Abstract
Helicobacter pylori infection of the human stomach causes chronic inflammation and forms a major risk factor for the development of peptic ulcer disease and gastric cancer. Current standard eradication therapies use an acid-suppressing drug and two antibiotics, now frequently supplemented with bismuth. Declining eradication efficiencies, off-target effects of lengthy broad-spectrum antibiotic treatments and the desire of a more systematic eradication in asymptomatic H. pylori carriers to suppress gastric cancer incidence spur a search for an effective vaccine and alternative therapeutic options. Here, we review the current progress in the field, with an emphasis on narrow-spectrum or nonantibiotic therapeutics.
Helicobacter pylori is a Gram-negative, microaerophilic stomach pathogen that chronically infects as much as half the world's population [Citation1]. The infection is typically associated with asymptomatic gastritis, but results in more severe clinical outcomes such as stomach and duodenal ulcers and stomach cancers in up to 10 or 1% of the infected, respectively [Citation2]. A recent study estimated that the worldwide fraction of noncardia gastric cancer attributable to H. pylori increased from 74.7% (2008) to 89.0% (2015) [Citation3]. This would correspond to approximately 120,000 additional cases of noncardia gastric cancer attributable to H. pylori infection for a total of around 780,000 cases or 6.2% of all cancers yearly worldwide.
When left untreated, H. pylori infections will remain in the host for a lifetime. To maintain chronic infection in the stomach, the bacterium colonizes the stomach mucosa and overlying mucus, a few hundred microns away from the noxious low pH lumen of the stomach. Low pH conditions can temporarily be overcome by the buffering effect of decomposing host-derived urea to NH3 and CO2 [Citation4]. Lophotrichous flagella and H. pylori's spiral shape allow it to move within the thick mucus layer, guided by pH- and urea-driven chemotaxis systems that bias motility toward the mucosa and away from the gastric lumen [Citation4,Citation5]. In the mucosa, the bacterium can maintain intimate interaction with the epithelial cells. H. pylori-specific outer membrane proteins (HOPs) provide the major class of adhesins that enable high-affinity binding with protein and glycan-based receptors found on the stomach wall [Citation6]. Expression of HOP adhesins is controlled by recombination and phase variation, creating dynamic adherence profiles during the course of infection, likely allowing the bacterium to respond to changing conditions at the infection site [Citation7–9]. At the sites of epithelial adherence, the bacterium alters tissue physiology and morphology by the delivery of virulence factors such as the CagA and VacA toxins, worsening the pathology of the infection [Citation10].
Humans form the primary host for H. pylori and it is assumed that in former days, close to 100% of the human population was infected with H. pylori [Citation11]. Although the use of antibiotics during the last decades and improved hygiene conditions has strongly reduced H. pylori prevalence over the last decades, infection incidence remains high on a global scale. About a third of the adults in northern European and North American populations are still infected, while in southern and eastern Europe, South America and Asia, the prevalence of H. pylori is often still higher than 50% [Citation12]. In developing countries and indigenous populations such as the Inuits and First Nation people, this can go up to 90% [Citation12], although the lower prevalence of infection in the younger generations suggests a further decline of H. pylori prevalence in the coming decades. Interpersonal transmission appears to be the main route of infection and low socioeconomic conditions in childhood form the most important risk factors for H. pylori infection [Citation13].
In adults, the current standard eradication treatment for H. pylori infections comprises triple or quadruple combination therapies. Triple therapy involves a proton pump inhibitor (PPI) as pH-control pharmaceutic, and the concomitant or consecutive treatment with two antibiotics (clarithromycin, metronidazole or amoxicillin) for 1–2 weeks. The success of this therapy is unfortunately under pressure due to a rising antibiotic resistance [Citation14]. Antibiotic resistance stems from escape mutations, drug inactivation, drug efflux pumps and altered membrane permeability but also antibiotic tolerance due to the presence of biofilm-embedded or dormant, nonreplicating bacteria can cause recalcitrant and recurrent infection [Citation15]. A meta-analysis of 87 studies from 2009 to 2014 showed that average rates of H. pylori antibiotic resistance were 47.22% (30.5–75.02%) for metronidazole, 19.74% (5.46–30.8%) for clarithromycin, 18.94% (14.19–25.28%) for levofloxacin and 14.67% (2–40.87%) for amoxicillin, 11.70% (0–50%) for tetracycline, 11.5% (0–23%) for furazolidone and 6.75% (1–12.45%) for rifabutin [Citation16,Citation17]. For this reason, the Maastricht group in Europe reviews H. pylori eradication efficacies and optimal treatment regimens per region on a 2-year basis [Citation18]. In recent years, treatment being prolonged to 2 weeks and quadruple therapy, which includes bismuth or a third antibiotic (tetracyclin, levofloxacin or furazolidone), is becoming more strongly recommended as a first-line treatment [Citation18,Citation19]. The high dosage and longitude of these treatments places a heavy burden on the patient and a lack in patient compliance is, therefore, another main reason for treatment failure. When treatment fails, rescue therapy is considered, but is recommended only in patients who have failed to respond with three or more prior treatments [Citation19]. A rifabutin-based therapy combined with a high-dose PPI is often prescribed [Citation20]. Also moenomycin, a transglycosylase inhibitor that blocks peptidoglycan synthesis and shows good antibiotic activity in Gram-positive bacteria, has been shown effective against H. pylori and to treat gastric ulcers [Citation21,Citation22]. Moenomycin showed antibacterial activity against multidrug-resistant strains, perhaps making it a good candidate for a rescue antibiotic [Citation21].
Besides concerns on dwindling eradication efficiencies, the prolonged treatment with multiple broad-spectrum antibiotics also brings health concerns due to adverse effects on the commensal microbiota. Antibiotic-induced dysbiosis frequently leads to intestinal discomfort, poses an acute risk for blooms of opportunistic pathogens such as Clostridium difficile, but also brings longer-term health risks including inflammatory bowel syndromes and may induce or expedite metabolic diseases such as obesity, fatty liver disease and Type 2 diabetes [Citation23,Citation24]. The collateral damage by broad-spectrum antibiotics to the gut flora is, therefore, a particular concern in pediatric H. pylori treatment.
Today, eradication therapy is prescribed primarily in H. pylori positive adults with peptic ulcer disease, mucosa-associated lymphoid tissue lymphoma, or in patients at increased risk or in remission of gastric cancer. A recent meta-analysis of randomized-controlled trials also showed that preventative eradication of H. pylori can reduce the incidence of gastric cancer in healthy asymptomatic infected individuals, at least in populations with a high gastric cancer burden [Citation25]. However, this is likely to exacerbate the spread of drug resistance, and also the health risks associated with available antibiotic treatments make large-scale deployment of H. pylori eradication programs unlikely unless novel therapeutic options or a vaccine are developed.
It is evident that the current treatment options are under pressure and that innovative antibiotics, alternative treatments or a vaccine are needed. Here, we review ongoing efforts for the development of new H. pylori treatments, with a particular emphasis on narrow-spectrum and nonantibiotic developments. Considering the high prevalence of H. pylori and the long-term risk of gastric cancer development also in asymptomatic carriers, many groups have investigated the development of an effective vaccine. The difficulty lies in the formulation of a protective and lasting mucosal vaccine that is also cross-reactive toward the many clinical isolates that show a high genetic diversity. For recent reviews of this progress in H. pylori vaccine development, the reader is directed to [Citation26,Citation27].
Future H. pylori treatments: broad-spectrum antibacterial agents
Most antibiotic classes in clinical use are of natural origin and have bacterio-static or -lytic properties by inhibiting household pathways such as DNA replication and protein synthesis, or by compromising membrane and cell wall integrity (). These pathways are widespread and their inhibitors often have broad-spectrum activity, although susceptibility can vary markedly among genera depending on the level of sequence conservation in the pathway or due to secondary properties such as cell wall composition. With the increased molecular understanding of bacterial physiology, additional pathways have come under scrutiny for de novo, synthetic drug discovery. The pathways of choice should fulfill essential roles in bacteria and be absent or phylogenetically distant in mammalia to avoid cross-reactivity and toxicity.
Typical first- and second-line broad-spectrum antibiotics used in H. pylori treatment target DNA synthesis and replication (1: metronidazole, levofloxacin, ciprofloxacin, furazolidone), transcription and translation of new proteins (2: tetracycline, clarithromycin), and membrane and cell wall synthesis (3: amoxycilin, moenomycin; PG). Novel broad-spectrum targets under investigation for H. pylori treatment include bacterial metabolic or synthetic pathways (4: shikimate pathway, succinylase, coenzyme A biosynthesis), electron transport chains and carriers (5: flavodoxins). In addition, a number of pathways are targeted that are expected to result in inhibitors with narrow-spectrum activity aimed at H. pylori specifically. These include pH control pathways (6: urease activity and maturation, Ni2+ import), H. pylori adherence (7) and virulence factor secretion and activity (8: CagA secretion, activity).
