detect and compare the expression profiles of plasma tsRNAs by sRNA-seq in FM-A and CON groups in order to identify the expression profiles of tsRNAs in the plasma of patients with FM and explore whether the levels of differentially expressed tsRNAs were associated with the initiation of FM. Our results showed that a total of 557 tsRNAs were differentially expressed and 13 tsRNAs were significantly differentially expressed, suggesting that they may serve as biomarkers in patients with FM. To further explore whether the levels of differentially expressed tsRNAs were associated with the progression of FM and could be served as potential biomarkers for early detection of FM, we also detected and compared the expression profiles of plasma tsRNAs by sRNA-seq in FM-A and FM-C groups, and the results showed that a total of 535 tsRNAs were differentially expressed and seven tsRNAs were significantly differentially expressed, suggesting that they may serve as biomarkers for early detection of FM and associated with the progression of FM or therapeutic interventions. As the differentially expressed tsRNAs between FM-A and FM-C groups may result from therapeutic interventions, we detected and compared the expression profile of plasma tsRNAs in FM-C and CON groups, and revealed that 703 tsRNAs showed no significant trends suggesting that these tsRNAs may not be affected by therapy. These results combined hint at the idea that two specific tsRNAs, tiRNA-Gln-TTG-001 and tRF-Gln-CTG-011, which were significantly changed in FM-A group compared with CON and FM-C group, may serve as potential biomarkers indicative of the initiation and progression of FM without being affected by therapeutic interventions.
Next, we proceeded to perform qRT-PCR with the specially designed small RNA TaqMan assays as described previously to further validate these sRNA-seq data [Citation13,Citation15]. Since our volunteers were children, we minimized the volume of collected blood samples. Therefore, we first performed qRT-PCR in FM-A and FM-C samples to exclude false-positive tsRNAs, and then, we performed qRT-PCR with the samples of FM-A, FM-C and CON groups to validate our candidate tsRNAs by the direct comparisons of the three groups. We also compared the expression levels of the candidate tsRNAs in patients with DCM (as HF controls) to evaluate whether they were specific responsible for the onset of FM. The results of the present study demonstrate that plasma tsRNAs are quantifiable using standard laboratory-based PCR techniques and differentially expressed between patients with FM and healthy volunteers. Plasma tiRNA-Gln-TTG-001 could be detected in patients with FM, and was a reliable marker to differentiate patients with FM in the acute phase from those in the convalescent phase, and form healthy and HF controls. These results suggest that plasma tiRNA-Gln-TTG-001 could be of use as an early marker for FM and the change of its levels may be associated with the initiation and progression of this disease. Furthermore, the evaluation of the relationships between expression levels of candidate tsRNAs and the serological or imaging parameters of FM during acute phase showed that expression levels of tiRNA-Gln-TTG-001 was associated with the values of hs-cTnT and some inflammation markers, while no correlation was observed between levels of tiRNA-Gln-TTG-001 and NT-proBNP. The reasons might be as follows: tiRNA-Gln-TTG-001 may be sensitive of myocyte injury, like cardiac troponins, and involved in the initiation and progression of FM, while NT-proBNP is released predominantly from the ventricular myocardium in response to increased ventricular wall stress; the half-time might be different: peripheral blood samples taken from patients with FM just before treatment initiation and upon arrival at the hospital, but the disease severity might be different among the patients, let alone individual differences.
Having demonstrated that tiRNA-Gln-TTG-001 is elevated in the plasma of patients with FM during acute phase, we sought to determine whether tiRNA-Gln-TTG-001 was detectable in cardiomyocyte. Fortunately, our study verified that tiRNA-Gln-TTG-001 could be detected in HCM and its levels increased significantly following induction of LPS (mimicking myocarditis). As tiRNA-Gln-TTG-001 may be secreted from cardiomyocyte, we next quantified its levels in the CCS, and found that tiRNA-Gln-TTG-001 could be detected in the CCS and its levels increased significantly following induction of LPS. This confirmed that tiRNA-Gln-TTG-001 was expressed and could be released from cardiomyocytes, and the production and extracellular release of tiRNA-Gln-TTG-001 increased after myocarditis-mimicked activity. It should be noted that this in vitro observation was limited to the effects of LPS on HCM and could not confirm that cardiomyocytes are the only source of tiRNA-Gln-TTG-001. Heart tissue contains many types of cells such as cardiomyocytes and immune cells, all of which may be involved in the pathogenesis of FM and need to be studied. Other organs and tissues besides the heart, such as the spleen, lymph nodes, liver and brain should also be investigated. Therefore, this in vitro observation will be further verified in vivo in a project currently underway in our laboratory.
