Abstract
Pimitespib (TAS-116) is the first heat shock protein 90 (HSP90) inhibitor approved in Japan, and it is indicated for the treatment of gastrointestinal stromal tumors (GIST) that have progressed after treatment with imatinib, sunitinib and regorafenib. This review describes the preclinical and clinical research with pimitespib, including its mechanism of action, pharmacokinetics, clinical antitumour activity and safety. In a phase III study, pimitespib significantly prolonged progression-free survival compared with placebo (median 2.8 vs 1.4 months; hazard ratio 0.51; 95% CI 0.30–0.87; p = 0.006). Common treatment-related adverse events were diarrhoea, decreased appetite, increase in serum creatinine, malaise, nausea and eye disorders. The efficacy and safety of pimitespib are being investigated in other tumour types and in combination with other anticancer therapies.
Plain Language Summary
A summary of the new anticancer drug, pimitespib, for treating gastrointestinal stromal tumors that are unresponsive to initial treatments & have spread to other parts of the body
What is this article about?
This article provides information about pimitespib, a drug that recently became available in Japan for the treatment of advanced gastrointestinal stromal tumors, or ‘GISTs’. GISTs are a type of cancer found in the gastrointestinal tract, and those that are considered ‘advanced’ have stopped responding to other treatments and have spread to other parts of the body.
What have studies shown?
Pimitespib works in a way unlike other drug treatments for cancer – it inhibits a protein called heat shock protein 90, and this stops cancer cells from developing and growing. Pimitespib is taken by mouth. Studies in Japanese patients with advanced GISTs showed an increase in the time taken for the cancer to progress further and in the length of time that patients survived among those treated with pimitespib, compared with patients who did not receive the drug. These studies also found that pimitespib was not associated with serious side effects, although diarrhoea occurred frequently. Eye disorders developed in some patients, but they could be managed by interrupting or stopping treatment with pimitespib. Pimitespib is also being studied for the treatment of other cancers, alone and in combination with other anticancer drugs.
What conclusions can be made from these studies?
There are very few treatments available for patients with advanced GISTs and, therefore, pimitespib is an important new option for such patients in Japan. If the results of ongoing studies are positive, pimitespib may become a treatment option for a wider range of cancer patients in the future.
Tweetable abstract
Check out our latest review examining the therapeutic potential and safety of the HSP90 inhibitor #pimitespib for advanced #gastrointestinalstromaltumors and other forms of cancer.
Heat shock proteins (HSP) are molecular chaperones involved in protein folding, and they contribute to a variety of cellular processes [Citation1]. HSP expression is increased in a range of human tumour cells [Citation2], where HSPs contribute to cancer cell homeostasis by regulating the formation and stability of oncogenic proteins, and preventing their degradation [Citation3].
HSPs are named according to their molecular weight; HSP60, HSP70 and HSP90 are the most well studied. The HSP90 family consists of various members that are present in different cellular compartments, including HSP90α and HSP90β (found in the cytoplasm), 94-kDa glucose-regulated protein ([GRP94]; found in the endoplasmic reticulum), and tumour necrosis factor receptor-associated protein 1 ([TRAP-1]; found in the mitochondria) [Citation4]. Many of the client proteins of HSP90 are signal transducers involved in cellular growth and control, and have been identified as cancer-related proteins necessary for tumour development, including v-kit Hardy-Zuckerman 4 feline sarcoma viral oncogene homologue (KIT), platelet-derived growth factor receptor-α (PDGFRA) and v-Raf murine sarcoma viral oncogene homolog B1 (BRAF; ) [Citation1,Citation5,Citation6]. In general, mutated tumour drivers are known to be highly dependent on HSP90 for their stability [Citation7]. HSP90 inhibition, therefore, represents a rational approach to the treatment of tumors associated with mutations of these proteins, including gastrointestinal stromal tumors (GISTs).
Table 1. Cancer-associated proteins that are clients of heat shock protein 90 chaperones.
GISTs are soft-tissue sarcomas of the gastrointestinal (GI) tract that develop from precursors of the interstitial cells of Cajal in the myenteric plexus, which regulate and maintain GI motility [Citation8]. GISTs most commonly develop in the stomach (60–65% of cases) and small intestine (20–35% of cases), but may also occur (albeit far less frequently) in the oesophagus, colon and rectum [Citation8]. Approximately 20–25% of gastric GISTs and 40–50% of small intestinal GISTs are clinically malignant [Citation9].
Surgery is the standard treatment for localised disease; however, relapse after surgical resection is common [Citation10–12] (although the risk of relapse is dependent on the location, size and mitotic index of the tumour [Citation13]). Further, advanced or metastatic GIST is poorly responsive to standard chemotherapy and radiotherapy.
Pimitespib (TAS-116; ) is the first HSP90 inhibitor to achieve regulatory approval in Japan, where it is currently indicated for the treatment of GIST that has progressed after treatment with imatinib, sunitinib and regorafenib [Citation14]. Pimitespib is also undergoing clinical evaluation for the treatment of GIST after imatinib failure and for other solid tumors.
Previous HSP90 inhibitors, including tanespimycin, retaspimycin hydrochloride (IPI-504), ganetespib (STA-9090), BIIB021 (CNF 2024), luminespib (NVP-AUY922) and onalespib (AT13387), have been investigated as potential treatments for GIST and other tumors, but none have been approved for GISTs, despite some reaching phase III clinical evaluation [Citation3]. The principal reasons that development of these early HSP90 inhibitors was halted were limited efficacy and the development of dose-limiting or serious toxicity, including hepatic, neurological and ocular toxicity [Citation15].
Unlike the previous HSP90 inhibitors, pimitespib has shown antitumour activity with an acceptable toxicity profile [Citation16]. The aim of the current narrative review is to describe the mechanism of action, pharmacokinetics, antitumour activity and clinical efficacy of pimitespib, as well as its likely place in the treatment of GIST.