IM: Inner membrane; OM: Outer membrane; PG: Peptidoglycan.
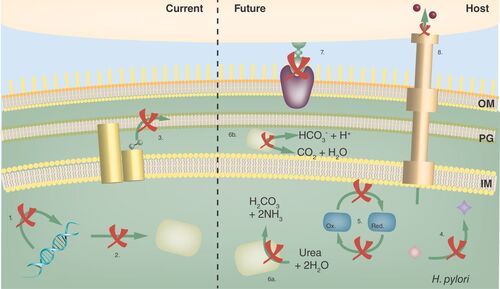
One such pathway that shows promise in targeting H. pylori is the shikimate pathway, responsible for the synthesis of aromatic amino acids and ubiquinone. Different steps in the pathway have been targeted, including the second- and third-step enzymes dehydroquinate synthase and dehydroquinase, providing multiple inhibitors, some with nanomolar affinity () [Citation28–30]. Most are reversible competitive binders, however, meaning that they do not have a lasting effect and require constant high doses to reach the bacteria. The kinase in the fifth step has also been targeted, resulting in inhibitors with a low micromolar affinity, including a compound with a noncompetitive inhibition mechanism [Citation29,Citation31–33]. Although multiple candidate inhibitors are in development, these still require in vivo validation. Another promising target for novel antibiotics is flavodoxin, an electron carrier protein essential for H. pylori viability. To identify inhibitors against H. pylori flavodoxin, a high-throughput screening was used and resulted in bacteriostatic and bactericidal compounds [Citation34]. These bactericidal compounds were later the basis for newly designed compounds with a greatly improved therapeutic index (ratio between the 50% toxic dose [LD50] and the 50% therapeutic dose [ED50] of a drug), coming from 5, 1.5 and 0.2–9, 38 and 12, respectively, but still need a follow-up with in vivo work [Citation35].
Table 1. Summary of Helicobacter pylori drug targets under investigation.
Coenzyme A is an essential cofactor involved in the citric acid cycle and fatty acid synthesis. A recent study looked for inhibitors of the penultimate step in coenzyme A biosynthesis, performed by the enzyme phosphopantetheine adenylyltransferase (PPAT) [Citation41]. hpPPAT shares almost no identity to the human homolog, thus making it a good candidate target. In virtual screens based on the protein's crystal structure, the chemotherapy and immune-suppressant antifolate agent methotrexate or D-amethopterin was found as candidate inhibitor of hpPPAT [Citation41]. This compound has also been found to antibacterial activity against Pneumocystis carinii [Citation60]. D-amethopterin showed a low micromolar-binding affinity for hpPPAT and inhibited its activity with an inhibition constant Ki of 362.9 μM. Based on the docked structure from the virtual screening, the authors suggest that several alterations could increase its affinity [Citation41].
Also, the succinylase pathway is specific to bacteria and forms the only pathway H. pylori has to produce lysine. DapE catalyzes the hydrolysis of N-succinyl-L,L-diaminopimelic acid, forming succinate and L,L-2,6-diaminoheptanedioate, an intermediate involved in the synthesis of lysine and meso-diaminopimelic acid, a peptidoglycan component. Deletion of dapE is lethal, spurring the search for small-molecule inhibitors [Citation37,Citation61]. Several potential candidates were discovered through molecular docking against the hpDapE structure, showing good theoretical-binding scores and higher than the known E. coli DapE inhibitor Captopril, although these compounds have yet to be validated in vitro for their DapE inhibitory potential [Citation37].
Additional steps in the peptidoglycan biosynthesis can be targeted. While many antibiotics have been found for the first and last steps of the pathway, such as inhibition of the MurA enzyme and the famous β-lactams inhibiting cross-linking, there are some crucial steps in between that are interesting targets. One of these is the glutamate racemase MurI that produces the D-glutamate to incorporate in the peptidoglycan. The company AstraZeneca (MA, USA/Gothenburg, Sweden) has performed a series of high-throughput screenings, resulting in potent inhibitors that show a specificity toward H. pylori glutamate racemase compared with other bacterial racemases [Citation38,Citation40,Citation62,Citation63]. Surprisingly, a series of leads were found that were noncompetitive inhibitors, interacting with a cryptic allosteric site when the enzyme is bound to its substrate. It was hypothesized that by targeting these cryptic sites, which are not catalytically active, resistance would be low. Unfortunately, in vivo studies in mice showed poor clearance and bioavailability and have stopped the project from moving on to clinical studies [Citation62,Citation64]. Additionally, H. pylori was found to raise resistance by mutating glutamate racemase, lowering the affinity for the glutamate substrate and lowering the inhibition [Citation64]. Nonetheless, the H. pylori MurI remains an attractive target, with two different cryptic allosteric sites and shows to be different from other bacterial MurI allowing for specificity.
Future H. pylori treatments: narrow-spectrum antibacterial agents
A caveat from broad-spectrum antibiotics is their off-target effect on the microbiome. This facilitates horizontal spread of resistance genes, and also antibiotic-induced dysbiosis is increasingly recognized as a long-term health concern [Citation23,Citation24]. An alternative approach is to focus on molecular pathways that are specific to and/or particularly vulnerable in H. pylori. For H. pylori, pH maintenance and pH responsiveness are critical and a lot of attention has gone to interfere with related pathways [Citation4]. The acidic conditions in the gastric lumen (≈pH 2) are noxious to bacteria, including H. pylori. The bacterium colonizes the gastric mucosa and inner layer of the overlying mucus, where the pH is near physiological pH 6, but can temporarily survive more acidic conditions thanks to its urease activity. Urease is a highly expressed protein in H. pylori, where it takes up about 15% of the total produced proteins [Citation4]. It catalyzes the hydrolysis of urea into carbamate and ammonia, followed by spontaneous decomposition of carbamate into a second ammonia and carbonic acid. The latter decomposes to water and carbon dioxide in a reaction catalyzed by the cytosolic β-carbonic anhydrase [Citation4] (). The released ammonia increases the local pH, allowing the bacterium to survive temporary exposure to gastric acid. H. pylori's urease differs from other bacterial ureases, consisting of two subunits forming a dodecameric architecture [Citation65]. Several potential inhibitors have been identified, including hydroxamic acids, phosphoramidates, quinones, polyphenols, benzimidazoles, flavonoids, heterocyclic compounds, metal ions and urea derivatives () [Citation66]. Some work by acting as a nonhydrolysable substrate or transition-state analog, thus binding the catalytic nickel ions as competitive inhibitors, such as the model inhibitors thiourea and hydroxyurea, acetohydroxamic acid (AHA), phosphoramidate or barbituric acid (-ii, A–C; iii, D & E) [Citation42,Citation66,Citation67]. Derivatives of barbituric acid have also been found to have an inhibiting potential and can be used for lead optimization [Citation42,Citation68]. Derivatives of benzimidazoles, benzoquinones, polyphenols and the organoselenium compound ebselen can inhibit urease likely due to their cysteine-binding properties [Citation43,Citation44,Citation66,Citation69,Citation70]. Their exact mode of action is not completely understood, although studies indicate that binding of a conserved catalytic cysteine in the loop near the active site would result in blocking the entrance to the active site and in a loss of its catalytic function (-iv) [Citation71,Citation72]. Ebselen shows a 226-nM nanomolar affinity for H. pylori urease and has been found safe to use in humans, making it a promising lead for future therapy [Citation44]. Notably, benzimidazole PPIs turn into thiol-reactive sulfamides under acidic conditions and several marketed PPIs such as omeprazole, rabeprazole and lansoprazole have also shown inhibition toward urease, possibly by targeting the same active site cysteine, albeit with micromolar affinity at most [Citation44,Citation70,Citation73].
Urease activity requires the cofactor nickel, which is imported into the periplasm by the TonB-dependent importer FrpB4 and possibly FecA3 [Citation74]. Nickel is then transported to the cytoplasm by NixA. The existence and nature of nickel siderophores or chaperones that facilitate capture and delivery of extracellular or periplasmic nickel to the transporters is unclear. Internalized nickel can be stored by interaction with Hpn or is delivered to apourease by the nickel chaperone UreE and the assembly factors UreF, UreG and UreH to yield active urease. Under high nickel concentrations, the response regulator NikR acts as a suppressor for the expression of nixA and as an enhancer for the expression of urease, to maintain the balance inside the cell [Citation75]. (i) The water accessible surface (semitransparent, green), ribbon and stick diagram of the H. pylori urease active site (PDB:1e9y; [Citation65]). The two catalytic Ni2+ are shown as magenta spheres. KCX219: carbamylated Lys219; (ii) Schematic diagram of proposed substrate-binding mechanism and structure of three substrate analog inhibitors: thioureum (A), hydroxyureum (B) and hydroxycarbamoyl derivatives (C; R: R-group); (iii) Schematic diagram of transition state complex and the structures of two transition state analogs: diamino phosphoric acid (D) and its acyl and aryl derivatives (E); (iv) Schematic diagram of the binding mechanism of thiol-reactive urease inhibitors such as the organoselenium drug ebselen (R = phenyl). Benzimidazole PPIs such as rabeprazole are thiolreactive under acidic conditions and were also found to inhibit urease, presumably by binding with Cys321 [Citation66,Citation76].
