Abstract
Aim: To evaluate the antibacterial and antibiofilm activity of ceragenin-conjugated nonspherical gold nanoparticles against the most common agents of otitis media. Methods: Minimal inhibitory and bactericidal concentrations and colony-counting assays, as well as colorimetric and fluorimetric methods, were used to estimate the antibacterial activity of compounds in phosphate-buffered saline and human cerumen. The nanosystems’ biocompatibility and ability to decrease IL-8 release was tested using keratinocyte cells. Results: The tested compounds demonstrated strong antimicrobial activity against planktonic and biofilm cultures at nontoxic doses due to the induction of oxidative stress followed by the damage of bacterial membranes. Conclusion: This study indicates that ceragenin-conjugated nonspherical gold nanoparticles have potential as new treatment methods for eradicating biofilm-forming pathogens associated with otitis media.
Lay abstract
Middle-ear infections can be painful and cause hearing difficulties. If untreated, they can lead to hearing loss. These infections are usually treated with antibiotic drugs. However, the microbes causing the infection can gain drug resistance. This article reports research into a new way of delivering antibiotics to kill the microbes and the communities they form (biofilms). The authors developed tiny gold particles loaded with the antimicrobial drug ceragenin and tested the drug-loaded particles on three common middle-ear infection-causing bacteria. Compared with ceragenin alone, the ceragenin-loaded particles were better at killing the bacteria and their biofilm communities.
Otitis media (OM) is a clinical condition characterized by the presence of an inflammatory reaction in the middle ear caused by a variety of infectious agents. It can be classified as acute OM (AOM) or chronic OM (COM), including OM with effusion (OME) distinguished by the presence of liquid in the cavities of the middle ear without signs of acute infection [Citation1]. Approximately 50% of children have at least one episode of AOM before school age, with the highest incidence between 3 and 24 months of age, as a consequence of a shorter and more horizontal eustachian tube, which predisposes to ascending route of the middle ear infection by pathogens from the nasopharynx [Citation2]. Aside from the anatomical causes, a defective immune system favoring the colonization of potential pathogens pose a risk in the pathogenesis of AOM [Citation3]. A recent study revealed that 98% of children aged ≥2 years with AOM received antibiotic treatment with amoxicillin being routinely prescribed [Citation4]. Clinically, AOM is characterized by ear pain, fever and temporary hearing difficulties. Unresolved and recurrent OM episodes may lead to severe complications, speech development problems, prolonged hearing loss, learning disabilities and decreased performance in educational skills [Citation5,Citation6].
Although the etiology of AOM is multifactorial, encompassing bacteria, viruses or mixed infections, the ‘classical otopathogens’ (i.e., Streptococcus pneumoniae, nontypeable Haemophilus influenzae and Moraxella catarrhalis), are still of the greatest clinical importance [Citation7,Citation8]. These pathogens persist in the form of mono- and multispecies biofilms, and their location in the extracellular matrix of the middle ear or nasopharynx leaves them protected from antibiotics due to poor penetration. The clinical importance of an untreated biofilm mass is implicated in recurrent AOM and COM [Citation8–10]. Interestingly, although the introduction in 2000 of heptavalent S. pneumoniae vaccine (PCV7) followed by a 13-valent pneumococcal conjugate vaccine (PCV13) initially reduced the relative prevalence of this pathogen in AOM, an increase of nonvaccine serotypes was observed, and a similar trend may be expected for serotypes not included in emerging pneumococcal vaccines [Citation1,Citation11–13]. It is also noteworthy that viral upper respiratory tract infections, caused mainly by respiratory syncytial virus, cytomegalovirus, influenza viruses, adenoviruses and coronaviruses, often precede or coincide with episodes of AOM. In particular, adenoviruses inhabit the nasopharyngeal niche and possibly gain entry to the middle ear upon interaction with other bacterial otopathogens [Citation14]. To date, a spectrum of effective treatment options is available for AOM, based on systemic administration of beta-lactam antibiotics, such as amoxicillin or amoxicillin/clavulanate and cephalosporins as the first-line therapy or macrolides and lincosamides as alternative drugs [Citation1]. Nevertheless, several issues in OM therapy must be addressed, including the growing resistance of otopathogens to these antibiotics, in particular cephalosporins, and a risk that they pose for development of resistance in bacteria [Citation13]; the aforementioned implication of viral etiology; and difficulties with treatment of polymicrobial biofilms. Moreover, the prevalence of AOM and the continuous emergence of drug-resistant organisms highlight the need for development of new treatment options and their alternative methods of delivery to the middle ear, such as transtympanic or intratympanic pathways, which would potentially improve the outcome of treatment and prevent the systemic administration of antibiotics to mitigate a selection of resistant pathogens [Citation15,Citation16].
Ceragenins represent a novel class of low molecular weight antimicrobial agents designed as nonpeptide and low-cost mimics of endogenous antimicrobial peptides (AMPs) [Citation17]. Chemically, ceragenins are positively charged derivatives of cholic acid, and therefore also called cationic steroid antimicrobials (CSAs); they are distinguished by various number and distribution of amine groups (e.g., CSA-13, CSA-44 or CSA-144). Like AMPs, ceragenins are broad-spectrum antimicrobials with potent killing action against multiple bacterial, fungal, protozal as well as viral pathogens, including multidrug-resistant organisms [Citation18]. For example, we previously reported the activity of CSAs against various oral and upper respiratory tract infection pathogens, including the classical otopathogens, for which minimal inhibitory (MIC) and bactericidal (MBC) concentrations were below 2 μg/ml [Citation19]. Similarly, Moscoso et al. reported a high activity of CSA-13 against both planktonic cultures and biofilms of S. pneumoniae [Citation20]. In addition, ceragenins exhibit potent microbicidal properties against polymicrobial Candida albicans and Staphylococcus aureus biofilms [Citation21]. CSA’s antimicrobial action is exerted through a direct interaction with the negatively charged bacterial membrane components and lipid bilayers in the case of viruses, leading to morphological changes and disruption [Citation18,Citation22,Citation23]. Importantly, a high level of resistance of ceragenins to inhibitory action of the host compounds present at high concentrations in inflamed sites such as mucin, DNA or F-actin [Citation24–26], makes them a strong candidate for novel agents to treat OM. Finally, the fact that bacteria do not easily develop resistance to ceragenins makes them suitably attractive as long-term antimicrobial agents for treatment of various resistant pathogens [Citation27,Citation28].
Nanoparticles have recently emerged as promising antimicrobial agents themselves and as carriers for other antimicrobials owing to their unique physicochemical properties, in particular a large surface area-to-volume ratio that ensures their strong interactions with microbial membranes [Citation29]. Among a spectrum of nanomaterials available to date, metallic nanoparticles, including those made of gold, have gained considerable interest due to their stability, good biocompatibility, favorable pharmacokinetic parameters [Citation30], potent antimicrobial activity [Citation31,Citation32], anti-inflammatory potential [Citation33] and synergistic interactions with commonly used antibiotics [Citation34]. An ever-growing number of studies also demonstrate high applicability of gold nanoparticles (AuNPs) as drug nanocarriers. In such applications, in recent years AuNPs were functionalized with aminoglycosides, amoxicillin or auranofin to achieve satisfactory therapeutic efficiency against burn wound-isolated Acinetobacter baumannii [Citation35], MRSA [Citation36] or S. pneumoniae and S. pyogenes [Citation37], which legitimates the validity of such approach. Nevertheless, these studies were performed using spherical AuNPs. A thorough analysis of antimicrobial activity of AuNPs performed to date clearly indicates that biological interactions of AuNPs are governed by a spectrum of physicochemical features – in particular, their size, shape (spherical, rod, cube, square, flake, irregular etc.) – and surface charge or type of material [Citation38,Citation39]. Therefore, one of the contemporary trends in the nanoantibiotic field is to explore the functional properties and effects of different geometrical shapes of nanoparticles for the treatment of resistant pathogens [Citation40] and our recent studies confirm that nonspherical nanomaterials should be considered as the potent antimicrobials [Citation41,Citation42]. Data collected to date also demonstrate the possibility of employing those nanomaterials as drug nanocarriers. Most recently, we discovered that AuNPs in the shape of rods, peanuts or stars are effective nanocarriers of ceragenin CSA-131 and through increased ceragenin density on the surface of AuNPs, improved therapeutic efficiency against multidrug-resistant strains can be accomplished [Citation43]. Nevertheless, there are still limited data on the activity of nonspherical, ceragenin-containing nanosystems in the presence of body fluids or their ability to decrease inflammatory burden. For this reason, the current study is aimed at investigating the antibacterial activity of ceragenins CSA-13, CSA-131 and CSA-44 alone and conjugated with peanut-shaped AuNPs (AuP NPs) against planktonic cells and biofilms produced by S. pneumoniae, H. influenzae and M. catarrhalis and to investigate the anti-inflammatory activities of these compounds. To that end, microbiological assays were performed in artificial media as well as in the presence of human cerumen to estimate the potential utility of these compounds for the local treatment of ear infections. Simultaneously, bacterial responses to the tested agents were captured by reactive oxygen species (ROS) generation, outer membrane permeability and protein leakage assays. Moreover, their anti-inflammatory potential was estimated by measurement of IL-8 production by bacteria-stimulated cells of keratinocyte cell line.