In addition, we also observed that the expression levels of tiRNA-Gln-TTG-001 from the three patients who died during acute phase were downregulated, instead of upregulated, compared with healthy volunteers. This might suggest that a threshold exists above which may be associated with the severity of FM, while below which may suggest poor prognosis, although larger studies are needed to confirm this. tRF-Gln-CTG-011 might also be biologically significant. Unfortunately, it had to be excluded from the investigation in the process of qRT-PCR validation, because it requires a minimum sequence of at least 17 bases to design a TaqMan probe, and the sequence of tRF-Gln-CTG-011 is only 14 bases.
tRNA-derived stress-induced RNAs (tiRNAs; also called tRNA halves) are important components of tsRNAs and mounting evidence has demonstrated their critical functional roles as regulatory factors in the pathophysiological processes of various diseases. tiRNAs are generated when mature tRNA is specifically cleaved under cellular stress [Citation30], and when they are ejected into the circulation, they constitute part of circulating tsRNAs. The underlying stress that precipitates tRNA cleavage and sources of such circulating tsRNAs in our study is unknown. As tRNA cleavage has been identified in response to processes such as inflammation, infection, ischemia and autophagy [Citation10–12,Citation30,Citation31], one could speculate that these processes might contribute to the observed tRNA cleavage in our study. Known as miRNAs in disguise, tsRNAs share many features with miRNAs, one could speculate that plasma tiRNA-Gln-TTG-001 might exert intracellular functions based on the similar principles like miRNAs. Previous studies have proven that circulating miRNAs can be released in an active (secretion) or passive (membrane leaking) manner from various tissues/organs into the body fluids following a stressful stimulus [Citation32,Citation33], and are taken up by the recipient cells. After they are internalized in the cells, these circulating miRNAs can bind to their targets and execute their biological functions like any other intracellular miRNA [Citation34].
Based on all above findings, we further employed bioinformatic analyses and KEGG pathway analysis to gather targeted genes of tiRNA-Gln-TTG-001 and explore possible signaling pathways, respectively. The potential target genes of tiRNA-Gln-TTG-001 were found to be related to myotube differentiation and metabolism, and it was speculated that the top Ras, MAPK, PI3K-Akt signaling pathways may exert crucial influence on the pathogenesis and progression of FM. The above signaling pathways participate in various processes, such as inflammation, immunity, cell proliferation, differentiation, apoptosis and autophagy [Citation35–37]. Indeed, our previous study indicated that the inflammation of experimental autoimmune myocarditis could be ameliorated by CD40siRNA, possibly by inhibiting the autophagy of cardiomyocytes [Citation38]. In agreement with our findings, Gu et al. observed that CVB3-induced myocarditis could be alleviated via activating the autophagy flux pathway to attenuate cardiomyocyte apoptosis [Citation39]. However, final proof requires further functional validation studies. Even though our study is still preliminary, the feasibility of tiRNA-Gln-TTG-001 as a novel biomarker and therapeutic target for FM should be considered.
Conclusion
This study provides comprehensive expression profiles of circulating tsRNAs in FM for the first time. Plasma tiRNA-Gln-TTG-001 was confirmed to a reliable marker to differentiate patients with FM in the acute phase from those in the convalescent phase, healthy controls and HF controls, and may be involved in the initiation and progression of FM. The potential target genes of tiRNA-Gln-TTG-001 were found to be related to myotube differentiation and metabolism, and Ras, MAPK, PI3K-Akt signaling pathways may exert crucial influence on the pathogenesis and progression of FM. However, the source of tiRNA-Gln-TTG-001 needs further investigation and the findings of this study need to be confirmed in vivo. Multicenter studies with larger sample sizes should be performed. Furthermore, the relationship between tiRNA-Gln-TTG-001 and Ras, MAPK and PI3K-Akt signaling pathways may be crucial for the development of FM and should therefore be studied in the future. Despite these limitations, our results highlight the significance of tsRNAs in FM and may provide new insights into the roles of tsRNAs in FM.
