Abstract
Aim: The goal of this study was to determine if a single AAV vector, encoding Cas9 and guide RNAs specific for the HPV16 E6 and E7 genes, could inhibit the growth of an HPV16-induced tumor in vivo. Materials & methods: We grew HPV16+, patient-derived anal cancer explants in immunodeficient mice and then challenged these by injection of AAV-based vectors encoding Cas9 and control or HPV16-specific guide RNAs. Results & conclusion: We observed a significant and selective reduction in tumor growth when the HPV16 E6 and E7 genes were targeted using Cas9. These studies provide proof of principle for the hypothesis that CRISPR/Cas has the potential to be used to selectively treat HPV-induced tumors in humans.
Although anal cancer makes up only 1.5% of all gastrointestinal malignancies, its incidence has been rising over the past few years at a rate of approximately 3% per year [Citation1]. Thus, in 2003 there were an estimated 4000 new cases of anal cancer in the USA and 10 years later, in 2013, there were an estimated 6500 new cases [Citation1]. For patients with locally advanced anal cancer, standard of care treatment consists of a combination of chemotherapy and radiation and with this approach 5-year survival is about 75% [Citation2,Citation3]. For patients who recur, salvage surgery is an option, but this can lead to significant postoperative complications including bacterial infections, poor wound healing, urinary retention and slow recovery. For patients who are not candidates for surgery, treatment consists of palliative chemotherapy for disease control. Unfortunately, few therapies have been shown to be clinically beneficial in this setting and therefore there is an unmet clinical need to develop new therapeutics for the treatment of anal cancer.
Human papillomaviruses (HPVs) are the etiologic agents responsible for almost all cervical carcinomas and an increasing percentage of both anal and head-and-neck cancers [Citation4–6]. Only a subset of the many different HPV serotypes present a high risk for malignant transformation, with HPV16 and HPV18 accounting for approximately 70% of cervical cancers, while HPV16 alone accounts for >80% of HPV+ anal and head-and-neck cancers. A unique aspect of HPV-induced tumors is that their growth and survival requires the ongoing expression of two HPV-encoded oncogenes, E6 and E7, which induce the degradation of several host cell tumor suppressor proteins, including p53 and the retinoblastoma protein [Citation4–9]. Therefore, one potential approach to the elimination of HPV-induced cancers is to block the expression of E6 and/or E7, which results in cell cycle arrest and apoptosis. Indeed, several groups have reported that the introduction into HPV18- or HPV16-transformed cervical carcinoma cell lines, such as HeLa or SiHa, of a vector encoding the Streptococcus pyogenes (Spy) Cas9 protein, together with single guide RNAs (sgRNAs) specific for either the HPV E6 or E7 gene, results not only in the efficient inactivation of E6 and/or E7 expression due to gene editing but also in the apoptotic death of the HPV-transformed cell culture, while cells transformed by factors other than HPV are unaffected [Citation10–12]. This suggests that the expression of Cas9 and an HPV E6- or E7-specific sgRNA might represent an effective and specific approach to the elimination of HPV-transformed cells in vivo. Here, we describe the construction of adeno-associated virus (AAV) vectors expressing the short Staphylococcus aureus (Sau) Cas9 protein and two guide RNAs specific for both the HPV16 E6 and E7 gene and show that these can significantly reduce the growth of patient-derived xenografts (PDXs) of HPV16+ anal tumors in vivo in immunodeficient mice.
Materials & methods
Identification of effective HPV16-specific guide RNAs
To test the ability of the Sau Cas9 protein to cleave an HPV16-specific target, we identified two conserved potential target sites for Sau Cas9 in the HPV16 E6 and E7 genes located 5′ to the Sau Cas9 protospacer adjacent motif (PAM) 5′-NNGRRT-3′, where R is purine and N is any nucleotide [Citation13]. The two selected HPV16 target sequences were as follows, with PAM sequences shown in lower case:
HPV16-E6: 5′-AACAACTATACATGATATAATATtagaat-3′
HPV16-E7: 5′-AATTCAATGACAGCTCAGAGGAGgaggat-3′
To test activity, we generated indicator constructs, as previously described [Citation10], bearing segments of the HPV16 E6 or E7 open reading frame (ORF) fused to the firefly luciferase (FLuc) ORF. We then transfected these indicator constructs into human 293T cells (250 ng) along with a vector, pSauCas9Dual, that expresses both the SaCas9 protein and an sgRNA (1000 ng) and a vector expressing renilla luciferase (RLuc), as an internal control (50 ng), using the calcium phosphate method. At 48 h post-transfection, cells were harvested and FLuc and RLuc levels determined.