Materials & methods
Materials
Reagents required for ceragenin-attached gold nanosystems [Citation43] were purchased from Sigma-Aldrich (MO, USA). Laboratory strains of OM-causing pathogens: H. influenzae ATCC 49766, M. catarrhalis ATCC 25238, Streptococcus pneumoniae ATCC 49619 were from American Type Culture Collection (VA, USA). Growth media were as follows: chocolate II Agar, blood No 2 LAB-AGAR+ 5% KB, brain heart infusion broth medium and required supplements: β-nicotinamide adenine dinucleotide (NAD) and hematin porcine were obtained from Becton Dickson (NJ, USA), BioMaxima (Lublin, Poland) and Sigma-Aldrich, respectively. Brain heart infusion broth medium was purchased from Sigma-Aldrich. Reagents for colorimetric and fluorimetric analysis: 1-N-phenylnaphtylamine (NPN), 2′,7′-dichlorofluorescin diacetate (DCFH-DA), Bradford reagent and 3-[4,5-dimethylthiazole-2-yl]-2,5-diphenyltetrazolium bromide (MTT) were from Sigma-Aldrich. IL-8 Human ELISA Kit was purchased from Thermo Fisher Scientific (MA, USA).
Synthesis & physicochemical analysis of ceragenin-decorated nanosystems
Ceragenins (CSA-13, CSA-131, CSA-44) were synthesized as previously reported [Citation44], dissolved in phosphate-buffered saline (PBS) and stored at 4°C. Ceragenin-containing nanosystems consisting of CSA-13, CSA-44 or CSA-131 attached to the surface of peanut-shaped AuNPs (AuP@CSA-13, AuP@CSA-44 and AuP@CSA-131, respectively) were synthetized according to the protocols presented in our previous papers [Citation39,Citation41,Citation43]. In general, peanut-shaped AuNPs, prepared via cetrimonium bromide (CTAB)-assisted method were surface functionalized with tested ceragenins using mercaptohexadecanoic acid (MHDA) as linker, allowing in following steps to form a covalent bonding between the –COOH group of MHDA and –NH3 groups of ceragenins. The morphology and composition of synthetized nanosystem was ensured due to the reproducible and highly controllable synthesis method of nanoparticles; appropriate selection of reaction times and temperature, which allows for collection of the suspension of uniform nanoparticles; and using MHDA in excess, thanks to which it can guaranteed that all ceragenins molecules are attached to the surface of the AuNP. Final concentration of CSAs and AuP NPs in prepared nanosystems were 2 mg/ml and 2.93 ng/ml, respectively. On this basis, loading efficiency of ceragenin on the surface of AuP NP was estimated as 1.33 × 105 molecules of CSA/1 AuP NP.
Scanning transmission electron microscopy (STEM) with a high-angle annular dark field detector (HAADF), in conventional and high-resolution mode, was used to determine the AuP NPs morphology. The STEM images were acquired on an aberration-corrected FEI Titan electron microscope operating at 300 kV equipped with a FEG cathode. A two-circle laboratory diffractometer, Panalytical X'Pert Pro, was implemented for analyzing the crystal microstructure of AuP NPs. The measurements were recorded using a lamp with standard θ-2θ geometry and Cu anode working at 40 kV and 30 mA. The x-ray beam emitted by the x-ray tube was converted into a parallel beam by a divergence slit with a constant height 1/2°, a parabolic graded W/Si mirror with an equatorial divergence less than 0.05, 0.04 rad Soller slit collimator and by a mask of constant width of 20 mm, which restricts the width of the beam. The diffracted beam optics consisted of the antiscattered slit with a height of 8.7 mm, 0.04 rad Soller slit collimator, a curved graphite monochromator to eliminate the contribution of the Cu Kβ radiation, and a semiconductor silicone stripe detector with an active length of 2.122°. The dispersion of the nanoparticles was dried on a zero-background holder and was placed on a sample spinner with rotation time of 16 s. The data was obtained in the range between 20–80° and step size of 0.08°. The time per step was equal to 7000 s. The Fullprof software was used for the evaluation of the diffractogram fitting, lattice constants and coherent scattering length [Citation45]. By collection of Fourier transform (FT)-Raman spectra the success and stability of the biofunctionalization and immobilization processes was investigated. Nicolet NXR 9650 FT-Raman Spectrometer equipped with an Nd:YAG laser (1064 nm) and a germanium detector was used for recording the spectra with the settings as described in our previous work [Citation43]. UV-visible spectroscopy was used to investigate the CSA molecules anchored on Au NPs. Accordingly Multiskan SkyHigh UV-VIS (Thermo Fisher Scientific) was used, with a resolution of 1 nm and scan speed of 240 nm/min. Calorimetric measurements were employed to investigate the temperature stability of nanoparticles functionalized by MHDA, as well as MHDA and ceragenins. This experiment was performed using TA Instruments’ DSC 2500 differential scanning calorimeter (DSC) equipped with a liquid nitrogen LN2P pump. The analyzed samples were placed in aluminium pans and crimped with hermetic lids. To study the thermal behavior of the samples, the given conditions was followed: dry N5.0 pure nitrogen purge (25 ml/min-1) in a temperature range from 10–200°C with heating rate at 5 and 10°C min-1 and cooling rate at 5°C min-1. The samples were held isothermally at the minimum and maximum temperature for 5 min. The peak temperatures and enthalpy values of the registered thermal events were calculated using TRIOS software. The thermogravimetric analysis (TGA) measurements were recorded using a TA Instruments’ TGA 5500 thermogravimetric analyzer with high-temperature platinum pans. The samples were either directly placed in open 100-μl platinum pans or previously enclosed inside hermetic aluminum pans to restrict the evaporation rate. Before starting the experiments with aluminium pans, a 0.9-mm-diameter hole was punched through the lid of the container. The samples were heated at a rate of 5 or 10°C min-1 rate up to 500°C under a flow of N5.0 pure nitrogen (25 ml/min-1). The temperature was calibrated using nickel and alumel standards.
Bacterial strains, media & experimental settings
Laboratory strains of OM-causing pathogens (H. influenzae ATCC 49766, M. catarrhalis ATCC 25238, S. pneumoniae ATCC 49619) were cultured using BBL Chocolate II Agar and 5% sheep blood agar plates at 37°C in 5% CO2 atmosphere. Hemophilus test medium supplemented with β-NAD and hematin porcine was prepared according to previously published protocols [Citation46]. For the purpose of antibiofilm activity assessment, brain–heart infusion broth medium was used.
All experiments assessing antibacterial activity of the tested compounds (discussed subsequently) were performed on 96-well microtiter plates in a final volume of 100 μl, with the exception of MIC/MBC measurement, which was carried out in a final volume of 200 μl. The absorbance or fluorescence measurements were recorded with Varioskan Lux microplate reader (Thermo Fisher Scientific).
Killing assay
Estimation of bacterial survival upon treatment with free ceragenins and CSA-containing nanosystems was performed using the colony counting (killing assay) method according to the protocol published previously [Citation47]. The plates were incubated at 37°C, 5% CO2 for further 18 h to determine the number of viable colonies. Survival of bacteria upon treatment with tested agents was calculated using the appropriate dilution factors and presented as decrease of log (CFU/ml). Concentrations of the tested agents required to limit the viability of bacterial population to 2 log CFU/ml, that is, 100 CFU/ml, was estimated by interpolation of dose–response curves.
Antimicrobial susceptibility testing
Minimal inhibitory (MIC) and minimal bactericidal (MBC) concentrations of CSA-13, CSA-131 and CSA-44, in a free form and conjugated with AuP NPs, for the otopathogens were determined using the broth microdilution method. Briefly, the bacterial inoculum was adjusted to 4 × 105 CFU/ml using the Hemophilus test medium and incubated with serial twofold dilutions of the tested agents ranging from 256 μg/ml to 0.5 μg/ml. Microbial growth was measured after 18–20 h of incubation at 37°C in 5% CO2. The MBCs were evaluated after 48 h of growth by plating the ≥ MIC representative values on chocolate or blood agar plates.
