Abstract
The field of epigenetics is now on a geometric rise, driven in a large part by the realization that modifiers of chromatin are key regulators of biological processes in vivo. The three major classes of epigenetic effectors are DNA methylation, histone post-translational modifications (such as acetylation, methylation or phosphorylation) and small noncoding RNAs (most notably microRNAs). In this article, I report from Select Biosciences Epigenetics Europe 2010 industry conference held on 14–15 September 2010 at The Burlington Hotel, Dublin, Ireland. This industry conference was extremely well attended with a global pool of delegates representing the academic research community, biotechnology companies and pharmaceutical companies, as well as the technology/tool developers. This conference represented the current state of the epigenetics community with cancer/oncology as a key driver. In fact, it has been estimated that approximately 45% of epigenetic researchers today identify cancer/oncology as their main area of focus vis-à-vis their epigenetic research efforts.
ESC: Embryonic stem cell; iPSC: Induced pluripotent stem cell.
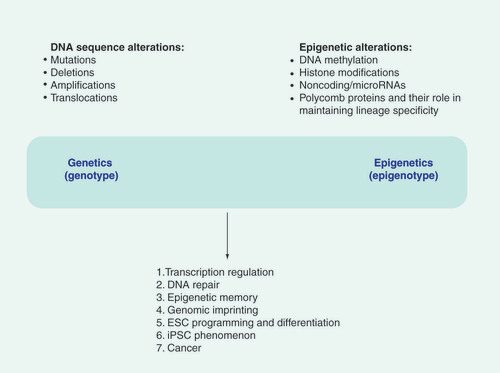
Epigenetics & microRNAs
The keynote presentation was delivered by Dr Manel Esteller, Cancer Epigenetics and Biology Program Director, Bellvitge Institution for Biomedical Research (IDIBELL, Barcelona, Spain) and was titled ‘Epigenetic and Genetic Disruption of Noncoding RNAs in Cancer‘. In this talk, Dr Esteller focused on the effects of epigenetic changes on the expression of microRNAs. Given that microRNAs have crucial roles in the cell – some serving as oncogenes and others as tumor suppressors – epigenetic silencing of microRNA- expression in vivo can alter the growth properties of cells, and in this manner drive cancer development. Dr Esteller and colleagues studied the DNA methylation patterns of microRNA loci and found, for example, a microRNA DNA methylation signature for human cancer metastasis. Specifically, in cell lines derived from lymph node metastases, three microRNAs were shown to possess CpG island hypermethylation – the three microRNAs being miR-148a, miR-34b/c and the miR-9 family. The putative targets of these microRNAs in vivo are c-Myc, EZH2, CDK6 and E2F3. In normal cells, the genes for the microRNAs, miR-148a and miR-34b/c are unmethylated, therefore these microRNAs are expressed in vivo, and downregulate the expression of various cell cycle proteins such as c-Myc, EZH2, CDK6 and E2F3. In a primary tumor without metastases, miR-148a and miR-34b/c stay unmethylated and the lymph nodes are not affected. In a primary tumor with metastases, aberrant methylation shuts down the expression of miR-148a and miR-34b/c. This in turn allows for the upregulated expression of c-Myc, EZH2, CDK6 and E2F3 cell cycle-promoting genes and drives lymph node metastases.
Another microRNA family which Dr Esteller focused on in his presentation was the miR-200 family – master regulators of the epithelial cell phenotype. Unmethylated miR-200 is expressed in the cell and downregulates the expression of various target genes including ZEB1/2 – such a scenario drives cells towards the epithelial phenotype. On the other hand, methylated miR-200 is transcriptionally silenced (by epigenetic silencing) and this in turn enables the ‘unchecked‘ expression of the ZEB1/2 proteins – this is a driver of the downregulation of the epithelial cell phenotype genes. There is dynamic epigenetic regulation of the miR-200 family during the epithelial-to-mesenchymal transition – a key biological process underlying tumor metastases. In summary, there is an intricate relationship between epigenetic modifications and the fine-tuning of gene expression, and hence, biological responses mediated by microRNAs.