IM: Inner membrane; OM: Outer membrane; PG: Peptidoglycan.
![Figure 2. The urease activity and biogenesis pathway show possible targets for treatment against H. pylori.Urease activity requires the cofactor nickel, which is imported into the periplasm by the TonB-dependent importer FrpB4 and possibly FecA3 [Citation74]. Nickel is then transported to the cytoplasm by NixA. The existence and nature of nickel siderophores or chaperones that facilitate capture and delivery of extracellular or periplasmic nickel to the transporters is unclear. Internalized nickel can be stored by interaction with Hpn or is delivered to apourease by the nickel chaperone UreE and the assembly factors UreF, UreG and UreH to yield active urease. Under high nickel concentrations, the response regulator NikR acts as a suppressor for the expression of nixA and as an enhancer for the expression of urease, to maintain the balance inside the cell [Citation75]. (i) The water accessible surface (semitransparent, green), ribbon and stick diagram of the H. pylori urease active site (PDB:1e9y; [Citation65]). The two catalytic Ni2+ are shown as magenta spheres. KCX219: carbamylated Lys219; (ii) Schematic diagram of proposed substrate-binding mechanism and structure of three substrate analog inhibitors: thioureum (A), hydroxyureum (B) and hydroxycarbamoyl derivatives (C; R: R-group); (iii) Schematic diagram of transition state complex and the structures of two transition state analogs: diamino phosphoric acid (D) and its acyl and aryl derivatives (E); (iv) Schematic diagram of the binding mechanism of thiol-reactive urease inhibitors such as the organoselenium drug ebselen (R = phenyl). Benzimidazole PPIs such as rabeprazole are thiolreactive under acidic conditions and were also found to inhibit urease, presumably by binding with Cys321 [Citation66,Citation76].IM: Inner membrane; OM: Outer membrane; PG: Peptidoglycan.](/cms/asset/3748091c-90a1-4cf1-8d34-3cc217bbe9bd/ifmb_a_12327638_f0002.jpg)
Although several urease inhibitory compounds have been identified, just one has reached the market thus far, AHA (Lithostat®), a drug approved in the USA and Europe for treatment of infection-induced struvite bladder stones [Citation66]. Other urease inhibitors have failed further development due to toxicity or fast hydrolysis in the stomach [Citation66,Citation77]. Some natural products also show an inhibitory effect against H. pylori urease [Citation78,Citation79]. Honey and plants are shown to contain one or more compounds that interact with urease, such as polyphenols or flavonoids, thiourea, dihydroxyacetone and sulforaphane [Citation46,Citation47,Citation80,Citation81].
Urease activity can also be targeted indirectly by cutting-off its availability of nickel ions, the cofactor it requires in order to be functional [Citation82]. Nickel is a scarce metal that requires active import over the outer membrane. Nickel is transported to the cytoplasm by the enzyme NixA, followed by nickel chaperones such as HypA and UreE to bring it to the urease complex during its maturation [Citation83,Citation84]. Other histidine-rich proteins found in H. pylori, such as HspA and Hpn have also suggested to play a role in the nickel homeostasis [Citation85]. Interestingly, bismuth used in quadruple therapy is also reported to inhibit urease activity by displacing the nickel cofactor [Citation86,Citation87]. As the molecular players and principals involved in nickel acquisition and urease maturation are being identified, these are likely to open up new to inhibit H. pylori's urease activity.
Carbonic anhydrase catalyzes the interconversion of carbon dioxide and water to bicarbonate and a proton. In H. pylori, this plays a role in pH homeostasis together with urease to maintain the neutral pH in the periplasm and cytoplasm, essential to grow in the gastric niche and a possible novel target for treatment () [Citation48,Citation49,Citation88]. H. pylori has two different anhydrases, namely the α- and β-carbonic anhydrase, whereas its close relative Campylobacter jejuni only has the β-class. Inhibitors have already been found, where the carbonic anhydrases have been shown to be highly inhibited by sulfonamides, down to nanomolar range. Interestingly, this included several clinically used and approved drugs such as topiramate, acetazolamide and methazolamide, the latter two also inhibiting growth [Citation48,Citation49,Citation88]. These drugs were reported to have an anti-ulcer effect in the early 1980s, but the mechanism remained unknown [Citation49]. The structure has recently been solved, which would allow to procure inhibitors that show a specificity to H. pylori carbonic anhydrases [Citation89]. Although the β-carbonic anhydrase would be a more interesting target, since the α-class is also found in humans and other mammals, it is situated in the cytoplasm compared with the periplasmic α-carbonic anhydrase, making it harder to reach.
Another possible narrow-range target that gained interest is the Pse or pseudaminic biosynthesis pathway. The pathway plays its role in flagella synthesis, glycosylating the flagellin with a pseudoaminic acid sugar. While found in other bacterial species, it is only been demonstrated to be essential for motility and flagellin assembly in H. pylori, C. jejuni, C. coli and A. caviae [Citation90,Citation91]. Since motility is essential to colonize the stomach initially and also to swim back to the mucus when the bacteria is detached, inhibiting this pathway would aid in eradicating H. pylori. A starting point in finding inhibitors has been achieved by virtual screening followed by high-throughput assays [Citation51]. They found new compounds showing inhibition of PseC or PseB, but some also inhibited PseG next to PseB, which increases efficacy and specificity. Follow-up with cellular assays has not yet been achieved, since hydrophobicity and membrane permeability need to be optimized [Citation51].
Future H. pylori treatments: natural agents
Over the course of history, plants and plant extracts have long formed the major therapeutic agents available and herbal medicine has frequently served as a leading source in the discovery and development of modern molecular drugs. Several plants traditionally used to treat gastritis and other gastric symptoms in the east and developing countries have proven to have killing or growth inhibiting effect on H. pylori [Citation92–94]. Two reviews by Zaidi et al. and Guadalupe et al. provide a good overview of the recent in vitro, in vivo and clinical studies [Citation95,Citation96]. They show that intake of several plants and plant extracts [Citation79] such as cranberry juice [Citation97], sulforaphane found in broccoli sprouts [Citation98,Citation99], xanthones [Citation100], green tea [Citation101] and red wine [Citation102] could potentially reduce the pathogenicity of H. pylori through anti-inflammatory and/or bactericidal effects. In other cases, in vitro activity did not correlate well with the in vivo data or was inconclusive due to the lack of reliable in vivo results [Citation96]. One possible reason for the lack of success in identifying and isolating therapeutic compounds from medicinal plant extracts may be that the molecular action is multifactorial, depending on the concomitant activity of multiple agents. Also, some plant-derived extracts had indirect beneficial effects like antiulcer effects, but did not seem to suppress or eradicate H. pylori [Citation103]. Plant extracts have also been found to have antiadhesive properties in some cases, such as green tea extracts, which inhibited binding of H. pylori to human- and mouse-immortalized epithelial cell lines, although an optimal effect was seen only when the bacteria were preincubated rather than when the bacteria were already attached [Citation96,Citation104]. Also roots extracts of ironweed (Vernomia kotschyma) or Cochlospermum tinctorium, which have been used in Malian traditional medicine for the treatment of gastric ulcers and root extracts from liquorice (Glycyrrhiza glabra) show a partial inhibition of H. pylori adherence to human gastric adenocarcinoma cells and human gastric tissue sections, respectively [Citation105,Citation106].
Another natural agent studied for its possible bactericidal or bacteriostatic effect against H. pylori is honey, known for its antibacterial activity due to its osmotic effect, acidity and hydrogen peroxide content [Citation107,Citation108]. Several honeys and their solvent extracts showed H. pylori growth inhibition in vitro, with some varieties having a MIC50 comparable to that of clarithromycin and amoxicillin [Citation108].
Finally, probiotics like several Lactobacillus spp., Bifidobacterium spp. and Bacillus subtilis have been investigated as a treatment supplement to reduce the side effects of current antibiotic-based H. pylori treatments [Citation96,Citation109,Citation110]. Several clinical trials with adult groups ranging from 60 to 160 dyspeptic patients have shown increased eradication ratios when triple-eradication therapy was supplemented with probiotics, as well as a lower incidence in adverse treatment effects [Citation109]. Importantly, clinical trials with dyspeptic and asymptomatic children in groups of 12–295 children show similar results. Probiotics are believed to exert their beneficial effect on H. pylori eradication by influencing pro- and anti-inflammatory cytokine release through binding with Toll-like receptors, thereby reducing inflammation, but also stimulating the secretion of IgAs and migration of monocytes and dendritic cells [Citation96,Citation109,Citation110]. They also procure an additional antibactericidal effect, providing partial eradication due to secreted factors like autolysins in Lactobacillus acidophilus and antibiotics, such as amicoumacin A, in B. subtilis [Citation96,Citation111,Citation112]. But Bifidobacterium bifidum has also been seen to have some antiadhesive effect on H. pylori by site competition and/or enhancing gastric mucin production [Citation113,Citation114].