Systemic therapy for GIST
With standard treatments, the median survival was 18–24 months after diagnosis of advanced or metastatic GIST [Citation17], and the 5-year survival rate was approximately 12% [Citation18]. However, genomic studies identified the molecular mechanisms underlying the development of GIST, paving the way for the development of targeted therapies. The most common mutations in GIST are gain-of-function mutations in exon 9 or 11 of the KIT gene [Citation19], and the next most common are mutations in the PDGFRA gene [Citation20]. These mutations result in ligand-independent activation of receptor tyrosine kinases.
Approximately 15% of GISTs do not have a mutation in either KIT or PDGFRA but have other genetic alterations; these may include succinate dehydrogenase (SDH A–D) mutations, neurotrophic tyrosine receptor kinase (NTRK) fusions or RAS pathway-related gene (BRAF, neurofibromatosis type 1 [NF1] or HRAS/NRAS/KRAS) mutations, although the prevalence of these mutations is reported to be very rare (<3%) [Citation8]. Imatinib, an inhibitor of KIT/PDGFRA tyrosine kinase, was the first targeted therapy approved for the treatment of metastatic and/or inoperable malignant GISTs; it is now recommended in international guidelines as first-line therapy to reduce the risk of GIST relapse in high-risk patients, and for locally advanced, inoperable and metastatic disease [Citation10–12,Citation21].
Patients with imatinib-refractory advanced or metastatic GIST harbouring a PDGFRA mutation may respond to other tyrosine kinase inhibitors (TKI) such as sunitinib, regorafenib, avapritinib, or ripretinib [Citation22], and international guidelines recommended sunitinib as second-line, regorafenib as third-line and ripretinib as fourth-line treatment for GISTs (although ripretinib is not approved for use in Japan) [Citation10,Citation12,Citation13,Citation23]. GIST with PDGFRA exon 18 mutations such as D842V are insensitive to imatinib, and the preferred first-line therapy in patients with these rare tumors is avapritinib [Citation13].
Treatment options are limited for GIST patients who do not respond to third-line therapy [Citation24]. For the small proportion of GIST patients with rare mutations, treatment options may include agents targeting their particular genetic alteration, such as larotrectinib or entrectinib for advanced/refractory GIST with NTRK fusions [Citation25,Citation26]. Pimitespib is the first of a new class of agents (HSP90 inhibitors) for the treatment of advanced GIST in patients who have previously received imatinib, sunitinib and regorafenib.
Mechanism of action of pimitespib
HSP90 is a dimer composed of two homologous monomers, each with three main conserved domains – an N-terminal domain, a C-terminal domain and a middle domain – connected by linkers [Citation4]. The N-terminal domain is the binding site for adenosine triphosphate (ATP) [Citation4]. After ATP binding, the HSP90 chaperone causes a conformational change in the client polypeptide chain, which transforms it into a functional protein [Citation5], as shown in . HSP90 inhibitors block the conformational change, resulting in a non-functional client protein that undergoes degradation through the ubiquitin-proteasome pathway [Citation5].
ATP: Adenosine triphosphate; CDK: Cyclin-dependent kinase; EGFR: Epidermal growth factor receptor; HER2: Human epidermal growth factor receptor 2; HIF-1: Hypoxia-inducible factor 1; HSP 90: Heat shock protein 90; KIT: V-kit Hardy-Zuckerman 4 feline sarcoma viral oncogene homologue; PDGFR: Platelet-derived growth factor receptor.
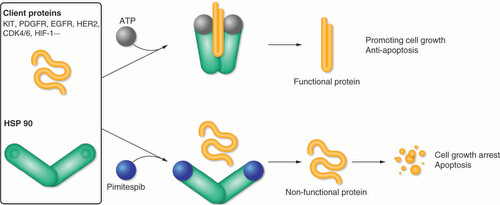
Unlike previous HSP90 inhibitors, pimitespib binds to HSP90 by occupying not only the ATP-binding pocket of the N-terminal domain but also a hydrophobic sub-pocket [Citation27,Citation28]. This unique binding mode of pimitespib leads to selective binding to cytosolic HSP90 α/β, and pimitespib has comparatively little interaction with the highly homologous members of the HSP90 family, TRAP1 (in mitochondria) and GRP94 (in the endoplasmic reticulum) [Citation29]. This is in contrast to other HSP90 inhibitors, which bind with varying affinity to all HSP90 proteins () [Citation29]. Further, pimitespib does not inhibit other ATPases including HSP70 [Citation29].
Table 2. Binding affinity of HSP90 inhibitors for proteins in the HSP90 family.
Pimitespib is orally active and has demonstrated antitumour activity in animal models of GIST, gastric cancer, lung cancer and leukaemia [Citation29–33]. Pimitespib has also shown synergistic antitumour activity when used with other agents, including bortezomib, dabrafenib, selumetinib and tipifarnib, in in vitro and in vivo models of multiple myeloma [Citation34,Citation35]. There are preclinical data suggesting that HSP90 inhibitors, including pimitespib, may also have synergistic anticancer effects when administered with cyclin-dependent kinase (CDK) 4/6 inhibitors [Citation36] or immune checkpoint inhibitors [Citation37,Citation38].
Pimitespib induced apoptosis and inhibited cancer cell growth in vitro in GIST cancer cell lines with KIT activation; the inhibition of cancer cell proliferation was seen in imatinib-resistant GIST cell lines with KIT secondary mutations D820Y or D820V, as well as in an imatinib-naive line [Citation32]. Pimitespib reduced the levels of autophosphorylated KIT in intracellular compartments, particularly the trans face of the Golgi apparatus, where mutated KIT tends to accumulate in GIST cells.