Future perspective
FM is not readily distinguishable and the treatment is mainly supportive and empirical. Evidences have mounted that tsRNAs play important roles in various diseases, but the potential roles in FM remain unknown. The present study demonstrated the overview profiles of differential tsRNAs in FM and provides a new basis for roles of tsRNAs in FM. However, multicenter studies with larger sample sizes should be performed, and the exact mechanisms remain to be delineated.
Fulminant myocarditis (FM) is a rare form of sudden and severe myocarditis that often affects a previously healthy individual, and is not readily distinguishable with mainly supportive and empirical treatment.
tRNA-derived small RNAs (tsRNAs) are a novel class of small noncoding RNAs produced by enzymatic cleavage of tRNA and play important roles in various diseases.
For the first time, this work provided comprehensive expression profiles of differentially expressed tsRNAs in children with FM.
Plasma tiRNA-Gln-TTG-001 was found to be overexpressed in FM-A group, with a 2.29-fold increase when compared with FM-C group, a 3.49-fold increase when compared with CON group and a 5.38-fold increase when compared with HF-CON group.
Expression levels of tiRNA-Gln-TTG-001 were positively associated with the values of highly sensitive cardiac troponin T, C-reactive protein and procalcitonin, and negatively associated with the values of neutrophil proportion.
In vitro studies confirmed that generation and extracellular release of tiRNA-Gln-TTG-001 were higher after myocarditis-mimicked activity in human cardiomyocytes.
Bioinformatics analysis revealed that the predicted target genes of tiRNA-Gln-TTG-001 are involved in myotube differentiation and metabolism, and Ras, MAPK and PI3K-Akt signaling pathways may exert crucial influence on the initiation and progression of FM.
Author contributions
Study design was done by J Wang and B Han. Sample collection and clinical evaluation were done by J Wang, B Han, Y Yi, Y Wang, H Jia, J Lv, X Yang, D Jiang and J Zhang. Performing and analyzing experiments were done by J Wang, Y Yi, Y Wang, L Zhang and H Jia. Writing manuscript was done by J Wang.
Ethical conduct of research
The authors state that they have obtained appropriate institutional review board approval (NSFC: NO. 2018-115) and have followed the principles outlined in the Declaration of Helsinki for all human investigations. In addition, for investigations involving human subjects, informed written consent has been obtained from the legal guardians of each participant.
Acknowledgments
The authors thank the patients and volunteers that participated in this study.
Supplementary data
To view the supplementary data that accompany this paper please visit the journal website at: www.tandfonline.com/doi/suppl/10.2217/epi-2021-0109
Financial & competing interests disclosure
This work was supported by grants from the National Natural Science Foundation of China (grant no.: 81873498), the Natural Science Foundation of Shandong Province, China (grant no.: ZR2019MH015) and Special Expert of Taishan Scholars (grant no.: ts201511099). The authors have no other relevant affiliations or financial involvement with any organization or entity with a financial interest in or financial conflict with the subject matter or materials discussed in the manuscript apart from those disclosed.
No writing assistance was utilized in the production of this manuscript.
Data sharing statement
The datasets used and/or analyzed in the current study are available from the corresponding author on reasonable request. And the high-throughput sequence data have been submitted to GEO with GEO accession numbers: GSE 163944.
References
- Kociol RD , CooperLT , FangJCet al. Recognition and initial management of fulminant myocarditis: a scientific statement from the American Heart Association. Circulation141(6), e69–e92 (2020).
- Sawamura A , OkumuraT , ItoMet al. Prognostic value of electrocardiography in patients with fulminant myocarditis supported by percutaneous venoarterial extracorporeal membrane oxygenation-analysis from the CHANGE PUMP Study. Circ. J.82(8), 2089–2095 (2018).