Future H. pylori treatments: antivirulence therapeutics
Another approach to combat H. pylori infection is to target its virulence factors, that is, those proteins and pathways that lead to pathogenic, symptomatic colonization of the stomach. Although many virulence factors are not indispensable, their inhibition can sufficiently attenuate infectivity to allow stand-alone therapy or can provide strong synergy with classic antibiotics, thereby increasing specificity and eradication efficiency of the therapy [Citation115]. In H. pylori, three virulence factors have since long been associated with overt disease and worsened infection prognosis: the CagA oncoprotein that is delivered into host cells by a Type IV secretion system encoded by the cytotoxin-associated genes pathogenicity island (cagPAI), the secreted vacuolating cytotoxin VacA and the cell surface adhesin BabA [Citation2,Citation116]. CagA modulates signal transduction pathways in gastric epithelial cells, causing cell remodeling and tissue damage, whereas VacA induces massive vacuole formation and localizes to the mitochondria where it induces apoptosis [Citation10]. H. pylori adherence helps to target colonization to the stomach mucosa and mucous layer, away from the noxious gastric lumen, potentiates the contact-dependent delivery of CagA and has been suggested to influence host responses [Citation117,Citation118].
Two groups have presented inhibitors of the H. pylori Cag Type IV secretion system [Citation54,Citation119]. In both cases, the compounds target the ATPase Cagα (VirB11), which is required to drive CagA secretion and delivery. Using high-throughput screening, Hillerangman et al. found three unrelated Cagα inhibitors with nanomolar range IC50 values. The most active compound, CHIR-1, inhibited H. pylori – CagA-induced elongation of cultured AGS cells, and showed a decrease in H. pylori colonization in a mouse infection model. However, full inhibition was only seen when the bacteria were preincubated with CHIR-1, making it less attractive for use in treatments [Citation119]. An in silico screen of a kinase-targeting compound library docked against the Cagα ATPase found a series of imidazo[1,2-a]pyrazine derivatives as lead compounds. Synthesis and derivatization of the best leads resulted in a series of compounds that acted as competitive inhibitors of the enzyme with IC50s in the low micromolar range [Citation54]. Also difluoromethylornithine (DFMO) has been found to inhibit H. pylori growth, to attenuate CagA delivery to cultured AGS cells and reduce the severity of gastritis in infected mice [Citation52]. These inhibitory effects of DFMO treatment were reversible, however, and required a continuous administration of 1% DFMO in the drinking water (estimated daily dosage 4000–7500 mg/kg). Although this dose is high, equivalent levels have been used in humans for treatment of trypanosomiasis [Citation120].
Targeting bacterial adherence can form a potent nonantibiotic treatment option as seen from examples in bacterial bladder infections [Citation121,Citation122]. In H. pylori, the blood group antigen-binding adhesin BabA binds Lewis B glycans in the stomach mucosa and forms a primary disease-associated virulence factor [Citation57,Citation116,Citation123]. Porcine milk proteins abundantly express Lewis B glycans, as well as sialyl Lewis X, the receptor for H. pylori's inflammation-associated adhesin SabA [Citation124,Citation125]. Oral treatment with porcine milk proteins showed reduced infection load and prevented new colonization of H. pylori in a mouse infection model [Citation124]. Also extracts of turmeric, borage and parsley have been found to inhibit binding of the bacterium to stomach sections expressing Lewis B and Lewis A [Citation126]. Since BabA does not bind Lewis A, the extract is hypothesized to also inhibit other adhesins, but its mechanism remains unknown. A recent structural study of the BabA adhesin showed a high degree of structural polymorphism in the Lewis B-binding pocket among different clinical isolates, giving a sobering prospect for the development of high affinity, cross-reactive adhesion inhibitors [Citation57]. However, a conserved disulfide in the receptor-binding site proved vulnerable to the reducing pharmaceutic N-acetylcysteine (NAC), which inhibited BabA-mediated adherence to gastric tissue sections (IC50: 10 mg/ml) and suppressed gastric infection load and neutrophil infiltration in a mouse model. Notably, in two small human trials, NAC supplements had previously shown a synergistic effect on H. pylori eradication with triple therapy, an activity that was ascribed to its mucolytic properties () [Citation127,Citation128].
An important component in the failure of antibiotic treatments is the low proportion that actually reaches the bacterium. H. pylori resides in the mucus, which is a protective layer for the stomach wall. Its high viscosity reduces solute diffusion rates, including those of antibiotics. In addition, biofilm formation is known to occur with H. pylori and can further reduce antibiotic bioavailability. To address this, mucolytic agents have been coadministered with antibiotics to break down the mucus and biofilm integrity, presumably allowing the antibiotics to reach H. pylori in a high-concentration manner [Citation127,Citation128]. In addition, the redox-active mucolytic N-acetylcysteine was recently found to inhibit BabA-mediated adherence to mucosal glycoproteins (inset) [Citation57].
![Figure 3. The mucus layer as a protective niche for Helicobacter pylori.An important component in the failure of antibiotic treatments is the low proportion that actually reaches the bacterium. H. pylori resides in the mucus, which is a protective layer for the stomach wall. Its high viscosity reduces solute diffusion rates, including those of antibiotics. In addition, biofilm formation is known to occur with H. pylori and can further reduce antibiotic bioavailability. To address this, mucolytic agents have been coadministered with antibiotics to break down the mucus and biofilm integrity, presumably allowing the antibiotics to reach H. pylori in a high-concentration manner [Citation127,Citation128]. In addition, the redox-active mucolytic N-acetylcysteine was recently found to inhibit BabA-mediated adherence to mucosal glycoproteins (inset) [Citation57].](/cms/asset/caa4aae2-93fc-4490-9c25-bd171cb60d33/ifmb_a_12327638_f0003.jpg)
Other factors that play a prominent role in the pathogenic process include inhibition of T-cell proliferation by γ-glutamyltranspeptidase, as well as cell junction remodeling by the secreted protease HtrA [Citation10,Citation129]. HtrA is a serine protease that disrupts epithelial cell–cell adhesion by cleavage of E-cadherin and was shown to be essential for H. pylori infection [Citation130,Citation131]. Using a receptor-based virtual screen, Löwer et al. identified six inhibitors that inhibited in vitro HtrA activity with micromolar range IC50 and reduced E-cadherin cleavage and cell scattering in cell-based studies [Citation55]. Later, Perna et al. used a fragment-based de novo design approach to generate inhibitor candidates, albeit with low micromolar inhibitory activity only [Citation56]. Thus, since the recent molecular description of HtrA's essential role in H. pylori infection, a number of medium affinity inhibitors have been identified, although their in vivo activity and efficacy in H. pylori eradication therapies have yet to be established.
Future H. pylori treatments: mucolytic agents
The mucus layer covering the gastric epithelium provides a shielding neutral environment that protects the stomach wall cells, but also forms the hospitable niche for H. pylori. It consists of an abundance of high-molecular-weight glycoproteins, called mucins and some lipids, small molecules and other proteins. Through disulfide bonding, the mucins form a viscoelastic layer that lowers diffusion rates and is thought to prevent the effective delivery of antibiotics. Several studies have evaluated the potential of mucolytic agents, that is, agents that lower mucus integrity and its barrier function, as an additive to antibiotic treatment (). Pronase is a protease known to be mucolytic and to reduce mucus viscosity [Citation58,Citation132]. Gotoh et al. showed in a clinical trial consisting of 135 patients assigned into two treatment groups that pronase improved the cure rate of triple therapy by 15% [Citation58]. Pronase did not directly interact with H. pylori in vitro, so that the authors suggested its mucolytic activity improved antibiotic delivery and/or made the gastric environment less hospitable for the bacterium. Although proven effective, pronase treatment is counter-indicated in patients with active ulcers due to the increased risk for life-threatening bleeding when disrupting the mucus layer. Erdosteine, another strong mucolytic agent was also tested as an additive with the standard triple treatment. In a double-blind, placebo-controlled clinical study, the eradication ratio increased from 52.6 to 78.9% when erdosteine was added, without any additional side effect, although treatment costs would increase by approximately 27% [Citation59]. NAC is another mucolytic agent to show an effect against H. pylori infection [Citation127,Citation128]. Cammarota et al. and Gurbuz et al. both showed that when NAC is added to antibiotic therapy in patients who had previously failed treatment, the eradication ratio increased from 20 to 65% and from 23 to 50%, respectively. Cammarota et al. also showed that when NAC is added to biopsies, H. pylori did not form a biofilm. More recently, Moonens et al. showed that under the concentrations used for treatment, NAC's synergistic activity in H. pylori eradication therapy may also stem from its inhibition of BabA-mediated adherence (see above) [Citation57]. At present NAC supplements require high dosage and are accompanied with patient discomfort due to their mucolytic activity. In the scenario that NAC's antibacterial activity stems primarily from its direct effect on bacterial adherence rather than from its mucolytic activity, increasing NAC's affinity for the BabA receptor-binding site may result in more potent and specific treatment supplements.