Activity of the tested compounds in the presence of human cerumen
In another set of experiments, all otopathogens were subjected to free ceragenins and CSA-containing nanosystems in the presence of human cerumen. Cerumen samples were collected from adult healthy volunteers under approval of the Bioethics Committee at the Jan Kochanowski University in Kielce, Faculty of Medicine and Health Sciences (no. 20/2019). Informed written consent has been obtained from the participants involved. In detail, human cerumen samples were collected with a sterile earwax hook from six healthy individuals and kept in a sterile tube at -80°C until use. All collected samples were free from bacterial or fungal contamination, as confirmed by seeding of cerumen samples on chocolate agar plates. The pooled cerumen was mixed thoroughly, weighed and suspended in a buffer containing of 5% of sodium bicarbonate (NaHCO3) and 30% glycerol (pH ∼ 8.2) at a concentration of 10% (weight/volume) [Citation48]. Bactericidal activity of the tested compounds was performed using the colony-counting method [Citation47] using a final concentration of antimicrobials of 0.5, 1 and 5 μg/ml and 30% glycerol and 5% NaHCO3 buffer.
Antibiofilm assay
Overnight cultures of H. influenzae ATCC 49766, M. catarrhalis ATCC 25238 and S. pneumoniae ATCC 49619 were brought in brain–heart infusion broth (BHI) to 2 × 107 CFU/ml, incubated with three concentrations (5, 20 and 50 μg/ml) of the tested compounds for 24 h at 37°C in 5% CO2 and stained using crystal violet as previously described [Citation49].
Investigation of mechanism of killing activity
Mechanisms by which developed nanosystems exert killing activity against AOM pathogens were investigated using fluorimetric and colorimetric probes recording permeability of outer membrane (NPN; final concentration of 40 μM), formation of reactive oxygen species (DCFH-DA; final concentration of 20 μM) and leakage of intracellular content from damaged bacterial cells (Bradford reagent; final concentration of 20%) according to previously published protocols [Citation42,Citation43]. Because the NPN assay is used for only Gram-negative bacteria, which is related to the need to enter the probe into the periplasmatic space, this test was not performed in relation to S. pneumoniae. To perform the preceding experiments, overnight cultures of bacteria were brought to OD600 ∼0.1 and exposed to the tested compounds in concentrations ranging from 5 to 50 μg/ml at 37°C, 5% CO2 for 10 min (for NPN assay) or 1 h (for ROS generation and protein leakage measurements). To clarify, the larger number of bacteria, and in turn the concentrations of agents, were adopted to the detection limit, that is, 107 CFU/ml of these methods. The incorporation of NPN in CSA-treated bacteria was detected at an excitation wavelength of 355 nm and emission wavelength of 405 nm. Generation of ROS was recorded fluorimetrically using excitation/emission wavelengths of 488/535 nm. Absorbance of Bradford reagent-incubated samples was measured at 595 nm.
Cell culture
Immortalized adult human skin keratinocytes cells (HaCaT), purchased from CLS Cell Lines Service (Eppelheim, Germany), were grown in high-glucose Dulbecco’s Modified Eagle Medium (DMEM) supplemented with 10% fetal bovine serum (FBS), glutamine (2 mM/l), penicillin (50 U/ml) and streptomycin (50 μg/ml) and maintained at 37°C in an atmosphere containing 5% CO2 with saturated humidity.
Determination of cell cytotoxicity
The cell cytotoxicity effects of tested compounds against human keratinocytes were determined using MTT assay. Previously reported methods have been implemented [Citation50].
Measurement of IL-8 concentration
To elucidate whether free ceragenins and CSA-based nanoformulations are able to decrease pathogen-induced inflammatory responses, release of IL-8 from bacteria-stimulated keratinocytes was measured using enzyme-linked immunosorbent assay (ELISA) [Citation50]. For this purpose, HaCaT cells at 1 × 104 density were seeded onto each well of 96-well plates and cultured until confluence of ∼85% was reached. In the next step, culture medium was replaced with H. influenzae ATCC 49766-infected DMEM (bacteria brought to OD600 ∼0.1) supplemented with tested agents at doses of 5 and 10 μg/ml. IL-8 secretion from bacteria-infected cells was measured in supernatant collected after 3 h incubation at 37°C, 5% CO2 using IL-8 Human ELISA Kit.
Statistical analysis
Data are reported as a mean ± standard deviation. Statistical significance was determined by two-tailed Student’s t-test using OriginPro 9.65 software (OriginLab, MA, USA).
Results
Synthesis & physicochemical analysis of developed ceragenin-based nanosystems
Nanosystems consisting of CSA-13, CSA-44 or CSA-131 attached to the surface of AuP NPs were developed using MHDA as a surface linker and investigated in terms of morphology, crystallographic properties and chemical structure using STEM, x-ray diffraction (XRD), FT-Raman technique as well as UV-Vis spectroscopy and calorimetric methods (). The scheme of the biofunctionalization and immobilization process of AuP NPs is shown in A. Bright field STEM images of AuP NPs showed that the synthesized nanoparticles have a peanut shape with an average size along the longitudinal axis around 60 nm and along the transverse axis ∼30 nm (B) [Citation41]. The size spread of the AuP NPs is negligible, as determined from measurements of 100 randomly selected nanopeanuts [Citation41]. Structural characterization of the synthesized nanoparticles was acquired using x-ray diffraction (C). The XRD pattern of the AuNPs was refined using Rietveld method. Within the detection limit, all peaks were attributed to the standard Bragg reflections (111), (200), (220), (311) and (222) of Au nanocrystals with face-centered cubic lattice [Citation51,Citation52] and the calculated cell parameter of the AuP NP was 4.078 Å with uncertainties of ∼0.001 Å. Successful AuP NP biofunctionalization and immobilization by ceragenin, as well as chemical stability of the nanosystem combined with AuP NPs and ceragenin (AuP@CSA-13, AuP@CSA-44, AuP@CSA-131, respectively), was determined by FT-Raman spectroscopy (D). Gold nanopeanuts underwent a reaction with the thiol group from MHDA resulting in AuP NP functionalization with a COOH group, which was further used to attach ceragenin to the AuP NPs. Therefore, in the FT-Raman spectra of AuP NP + MHDA and nanosystems, the disappearance of the peak originating from the thiol group (2743 cm-1) confirms a successful attachment of the MHDA to the surface of gold nanopeanuts [Citation4]. Furthermore, in the FT-Raman spectra presented in D, peak at 278 cm-1 corresponding to Au-S stretching vibrations were visible. These bonds are responsible for creating a connection in between surface of AuNPs and sulphur from MHDA [Citation53]. Moreover, in the FT-Raman spectra of AuP NP + MHDA + ceragenin, peaks corresponding to the C=O vibrations (1680 cm-1) were observed [Citation54]. This group is responsible for linking ceragenin with biofunctional surfactants on the AuP NP surface [Citation55]. Both observations provide evidence of the success of AuP NP biofunctionalization and ceragenin immobilization. E shows SPR peaks of ~725 nm (black spectrum) in the UV-Vis absorption spectra. Comparing the UV-VIS spectra of synthesized AuP NPs with the nanoparticles functionalized with ceragenins (spectra red, blue and green), a shift to higher wavelengths of the respective AuP NPs peaks by about 30 nm was observed. This shift indicates that the immobilization of ceragenins on the nanoparticles surface indeed occurred, because the size of the AuP NPs increased as a result of anchoring ceragenins molecules on the nanoparticles. Indeed, it is well known that with increasing particle size, the band gap of the nanogold decreases, resulting in a shift of the SPR peak toward higher wavelength values [Citation56]. Taken together the results from calorimetric experiments indicated that all the samples remained thermally stable up to 100°C at a 5°C min-1 heating rate (F). Moreover, the DSC thermogram displayed no signs of any significant thermal anomalies up to 150°C. The anomalies describe earlier indicate that temperature, as well as the baseline shift, are due to the rapid boiling and sample decomposition or destabilization of MHDA [Citation57].
(A) Scheme of the AuP NPs biofunctionalization and ceragenin (CSA) immobilization process. (B) Overview scanning transmission electron microscopy image. (C) X-ray diffraction (XRD) taken for AuP NPs. Experimental (blue dots) and calculated (solid black line) Rietveld refinement plot of the XRD pattern for the AuP NPs. The red line (YOBS–YCALC) represents the difference between the observed and calculated data. Tick marks show allowed Bragg reflections. (D) FT-Raman spectra of nanosystems: AuP NPs + MHDA + CSA-13 (red spectrum); AuP NPs + MHDA + CSA-44 (blue spectrum); AuP NPs + MHDA + CSA-131 (green spectrum). (E) Ultraviolet-Vis spectra of AuP NPs (black spectrum) and AuP NPs + MHDA + CSA-13 (red spectrum); AuP NPs + MHDA + CSA-44 (blue spectrum); AuP NPs + MHDA + CSA-131 (green spectrum). (F) Thermogravimetric analysis and differential scanning calorimetry data of AuP NPs + MHDA and AuP@CSA-13 (red curve), AuP@CSA-44 (blue curve), AuP@CSA-131 (green curve), showing the solvent removal and decomposition of the product.