Derivation & preparation of AAV vectors
Two AAV vectors, based on the AAV8 capsid, expressing the Sau Cas9 protein and two sgRNAs, were prepared as previously described [Citation11]. One vector expressed the two HPV16-specific sgRNAs while the second, control vector expressed two nontargeting sgRNAs. The AAV vectors were commercially packaged by ViGene Biosciences (MD, USA) to give two AAV vector preparations that were then diluted to a concentration of 1010 AAV genome equivalents per μl, as determined by quantitative PCR.
Anal cancer PDX development & PCR analysis
Anal cancer specimens were collected under a Duke IRB approved protocol (Pro00002435) and all mouse experiments were carried out in accordance with animal guidelines and with the approval of the Institutional Animal Care and Use Committee (IACUC) at Duke University Medical Center under a Duke IACUC protocol (A84–15–06). All participants provided written informed consent to participate in the study and all methods used in this study were performed in accordance with the guidelines and regulations set forth by the Duke IRB and IACUC, respectively. PDXs were developed as described previously [Citation14,Citation15]. Briefly, anal cancer tissues were washed with phosphate buffered saline and then minced into pieces approximately 2 mm in size and injected into the flanks of 10-week-old JAX NOD.CB17-PrkdcSCID-J mice obtained from Duke University Rodent Genetic and breeding core.
PCR analysis was used to test for the presence of the HPV16 genome in the anal cancer PDXs and was also used to confirm that the targets for the above HPV16 E6 and E7-specific sgRNAs were indeed fully conserved in this primary anal tumor.
FLuc imaging of anal cancer PDX
Anal cancer PDXs were grown to 200 mm3 in size and were then injected with 10 μl of an AAV vector preparation, containing 1010 AAV particles per μl, intratumorally. This vector, called FLuc-AAV, was also based on the AAV8 capsid and was designed to express the FLuc reporter gene in transduced cells. Monitoring of FLuc expression was performed every 3–4 days using an in vivo imaging system.
Analysis of the ability of AAV vectors to inhibit HPV16+ primary tumor growth in vivo
Treatment of anal cancer PDXs was performed as follows: mice bearing anal cancer PDXs were randomized to control (n = 7) and treatment groups (HPV-AAV, n = 8) and, once the tumor reached a size of approximately 200 mm3, 10 μl of AAV, at 1010 AAV particles per μl, was injected intratumorally at three different sites on day 0 and again on day 7. Tumor volume was measured twice per week by caliper and the following formula used to calculate tumor volume: (length × (width)2)/2. Mouse experiments were terminated at 2 weeks after the initial injection and the PDXs then recovered. DNA isolated from the PDXs was then used for sequence analysis of the HPV16 E6 gene. Statistical analyses were performed using the GraphPad Prism software (CA, USA).
Results
Construction of an AAV vector expressing Cas9 & HPV16-specific guide RNAs
In order to test the ability of CRISPR/Cas to inhibit the growth of HPV16+ tumors in vivo, we aimed to construct a viral vector, based on AAV, that can express Cas9 and an HPV16-specific sgRNA in transduced cells, as AAV can be prepared at titers as high as 1014/ml. Unfortunately, the Spy Cas9 gene used in most previous studies on CRISPR/Cas-mediated gene editing is approximately 4.2 kb in size. AAV has a maximum packaging capacity of approximately 4.8 kb in which to encompass not only a Cas9 cDNA but also the sgRNA(s) and cis-acting sequences required for expression, so Spy Cas9 is too large for use in an AAV-based vector. However, the Cas9 gene expressed by S. aureus (Sau), is only approximately 3.2 kb in length and, as we have previously demonstrated, AAV vectors encoding Sau Cas9, and two sgRNAs, can be readily generated and edit genes in transduced cells in culture [Citation13,Citation16].
To test the ability of Sau Cas9 to cleave an HPV16-specific target, we identified conserved potential target sites for Sau Cas9 in the HPV16 E6 and E7 genes located 5′ to the Sau Cas9 PAM 5′-NNGRRT-3′ [Citation13]. To test activity, we generated indicator constructs, as previously described [Citation11], bearing segments of the HPV16 E6 or E7 ORF fused to the FLuc ORF (). We then transfected these indicator constructs into 293T cells along with vectors expressing Sau Cas9 and an HPV16-specific or control sgRNA, and a vector expressing RLuc, as an internal control. At 48 h post-transfection, cells were harvested and FLuc and RLuc levels determined (). Both HPV16-specific sgRNAs were found to induce a significant and specific approximately 97% inhibition of FLuc expression when compared with the control sgRNA.