Conclusion
H. pylori's role as a gastric pathogen and the primary role in peptic ulcer and gastric cancer development were established and accepted by the medical community only as recently as the 1980s. Symptomatic H. pylori infections are since treated with available antibiotics and acid-suppressing drugs, but the species quickly proved a resilient pest. The long and burdensome antibiotic treatment against H. pylori infection is increasingly starting to fail, and new, innovative paths towards treatment need to be taken. A number of broad-range antibacterial targets have led to candidate antibiotics also active against H. pylori. These can fuel the arsenal of second-line and rescue eradication treatments, but their low selectivity toward H. pylori still prevents the precautionary treatment in children and precludes more wide-spread eradication programs aimed at suppressing gastric cancer incidence.
H. pylori urease has been at the center of attention for many years for the development of more narrow-spectrum treatment or treatment supplements, and several potent in vitro inhibitors have been found. Nevertheless, many suffer from a lack of specificity and the only marketed bacterial urease inhibitor, AHA (Lithostat), is approved only as an orphan drug for use in struvite inducing chronic urinary tract infections caused by urea-splitting pathogens and is not advised for H. pylori eradication (co-)therapy due to its many and frequent side effects. Two approved and marketed mucolytic agents, erdosteine and NAC, have proven to increase H. pylori eradication efficiencies in clinical trials when given in supplement with triple therapy. Nevertheless, they have not made general use in H. pylori treatment regimens due to the high dosage required, increased cost of treatment and the additional patient discomfort and increased risk for bleeding peptic ulcers associated with mucolytic agents. They do show that nonantibiotic treatments that influence the bacterium's specialized and narrow niche can have beneficial effects on H. pylori eradication.
Future perspective
With the recent advancements in the molecular description of H. pylori pathogenesis, new and promising candidates related to the pathogen's persistence in the host, such as metal cofactor acquisition, adherence and the modulation of the epithelial barrier and the host's immune response, are emerging. Antivirulence agents are advancing into clinical development in infections such as uropathogenic E. coli, where targeting the pathogen's adherence can selectively deplete it from the urinary tract and its gastrointestinal reservoir [Citation121,Citation122]. However, recent structural insights in H. pylori's adhesins have shown the pathogen manages to combine high binding affinity with an extreme genetic diversity in the receptor-binding site, making the development of potent inhibitors active against different clinical isolates a daunting task [Citation57]. H. pylori's secreted and surface-localized virulence proteome shows an increased genetic diversity, making it advisable that future antivirulence developments target its more conserved assembly and secretion pathways instead. Future developments will tell whether potent and specific inhibitors of these pathways can be derived and what their contribution to H. pylori treatment can be.
Helicobacter pylori multidrug resistance is rising to the point where quadruple therapy is now becoming the first-line treatment and future therapeutic efficacy is under increasing threat.
Prolonged treatment regimens with multiple broad-spectrum antibiotics bring patient discomfort and health concerns due to adverse effects on the commensal microbiota.
Novel broad-spectrum agents targeting the shikimate or peptidoglycan synthesis pathway have shown promising antibacterial activity toward H. pylori ex vivo, but await in vivo validation in clinical trials.
Narrow-spectrum drug developments targeting H. pylori have largely concentrated on the pathogen's urease pH buffering pathway. Although several urease inhibitory compounds have been identified, just one has reached the market thus far, albeit for treatment of infection-induced struvite bladder stones rather than as a supplement in H. pylori treatment.
The high morbidity of gastritis and H. pylori infections have resulted in the use of several natural agents in traditional medicine to treat gastric symptoms, particularly in the Far East and in developing countries. Although some of these have proven killing or growth inhibiting activity on H. pylori, isolation of single active ingredients or insight in the molecular basis for their activity is often lacking.
Virulence-targeting antibiotics are coming of age and start to enter clinical trials, most promisingly in the treatment and prevention of bacterial urinary tract infections. Efforts targeting H. pylori virulence are lagging, however, and are complicated by the high genetic diversity of the pathogen. Nevertheless, the increasing molecular and structural insights into the pathogen's virulence pathways are spurring new developments.
The lack of a good and representative nonprimate animal model for H. pylori infection and the usually late onset of clinical manifestations (years) complicate the development and evaluation of antivirulence therapeutics.
Financial & competing interests disclosure
This work was supported by the FWO Flanders through grant G033717N. The authors have no other relevant affiliations or financial involvement with any organization or entity with a financial interest in or financial conflict with the subject matter or materials discussed in the manuscript apart from those disclosed.
No writing assistance was utilized in the production of this manuscript.
Additional information
Funding
References
- Suerbaum S , MichettiP . Helicobacter pylori infection. N. Engl. J. Med.347 (15), 1175–1186 (2002).
- Peek RM , BlaserMJ . Helicobacter pylori and gastrointestinal tract adenocarcinomas. Nat. Rev. Cancer2 (1), 28–37 (2002).
- Plummer M , FranceschiS, VignatJ, FormanD, de MartelC . Global burden of gastric cancer attributable to Helicobacter pylori. Int. J. Cancer136 (2), 487–490 (2015).
- Sachs G , WeeksDL, WenY, MarcusEA, ScottDR, MelchersK . Acid acclimation by Helicobacter pylori. Physiology20 (6), 429–438 (2005).
- Croxen MA , SissonG, MelanoR, HoffmanPS . The Helicobacter pylori chemotaxis receptor TlpB (HP0103) is required for pH taxis and for colonization of the gastric mucosa. J. Bacteriol.188 (7), 2656–2665 (2006).
- Oleastro M , MénardA . The role of Helicobacter pylori outer membrane proteins in adherence and pathogenesis. Biology2 (3), 1110–1134 (2013).
- Salaün L , LinzB, SuerbaumS, SaundersNJ . The diversity within an expanded and redefined repertoire of phase-variable genes in Helicobacter pylori. Microbiology150 (4), 817–830 (2004).
- Goodwin AC , WeinbergerDM, FordCBet al. Expression of the Helicobacter pylori adhesin SabA is controlled via phase variation and the ArsRS signal transduction system. Microbiology154 (8), 2231–2240 (2008).
- Styer CM , HansenLM, CookeCLet al. Expression of the BabA adhesin during experimental infection with Helicobacter pylori. Infect. Immun.78 (4), 1593–1600 (2010).
- Posselt G , BackertS, WesslerS . The functional interplay of Helicobacter pylori factors with gastric epithelial cells induces a multi-step process in pathogenesis. Cell Commun. Signal.11, 77 (2013).
- Suerbaum S , JosenhansC . Helicobacter pylori evolution and phenotypic diversification in a changing host. Nat. Rev. Micro.5 (6), 441–452 (2007).
- Leja M , AxonA, BrennerH . Epidemiology of Helicobacter pylori infection. Helicobacter21 (Suppl. 1), 3–7 (2016).
- Brown LM . Helicobacter pylori: epidemiology and routes of transmission. Epidemiol. Rev.22 (2), 283–297 (2000).
- Gatta L , VakilN, VairaD, ScarpignatoC . Global eradication rates for Helicobacter pylori infection: systematic review and meta-analysis of sequential therapy. BMJ347, f4587 (2013).
- Francesco VD , ZulloA, HassanC, GiorgioF, RosaniaR, IerardiE . Mechanisms of Helicobacter pylori antibiotic resistance: an updated appraisal. World J. Gastrointest. Pathophysiol.2 (3), 35–41 (2011).
- Ghotaslou R , LeylabadloHE, AslYM . Prevalence of antibiotic resistance in Helicobacter pylori: a recent literature review. World J. Methodol.5 (3), 164–174 (2015).
- Thung I , AraminH, VavinskayaVet al. Review article: the global emergence of Helicobacter pylori antibiotic resistance. Aliment Pharmacol. Ther.43 (4), 514–533 (2016).
- Malfertheiner P , MegraudF, O'MorainCAet al. Management of Helicobacter pylori infection – the Maastricht V/Florence Consensus Report. Gut66 (1), 6–30 (2017).
- Fallone CA , ChibaN, van ZantenSVet al. The Toronto Consensus for the treatment of Helicobacter pylori infection in adults. Gastroenterology151 (1), 51.e14–69.e14 (2016).
- Lim HC , LeeYJ, AnB, LeeSW, LeeYC, MoonBS . Rifabutin-based high-dose proton-pump inhibitor and amoxicillin triple regimen as the rescue treatment for Helicobacter pylori. Helicobacter19 (6), 455–461 (2014).