AuP NP: Gold peanut-shaped nanoparticle; CSA: Cationic steroid antimicrobial; FT: Fourier transform; MHDA: Mercaptohexadecanoic acid.
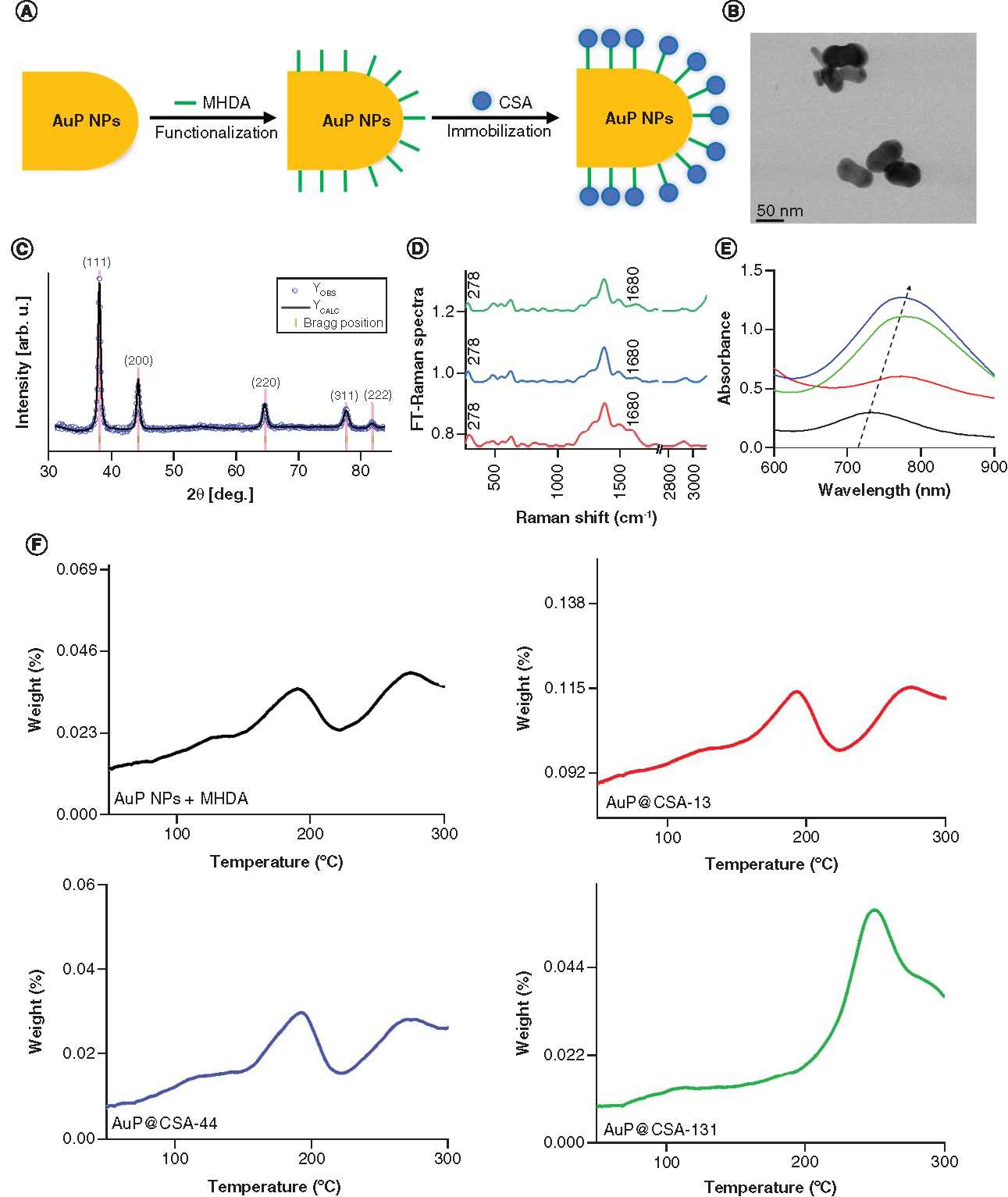
Conjugation of ceragenins with Au NPs augments their bactericidal & antibiofilm activity
The colony-counting method (killing assay) was employed to estimate bactericidal activities of ceragenins and ceragenin-containing nanosystems against H. influenzae ATCC 49766, M. catarrhalis ATCC 25238 and S. pneumoniae ATCC 49619 strains. As demonstrated in , all ceragenins exert bactericidal activity against H. influenzae ATCC 49766, M. catarrhalis ATCC 25238 and S. pneumoniae ATCC 49619 (, panels A1, B1 & C1, respectively) in a concentration range from 0.5 to 10 mg/ml, with the exception of CSA-13, which was able to eradicate H. influenzae bacteria at 20 μg/ml. Importantly, conjugation of the ceragenins with Au NPs strongly enhanced their killing efficiency, as all CSA-based nanosystems (AuP@CSA-13, AuP@CSA-44 and AuP@CSA-131) eradicated the tested otopathogens in doses ranging from 0.5 to 5 μg/ml. To more quantitively compare the efficiency of tested agents against AOM pathogens, we estimated doses that are required to limit the survival of bacterial population to 100 CFU/ml (2 log [CFU/ml], i.e., colony-counting assay detection limit) (, panels A2–C2). Accordingly, CSA-131 was recorded as the most potent ceragenin, limiting the colony-forming ability of tested bacteria at doses of 1.60 ± 0.01, 0.62 ± 0.01 and 3.51 ± 0.15 μg/ml (for H. influenzae, M. catarrhalis and S. pneumoniae, respectively). Notably, in nearly all comparisons, CSA-based nanosystems were far more active than free ceragenins. This effect was particularly prominent for CSA-13 because treatment of H. influenzae with AuP@CSA-131 allowed to decrease effective dose of agent nearly 50-fold, from 16.11 ± 0.2 μg/ml to 0.313 ± 0.01 μg/ml (, panel A2). A similar tendency was maintained in the presence of high nutritious medium. As presented in , MIC and MBC values ranged for free ceragenins from 0.5 to 4 μg/ml and 0.5 to 16 μg/ml, respectively. In that respect, the nanosystems were at least equally effective or more potent than ceragenins alone, except AuP@CSA-131 against H. influenzae ATCC 49766 and S. pneumoniae ATCC 49619, which showed one dilution higher MIC/MBC values (1/1 μg/ml vs 2/2 μg/ml).
Activity of ceragenins CSA-13 (black squares), CSA-44 (red circles), CSA-131 (blue triangles) and ceragenin-containing nanosystems, AuP@CSA-13 (green squares), AuP@CSA-44 (purples circles) and AuP@CSA-131 (gold triangles) against (A)Haemophillus influenzae ATCC 49766, (B)Moraxella catarrhalis ATCC 25238 and (C)Streptococcus pneumoniae ATCC 49619 strains was evaluated. For each strain, logarithm of number of colonies outgrown [log (CFU)] (panels A1, B1 and C1) and concentration of compound reducing bacteria survival to 2 log (CFU/ml) was calculated (panels A2, B2 and C2). Results are presented as mean ± standard deviation from three replicates.
* and ∧ indicates statistical significance p < 0.05 when compared to untreated control and free ceragenins, respectively. (Where there is more than one *, the values overlap).
ATCC: American Type Culture Collection; AuP: Gold peanut-shaped nanoparticle; CFU: Colony-forming unit; CSA: Cationic steroid antimicrobial.
![Figure 2. Bactericidal activity of tested compounds against otitis media-causing pathogens.Activity of ceragenins CSA-13 (black squares), CSA-44 (red circles), CSA-131 (blue triangles) and ceragenin-containing nanosystems, AuP@CSA-13 (green squares), AuP@CSA-44 (purples circles) and AuP@CSA-131 (gold triangles) against (A)Haemophillus influenzae ATCC 49766, (B)Moraxella catarrhalis ATCC 25238 and (C)Streptococcus pneumoniae ATCC 49619 strains was evaluated. For each strain, logarithm of number of colonies outgrown [log (CFU)] (panels A1, B1 and C1) and concentration of compound reducing bacteria survival to 2 log (CFU/ml) was calculated (panels A2, B2 and C2). Results are presented as mean ± standard deviation from three replicates.* and ∧ indicates statistical significance p < 0.05 when compared to untreated control and free ceragenins, respectively. (Where there is more than one *, the values overlap).ATCC: American Type Culture Collection; AuP: Gold peanut-shaped nanoparticle; CFU: Colony-forming unit; CSA: Cationic steroid antimicrobial.](/cms/asset/3de11caa-cae2-43f5-8d4a-79f94dcd31bf/innm_a_12342005_f0002.jpg)
Table 1. MIC and MBC of ceragenins CSA-13, CSA-44 and CSA-131 in free form and attached to the surface of AuPs against laboratory strains of H. influenzae, M. catarrhalis and S. pneumoniae.