Next, we generated AAV vectors, based on the AAV8 capsid, expressing Sau Cas9 as well as both the HPV16-E6 and HPV16-E7 sgRNA (AAV–HPV), or two control nontargeting sgRNAs (AAV–Control), using a previously described AAV vector design [Citation13,Citation16]. Briefly, in this vector design, the two sgRNAs are transcribed by RNA polymerase III (Pol III)-dependent promoters, derived either from the human glutamine tRNA gene or the U6 snRNA gene, while Cas9 is expressed from a minimal form of the Pol II-dependent human cytomegalovirus immediate early promoter (). The two AAV vectors were then packaged to generate AAV vector preparations that were then diluted to a titer of 1013 genome equivalents per ml.
Indicator constructs containing segments of the HPV16 E6 or E7 ORF fused in frame with the FLuc indicator gene [Citation10] were transfected into 293T cells along with vectors encoding Sau Cas9 and an sgRNA specific for the HPV16 E6 or E7 gene, or a control sgRNA. An internal control vector encoding RLuc was also co-transfected. At 48 h post-transfection, the cells were harvested and FLuc and RLuc levels determined. FLuc levels were normalized to the RLuc internal control and are given relative to the control sgRNA, which was set at 1. Average of three experiments with SD indicated.
FLuc: Firefly luciferase; ORF: Open reading frame; RLuc: Renilla luciferase; SD: Standard deviation; sgRNA: Single guide RNA.
![Figure 1. Inhibition of HPV16 E6 and E7 function using Sau Cas9.Indicator constructs containing segments of the HPV16 E6 or E7 ORF fused in frame with the FLuc indicator gene [Citation10] were transfected into 293T cells along with vectors encoding Sau Cas9 and an sgRNA specific for the HPV16 E6 or E7 gene, or a control sgRNA. An internal control vector encoding RLuc was also co-transfected. At 48 h post-transfection, the cells were harvested and FLuc and RLuc levels determined. FLuc levels were normalized to the RLuc internal control and are given relative to the control sgRNA, which was set at 1. Average of three experiments with SD indicated.FLuc: Firefly luciferase; ORF: Open reading frame; RLuc: Renilla luciferase; SD: Standard deviation; sgRNA: Single guide RNA.](/cms/asset/3bce15f4-e250-4d83-9058-6c19808fab76/ifvl_a_12335664_f0001.jpg)
This AAV8-based vector, which expresses two sgRNAs plus the Sau Cas9 protein in transduced cells, falls well below the approximately 4.8 kb packaging size limit for AAV vectors. sgRNAs were expressed using RNA polymerase III (pol III)-dependent promoters while Sau Cas9 was expressed using a minimal CMV-derived promoter.
AAV: Adeno-associated virus; CMV: Cytomegalovirus; ITR: Inverted terminal repeat; sgRNA: Single guide RNA.
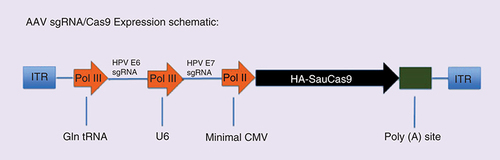
Passage of HPV16-positive patient-derived tumor explants in mice
We next generated PDXs as previously described [Citation15]. A shows that the histological features of these PDXs are similar to that of the primary anal tumor from which they were derived. A total of three anal cancer PDXs were generated and B shows that one PDX was HPV16 positive (ANA001). Moreover, sequencing of PCR amplicons confirmed that both potential sgRNA targets identified in the HPV16 E6 and E7 genes, as well as the adjacent PAM sequences, were fully conserved in ANA001 (data not shown).
To determine whether or not AAV8-based vectors could infect ANA001 by intratumoral injection, ANA001 was injected with FLuc-AAV. An in vivo imaging system was then used to detect FLuc expression every 3 days for 2 weeks (). Expression of FLuc was indeed detected at all time points analyzed, though we did see reduced FLuc expression by 13 days after infection by FLuc-AAV. These data confirmed that an AAV8 capsid-based FLuc-AAV vector can rapidly infect anal cancer PDXs in vivo and showed that gene expression from the injected AAV vector can persist for up to 2 weeks.