Epigenetics in various disease states
Dr Bas Heijmans, associate professor at Leiden University Medical Center (Leiden, The Netherlands) presented a talk on the topic of ‘Epigenetics: linking early development with adulthood disease‘. The focus of this presentation was on assessing the impact of an adverse environment in early childhood on disease development later in life. The hypothesis being such that an environment sets the stage for epigenetic changes which manifest themselves as disease sequelae after a period of time. Dr Heijmans focused on the Dutch food embargo during World War II in the winter of 1944–1945. Owing to precise epidemiological records being available in The Netherlands, he was able to retrospectively identify and trace individuals six decades later who had severe prenatal exposure to ultra-low caloric intake. The candidate genetic locus studied by Dr Heijmans in these studies was the IGF2 gene and its associated methylation pattern in these subjects. The data from these epidemiological studies demonstrated that the prenatal environment may cause epigenetic changes in humans that persist throughout life, and that there exists epigenetic plasticity – for example, in the direction of methylation which is locus-, exposure- and sex-specific. Indeed, these altered loci can potentially serve as biomarkers for disease studies of compromised early development. In summary, adverse prenatal conditions may induce epigenetic signatures which can serve as potential biomarkers and genome-wide studies are required to identify potentially causal effects.
Dr Charles De Smet, Group Leader at the Catholic University of Louvain (Brussels, Belgium) delivered a talk entitled: ‘Genome Hypomethylation and Activation of Germline-specific Genes in Tumors‘. The studies reported focussed on altered DNA methylation patterns in tumors. Tumor antigens are believed to arise through the aberrant activation of germline genes. The cancer germline (CG) genes are composed of approximately 50 genes or gene families with variable functions (perhaps oncogenic), which are activated in a wide variety of tumors, and are regulated by DNA methylation. There is gross DNA hypomethylation and CG gene activation in tumors. An example is the site-specific hypomethylation within the MAGEA1 locus. It was demonstrated that there is a lack of a permanent demethylation activity targeted to the MAGEA1 promoter in expressing tumor cells. The 5´-region of MAGEA1 is protected against de novo methylation. Furthermore, transient DNA demethylation results in stable MAGEA1 promoter hypomethylation. This represents a model for the activation of CG genes during tumor progression; a transient global demethylation followed by the binding of the relevant transcription factors, as well as the steric effects mediated by the transcriptional activators which prevent local remethylation in the vicinity of the CG promoter elements. In summary, DNA hypomethylation in tumors appears to be a tumor-related process and not a stem cell-like event and may in turn ‘stimulate‘ elements that maintain, and perhaps, even progress the tumor state.
Dr Chris Watson, postdoctoral fellow at University College Dublin (Dublin, Ireland) delivered a presentation entitled “Hypoxia alters the DNA Methylation Profile in Cardiac Fibroblasts”. Cardiac fibrosis is the universal response of the heart to most types of injury. The focus of this talk was on the process of ischemia and cardiac fibrosis which results when oxygen demand exceeds supply, and the thesis presented was that epigenetic modifications may have a role to play in the regulation of gene expression following hypoxic insult. Thy-1, a membrane-bound glycoprotein which is a regulator of cell–cell and cell–matrix interactions, and a regulator of intracellular signaling pathways, was studied. Loss of Thy-1 in lung fibroblasts is associated with myofibroblast differentiation in fibroblastic foci. Hypoxia induces the downregulation of Thy-1 and hypoxia induces Thy-1 gene hypermethylation. Indeed, hypoxia was demonstrated to induce genome-wide changes in DNA methylation. There was increased DNA methylation in hypoxic regions and this was concomitant with an increased expression of the DNA methyltransferase Dnmt3B in hypoxia. Dnmt3B contains a hypoxia-response element within its 5´-UTR, a potential binding site for HIF-1α. In addition, microRNAs have also been implicated in Dnmt3B regulation – especially the miR-29 and miR-148a families. In fact, expression of miR-148a is 30% downregulated in primary human cardiac fibroblasts following 8 days exposure to 1% hypoxia. In addition, DNA hypermethylation of the miR-148a gene region has been reported. These findings bring together epigenetic silencing via methylation and microRNAs in a relevant disease system – ischemic disease which results in significant morbidity and mortality in patients.