Furthermore, substantial antibiofilm activities were recorded for the tested agents in concentrations from 5 to 20 μg/ml, resulting in a decrease of biofilm mass up to 90%. Importantly, when comparing the formation of biofilm at the lowest doses tested, that is, 5 μg/ml, it is prominent that a mass of biofilm formed in the presence of ceragenins varied between 95.96 ± 18.58% and 21.61 ± 2.28%, whereas for CSA-based nanosystems, this effect was considerably stronger because detected biofilm mass ranged from 42.51 ± 7.05% to 22.31 ± 2.01% (). To more accurately elucidate how nontoxic doses of the developed nanomaterials affect the formation of biofilms, we estimated viability of biofilms on treatment with 10 μg/ml of CSA/AuP@CSA – that is, the doses demonstrating antibacterial activity in killing assay, but still safe for mammalian cells (, panels A2–C2). As demonstrated, biofilm mass at this endpoint ranged from 21.09 ± 2.14 to 87.07. ± 22.82% (for ceragenin-treated samples) and from 19.45 ± 5.18 to 36.71 ± 7.44% (for nanosystem-treated biofilms) compared with untreated samples. This indicates that ceragenin-containing nanosystems effectively inhibit the formation of biofilms by AOM pathogens at doses significantly lower than free ceragenins and are able to exert therapeutic effect at doses which are recognized as nontoxic for host cells.
Prevention of biofilm formation by (A)Haemophillus influenzae ATCC 49766, (B)Moraxella cattarhalis ATCC 25238 and (C)Streptococcus pneumoniae ATCC 49619 bacteria treated with ceragenins CSA-13, CSA-44, CSA-131 and CSA-based nanosystems (AuP@CSA-13, AuP@CSA-44, AuP@CSA-131) at concentrations of 5 (gold bars), 20 (green stripped bars) and 50 μg/ml (blue bars) (panels A1, B1 and C1). On the basis of the recorded dose–response curves, mass of biofilm formed in the presence of 10 μg/ml of compound was calculated (panels A2, B2 and C2). Results are presented as mean ± standard deviation from three replicates.
* and ∧ indicate statistical significance (p < 0.05) compared with untreated control and free ceragenins, respectively.
ATCC: American Type Culture Collection; AuP: Gold peanut-shaped nanoparticle; CSA: Cationic steroid antimicrobial.
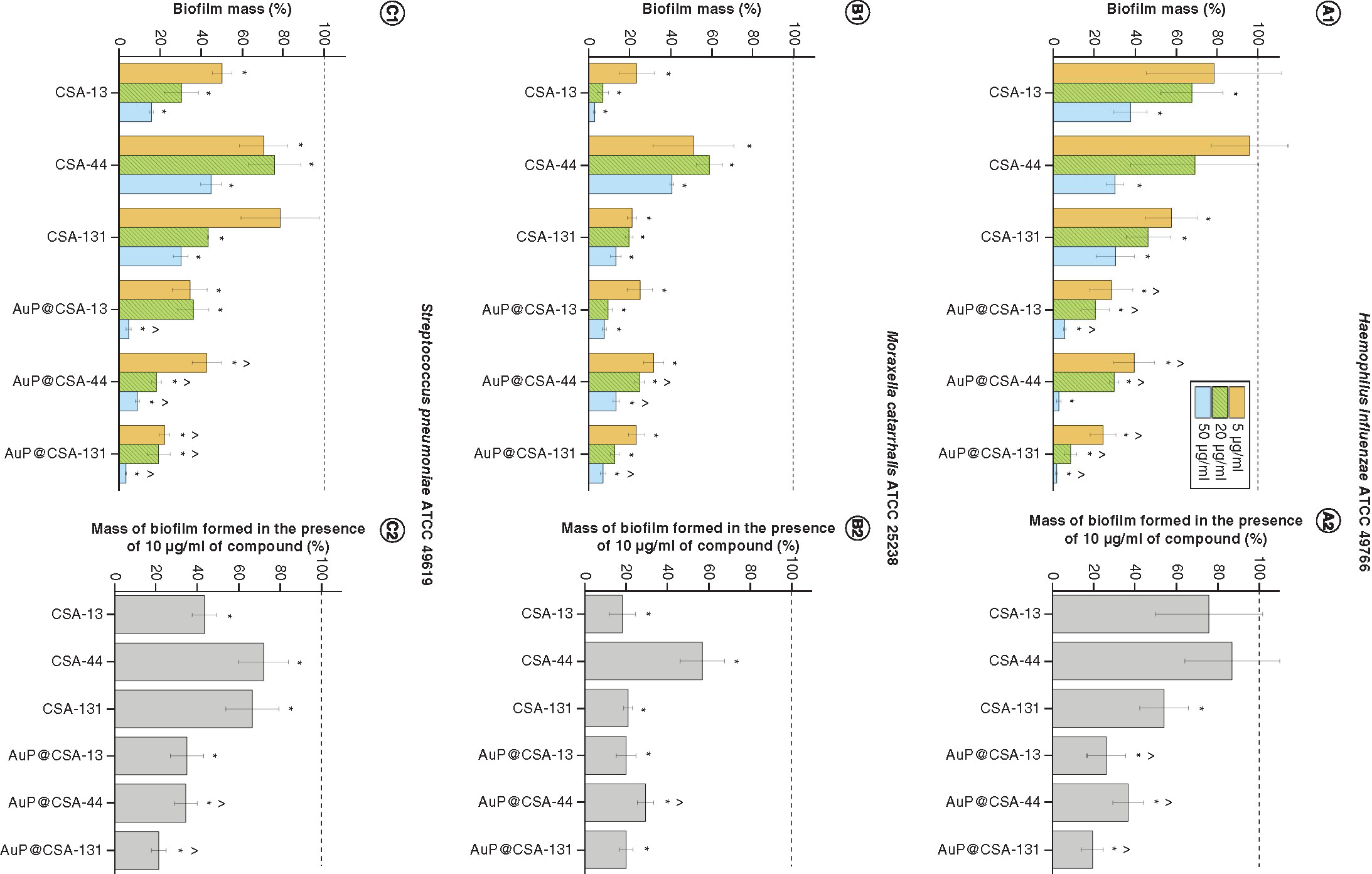
Bactericidal activity of CSAs & CSA-containing nanosystems is not affected by human cerumen
As shown in , the bactericidal effects of the tested compounds are not only preserved but even augmented in the presence of the human cerumen. In detail, in a great majority of tested CSA/AuP@CSA comparisons at concentrations ranging from 0.5 to 5 μg/ml, reduction of bacterial growth log (CFU/ml) values were significantly lower in 10% cerumen-containing samples, compared with controls without cerumen. In accordance with previous results, all tested bacterial strains, in particular H. influenzae ATCC 49766 (A) and S. pneumoniae ATCC 49619 (C), were more susceptible to AuP@CSAs than to ceragenins alone.
Activity of ceragenins CSA-13, CSA-44, CSA-131 and ceragenin-containing nanosystems AuP@CSA-13, AuP@CSA-44 and AuP@CSA-131 at doses of 0.5, 1 and 5 μg/ml was tested against (A)Haemophillus influenzae ATCC 49766, (B)Moraxella catarrhalis ATCC 25238 and (C)Streptococcus pneumoniae ATCC 49619. Results are presented as mean ± standard deviation from three replicates.
* and ∧ indicate statistical significance p < 0.05 compared with untreated controls and free ceragenins, respectively.
#indicates statistical significance p < 0.05 compared with viability of bacteria in 10% CB and phosphate-buffered saline.
ATCC: American Type Culture Collection; AuP: Gold peanut-shaped nanoparticle; CB: Cerumen buffer; CSA: Cationic steroid antimicrobial; CFU: Colony-forming unit; PBS: Phosphate-buffered solution.