(A) H&E-stained sections of matched patient tumor and PDX of an anal cancer revealed similar histological features. (B) PCR was used to detect the HPV16 genome in one out of three anal cancer PDXs.
H&E: Hemotoxylin and eosin; Lane 1: HPV16+ SiHA cervical carcinoma cells (positive control); Lanes 2–4: Anal cancer PDXs; PDX: Patient-derived xenograft.
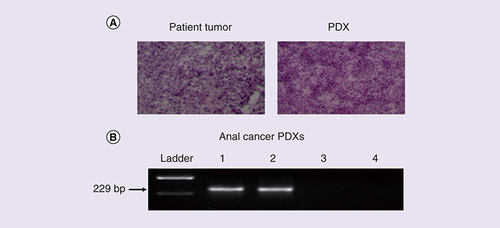
An AAV8 capsid-based AAV vector encoding FLuc was packaged and then diluted to 1010 AAV particles per μl. A total of 10 μl were then injected IT into an anal cancer PDX in mice. FLuc expression was then monitored using an in vivo imaging system.
AAV: Adeno-associated virus; FLuc: Firefly luciferase; IT: Intratumorally; PDX: Patient-derived xenograft.
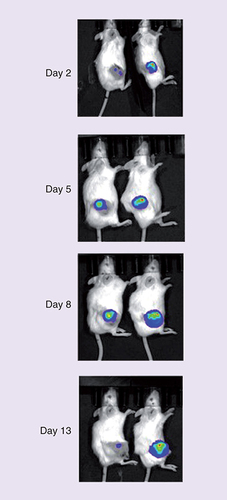
Inhibition of the growth of an HPV16 positive anal tumor in vivo using CRISPR/Cas
We next tested the ability of HPV-AAV to specifically inhibit the growth of HPV16+ cancer cells in vivo. We generated 15 mice bearing the ANA001 anal cancer PDX and, once the tumor had reached a size of 200 mm3, the mice were randomized to a control group (n = 7) and a treatment group (n = 8). The mice were then injected with an AAV vector preparation intratumorally at three different sites at day 0 and day 7 and tumor volume determined every 3–4 days up to a total of 14 days. shows that the HPV-AAV vector induced a readily detectable, >twofold decrease in tumor volume, when compared with the control AAV preparation, by 2 weeks after treatment was initiated. This decrease was statistically highly significant (analysis of variance; p < 0.01).
HPV-AAV, or a control AAV vector encoding two nontargeting sgRNAs, was injected IT into an anal cancer PDX in mice. Tumor volume was measured twice per week. A statistically significant (p < 0.01, as determined by one-way analysis of variance) inhibition of tumor growth was observed in the anal cancer PDXs treated with HPV-AAV (n = 8) when compared with the control AAV (n = 7).
AAV: Adeno-associated virus; HPV: Human papillomavirus; IT: Intratumorally; PDX: Patient-derived xenograft; sgRNA: Single guide RNA.
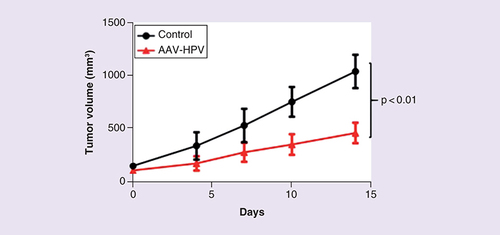
Discussion
Treatment of anal cancer has evolved over the past few decades from surgery to chemoradiation. Efforts to improve treatment have focused on altering dosage and frequency of radiation in addition to evaluating different types of chemotherapy. Unfortunately, minimal improvement has been made using these treatment modalities and new, more specific and more effective therapies are clearly needed.
Although mouse models of cancer have been used in front-line preclinical studies to study the efficacy and toxicity of anticancer drugs [Citation17,Citation18], currently, there are limited preclinical models of anal cancer. For example, a mouse model of K14E6 and K14E7 transgenic mice, in which the HPV16 E6 and E7 genes are directed in their expression to stratified squamous epithelia, has been developed [Citation18]. Recently, we and others demonstrated that an approach of rapid engraftment of patient tumor tissue into immunodeficient mice to develop PDXs can provide a more clinically applicable murine model to study drug sensitivities [Citation15,Citation19–23]. In this study, we used the same method to develop three anal cancer PDXs, one of which was found to be HPV16+. More importantly, these anal cancer PDXs can now be used as preclinical models to further study and identify new therapies for anal cancer.