In vivo antitumour activity of pimitespib was also seen in murine xenograft models of GIST tumors, including imatinib-resistant tumour models, with significant reduction in tumour size compared with vehicle-treated animals, but no significant body weight loss [Citation32]. Consistent with the in vitro results, pimitespib induced apoptosis and inhibited proliferation of cancer cells even in xenograft tumors. In a murine model of caecal GIST caused by KIT-D820Y mutation, oral pimitespib significantly reduced tumour volume compared with vehicle-treated control animals [Citation31]. Pimitespib significantly inhibited KIT phosphorylation within the tumors, and suppressed downstream signalling pathways, including phosphoinositol-3-kinase (PI3K)/AKT, mitogen-activated protein kinase (MAPK)/ERK, and the Janus kinase (JAK)-signal transducer and activator of transcription (STAT) pathway.
Similar effects were seen in a murine xenograft model of human epidermal growth factor receptor 2 (HER2)-expressing gastric cancer; treatment with pimitespib down-regulated levels of HER2, HER3 and AKT, and inhibited the PI3K/AKT and MAPK/ERK pathways [Citation29]. Pimitespib also enhanced radiosensitivity in human cancer cells and human tumour xenografts [Citation39].
Preclinical research of ocular toxicity
One of the most notable HSP90-related adverse events (AE), universally observed in the clinic but to differing degrees, is ocular toxicity. Preclinical studies have indicated that oral pimitespib is likely to show favourable tolerability, especially in terms of ocular toxicities. First, tumour-bearing nude rat models showed that pimitespib was highly distributed, but preferentially retained in tumors rather than plasma, and displayed much greater distribution to the tumour than the retina (tumour to retina ratio of 5.7, compared with between 0.7 and 2.3 for other tested HSP90 inhibitors, alvespimycin, ganetespib, SNX-5422 and luminespib) [Citation29]. Therefore, the retinal distribution of pimitespib was much lower than that of luminespib (), an HSP90 inhibitor that was associated with ocular toxicity in early clinical trials [Citation29].
iv.: Intravenously; PO: Orally.
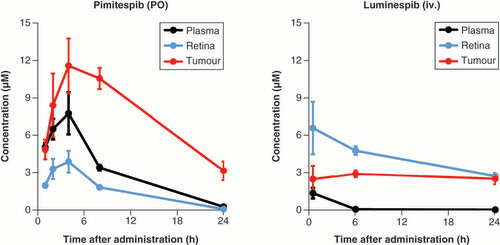
Second, in Sprague Dawley rats without tumors, oral pimitespib did not show any detectable ocular toxicity at doses with antitumour activity, while luminespib caused marked degeneration and disarrangement of the outer nuclear layer of the retina and induced photoreceptor cell death [Citation29].
In addition, in vitro studies indicate that pimitespib is much less cytotoxic in retinal pigment cells compared with the first-generation HSP90 inhibitor geldanamycin [Citation40].
Pharmacokinetics of pimitespib
Animal studies indicated that pimitespib was well absorbed after oral administration and had good bioavailability [Citation29]. In addition, pimitespib shows high tumour tissue penetration, with minimal distribution to retinal tissue as described above () [Citation29].
Systemic exposure increased dose proportionally in the initial phase I study in patients with cancer. There seemed to be no ethnic differences in pharmacokinetics between Japanese and Caucasian patients after multiple-dose administration of pimitespib [Citation16]. The median time to peak plasma concentration after oral administration of pimitespib is 2.48 hours, indicating rapid absorption [Citation41]. Absorption appears to be enhanced by food, as postprandial administration of pimitespib is associated with a higher maximal concentration (Cmax) and area under the concentration-time curve compared with fasting administration [Citation42]. In patients with GI tumors, drug pharmacokinetics can be altered by the effects of the tumor and cancer treatment on the GI tract through changes in GI anatomy (after surgery), gastric pH or emptying, colonic transit time, or the microbiome [Citation43]. The bioavailability of pimitespib tends to be reduced in patients who have had a total gastrectomy, but not in those who have had a partial gastrectomy, compared with patients who have had no gastric resection [Citation41].
Efficacy & safety of pimitespib in GIST
Phase I trial
The first-in-human study with pimitespib used a dose-escalation and dose-expansion design and enrolled patients with advanced solid tumors in Japan and the UK [Citation16]. 36 patients received pimitespib in the dose-escalation phase (three patients had GIST); pimitespib was administered orally, in 21-day cycles, at doses ranging from 4.8 to 150.5 mg/m2 once daily or 107.5 to 295.0 mg/m2 every other day. Two dosing regimens, once daily and every other day, were evaluated in 25 patients (four with GIST) during the dose-expansion phase: 160 mg once daily for 5 days then 2 days off per week, or 340.0 mg every other day.
Across all dose levels, pimitespib dose-dependently inhibited HSP90, as shown by levels of HSP70 expression in peripheral blood mononuclear cells. 60 patients could be evaluated for tumour response across both the dose-escalation and -expansion phases; three partial responses (PR) were seen, and 13 patients had stable disease (SD) for ≥12 weeks, for a disease control rate (DCR) of 27%. Of the seven GIST patients in the study, one had PR and one had SD, for a DCR of 28.6% among these patients. One of the PRs was in a patient with GIST and no detectable KIT mutation who received 150.5 mg/m2 once daily initially and then 107.5 mg/m2 once daily from day 42; this patient continued on pimitespib for more than 6 months and their PR lasted for 239 days. The other two PRs were in patients with non-small-cell lung cancer (NSCLC); duration of PR was 173 days in a patient without detectable EGFR and ALK mutations receiving pimitespib 107.5 mg/m2 daily, and 463 days (at the time of data cut-off) in an NSCLC patient with an EGFR exon 19 deletion mutation receiving pimitespib 150.5 mg/m2 every other day. Another patient with GIST had a long period of SD (393 days); at enrolment, this patient had received five prior treatments (including imatinib twice, sunitinib, regorafenib and an investigational drug), and had secondary KIT mutations in exon 17 (D820Y and N822K), which are associated with resistance to imatinib and other TKIs.
In this phase I study, the most common treatment-related AE was diarrhoea, which developed in 12/16 patients (75.0%) receiving pimitespib daily, 15/19 patients (78.9%) receiving the 5 days on/2 days off schedule, and 24/26 patients (92.3%) receiving pimitespib every other day [Citation16].