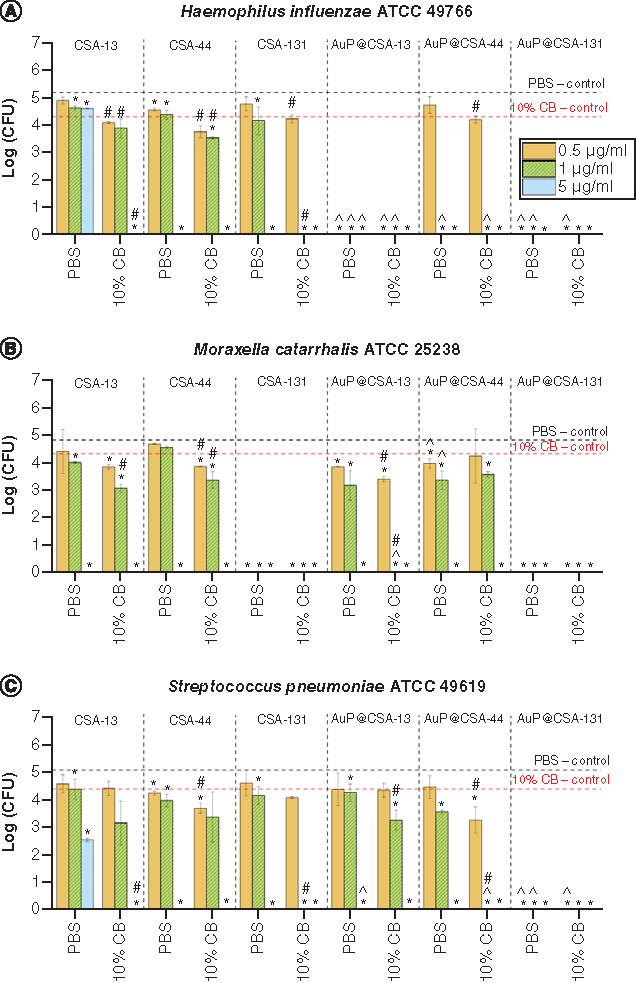
Mechanism of AuP@CSA-mediated killing involves generation of oxidative stress followed by permeabilization of bacterial membranes
For the purpose of killing mechanism determination, fluorimetric and colorimetric methods were employed. As demonstrated in , the tested agents induced intracellular ROS production in H. influenzae ATCC 49766 and S. pneumoniae ATCC 49619, but not by M. catarrhalis ATCC 25238 strains. This effect was significantly more pronounced for CSA-based nanosystems than free CSAs, in particular for S. pneumoniae, where CSA-13 and CSA-44 in doses >20 μg/ml triggered substantially less ROS production than their counterparts: AuP@CSA-13 and AuP@CSA-44 (C). As expected, AuP NP-based nanosystems more effectively interacted with bacterial membranes, as evidenced by the NPN () and Bradford assay (). By measurement of entry of NPN into periplasmic space of Gram-negative bacteria, it is possible to estimate the membrane-permeabilizing properties of tested compounds [Citation43]. As demonstrated in , in both tested Gram-negative pathogens incubation of bacteria with nanopeanuts decorated with CSA-13 and CSA-44 resulted in a far stronger permeabilization of the outer membrane than CSAs alone, indicating increased interaction with the bacterial surface. Although at the 5 μg/ml concentration there were no significant differences between free ceragenins and CSA-based nanoformulations, a strong increase in NPN fluorescence was observed at a dose of >20 μg/ml (). Likewise, CSA-based nanosystems exerted more intense protein leakage from the treated bacterial strains, highlighting better efficiency of CSA-based nanosystems than ceragenin alone ().
ROS-inducing abilities of ceragenins CSA-13, CSA-44, CSA-131 and ceragenin-containing nanosystems AuP@CSA-13, AuP@CSA-44 and AuP@CSA-44 at a dose of 5 μg/ml (gold bars), 20 μg/ml (green stripped bars) and 50 μg/ml (blue bars) were tested against (A)Haemophillus influenzae ATCC 49766, (B)Moraxella catarrhalis ATCC 25238 and (C)Streptococcus pneumoniae ATCC 49619 (panel C). Results are presented as mean ± standard error of the mean from three to six individual experiments with three replicates each.
* and ∧ indicate statistical significance (p < 0.05) compared with untreated control and free ceragenins, respectively.
ATCC: American Type Culture Collection; AuP: Gold peanut-shaped nanoparticle; CSA: Cationic steroid antimicrobial; ROS: Reactive oxygen species.
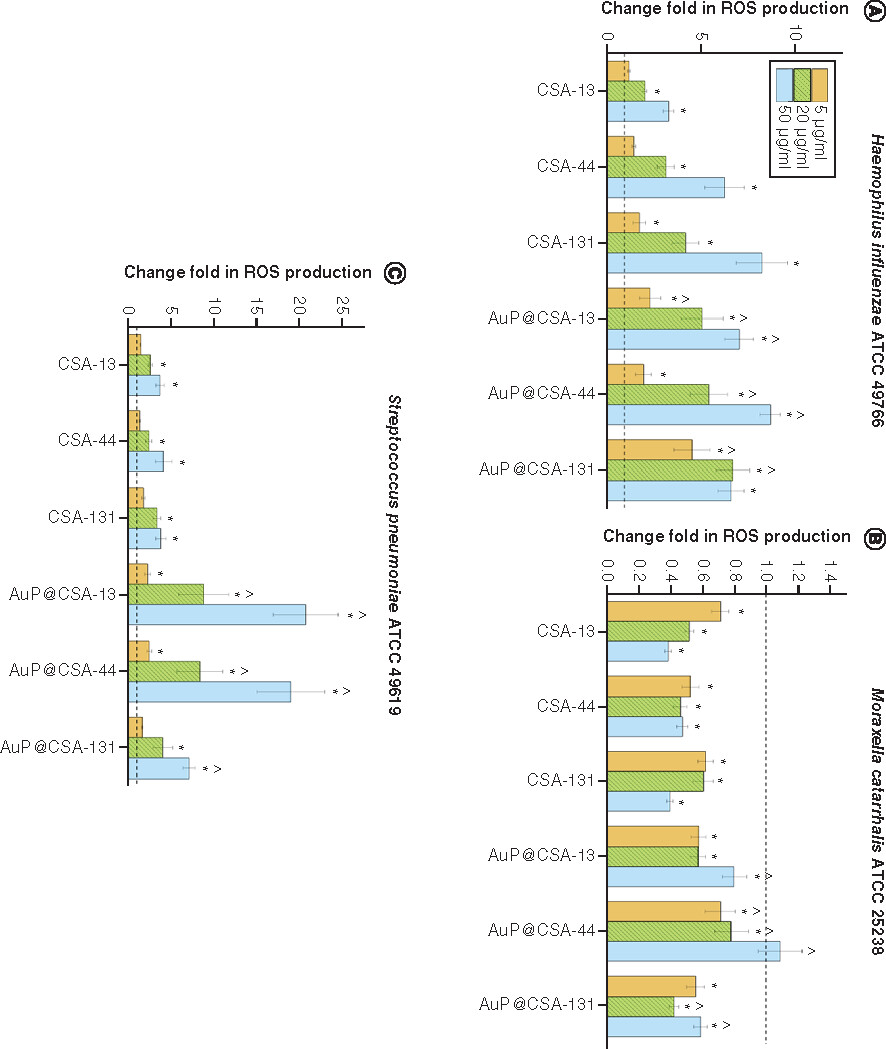
Disruption of bacterial membrane in (A)Haemophillus influenzae ATCC 49766 and (B)Moraxella catarrhalis ATCC 25238 bacteria treated with ceragenins CSA-13, CSA-44, CSA-131 and CSA-based nanosystems (AuP@CSA-13, AuP@CSA-44 and AuP@CSA-131) at concentrations of 5 (gold bars), 20 (green stripped bars) and 50 μg/ml (blue bars). Results are presented as mean ± standard deviation from two individual experiments with three replicates each.
* and ∧ indicate statistical significance p < 0.05 compared with untreated control and free ceragenins, respectively.
ATCC: American Type Culture Collection; AuP: Gold peanut-shaped nanoparticle; CSA: Cationic steroid antimicrobial; NPN: N-phenyl-1-naphthylamine.