- Tseng Y-Y , LiouJ-M, HsuT-L, ChengW-C, WuM-S, WongC-H . Development of bacterial transglycosylase inhibitors as new antibiotics: moenomycin A treatment for drug-resistant Helicobacter pylori. Bioorg. Med. Chem. Lett.24 (11), 2412–2414 (2014).
- Cheng T-JR , SungM-T, LiaoH-Yet al. Domain requirement of moenomycin binding to bifunctional transglycosylases and development of high-throughput discovery of antibiotics. Proc. Natl Acad. Sci. USA105 (2), 431–436 (2008).
- Keeney KM , Yurist-DoutschS, ArrietaM-C, FinlayBB . Effects of antibiotics on human microbiota and subsequent disease. Annu. Rev. Microbiol.68, 217–235 (2014).
- Boulangé CL , NevesAL, ChillouxJ, NicholsonJK, DumasM-E . Impact of the gut microbiota on inflammation, obesity, and metabolic disease. Genome Med.8, 42 (2016).
- Ford AC , FormanD, HuntRH, YuanY, MoayyediP . Helicobacter pylori eradication therapy to prevent gastric cancer in healthy asymptomatic infected individuals: systematic review and meta-analysis of randomised controlled trials. BMJ348, g3174 (2014).
- Walduck A , AndersenLP, RaghavanS . Inflammation, immunity, and vaccines for Helicobacter pylori infection. Helicobacter20 (Suppl. 1), 17–25 (2015).
- Velin D , StraubingerK, GerhardM . Inflammation, immunity, and vaccines for Helicobacter pylori infection. Helicobacter21 (Suppl. 1), 26–29 (2016).
- Sánchez-Sixto C , PrazeresVFV, CastedoLet al. Competitive inhibitors of Helicobacter pylori Type II dehydroquinase: synthesis, biological evaluation, and NMR studies. ChemMedChem3 (5), 756–770 (2008).
- Gonzalez-Bello C . Inhibition of shikimate kinase and type II dehydroquinase for antibiotic discovery: structure-based design and simulation studies. Curr. Top. Med. Chem.16 (9), 960–977 (2016).
- Prazeres VFV , TizónL, OteroJMet al. Synthesis and biological evaluation of new nanomolar competitive inhibitors of Helicobacter pylori type II dehydroquinase. Structural details of the role of the aromatic moieties with essential residues. J. Med. Chem.53 (1), 191–200 (2010).
- Cheng W-C , ChenY-F, WangH-Jet al. Structures of Helicobacter pylori shikimate kinase reveal a selective inhibitor-induced-fit mechanism. PLoS ONE7 (3), e33481 (2012).
- Han C , ZhangJ, ChenL, ChenK, ShenX, JiangH . Discovery of Helicobacter pylori shikimate kinase inhibitors: bioassay and molecular modeling. Bioorg. Med. Chem.15 (2), 656–662 (2007).
- Han C , WangL, YuKet al. Biochemical characterization and inhibitor discovery of shikimate dehydrogenase from Helicobacter pylori. FEBS J.273 (20), 4682–4692 (2006).
- Cremades N , Velázquez-CampoyA, Martínez-JúlvezMet al. Discovery of specific flavodoxin inhibitors as potential therapeutic agents against Helicobacter pylori infection. ACS Chem. Biol.4 (11), 928–938 (2009).
- Galano JJ , AlíasM, PérezR, Velázquez-CampoyA, HoffmanPS, SanchoJ . Improved flavodoxin inhibitors with potential therapeutic effects against Helicobacter pylori infection. J. Med. Chem.56 (15), 6248–6258 (2013).
- Liu JS , ChengWC, WangHJ, ChenYC, WangWC . Structure-based inhibitor discovery of Helicobacter pylori dehydroquinate synthase. Biochem. Biophys. Res. Comm.373 (1), 1–7 (2008).
- Mandal RS , DasS . In silico approach towards identification of potential inhibitors of Helicobacter pylori DapE. J. Biomol. Struct. Dyn.33 (7), 1460–1473 (2015).
- Geng B , BasarabG, Comita-PrevoirJet al. Potent and selective inhibitors of Helicobacter pylori glutamate racemase (MurI): pyridodiazepine amines. Bioorg. Med. Chem. Lett.19 (3), 930–936 (2009).
- Basarab GS , HillP, EyermannCJ, GowravaramM, KäckH, OsimoniE . Design of inhibitors of Helicobacter pylori glutamate racemase asselective antibacterial agents: incorporation of imidazoles onto a corepyrazolopyrimidinedione scaffold to improve bioavailabilty. Bioorg. Med. Chem. Lett.22 (17), 5600–5607 (2012).
- de Jonge BLM , KutschkeA, NewmanJV, RooneyMT, YangW, CederbergC . Pyridodiazepine amines are selective therapeutic agents for Helicobacter pylori by suppressing growth through inhibition of glutamate racemase but are predicted to require continuous elevated levels in plasma to achieve clinical efficacy. Antimicrob. Agents Chemother.59 (4), 2337–2342 (2015).
- Cheng C-S , JiaK-F, ChenT, ChangS-Y, LinM-S, YinH-S . Experimentally validated novel inhibitors of Helicobacter pylori phosphopantetheine adenylyltransferase discovered by virtual high-throughput screening. PLoS ONE8 (9), e74271 (2013).
- Azizian H , NabatiF, SharifiA, SiavoshiF, MahdaviM, AmanlouM . Large-scale virtual screening for the identification of new Helicobacter pylori urease inhibitor scaffolds. J. Mol. Model.18 (7), 2917–2927 (2012).
- Xiao Z-P , ShiD-H, LiH-Q, ZhangL-N, XuC, ZhuH-L . Polyphenols based on isoflavones as inhibitors of Helicobacter pylori urease. Bioorg. Med. Chem.15 (11), 3703–3710 (2007).
- Macegoniuk K , GrelaE, PalusJet al. 1,2-Benzisoselenazol-3(2H)-one derivatives as a new class of bacterial urease inhibitors. J. Med. Chem.59 (17), 8125–8133 (2016).
- You ZL , NiLL, ShiDH, BaiS . Synthesis, structures, and urease inhibitory activities of three copper(II) and zinc(II) complexes with 2-{[2-(2-hydroxyethylamino)ethylimino]methyl}-4-nitrophenol. Europ. J. Med. Chem.45 (7), 3196–3199 (2010).
- Rückriemen J , KlemmO, HenleT . Manuka honey (Leptospermum scoparium) inhibits jack bean urease activity due to methylglyoxal and dihydroxyacetone. Food Chem.230, 540–546 (2017).
- Fahey JW , StephensonKK, WadeKL, TalalayP . Urease from Helicobacter pylori is inactivated by sulforaphane and other isothiocyanates. Biochem. Biophys. Res. Commun.435 (1), 1–7 (2013).
- Nishimori I , MinakuchiT, KohsakiTet al. Carbonic anhydrase inhibitors: the beta-carbonic anhydrase from Helicobacter pylori is a new target for sulfonamide and sulfamate inhibitors. Bioorg. Med. Chem. Lett.17 (13), 3585–3594 (2007).
- Buzás GM , SupuranCT . The history and rationale of using carbonic anhydrase inhibitors in the treatment of peptic ulcers. In memoriam Ioan Puşcaş (1932–2015). J. Enzyme Inhib. Med. Chem.31 (4), 527–533 (2016).
- Maresca A , VulloD, ScozzafavaA, SupuranCT . Inhibition of the alpha- and beta-carbonic anhydrases from the gastric pathogen Helicobacter pylori with anions. J. Enzyme Inhib. Med. Chem.28 (2), 388–391 (2013).
- Ménard R , SchoenhofenIC, TaoLet al. Small-molecule inhibitors of the pseudaminic acid biosynthetic pathway: targeting motility as a key bacterial virulence factor. Antimicrob. Agents Chemother.58 (12), 7430–7440 (2014).
- Barry DP , AsimM, LeimanDAet al. Difluoromethylornithine is a novel inhibitor of Helicobacter pylori growth, CagA translocation, and interleukin-8 induction. PLoS ONE6 (2), e17510 (2011).
- Hilleringmann M , PansegrauW, DoyleMet al. Inhibitors of Helicobacter pylori ATPase Cag-alpha block CagA transport and cag virulence. Microbiology 152 (Pt 10), 2919–2930 (2006).
- Sayer JR , WalldénK, PesnotTet al. 2- and 3-substituted imidazo[1,2-a]pyrazines as inhibitors of bacterial type IV secretion. Bioorg. Med. Chem.22 (22), 6459–6470 (2014).
- Löwer M , GeppertT, SchneiderP, HoyB, WesslerS, SchneiderG . Inhibitors of Helicobacter pylori protease HtrA found by “virtual ligand” screening combat bacterial invasion of epithelia. PLoS ONE6 (3), e17986 (2011).
- Perna AM , RodriguesT, SchmidtTPet al. Fragment-based de novo design reveals a small-molecule inhibitor of Helicobacter pylori HtrA. Angew. Chem. Int. Ed. Engl.54 (35), 10244–10248 (2015).