Grade ≥3 AEs were diarrhoea (n = 4 [6.6%]), aspartate aminotransferase (AST) increased (n = 4 [6.6%]), anorexia (n = 3 [4.9%]), eye disorders (n = 2 [3.3%]), alanine aminotransferase (ALT) increased (n = 2 [3.3%]), platelet count decrease (n = 2 [3.3%]), nausea (n = 1 [1.6%]), alkaline phosphatase (ALP) increased (n = 1 [1.6%]) and haemoglobin present in urine (n = 1 [1.6%]). Grade 3 diarrhoea developed in one patient during daily treatment with 107.5 mg/m2/day, in one during 5 days on/2 days off with 160 mg, and in two receiving 295 mg/m2 every other day.
Eye disorders occurring during pimitespib were night blindness (n = 13), blurred vision (n = 3) and visual impairment (n = 3). Eye disorders of grade ≥2 developed at the maximum tolerated dose (MTD) or maximum administered dose during daily dosing, and at the maximum administered dose (295 mg/m2) during every-other-day dosing. None of the eye disorders were of grade >1 severity when pimitespib was administered using the 5 days on/2 days off or at the MTD of the every-other-day schedule. All eye disorders resolved with interruption or discontinuation of pimitespib.
Based on the efficacy and safety of pimitespib in the phase I study, and the goal of maintaining the lowest effective plasma concentration over the longest tolerated time, the regimen chosen for the phase II study was 160 mg once daily for 5 days followed by 2 days off per week in a 21-day cycle [Citation41].
Phase II trial
The phase II clinical trial was undertaken with pimitespib in 40 Japanese patients with advanced GIST that was refractory to imatinib, sunitinib and regorafenib (JapicCTI-163182) [Citation41]. As described above, the study used a pimitespib regimen of 160 mg once daily for 5 days followed by 2 days off per week in a 21-day cycle until disease progression, unacceptable AEs or withdrawal of consent. The primary end point was centrally assessed progression-free survival (PFS) based on Response Evaluation Criteria in Solid Tumors.
The median duration of treatment of 77.5 days (interquartile range: 42–126 days). Centrally assessed median PFS was 4.4 months (95% CI: 2.8–6.0), and the PFS rate at 12 weeks was 73.4%. The median overall survival (OS) was 11.5 months (95% CI: 7.0–not reached). The DCR was 85.0% (95% CI: 70.2–94.3), all SD, although a PR was confirmed in one patient after the data cut-off date.
Mutations in KIT, PDGFRA and BRAF were detected in 30 (75.0%) patients, with secondary KIT mutations present in 17 patients. Most patients with KIT mutations had SD as their best response. One patient with a PDGFRA mutation (D842V) had SD for 4.1 months, and one with a BRAF mutation (A728T) had SD for 10 months.
All patients receiving pimitespib developed at least one treatment-related AE, 12 (30.0%) developed a serious AE and six (15.0%) serious AEs were treatment-related, but there were no treatment-related deaths. Overall, 21 patients (52.5%) developed a treatment-related grade ≥3 AE. The most common types of treatment-related AEs of any grade were similar to those in the phase I study: diarrhoea (n = 32 [80.0%]), decreased appetite (n = 18 [45.0%]), creatinine increased (n = 17 [42.5%]), nausea (n = 16 [40.0%]), ALT increased (n = 10 [25.0%]), ALP increased (n = 9 [22.5%]) and AST increased (n = 9 [22.5%]; ). Treatment-related grade ≥3 AEs that occurred in at least 5% of patients were diarrhoea (n = 9 [22.5%]), decreased appetite (n = 5 [12.5%]), anaemia (n = 4 [10.0%]), nausea (n = 2 [5.0%]), neutrophil decreased (n = 2 [5.0%]) and hepatobiliary disorders (n = 2 [5.0%]).
Table 3. Key patient characteristics, study end points and treatment-related adverse events seen in the phase II and III studies with pimitespib.
Eight patients (20.0%) had eye disorders, specifically night blindness (n = 4 [10.0%]), vision blurred (n = 3 [7.5%]) and visual impairment (n = 1 [2.5%]). All eye disorders were grade 1, and all eye and GI disorders, including grade 3 diarrhoea, resolved after dose interruption.
Overall, 20 (50.0%) and 32 (80.0%) patients had a dose reduction or treatment interruption due to treatment-related AEs, respectively. Two patients discontinued treatment because of AEs, but these events were not considered to be treatment related [Citation41].
Phase III trial
The phase III trial of pimitespib was called CHAPTER-GIST-301 (JapicCTI-184094), and it included patients with histologically confirmed GIST who were refractory to imatinib, sunitinib and regorafenib [Citation44]. Patients were randomized 2:1 to pimitespib at the schedule used in the phase II study (160 mg once daily for 5 days followed by 2 days off) or placebo, but patients in the placebo arm who developed progressive disease, as assessed by blinded central radiological review (BCRR), could crossover to open-label pimitespib at unblinding.
The primary end point was PFS by BCRR; secondary end points included OS, PFS in patients who crossed over to pimitespib (secondary PFS), health-related quality of life (HRQOL) and safety. Rank-preserving structural failure time (RPSFT) modelling was used to adjust survival for crossover status.
Of the 86 patients enrolled, 58 were randomized to pimitespib and 28 to placebo. Median PFS by BCRR was 2.8 months (95% CI: 1.6–2.9) in the pimitespib group and 1.4 months (95% CI: 0.9–1.8) in the placebo group (hazard ratio [HR] 0.51; 95% CI: 0.30–0.87; p = 0.006, one-sided stratified log-rank test).