In our current work, we are the first to demonstrate that targeting of the HPV16 genome in primary human anal cancer cells in vivo using CRISPR/Cas can significantly slow tumor growth (). However, a complete response was not observed. Nevertheless, tumor growth inhibition by HPV-AAV was both readily detectable and statistically significant. We hypothesize that the lack of complete tumor clearance reflects the fact that only a subset of tumor cells actually took up the AAV vector and expressed Cas9, although it is also possible that the editing efficiency of Sau Cas9 in these in vivo experiments was insufficient to introduce indels into all copies of the HPV16 genome. Of note, sequencing of the HPV16 E6 gene present in the anal tumor PDX at day 14, when the experiment was terminated and the mice sacrificed, did not reveal any indels at the predicted Cas9 cleavage site in E6 (data not shown), thus demonstrating that tumor growth was not due to acquisition of resistance to the specific sgRNAs used. We hypothesize that tumor cells that underwent Cas9-mediated gene editing of the HPV16 E6 or E7 gene were rapidly lost from the tumor, thus explaining both the reduced tumor volume and our inability to observe any viral indels. Nevertheless, these data do suggest that CRISPR/Cas has the potential to eliminate HPV16+ tumors in vivo if delivery to tumor cells could be made more efficient.
Conclusion
The results presented in this manuscript demonstrate that AAV vectors designed to deliver the Sau Cas9 gene and cognate sgRNAs into cells in vivo have the potential to induce the efficient editing of chromosomal genes.
Future perspective
Given the intense efforts currently ongoing to generate the tools required to move the CRISPR/Cas from the laboratory into the clinic, it seems very likely that this technology will soon be in clinical trials for a range of human diseases. Chronic disease caused by DNA viruses, including HPV-induced cancers, seem to be an ideal area for this approach especially as potential off-target editing is of less consequence if the idea is to kill the cell that is edited and if the patient already has late stage cancer. Clearly, these experiments need to repeated with a range of HPV16+ anal and head-and-neck PDXs in mice, and with a range of different AAV-vector constructs, using mouse numbers that would allow a clearer definition of whether high levels of selective tumor cell killing can be achieved in vivo. However, the current data do provide proof of concept that CRISPR/Cas indeed has the potential to selectively deplete HPV-transformed cells in vivo.
We generated a single AAV8-based vector expressing the Sau Cas9 protein and single guide RNAs specific for the HPV16 E6 and E7 genes.
We established patient-derived explants (PDXs) from a human anal cancer that was HPV16+ and showed that these PDXs can grow and be passaged in mice.
We demonstrated that AAV8-based vectors efficiently infect anal tumor PDXs after direct injection in vivo in mice.
We showed that direct injection of the AAV vector expressing Sau Cas9 and HPV16-specific single guide RNAs markedly and significantly inhibits the growth of human anal tumor PDXs in mice.
Author’s contributions
DS Hsu, EM Kennedy and BR Cullen devised the initial idea for this study and planned the experiments. AVR Kornepati and W Glover performed the experiments. DS Hsu, AVR Kornepati and BR Cullen analyzed the results. DS Hsu and BR Cullen supervised the study and wrote and edited the paper.
Financial & competing interests disclosure
This work was supported by grant R21-CA198050 from the National Cancer Institute. The authors have no other relevant affiliations or financial involvement with any organization or entity with a financial interest in or financial conflict with the subject matter or materials discussed in the manuscript apart from those disclosed.
No writing assistance was utilized in the production of this manuscript.
References
- Siegel R , NaishadhamD, JemalA . Cancer statistics, 2013 . CA Cancer J. Clin.63, 11 – 30 ( 2013 ).
- Ajani JA , WinterKA, GundersonLLet al. Fluorouracil, mitomycin, and radiotherapy vs fluorouracil, cisplatin, and radiotherapy for carcinoma of the anal canal: a randomized controlled trial . JAMA299, 1914 – 1921 ( 2008 ).
- Gunderson LL , WinterKA, AjaniJAet al. Long-term update of US GI intergroup RTOG 98–11 Phase III trial for anal carcinoma: survival, relapse, and colostomy failure with concurrent chemoradiation involving fluorouracil/mitomycin versus fluorouracil/cisplatin . J. Clin. Oncol.30, 4344 – 4351 ( 2012 ).
- Mighty KK , LaiminsLA . The role of human papillomaviruses in oncogenesis . Recent Results Cancer Res.193, 135 – 148 ( 2014 ).