- Moonens K , GideonssonP, SubediSet al. Structural insights into polymorphic ABO glycan binding by Helicobacter pylori. Cell Host Microbe.19 (1), 55–66 (2016).
- Gotoh A , AkamatsuT, ShimizuTet al. Additive effect of pronase on the efficacy of eradication therapy against Helicobacter pylori. Helicobacter7 (3), 183–191 (2002).
- Abut E , YaşarB, GüveliHet al. Effect of the mucolytic erdosteine on the success rate of PPI-based first-line triple therapy for Helicobacter pylori eradication: a prospective, double-blind, randomized, placebo-controlled study. Scand. J. Gastroenterol.45 (6), 677–683 (2010).
- Bartlett MS , ShawM, NavaranP, SmithJW, QueenerSF . Evaluation of potent inhibitors of dihydrofolate reductase in a culture model for growth of Pneumocystis carinii. Antimicrob. Agents Chemother.39 (11), 2436–2441 (1995).
- Karita M , EtterbeekML, ForsythMH, TummuruMK, BlaserMJ . Characterization of Helicobacter pylori dapE and construction of a conditionally lethal dapE mutant. Infect. Immun.65 (10), 4158–4164 (1997).
- Basarab GS , HillPJ, RastagarA, WebbornPJH . Design of Helicobacter pylori glutamate racemase inhibitors as selective antibacterial agents: a novel pro-drug approach to increase exposure. Bioorg. Med. Chem. Lett.18 (16), 4716–4722 (2008).
- de Jonge BLM , KutschkeA, Uria-NickelsenM, KampHD, MillsSD . Pyrazolopyrimidinediones are selective agents for Helicobacter pylori that suppress growth through inhibition of glutamate racemase (MurI). Antimicrob. Agents Chemother.53 (8), 3331–3336 (2009).
- Keating TA . Resistance mechanism to an uncompetitive inhibitor of a single-substrate, single-product enzyme: a study of Helicobacter pylori glutamate racemase. Future Med. Chem.5 (11), 1203–1214 (2013).
- Ha NC , OhST, SungJY, ChaKA, LeeMH, OhBH . Supramolecular assembly and acid resistance of Helicobacter pylori urease. Nat. Struct. Biol.8 (6), 505–509 (2001).
- Kosikowska P , BerlickiŁ . Urease inhibitors as potential drugs for gastric and urinary tract infections: a patent review. Expert Opin. Ther. Pat.21 (6), 945–957 (2011).
- Phillips K , MunsterDJ, AllardyceRA, BagshawPF . Antibacterial action of the urease inhibitor acetohydroxamic acid on Helicobacter pylori. J. Clin. Pathol.46 (4), 372–373 (1993).
- Khan KM , AliM, WadoodAet al. Molecular modeling-based antioxidant arylidene barbiturates as urease inhibitors. J. Mol. Graph. Model.30, 153–156 (2011).
- Amtul Z , RahmanA-U, SiddiquiRA, ChoudharyMI . Chemistry and mechanism of urease inhibition. Curr. Med. Chem.9 (14), 1323–1348 (2002).
- Kühler TC , FryklundJ, BergmanNA, WeilitzJ, LeeA, LarssonH . Structure–activity relationship of omeprazole and analogues as Helicobacter pylori urease inhibitors. J. Med. Chem.38 (25), 4906–4916 (1995).
- Macegoniuk K , KowalczykR, RudzińskaA, PsurskiM, WietrzykJ, BerlickiŁ . Potent covalent inhibitors of bacterial urease identified by activity–reactivity profiling. Bioorganic Med. Chem. Lett.27 (6), 1346–1350 (2017).
- Mazzei L , CianciM, MusianiF, CiurliS . Inactivation of urease by 1,4-benzoquinone: chemistry at the protein surface. Dalton Trans.45 (13), 5455–5459 (2016).
- Sj⊘str⊘m JE , KühlerT, LarssonH . Basis for the selective antibacterial activity in vitro of proton pump inhibitors against Helicobacter spp. Antimicrob. Agents Chemother.41 (8), 1797–1801 (1997).
- de Reuse H , VinellaD, CavazzaC . Common themes and unique proteins for the uptake and trafficking of nickel, a metal essential for the virulence of Helicobacter pylori. Front. Cell Infect. Microbiol.3, 94 (2013).
- van Vliet AHM , ErnstFD, KustersJG . NikR-mediated regulation of Helicobacter pylori acid adaptation. Trends Microbiol.12 (11), 489–494 (2004).
- Park JB , ImamuraL, KobashiK . Kinetic studies of Helicobacter pylori urease inhibition by a novel proton pump inhibitor, rabeprazole. Biol. Pharm. Bull.19 (2), 182–187 (1996).
- Hassan STS , ŠudomováM . The development of urease inhibitors: what opportunities exist for better treatment of Helicobacter pylori infection in children?Children4 (1), (2017).
- Hassan STS , ŽemličkaM . Plant-derived urease inhibitors as alternative chemotherapeutic agents. Arch. Pharm.349 (7), 507–522 (2016).
- Amin M , AnwarF, NazF, MehmoodT, SaariN . Anti-Helicobacter pylori and urease inhibition activities of some traditional medicinal plants. Molecules18 (2), 2135–2149 (2013).
- Ramsay KST , WafoP, AliZet al. Chemical constituents of Stereospermum acuminatissimum and their urease and α-chymotrypsin inhibitions. Fitoterapia83 (1), 204–208 (2012).
- Shin J-E , KimJ-M, BaeE-A, HyunY-J, KimD-H . In vitro inhibitory effect of flavonoids on growth, infection and vacuolation of Helicobacter pylori. Planta Med.71 (3), 197–201 (2005).
- Hawtin PR , DelvesHT, NewellDG . The demonstration of nickel in the urease of Helicobacter pylori by atomic absorption spectroscopy. FEMS Microbiol. Lett.61 (1), 51–54 (1991).
- Johnson RC , HuHQ, MerrellDS, MaroneyMJ . Dynamic HypA zinc site is essential for acid viability and proper urease maturation in Helicobacter pylori. Metallomics7 (4), 674–682 (2015).
- Yang X , LiH, ChengT, XiaW, LaiY-T, SunH . Nickel translocation between metallochaperones HypA and UreE in Helicobacter pylori. Metallomics6 (9), 1731–1736 (2014).
- Rowinska-Zyrek M , WitkowskaD, BielinskaS, KamyszW, KozlowskiH . The -Cys-Cys- motif in Helicobacter pylori's Hpn and HspA proteins is an essential anchoring site for metal ions. Dalton Trans.40 (20), 5604–5610 (2011).
- Rowinska-Zyrek M , WitkowskaD, ValensinD, KamyszW, KozlowskiH . The C terminus of HspA – a potential target for native Ni(II) and Bi(III) anti-ulcer drugs. Dalton Trans.39 (25), 5814–5826 (2010).
- Ge R , SunX, GuQet al. A proteomic approach for the identification of bismuth-binding proteins in Helicobacter pylori. J. Biol. Inorg. Chem.12 (6), 831–842 (2007).
- Nishimori I , OnishiS, TakeuchiH, SupuranCT . The alpha and beta classes carbonic anhydrases from Helicobacter pylori as novel drug targets. Curr. Pharm. Des.14 (7), 622–630 (2008).
- Modak JK , ModakhJK, LiuYC, MachucaMA, SupuranCT, RoujeinikovaA . Structural basis for the inhibition of Helicobacter pyloriα-carbonic anhydrase by sulfonamides. PLoS ONE10 (5), e0127149 (2015).
- Guerry P , EwingCP, SchirmMet al. Changes in flagellin glycosylation affect Campylobacter autoagglutination and virulence. Mol. Microbiol.60 (2), 299–311 (2006).
- Schoenhofen IC , McNallyDJ, BrissonJ-R, LoganSM . Elucidation of the CMP-pseudaminic acid pathway in Helicobacter pylori: synthesis from UDP-N-acetylglucosamine by a single enzymatic reaction. Glycobiology16 (9), 8C–14C (2006).
- Bakal SN , BereswillS, HeimesaatMM . Finding novel antibiotic substances from medicinal plants – antimicrobial properties of Nigella sativa directed against multidrug-resistant bacteria. Eur. J. Microbiol. Immunol. (Bp).7 (1), 92–98 (2017).
- Kouitcheu Mabeku LB , Eyoum BilleB, TchouangueuTF, NguepiE, LeundjiH . Treatment of Helicobacter pylori infected mice with Bryophyllum pinnatum, a medicinal plant with antioxidant and antimicrobial properties, reduces bacterial load. Pharm. Biol.55 (1), 603–610 (2017).
- Zhou J-T , LiC-L, TanL-Het al. Inhibition of Helicobacter pylori and its associated urease by palmatine: investigation on the potential mechanism. PLoS ONE12 (1), e0168944 (2017).