Median OS was also longer in pimitespib- than placebo-treated patients, but the difference did not reach statistical significance: 13.8 months (95% CI: 9.2–not reached) with pimitespib versus 9.6 months (95% CI: 5.5–not reached) with placebo (HR 0.63; 95% CI: 0.32–1.21; p = 0.081). 17 of the 28 patients who were initially randomized to placebo later crossed over to pimitespib (60.7%). Secondary PFS in this group was 2.7 months (95% CI: 0.7–4.1). After adjusting for the crossover effect using the RPSFT model, the median OS was 13.8 months (95% CI: 9.2–not reached) with pimitespib and 7.6 months (95% CI: 5.3–14.9) with placebo (HR 0.42; 95% CI: 0.21–0.85; one-sided p = 0.007). A pharmacogenomic analysis found that the efficacy of pimitespib was demonstrated irrespective of KIT mutation status.
The safety profile of pimitespib in the CHAPTER-GIST-301 study was consistent with results of the phase II study (). Grade ≥3 treatment-related AEs that occurred in >10% of patients were diarrhoea (13.8% with pimitespib vs 0 with placebo) and anaemia (5.2% vs 0). The most frequent eye disorder was grade 1 night blindness (n = 8 [13.8%]), which usually resolved, and did not lead to discontinuation or dose modification; the most severe eye disorders were grade 2 (retinal vein occlusion in one patient and one visual impairment in another). Both of these eye disorders resolved in response to pimitespib discontinuation (retinal vein occlusion) or dose interruption/reduction (visual impairment). The incidence of treatment-related AEs leading to permanent discontinuation was low in both treatment groups: n = 3 in the pimitespib group (5.2%) and 0 in the placebo group. There were no treatment-related deaths in either group. Overall, pimitespib was well tolerated, and the safety profile was manageable the with appropriate dose modifications. With respect to HRQOL, no significant differences between pimitespib and placebo were observed for time to deterioration of 10 points in global health status [Citation44].
Conclusion
The approval of pimitespib provides a new treatment option for patients in Japan with advanced GIST once they have relapsed or proven to be refractory to three or more previous lines of TKI therapy. Like ripretinib (which is approved as fourth-line treatment for GIST in Europe and the USA but not Japan) [Citation24], pimitespib has demonstrated superiority to placebo on the primary end point of PFS and a survival benefit in terms of OS in a phase III trial of patients with advanced GIST [Citation44]. It is not possible to directly compare the efficacy of these two fourth-line options for GIST because of differences in the design of the pivotal trials (ripretinib was investigated in an international phase III trial [Citation24], while pimitespib was assessed in a phase III trial conducted exclusively in Japan [Citation44]); however, it appears that the safety profile of the drugs differs, with that of ripretinib being characterised by alopecia and that of pimitespib by diarrhoea.
As described earlier, patients with advanced GIST currently have few treatment options, although other strategies are under investigation such as combinations of TKIs with complementary activity [Citation45] to limit the formation of secondary mutations, or an immune checkpoint inhibitor in combination with existing treatment [Citation46].
Combination therapy that includes pimitespib is also being studied in patients with advanced GIST. The CHAPTER-GIST-101 study (NCT05245968) is a phase I study investigating the best dose regimen for the combination of pimitespib and imatinib. Completion of this study is expected in 2024.
To date, clinical development of pimitespib has focused on GIST [Citation47], but clinical studies with pimitespib are underway in other types of solid tumors, based on the preclinical antitumour activity that it has shown in a range of other solid tumour types, including gastric cancer and lung cancer [Citation29,Citation31,Citation32]. In addition, pimitespib has shown activity in preclinical models of haematological malignancies, including adult T-cell leukaemia [Citation30] and multiple myeloma [Citation34,Citation35]. In the multiple myeloma models, pimitespib showed synergistic antitumour activity in combination with bortezomib, dabrafenib, selumetinib, and tipifarnib [Citation34,Citation35].
A particularly promising strategy appears to be the use of HSP90 inhibitors in combination with immune checkpoint inhibitors. HSP90 controls the stability of the nuclear transcription factor STAT1, which is responsible for the gene expression of immune checkpoint molecules, including programmed cell death-1 (PD-1) [Citation37]. In preclinical cancer models, sub-cytotoxic doses of an HSP90 inhibitor decreased the expression of immune checkpoint proteins, including programmed death ligand 1 (PD-L1) and PD-L2 [Citation38], which suggests that a low dose of HSP90 inhibitor could enhance cancer immunotherapy.
Pimitespib has been investigated in combination with a PD-1 inhibitor, nivolumab, in a phase I study in patients with solid tumors (colorectal cancer [CRC; n = 29], gastric cancer [n = 8], sarcoma [n = 5], NSCLC [n = 1], or melanoma [n = 1]) [Citation48]. The MTD for pimitespib was 160 mg/day for 5 days on/2 days off when used in combination with nivolumab 3 mg/kg every 2 weeks. Objective tumour response was seen in 6/44 patients (13.6%), all of whom were naive to PD-1 or PD-L1 inhibitor therapy. Five of the six responders had CRC (microsatellite stable [MSS] in four and microsatellite instability high [MSI-H] in one) and one had leiomyosarcoma, resulting in an objective response rate of 16% in MSS colorectal cancer. Median PFS was 3.0 months (95% CI: 2.8–4.2) in the overall study group and 3.2 months (95% CI: 2.8–4.4) in MSS colorectal cancer patients who had not received prior anti–PD-1/PD-L1 treatment [Citation48]. Biomarker analysis showed that pimitespib inhibited the activity of regulatory T cells in peripheral blood mononuclear cells and tumour-infiltrating lymphocytes. The safety profile of the pimitespib + nivolumab combination was consistent with data from the monotherapy studies with each agent.
The combination of pimitespib with an investigational PD-1 inhibitor (zimberelimab [AB122]) is also being assessed in a phase I study in patients with advanced or metastatic solid tumors (NCT04999761). In this study, the combination of pimitespib and zimberelimab will be administered to patients with pancreatic ductal carcinoma, metastatic unresectable proficient mismatch repair (pMMR)/MSS CRC, or unresectable metastatic NSCLC without actionable gene alterations. The primary outcome of the phase Ia portion of the study is the percentage of participants who experience a dose-limiting toxicity, while objective response rate is the primary end point of the phase Ib part of the study. The study is expected to be complete in May 2024.