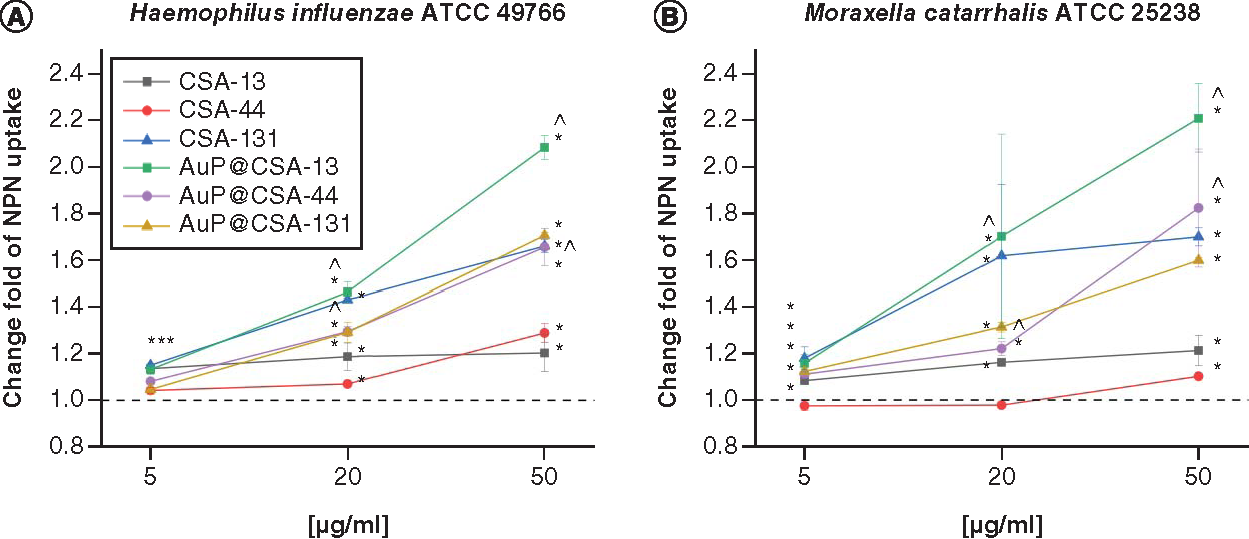
Release of proteins from (A)Haemophillus influenzae ATCC 49766, (B)Moraxella cattarhalis ATCC 25238 and (C)Streptococcus pneumoniae ATCC 49619 bacteria treated with ceragenins CSA-13, CSA-44, CSA-131 and CSA-based nanosystems (AuP@CSA-13, AuP@CSA-44, AuP@CSA-131) at concentrations of 5 (gold bars), 20 (green stripped bars) and 50 μg/ml (blue bars). Results are presented as mean ± standard deviation from three replicates.
* and ∧ indicate statistical significance (p < 0.05) compared with untreated control and free ceragenins, respectively.
ATCC: American Type Culture Collection; AuP: Gold peanut-shaped nanoparticle; CSA: Cationic steroid antimicrobial.
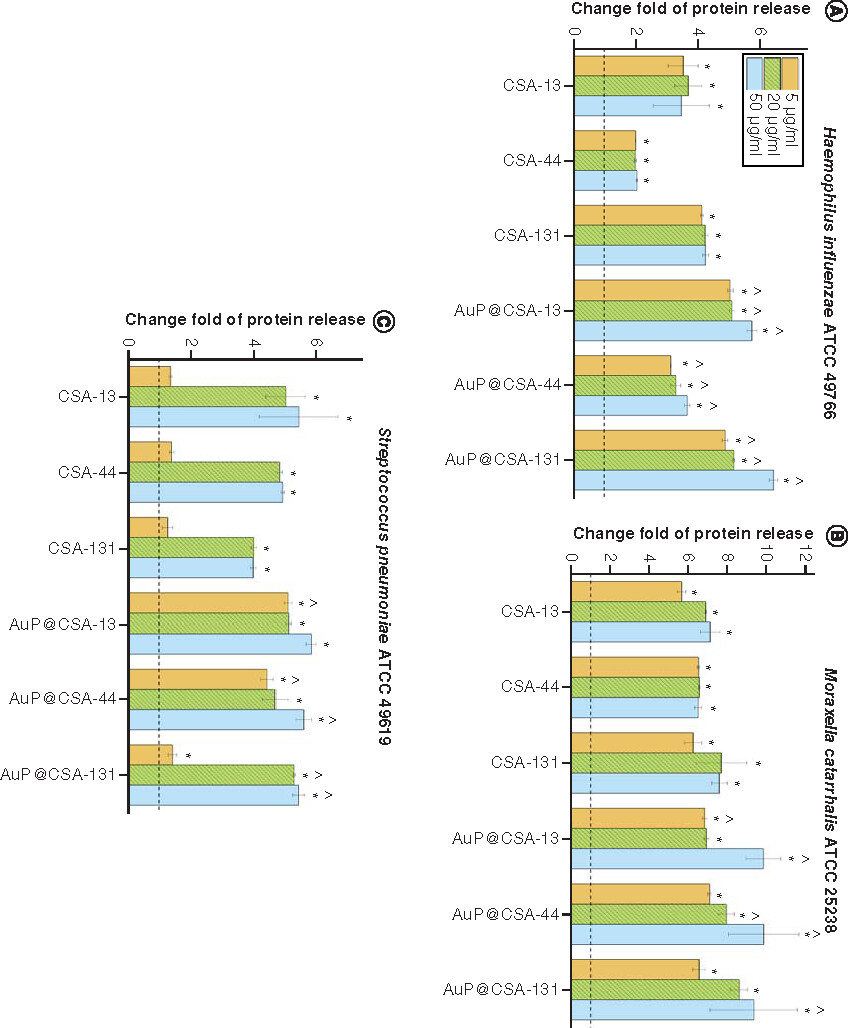
CSAs & CSA-containing nanosystems display promising biocompatibility & anti-inflammatory activity in bactericidal concentrations
As presented in A, the bactericidal concentrations (i.e., 1–10 μg/ml) of ceragenin-based compounds exerted an acceptable toxicity on human keratinocytes, leading to decrease of metabolic activity in no more than 30% of these cells (with the exception on AuP@CSA-13, which was more toxic than other nanoformulations). Simultaneously, due to the immobilization of ceragenins on the surface of Au NPs, decreased inflammatory response in keratinocytes stimulated by H. influenzae compared with free CSAs was observed (B). In detail, the former reduced IL-8 release from 1482 to 477.8–657.8 pg/ml, whereas for CSAs this reduction was less pronounced (782.8–1112.5 pg/ml). These results suggest that the developed nanosystems exert both potent bactericidal and antiinflammatory activity, which would be favorable in the treatment of OM infections.
(A) Cytotoxicity of ceragenins CSA-13, CSA-44, CSA-131 and CSA-containing nanosystems AuP@CSA-13, AuP@CSA-44 and AuP@CSA-131 in relations to human keratinocytes (HaCaT) treated with 24 h with tested agents at concentrations ranging from 1 to 20 μg/ml. (B) Decrease of IL-8 release from human keratinocytes stimulated with H. influenzae ATCC 49766 bacteria and treated with tested agents for 24 h. Results are presented as mean ± standard deviation from three replicates.
* and ∧ indicate statistical significance (p < 0.05) compared with untreated control and free ceragenins, respectively. (Where there is more than one *, the values overlap).
AuP: Gold peanut-shaped nanoparticle; CSA: Cationic steroid antimicrobial.
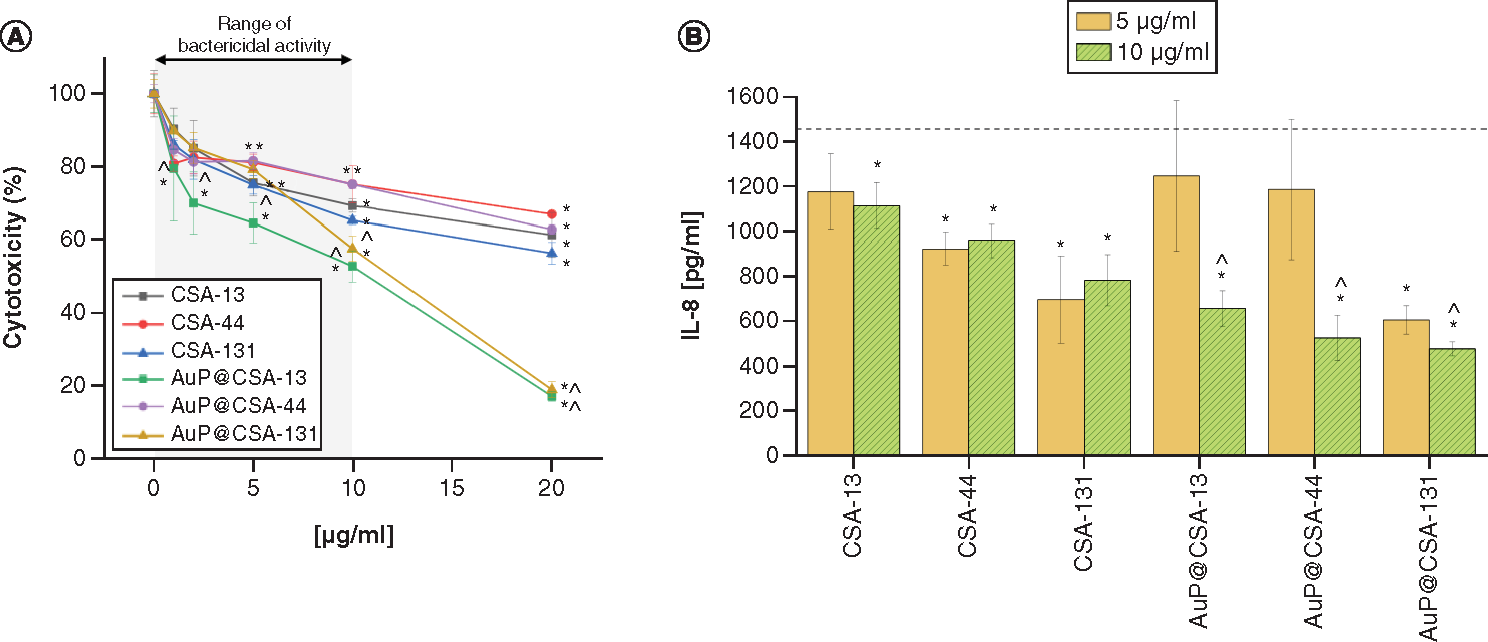
Discussion
Given the common occurrence of persistent acute OM infections and relatively high administration of antibiotics in the pediatric population [Citation58,Citation59], novel approaches for treatment OM infections are needed. In line with these, we assessed the efficacy of ceragenins and their corresponding Au NP formulations against the three leading pathogens of OM.