- McLaughlin-Drubin ME , MüngerK . Oncogenic activities of human papillomaviruses . Virus Res.143, 195 – 208 ( 2009 ).
- Howley PM . Role of the human papillomaviruses in human cancer . Cancer Res.51, s5019 – s5022 ( 1991 ).
- DeFilippis RA , GoodwinEC, WuL, DiMaioD . Endogenous human papillomavirus E6 and E7 proteins differentially regulate proliferation, senescence, and apoptosis in HeLa cervical carcinoma cells . J. Virol.77, 1551 – 1563 ( 2003 ).
- Goodwin EC , DiMaioD . Repression of human papillomavirus oncogenes in HeLa cervical carcinoma cells causes the orderly reactivation of dormant tumor suppressor pathways . Proc. Natl Acad. Sci. USA97, 12513 – 12518 ( 2000 ).
- Scheffner M , WernessBA, HuibregtseJM, LevineAJ, HowleyPM . The E6 oncoprotein encoded by human papillomavirus types 16 and 18 promotes the degradation of p53 . Cell63, 1129 – 1136 ( 1990 ).
- Kennedy EM , KornepatiAV, GoldsteinMet al. Inactivation of the human papillomavirus E6 or E7 gene in cervical carcinoma cells by using a bacterial CRISPR/Cas RNA-guided endonuclease . J. Virol.88, 11965 – 11972 ( 2014 ).
- Zhen S , HuaL, TakahashiY, NaritaS, LiuYH, LiY . In vitro and in vivo growth suppression of human papillomavirus 16-positive cervical cancer cells by CRISPR/Cas9 . Biochem. Biophys. Res. Commun.450, 1422 – 1426 ( 2014 ).
- Hu Zheng , YuLan, ZhuDaet al. Disruption of HPV16-E7 by CRISPR/Cas system induces apoptosis and growth inhibition in HPV16 positive human cervical cancer cells . Biomed. Res. Int. 2014, 612823 ( 2014 ).
- Friedland AE , BaralR, SinghalPet al. Characterization of Staphylococcus aureus Cas9: a smaller Cas9 for all-in-one adeno-associated virus delivery and paired nickase applications . Genome Biol.16, 257 ( 2015 ).
- Kim MK , OsadaT, BarryWTet al. Characterization of an oxaliplatin sensitivity predictor in a preclinical murine model of colorectal cancer . Mol. Cancer Ther.11, 1500 – 1509 ( 2012 ).
- Uronis JM , OsadaT, McCallSet al. Histological and molecular evaluation of patient-derived colorectal cancer explants . PLoS ONE7, e38422 ( 2012 ).
- Kennedy EM , KornepatiAVR, MefferdALet al. Optimization of a multiplex CRISPR/Cas system for use as an antiviral therapeutic . Methods91, 82 – 86 ( 2015 ).
- Suggitt M , BibbyMC . 50 years of preclinical anticancer drug screening: empirical to target-driven approaches . Clin. Cancer Res.11, 971 – 981 ( 2005 ).
- Stelzer MK , PitotHC, LiemAet al. A mouse model for human anal cancer . Cancer Prev. Res. (Phila.)3, 1534 – 1541 ( 2010 ).
- Fichtner I , SlisowW, GillJet al. Anticancer drug response and expression of molecular markers in early-passage xenotransplanted colon carcinomas . Eur. J. Cancer40, 298 – 307 ( 2004 ).
- Dangles-Marie V , PocardM, RichonSet al. Establishment of human colon cancer cell lines from fresh tumors versus xenografts: comparison of success rate and cell line features . Cancer Res.67, 398 – 407 ( 2007 ).
- Guenot D , GuérinE, Aguillon-RomainSet al. Primary tumour genetic alterations and intra-tumoral heterogeneity are maintained in xenografts of human colon cancers showing chromosome instability . J. Pathol.208, 643 – 652 ( 2006 ).
- Bertotti A , MigliardiG, GalimiFet al. A molecularly annotated platform of patient-derived xenografts (“xenopatients”) identifies HER2 as an effective therapeutic target in cetuximab-resistant colorectal cancer . Cancer Discov.1, 508 – 523 ( 2011 ).
- Tentler JJ , NallapareddyS, TanACet al. Identification of predictive markers of response to the MEK1/2 inhibitor selumetinib (AZD6244) in K-ras-mutated colorectal cancer . Mol. Cancer Ther.9, 3351 – 3362 ( 2010 ).