Other potential combinations include pimitespib with CDK4/6 inhibitors, based on data showing synergistic effects in breast cancer and colon cancer cells in vitro [Citation36]. Similarly, the combination of a poly (ADP-ribose) polymerase (PARP) inhibitor with an HSP90 inhibitor (onalespib) has been shown to be feasible and associated with preliminary evidence of antitumour activity in an early clinical study [Citation49]. Additionally, a phase I study of niraparib and pimitespib in patients with solid tumors is currently ongoing in Japan (jRCT2031220179). Combining pimitespib with these molecular-targeted drugs has the potential to be a future treatment option.
Finally, in preclinical models, pimitespib enhanced the sensitivity of human cancer cells and human tumour xenografts to radiation [Citation39], suggesting that it may also have a role as adjuvant treatment with radiotherapy.
In summary, pimitespib is the first HSP90 inhibitor to have received regulatory approval in Japan. It has proven efficacy in patients with advanced GIST who have received at least three prior lines of treatment, offering a treatment approach for patients who currently have few remaining therapeutic options. Pimitespib has an acceptable safety/tolerability profile and AEs including eye disorders are mostly manageable by dose reduction or interruption. Ongoing studies are examining the therapeutic potential of pimitespib in combination with other agents including molecular-targeted agents and immune checkpoint inhibitors for GIST or as treatment for other forms of cancer.
Systemic therapy for gastrointestinal stromal tumors (GIST)
Patients with metastatic or advanced GIST usually receive tyrosine kinase inhibitor (TKI) therapy, but there are few treatment options for patients who do not respond to, or relapse after, TKI treatment.
Mechanism of action of pimitespib
Pimitespib is a heat shock 90 (HSP90) inhibitor, the first agent of this class approved for use in GIST in Japan; it renders cancer-related proteins nonfunctional, leading to their intracellular degradation.
Pharmacokinetics of pimitespib
Pimitespib is rapidly absorbed after oral administration, with high bioavailability and preferentially penetrates the tumour over the retina in preclinical models.
Efficacy & safety of pimitespib in GIST
Pimitespib significantly prolonged progression-free survival and crossover-adjusted overall survival compared with placebo in a phase III study, and it had a manageable tolerability/safety profile with no deterioration in quality of life.
Common treatment-related adverse events in phase II and three clinical studies were diarrhoea, decreased appetite, increase in serum creatinine, malaise, nausea and eye disorders. Eye disorders were consistently grade ≤2 and reversible.
Current & potential place of pimitespib in therapy
Pimitespib is now approved for fourth-line use in Japan and represents an important advance for patients with GIST that has progressed despite prior treatments.
The therapeutic potential of pimitespib is being investigated in a range of solid tumors and haematological malignancies, as monotherapy and in combination with other treatments, including TKIs and immune checkpoint inhibitors.
Author contributions
All authors developed the concept for the review, S Ohkubo searched and analysed the literature, and T Doi, N Yamamoto and S Ohkubo reviewed and revised the paper. All authors approved the final draft of the manuscript for submission.
Financial disclosure
This article was supported by Taiho. T Doi has received research grants from Abbvie, BMS, Boehringer Ingelheim, Chugai Pharma, Daiichi Sankyo, Eisai, IQVIA, Janssen Pharma, Lilly, Merck Biopharma, MSD, Novartis, Pfizer, Sumitomo Dainippon and Taiho; honoraria from AstraZeneca, BMS, Daiichi Sankyo, Rakuten Medical and Ono Pharma; consulting fees from Abbvie, Bayer, Chugai Pharma, Kaken Pharma, Kyowa Kirin, Otsuka Pharma, PRA Health Science, Rakuten Medical, Shionogi, Sumitomo Dainippon, Takeda and Taiho; and has served on advisory boards for Abbvie, Amgen, Astellas Pharma, Bayer, Boehringer Ingelheim, Daiichi Sankyo, Janssen Pharma, MSD and Novartis. N Yamamoto has received research grants from Astellas, Bayer, BMS, Boehringer Ingelheim, Carna Biosciences, Chiome Bioscience Inc., Chugai, Daiichi-Sankyo, Eisai, Genmab, GSK, Janssen Pharma, Kyowa-Hakko Kirin, Lilly, Merck, MSD, Novartis, Ono Pharmaceutical Co., Ltd, Otsuka, Pfizer, Quintiles, Shionogi, Sumitomo Dainippon, Taiho, Takeda and Toray; honoraria from AstraZeneca, BMS, Chugai, Eisai, Ono Pharmaceutical Co., Ltd, Pfizer and Sysmex; and consulting fees from Boehringer Ingelheim, Cimic, Eisai, Otsuka and Takeda. S Ohkubo is a full-time employee of Taiho Pharmaceutical Co., Ltd. The authors have no other relevant affiliations or financial involvement with any organisation or entity with a financial interest in or financial conflict with the subject matter or materials discussed in the manuscript apart from those disclosed.
Competing interests disclosure
The authors have no competing interests or relevant affiliations with any organization or entity with the subject matter or materials discussed in the manuscript. This includes employment, consultancies, honoraria, stock ownership or options, expert testimony, grants or patents received or pending, or royalties.
Writing disclosure
Medical writing support was provided by Catherine Rees on behalf of inScience Communications, Springer Healthcare, and was funded by Taiho Pharmaceuticals.
Open access
This work is licensed under the Attribution-NonCommercial-NoDerivatives 4.0 Unported License. To view a copy of this license, visit http://creativecommons.org/licenses/by-nc-nd/4.0/
Infographic
Download PDF (100.5 KB)Supplementary Material
An infographic accompanies this paper. To view or download this infographic in your browser please click here: https://www.tandfonline.com/doi/suppl/10.2217/FON-2022-1172
References
- Whitesell L, Lindquist SL. HSP90 and the chaperoning of cancer. Nat. Rev. Cancer 5(10), 761–772 (2005).