- Zaidi SF , MuhammadJS, UsmanghaniK, SugiyamaT . Review: pharmacological ins and outs of medicinal plants against Helicobacter pylori: a review. Pak. J. Pharm. Sci.28 (3 Suppl.), 1171–1176 (2015).
- Ayala G , Escobedo-HinojosaWI, de la Cruz-HerreraCF, RomeroI . Exploring alternative treatments for Helicobacter pylori infection. World J. Gastroenterol.20 (6), 1450–1469 (2014).
- Seyyedmajidi M , AhmadiA, HajiebrahimiSet al. Addition of cranberry to proton pump inhibitor-based triple therapy for Helicobacter pylori eradication. J. Res. Pharm. Pract.5 (4), 248–251 (2016).
- Yanaka A . Sulforaphane enhances protection and repair of gastric mucosa against oxidative stress in vitro, and demonstrates anti-inflammatory effects on Helicobacter pylori-infected gastric mucosae in mice and human subjects. Curr. Pharm. Des.17 (16), 1532–1540 (2011).
- Fahey JW , HaristoyX, DolanPMet al. Sulforaphane inhibits extracellular, intracellular, and antibiotic-resistant strains of Helicobacter pylori and prevents benzo[a]pyrene-induced stomach tumors. Proc. Natl Acad. Sci. USA99 (11), 7610–7615 (2002).
- Nontakham J , CharoenramN, UpamaiW, TaweechotipatrM, SuksamrarnS . Anti-Helicobacter pylori xanthones of Garcinia fusca. Arch. Pharm. Res.37 (8), 972–977 (2014).
- Stoicov C , SaffariR, HoughtonJ . Green tea inhibits Helicobacter growth in vivo and in vitro. Int. J. Antimicrob. Agents33 (5), 473–478 (2009).
- Ruggiero P , RossiG, TombolaFet al. Red wine and green tea reduce H pylori- or VacA-induced gastritis in a mouse model. World J. Gastroenterol.13 (3), 349–354 (2007).
- da Silva Junior IF , BalogunSO, de OliveiraRG, DamazoAS, Martins DT deO . Piper umbellatum L.: a medicinal plant with gastric-ulcer protective and ulcer healing effects in experimental rodent models. J. Ethnopharmacol.192, 123–131 (2016).
- Lee J-H , ShimJS, ChungM-S, LimS-T, KimKH . In vitro anti-adhesive activity of green tea extract against pathogen adhesion. Phytother. Res.23 (4), 460–466 (2009).
- Inngjerdingen KT , ThöleC, DialloD, PaulsenBS, HenselA . Inhibition of Helicobacter pylori adhesion to human gastric adenocarcinoma epithelial cells by aqueous extracts and pectic polysaccharides from the roots of Cochlospermum tinctorium A. Rich. and Vernonia kotschyana Sch. Bip. ex Walp. Fitoterapia95, 127–132 (2014).
- Wittschier N , FallerG, HenselA . Aqueous extracts and polysaccharides from liquorice roots (Glycyrrhiza glabra L.) inhibit adhesion of Helicobacter pylori to human gastric mucosa. J. Ethnopharmacol.125 (2), 218–223 (2009).
- Boyanova L , IlievaJ, GergovaG, VladimirovB, NikolovR, MitovI . Honey and green/black tea consumption may reduce the risk of Helicobacter pylori infection. Diagn. Microbiol. Infect. Dis.82 (1), 85–86 (2015).
- Manyi-Loh CE , ClarkeAM, MunzheleleT, GreenE, MkwetshanaNF, NdipRN . Selected South African honeys and their extracts possess in vitro anti-Helicobacter pylori activity. Arch. Med. Res.41 (5), 324–331 (2010).
- Patel A , ShahN, PrajapatiJB . Clinical application of probiotics in the treatment of Helicobacter pylori infection - a brief review. J. Microbiol. Immunol. Infect.47 (5), 429–437 (2014).
- Gill HS . Probiotics to enhance anti-infective defences in the gastrointestinal tract. Best Pract. Res. Clin. Gastroenterol.17 (5), 755–773 (2003).
- Lorca GL , WadströmT, ValdezGF, LjunghA . Lactobacillus acidophilus autolysins inhibit Helicobacter pylori in vitro. Curr. Microbiol.42 (1), 39–44 (2001).
- Pinchuk IV , BressollierP, VerneuilBet al. In vitro anti-Helicobacter pylori activity of the probiotic strain Bacillus subtilis 3 is due to secretion of antibiotics. Antimicrob. Agents Chemother.45 (11), 3156–3161 (2001).
- Gomi A , Harima-MizusawaN, Shibahara-SoneH, KanoM, MiyazakiK, IshikawaF . Effect of Bifidobacterium bifidum BF-1 on gastric protection and mucin production in an acute gastric injury rat model. J. Dairy Sci.96 (2), 832–837 (2013).
- Chenoll E , CasinosB, BatallerEet al. Novel probiotic Bifidobacterium bifidum CECT 7366 strain active against the pathogenic bacterium Helicobacter pylori. Appl. Environ. Microbiol.77 (4), 1335–1343 (2011).
- Ruer S , PinotsisN, SteadmanD, WaksmanG, RemautH . Virulence-targeted antibacterials: concept, promise, and susceptibility to resistance mechanisms. Chem. Biol. Drug Des.86 (4), 379–399 (2015).
- Prinz C , SchönigerM, RadRet al. Key importance of the Helicobacter pylori adherence factor blood group antigen binding adhesin during chronic gastric inflammation. Cancer Res.61 (5), 1903–1909 (2001).
- Javaheri A , KruseT, MoonensKet al. Helicobacter pylori adhesin HopQ engages in a virulence-enhancing interaction with human CEACAMs. Nat. Microbiol.2, 16189 (2016).
- Ishijima N , SuzukiM, AshidaHet al. BabA-mediated adherence is a potentiator of the Helicobacter pylori type IV secretion system activity. J. Biol. Chem.286 (28), 25256–25264 (2011).
- Hilleringmann M , PansegrauW, DoyleMet al. Inhibitors of Helicobacter pylori ATPase Cagalpha block CagA transport and cag virulence. Microbiology152 (10), 2919–2930 (2006).
- Doua F , BoaFY, SchechterPJet al. Treatment of human late stage gambiense trypanosomiasis with α-difluoromethylornithine (eflornithine): efficacy and tolerance in 14 cases in côte d'ivoire. Am. J. Trop. Med. Hyg.37 (3), 525–533 (1987).
- Cusumano CK , PinknerJS, HanZet al. Treatment and prevention of urinary tract infection with orally active FimH inhibitors. Sci. Transl. Med.3 (109), 109ra115 (2011).
- Spaulding CN , KleinRD, RuerSet al. Selective depletion of uropathogenic E. coli from the gut by a FimH antagonist. Nature546 (7659), 528–532 (2017).
- Ilver D . Helicobacter pylori adhesin binding fucosylated histo-blood group antigens revealed by retagging. Science279, 373–377 (1998).
- Gustafsson A , HultbergA, SjöströmRet al. Carbohydrate-dependent inhibition of Helicobacter pylori colonization using porcine milk. Glycobiology16 (1), 1–10 (2006).
- Mahdavi J , SondénB, HurtigMet al. Helicobacter pylori SabA adhesin in persistent infection and chronic inflammation. Science297 (5581), 573–578 (2002).
- O'Mahony R , Al-KhtheeriH, WeerasekeraDet al. Bactericidal and anti-adhesive properties of culinary and medicinal plants against Helicobacter pylori. World J. Gastroenterol.11 (47), 7499–7507 (2005).
- Cammarota G , SanguinettiM, GalloA, PosteraroB . Review article: biofilm formation by Helicobacter pylori as a target for eradication of resistant infection. Aliment. Pharmacol. Ther.36 (3), 222–230 (2012).
- Gurbuz AK , OzelAM, OzturkR, YildirimS, YazganY, DemirturkL . Effect of N-acetyl cysteine on Helicobacter pylori. South. Med. J.98 (11), 1095–1097 (2005).
- Salama NR , HartungML, MüllerA . Life in the human stomach: persistence strategies of the bacterial pathogen Helicobacter pylori. Nat. Rev. Microbiol.11 (6), 385–399 (2013).
- Hoy B , LöwerM, WeydigCet al. Helicobacter pylori HtrA is a new secreted virulence factor that cleaves E-cadherin to disrupt intercellular adhesion. EMBO Rep.11 (10), 798–804 (2010).
- Tegtmeyer N , MoodleyY, YamaokaYet al. Characterisation of worldwide Helicobacter pylori strains reveals genetic conservation and essentiality of serine protease HtrA. Mol. Microbiol.99 (5), 925–944 (2016).
- Hashimoto Y , TsuikiS, NisizawaK, PigmanW . Action of proteolytic enzymes on purified bovine submaxillary mucin. Ann. NY Acad. Sci.106, 233–246 (1963).