- Moslehi JJ , BrinkleyDM , MeijersWC. Fulminant myocarditis: evolving diagnosis, evolving biology, evolving prognosis. J. Am. Coll. Cardiol.74(3), 312–314 (2019).
- Hay SI , AbajobirAA , AbateKHet al. Global, regional, and national disability-adjusted life-years (DALYs) for 333 diseases and injuries and healthy life expectancy (HALE) for 195 countries and territories, 1990–2016: a systematic analysis for the Global Burden of Disease Study 2016. Lancet390(10100), 1260–1344 (2017).
- Wang S , WeiX , HuH. STAR evidence evaluation of viral fulminant myocarditis: specificity, timeliness, accessibility, risk. Eur. Heart J.41(34), 3281–3282 (2020).
- Caforio AL , PankuweitS , ArbustiniEet al. Current state of knowledge on aetiology, diagnosis, management, and therapy of myocarditis: a position statement of the European Society of Cardiology Working Group on Myocardial and Pericardial Diseases. Eur. Heart J.34(33), 2636–2648, 2648a–2648d (2013).
- Lazaros G , OikonomouE , TousoulisD. Established and novel treatment options in acute myocarditis, with or without heart failure. Expert Rev. Cardiovasc. Ther.15(1), 25–34 (2017).
- Magee R , RigoutsosI. On the expanding roles of tRNA fragments in modulating cell behavior. Nucleic Acids Res.48(17), 9433–9448 (2020).
- Schimmel P . The emerging complexity of the tRNA world: mammalian tRNAs beyond protein synthesis. Nat. Rev. Mol. Cell. Biol.19(1), 45–58 (2018).
- Fricker R , BrogliR , LuidaleppHet al. A tRNA half modulates translation as stress response in Trypanosoma brucei. Nat. Commun.10(1), 118 (2019).
- Wang Q , LeeI , RenJ , AjaySS , LeeYS , BaoX. Identification and functional characterization of tRNA-derived RNA fragments (tRFs) in respiratory syncytial virus infection. Mol. Ther.21(2), 368–379 (2013).
- Maute RL , SchneiderC , SumazinPet al. tRNA-derived microRNA modulates proliferation and the DNA damage response and is down-regulated in B cell lymphoma. Proc. Natl Acad. Sci. USA110(4), 1404–1409 (2013).
- Hogg MC , RaoofR , ElNaggar Het al. Elevation in plasma tRNA fragments precede seizures in human epilepsy. J. Clin. Invest.129(7), 2946–2951 (2019).
- Yu M , LuB , ZhangJ , DingJ , LiuP , LuY. tRNA-derived RNA fragments in cancer: current status and future perspectives. J. Hematol. Oncol.13(1), 121 (2020).
- Dhahbi JM , SpindlerSR , AtamnaH 5′ tRNA halves are present as abundant complexes in serum, concentrated in blood cells, and modulated by aging and calorie restriction. BMC Genomics14, 298 (2013).
- Friedrich MG , SechtemU , Schulz-MengerJet al. Cardiovascular magnetic resonance in myocarditis: a JACC White Paper. J. Am. Coll. Cardiol.53(17), 1475–1487 (2009).
- Pollack A , KontorovichAR , FusterV , DecGW. Viral myocarditis--diagnosis, treatment options, and current controversies. Nat. Rev. Cardiol.12(11), 670–680 (2015).
- Heymans S , ErikssonU , LehtonenJ , CooperLTJr. The quest for new approaches in myocarditis and inflammatory cardiomyopathy. J. Am. Coll. Cardiol.68(21), 2348–2364 (2016).
- Rose NR . Viral myocarditis. Curr. Opin. Rheumatol.28(4), 383–389 (2016).
- Sagar S , LiuPP , CooperLTJr. Myocarditis. Lancet379(9817), 738–747 (2012).
- Isogai T , YasunagaH , MatsuiHet al. Hospital volume and cardiac complications of endomyocardial biopsy: a retrospective cohort study of 9508 adult patients using a nationwide inpatient database in Japan. Clin. Cardiol.38(3), 164–170 (2015).