- Zhang Z, Jing J, Ye Y et al. Characterization of the dual functional effects of heat shock proteins (HSPs) in cancer hallmarks to aid development of HSP inhibitors. Genome Med. 12(1), 101 (2020).
- Serrano C, George S, Valverde C et al. Novel insights into the treatment of imatinib-resistant gastrointestinal stromal tumors. Target Oncol. 12(3), 277–288 (2017).
- Birbo B, Madu EE, Madu CO, Jain A, Lu Y. Role of HSP90 in cancer. Int. J. Mol. Sci. 22(19), 10317 (2021).
- Garcia-Carbonero R, Carnero A, Paz-Ares L. Inhibition of HSP90 molecular chaperones: moving into the clinic. Lancet Oncol. 14(9), e358–369 (2013).
- Neckers L, Workman P. Hsp90 molecular chaperone inhibitors: are we there yet? Clin. Cancer Res. 18(1), 64–76 (2012).
- Shimamura T, Lowell AM, Engelman JA, Shapiro GI. Epidermal growth factor receptors harboring kinase domain mutations associate with the heat shock protein 90 chaperone and are destabilized following exposure to geldanamycins. Cancer Res. 65(14), 6401–6408 (2005).
- Blay JY, Kang YK, Nishida T, von Mehren M. Gastrointestinal stromal tumours. Nat. Rev. Dis. Primers 7(1), 22 (2021).
- Miettinen M, Lasota J. Gastrointestinal stromal tumors: review on morphology, molecular pathology, prognosis, and differential diagnosis. Arch. Pathol. Lab. Med. 130(10), 1466–1478 (2006).
- Judson I, Bulusu R, Seddon B, Dangoor A, Wong N, Mudan S. UK clinical practice guidelines for the management of gastrointestinal stromal tumors (GIST). Clin. Sarcoma Res. 7, 6 (2017).
- Casali PG, Abecassis N, Aro HT et al. Gastrointestinal stromal tumours: ESMO-EURACAN Clinical Practice Guidelines for diagnosis, treatment and follow-up. Ann. Oncol. 29(Suppl. 4), iv68–iv78 (2018).
- Koo DH, Ryu MH, Kim KM et al. Asian consensus guidelines for the diagnosis and management of gastrointestinal stromal tumor. Cancer Res. Treat. 48(4), 1155–1166 (2016).
- National Comprehensive Cancer Network . NCCN Guidelines Version 1.2023 Gastrointestinal Stromal Tumors (2023). www.nccn.org/professionals/physician_gls/pdf/gist.pdf (Accessed 19 June 2023 ).
- Taiho Pharma . Taiho Pharmaceutical obtains approval to manufacture and market HSP90 inhibitor Jeselhy® tablets 40mg (pimitespib) for gastrointestinal stromal tumor (GIST) (2022). www.taiho.co.jp/en/release/2022/20220620.html (Accessed 21 July 2022 ).
- Chatterjee S, Bhattacharya S, Socinski MA, Burns TF. HSP90 inhibitors in lung cancer: promise still unfulfilled. Clin. Adv. Hematol. Oncol. 14(5), 346–356 (2016).
- Shimomura A, Yamamoto N, Kondo S et al. First-in-human phase I study of an oral HSP90 inhibitor, TAS-116, in patients with advanced solid tumors. Mol. Cancer Ther. 18(3), 531–540 (2019).
- Rubin BP, Heinrich MC, Corless CL. Gastrointestinal stromal tumour. Lancet 369(9574), 1731–1741 (2007).
- Ng EH, Pollock RE, Munsell MF, Atkinson EN, Romsdahl MM. Prognostic factors influencing survival in gastrointestinal leiomyosarcomas. Implications for surgical management and staging. Ann. Surg. 215(1), 68–77 (1992).
- Hirota S, Isozaki K, Moriyama Y et al. Gain-of-function mutations of c-kit in human gastrointestinal stromal tumors. Science 279(5350), 577–580 (1998).
- Heinrich MC, Corless CL, Duensing A et al. PDGFRA activating mutations in gastrointestinal stromal tumors. Science 299(5607), 708–710 (2003).
- Demetri GD, von Mehren M, Antonescu CR et al. NCCN Task Force report: update on the management of patients with gastrointestinal stromal tumors. J. Natl Compr. Canc. Netw. 8(Suppl. 2), S1–41; quiz S42–44 (2010).
- Kelly CM, Gutierrez Sainz L, Chi P. The management of metastatic GIST: current standard and investigational therapeutics. J. Hematol. Oncol. 14(1), 2 (2021).
- Casali PG, Blay JY, Abecassis N et al. Gastrointestinal stromal tumours: ESMO-EURACAN-GENTURIS Clinical Practice Guidelines for diagnosis, treatment and follow-up. Ann. Oncol. 33(1), 20–33 (2022).
- Blay JY, Serrano C, Heinrich MC et al. Ripretinib in patients with advanced gastrointestinal stromal tumours (INVICTUS): a double-blind, randomised, placebo-controlled, Phase 3 trial. Lancet Oncol. 21(7), 923–934 (2020).
- US Food and Drug Administration . Prescribing information - Vitrakvi (2018). www.accessdata.fda.gov/drugsatfda_docs/label/2018/211710s000lbl.pdf (Accessed 10 March 2022 ).
- US Food and Drug Administration . Prescribing information - Rozlytrek (2019). www.accessdata.fda.gov/drugsatfda_docs/label/2019/212725s000lbl.pdf (Accessed 10 March 2022 ).
- Uno T, Kawai Y, Yamashita S et al. Discovery of 3-Ethyl-4-(3-isopropyl-4-(4-(1-methyl-1 H-pyrazol-4-yl)-1 H-imidazol-1-yl)-1 H-pyrazolo[3,4- b]pyridin-1-yl)benzamide (TAS-116) as a potent, selective, and orally available HSP90 inhibitor. J. Med. Chem. 62(2), 531–551 (2019).