Epigenetic imprinting & microRNAs
The concept of imprinted genes is becoming more and more pervasive in studying disease. For instance, the loss-of-imprinting of the IGF2 locus has been associated with an increased risk of colorectal cancer, in other words, patients with colorectal cancer exhibit an increased penetrance of two unmethylated alleles at the IGF2 locus. There are approximately 80–100 genes that demonstrate imprinting in vivo with the reasons for this phenomenon remaining obscure at present; however, it appears to involve the maintenance of gene dosage in the cell. An excellent example of this is afforded by the imprinting of one of the X chromosomes in female cells, that is, monoallelic expression dependent on parental origin.
Dr Sue Varmuza, Department of Cell and Systems Biology, University of Toronto (Toronto, Canada) presented a talk entitled: ‘Recent Acquisition of Imprinted Regulation in the Rodent PcG Gene Sfmbt2 by Insertion of microRNA‘. The mouse Sfmbt2 gene is imprinted, whereas the human homolog of this gene is not imprinted. Interestingly, the mouse and rat Sfmbt2 genes possess a block of microRNAs within them. The question addressed within this talk was whether the imprinting of the Sfmbt2 gene is a function of rearrangement or caused by a block of microRNAs contained within the gene? The data presented in this talk demonstrated that the rat gene is imprinted and that the microRNA block (insertion) may in fact drive epigenetic silencing. The intron in the mouse gene contains 110 annotated microRNAs, whereas the rat intron contains 21 microRNAs. The microRNAs reside in the miR-467 family. These intriguing data suggest the possibility that small noncoding RNAs may in fact drive imprinting. In support of this hypothesis is the observation that a locus in the human genome (19q13.4) containing a large block of microRNAs displays parent-of-origin effects; the primary transcript encoding these microRNAs is expressed in the placenta from the paternal genome. This suggests that these microRNAs are imprinted, that is, expressed from one parental genome and not the other. In summary, the mouse and rat Sfmbt2 genes are imprinted; whereas the human, pig and cow genes are biallelic. Both mice and rats possess a block of microRNAs not present in other mammals, consistent with their recent acquisition in the rodent lineage. Therefore, the microRNAs may in fact be driving imprinting at this locus.
Conclusion
This conference brought together some of the current topics in epigenetics, namely epigenetics in cancer, epigenetics in other diseases as well as the convergence of epigenetics and microRNAs in the fine-structure regulation of some genes in vivo. The full significance of epigenetic silencing in vivo is not fully understood, but it is important to note that methylation is a key mechanism in epigenetic silencing. The role of epigenetics in cancer is unquestioned and this conference provided yet more specific evidence that a link does indeed exist. Furthermore, epigenetic silencing via methylation may in fact be involved in other diseases, such as the cardiovascular disease ischemia.
Finally, the association of epigenetics and microRNAs suggests the common evolution of these mechanisms of control in vivo and further underscores the complex and intricate ways in which our genome is regulated to give rise to the complex biological phenotypes in normal physiology and in disease processes.
Financial & competing interests disclosure
Dr Razvi is Vice President of System Biosciences, a company that developes tools and reagents for the study of stem cells and microRNAs. The author has no other relevant affiliations or financial involvement with any organization or entity with a financial interest in or financial conflict with the subject matter or materials discussed in the manuscript apart from those disclosed.
No writing assistance was utilized in the production of this manuscript.