- Singh V , MendirichagaR , SavaniGTet al. Comparison of utilization trends, indications, and complications of endomyocardial biopsy in native versus donor hearts (from the Nationwide Inpatient Sample 2002 to 2014). Am. J. Cardiol.121(3), 356–363 (2018).
- Ammirati E , CiprianiM , MoroCet al. Clinical presentation and outcome in a contemporary cohort of patients with acute myocarditis: multicenter lombardy registry. Circulation138(11), 1088–1099 (2018).
- Ammirati E , CiprianiM , LilliuMet al. Survival and left ventricular function changes in fulminant versus nonfulminant acute myocarditis. Circulation136(6), 529–545 (2017).
- Wang J , HanB. Dysregulated CD4+ T Cells and microRNAs in myocarditis. Front. Immunol.11, 539 (2020).
- Corsten MF , DennertR , JochemsSet al. Circulating MicroRNA-208b and MicroRNA-499 reflect myocardial damage in cardiovascular disease. Circ. Cardiovasc. Genet.3(6), 499–506 (2010).
- Corsten MF , PapageorgiouA , VerhesenWet al. MicroRNA profiling identifies microRNA-155 as an adverse mediator of cardiac injury and dysfunction during acute viral myocarditis. Circ. Res.111(4), 415–425 (2012).
- Navarro IC , FerreiraFM , NakayaHIet al. MicroRNA transcriptome profiling in heart of trypanosoma cruzi-infected mice: parasitological and cardiological outcomes. PLoS Negl. Trop. Dis.9(6), e0003828 (2015).
- Liu YL , WuW , XueYet al. MicroRNA-21 and -146b are involved in the pathogenesis of murine viral myocarditis by regulating TH-17 differentiation. Arch. Virol.158(9), 1953–1963 (2013).
- Tao EW , ChengWY , LiWL , YuJ , GaoQY. tiRNAs: a novel class of small noncoding RNAs that helps cells respond to stressors and plays roles in cancer progression. J. Cell. Physiol.235(2), 683–690 (2020).
- Li Q , HuB , HuGWet al. tRNA-derived small non-coding RNAs in response to ischemia inhibit angiogenesis. Sci. Rep.6, 20850 (2016).
- Siracusa J , KoulmannN , BanzetS. Circulating myomiRs: a new class of biomarkers to monitor skeletal muscle in physiology and medicine. J. Cachexia Sarcopenia Muscle9(1), 20–27 (2018).
- Turchinovich A , TonevitskyAG , BurwinkelB. Extracellular miRNA: a collision of two paradigms. Trends Biochem. Sci.41(10), 883–892 (2016).
- Singh R , RamasubramanianB , KanjiS , ChakrabortyAR , HaqueSJ , ChakravartiA. Circulating microRNAs in cancer: hope or hype?Cancer Lett.381(1), 113–121 (2016).
- Kinsey CG , CamolottoSA , BoespflugAMet al. Protective autophagy elicited by RAF-->MEK-->ERK inhibition suggests a treatment strategy for RAS-driven cancers. Nat. Med.25(4), 620–627 (2019).
- Kortlever RM , SodirNM , WilsonCHet al. Myc cooperates with Ras by programming inflammation and immune suppression. Cell171(6), 1301–1315, e1314 (2017).
- Liang F , RenC , WangJet al. The crosstalk between STAT3 and p53/RAS signaling controls cancer cell metastasis and cisplatin resistance via the Slug/MAPK/PI3K/AKT-mediated regulation of EMT and autophagy. Oncogenesis8(10), 59 (2019).
- Honglin S , BoH , JingWet al. ffects of c-Jun N-terminal kinase regulated by CD40siRNA on the autophagy of cardiomyocytes in rats with autoimmune myocarditis. J. Shandong University (Health Sci.)57(4), 9–14 (2019).
- Gu X , LiY , ChenKet al. Exosomes derived from umbilical cord mesenchymal stem cells alleviate viral myocarditis through activating AMPK/mTOR-mediated autophagy flux pathway. J. Cell. Mol. Med.24(13), 7515–7530 (2020).