- Yoshimura C, Nagatoishi S, Kuroda D et al. Thermodynamic dissection of potency and selectivity of cytosolic Hsp90 inhibitors. J. Med. Chem. 64(5), 2669–2677 (2021).
- Ohkubo S, Kodama Y, Muraoka H et al. TAS-116, a highly selective inhibitor of heat shock protein 90alpha and beta, demonstrates potent antitumor activity and minimal ocular toxicity in preclinical models. Mol. Cancer Ther. 14(1), 14–22 (2015).
- Ikebe E, Shimosaki S, Hasegawa H et al. TAS-116 (pimitespib), a heat shock protein 90 inhibitor, shows efficacy in preclinical models of adult T-cell leukemia. Cancer Sci. 113(2), 684–696 (2022).
- Kihara T, Yuan J, Watabe T et al. Pimitespib is effective on cecal GIST in a mouse model of familial GISTs with KIT-Asp820Tyr mutation through KIT signaling inhibition. Exp. Mol. Pathol. 123, 104692 (2021).
- Saito Y, Takahashi T, Obata Y et al. TAS-116 inhibits oncogenic KIT signalling on the Golgi in both imatinib-naive and imatinib-resistant gastrointestinal stromal tumours. Br. J. Cancer 122(5), 658–667 (2020).
- Watanabe S, Goto Y, Yasuda H et al. HSP90 inhibition overcomes EGFR amplification-induced resistance to third-generation EGFR-TKIs. Thorac. Cancer 12(5), 631–642 (2021).
- Suzuki R, Hideshima T, Mimura N et al. Anti-tumor activities of selective HSP90alpha/beta inhibitor, TAS-116, in combination with bortezomib in multiple myeloma. Leukemia 29(2), 510–514 (2015).
- Suzuki R, Kikuchi S, Harada T et al. Combination of a selective HSP90α/β inhibitor and a RAS-RAF-MEK-ERK signaling pathway inhibitor triggers synergistic cytotoxicity in multiple myeloma cells. PLOS ONE 10(12), e0143847 (2015).
- Zhao S, Zhou L, Dicker DT et al. Anti-cancer efficacy including Rb-deficient tumors and VHL-independent HIF1alpha proteasomal destabilization by dual targeting of CDK1 or CDK4/6 and HSP90. Sci. Rep. 11(1), 20871 (2021).
- Tang D, Kang R. HSP90 as an emerging barrier to immune checkpoint blockade therapy. Oncoscience 9, 20–22 (2022).
- Zavareh RB, Spangenberg SH, Woods A, Martinez-Pena F, Lairson LL. HSP90 inhibition enhances cancer immunotherapy by modulating the surface expression of multiple immune checkpoint proteins. Cell. Chem. Biol. 28(2), 158–168 e155 (2021).
- Lee Y, Sunada S, Hirakawa H, Fujimori A, Nickoloff JA, Okayasu R. TAS-116, a novel Hsp90 inhibitor, selectively enhances radiosensitivity of human cancer cells to X-rays and carbon ion radiation. Mol. Cancer Ther. 16(1), 16–24 (2017).
- Ranta-aho S, Piippo N, Korhonen E, Kaarniranta K, Hytti M, Kauppinen A. TAS-116, a well-tolerated Hsp90 inhibitor, prevents the activation of the NLRP3 inflammasome in human retinal pigment epithelial cells. Int. J. Mol. Sci. 22(9), 4875 (2021).
- Doi T, Kurokawa Y, Sawaki A et al. Efficacy and safety of TAS-116, an oral inhibitor of heat shock protein 90, in patients with metastatic or unresectable gastrointestinal stromal tumour refractory to imatinib, sunitinib and regorafenib: a phase II, single-arm trial. Eur. J. Cancer 121, 29–39 (2019).
- Komatsu Y, Shimokawa T, Akiyoshi K et al. An open-label, crossover study to compare different formulations and evaluate effect of food on pharmacokinetics of pimitespib in patients with advanced solid tumors. Invest. New Drugs 40(5), 1011–1020 (2022).
- Vinarov Z, Abdallah M, Agundez JAG et al. Impact of gastrointestinal tract variability on oral drug absorption and pharmacokinetics: an UNGAP review. Eur. J. Pharm. Sci. 162, 105812 (2021).
- Kurokawa Y, Honma Y, Sawaki A et al. Pimitespib in patients with advanced gastrointestinal stromal tumor (CHAPTER-GIST-301): a randomized, double-blind, placebo-controlled Phase III trial. Ann. Oncol. 33(9), 959–967 (2022).
- Wagner AJ, Severson PL, Shields AF et al. Association of combination of conformation-specific KIT inhibitors with clinical benefit in patients with refractory gastrointestinal stromal tumors: a Phase 1b/2a nonrandomized clinical trial. JAMA Oncol. 7(9), 1343–1350 (2021).
- Pantaleo MA, Tarantino G, Agostinelli C et al. Immune microenvironment profiling of gastrointestinal stromal tumors (GIST) shows gene expression patterns associated to immune checkpoint inhibitors response. Oncoimmunology 8(9), e1617588 (2019).
- Adis Insight . Pimitespib - Taiho Pharmaceutical (2022). https://adisinsight.springer.com/drugs/800041893 (Accessed 14 March 2022 ).
- Kawazoe A, Itahashi K, Yamamoto N et al. TAS-116 (Pimitespib), an oral HSP90 inhibitor, in combination with nivolumab in patients with colorectal cancer and other solid tumors: an open-label, dose-finding, and expansion phase Ib trial (EPOC1704). Clin. Cancer Res. 27(24), 6709–6715 (2021).
- Konstantinopoulos PA, Cheng SC, Supko JG et al. Combined PARP and HSP90 inhibition: preclinical and Phase 1 evaluation in patients with advanced solid tumours. Br. J. Cancer 126(7), 1027–1036 (2022).