Due to their favorable physical features, including size range and high surface-to-volume ratio, Au NPs, particularly nonspherical NPs, should be considered as antimicrobials with clinical potential. Recently, we demonstrated that Au NPs with morphology other than spheres (i.e., rod-, peanut- and star-shaped) display potent killing activity against a spectrum of bacterial and fungal pathogens, regardless of their resistance to conventional antibiotics, at concentrations as low as ng/ml, which is determined by their membrane-permeabilizing capabilities and ROS-promoting activities [Citation41]. Moreover, we demonstrated that gold nanorods are highly effective against Candida strains isolated from patients suffering from hemato-oncological conditions and filamentous fungi from Aspergillus, Cladosporium or Fusarium genera [Citation42]. Importantly, nonspherical Au NPs were noted to decrease the formation and viability of pathogenic biofilms, which results from Au-NP-mediated ability to decrease the adhesion of microbes to abiotic surfaces [Citation41,Citation42]. Those reports strongly justify the further research on usefulness of these nanomaterials in therapy of drug-resistant pathogens. To date, the application of Au NPs in middle-ear infections has not been explored. With the advancement of nanomedicine, targeted drug-delivery systems and nanotherapeutics incorporating silver NPs with bactericidal activity in middle-ear prosthesis have been reported [Citation60,Citation61]. Also, silver and magnetic nanoparticles were tested as contrast agents for ear imaging including MRI and CT scans. In the majority of previous studies, the potency of differently shaped Au NPs was implemented for the eradication of Pseudomonas aeruginosa and S. aureus biofilms which commonly associate with otitis externa (swimmer’s ear) [Citation40,Citation41,Citation62]. Notably, according to our best knowledge, there is no literature on the bactericidal effects and toxicity of Au NP application to the middle-ear mucosa. However, studies on the use of Au NPs for inner-ear imaging investigated its efficacy with no toxicity to mouse cochlear cell lines (HEI-OC1), which encourages the future tests in this aspect [Citation63].
In addition to aforementioned research, a compelling number of studies provide the evidence on utility of Au NPs as platforms for immobilization of therapeutics and other functional ligands on their surface. To date, multiple studies confirm that attachment of conventional antibiotics to the surface of metallic NPs via either noncovalent interactions [Citation64] or covalent bonds [Citation65] might be used to combat MDR bacteria, including MRSA or Acinetobacter baumannii because both of these methods have been reported to enhance killing efficiency against pathogenic bacteria compared with free, unconjugated antibiotics [Citation35,Citation36]. Reasons for such phenomena include both improved internalization of antibiotics into the bacterial cells, as well synergistic effects between NPs and the attached antimicrobial without the disturbance of the inherent mechanism of action of the antibiotic [Citation66]. Similarly, antimicrobial activity of ceragenins might also be modulated, as we demonstrated previously using iron oxide NPs as drug nanocarriers [Citation67,Citation68]. We verified that through NP-assisted, enhanced intracellular internalization of CSA and increased ability of compounds to generate ROS, a considerable membrane perturbation and leakage of intracellular content occurs [Citation67,Citation68]. Nevertheless, a majority of such developed nanosystems is based on spherical-shaped nanoparticles [Citation35,Citation36]. A compelling amount of data presented to date clearly point out the possibility of modifying the biological activity of Au NPs by controlling their size and shape, making them more therapeutically efficient and allowing them to attach more therapeutic compound on their surface [Citation39–42]. In general, nonspherical Au NPs, including those with flower, star or rod morphology [Citation40,Citation69], are reported to exert enhanced bactericidal effects due to intensified ROS generation, which leads to excessive oxidative stress and enhanced bacterial membrane rupture [Citation40,Citation69]. Considering these promising reports, an attempt has been made to employ nonspherical nanomaterials as ceragenins carriers [Citation43]; nevertheless their effectiveness in the presence of body secretions has not been tested to date. In this study, we used newly-developed, non-spherical AuNPs in the shape of nanopeanuts as nanocarriers for ceragenins CSA-13, CSA-44 and CSA-131. Notably, we observed the augmentation of antibacterial efficiency of ceragenins after their conjugation with Au NPs; however, the magnitude of this effect appears to be strongly dependent on a type of ceragenin and/or bacterial species as well as methodology used for its estimation. For example, the MIC/MBC values for AuP@CSA-13 and AuP@CSA-44 nanoparticles are twofold and threefold lower in comparison to CSA-13 and CSA-44 in a free form, but only against M. catarrhalis, whereas their activity, along with AuP@CSA-131, against S. pneumoniae and H. influenzae is comparable to CSAs alone in MIC/MBC testing. On the other hand, Au NPs clearly enhance the bactericidal action of ceragenins against S. pneumoniae in killing assay experiments. Hypothetically, such phenomena could be achievable due to potent activity of AuP NPs themselves and synergistic interactions between ceragenins and gold nanomaterials. According to our previously published data, Au NPs in shape of peanuts, similarly as those rod- and star-shaped [Citation41,Citation42], exert nanogram killing efficiency against spectrum of bacterial and fungal pathogens. Nevertheless, based on calculation of gold content in developed nanoformulations we exclude the possibility of synergistic interactions between AuP NPs and CSAs. As stated above, our developed nanosystems consist of two compounds: 2 mg/ml ceragenin (CSA-13, CSA-44 or CSA-131) and 2.93 ng/ml of nonspherical AuNPs. On this basis, we estimated that 5 μg/ml of Au NPs@CSA-131 (the highest bactericidal dose of nanosystems recorded using colony-counting measurements) includes 0.007325 ng/ml (7.325 pg/ml) of Au NPs. To test whether such amount of AuP NPs might affect the viability of OM pathogens, we performed an additional colony counting-based measurement. As recorded, such small concentrations of AuNPs does not exert any killing efficiency (Supplementary Figure 1), which is why we state that more potent activity of nanosystem depends on local immobilization of ceragenin CSA-131 on the surface of Au NPs than on additive effects of gold and ceragenin.
In our study, all CSAs and their nanosystems demonstrate strong activity against bacteria in biofilms – structures that play crucial role in pathogenesis of AOM and recurrent otitis infections as well as factors impeding their successful treatment. For instance, S. pneumoniae and M. catarrhalis are able to create multispecies biofilms where they can protect each other from the activity of macrolide and beta-lactam antibiotics, respectively [Citation70]. Because CSAs are characterized by multiple mode of action and a low risk of resistance development this form of bacterial cooperation is highly unlikely to succeed [Citation27,Citation28]. Importantly, ceragenin-containing nanosystems effectively inhibited the formation of biofilms by OM pathogens at doses significantly lower than free ceragenins. Possibly, this observation is attributed to the increased local concentration of CSA on the surface of AuP NPs inducing stress on the bacterial membrane as well as the electrostatic interactions between the gold nanoparticle functionalized ceragenins and the pathogen [Citation62].
One of the primary antibacterial mechanism of ceragenins is their selective association with bacterial membranes, especially with Gram-negative bacteria, altering the cell morphology and microbial survival [Citation18,Citation71]. Likewise, metal nanoparticles also easily induce membrane permeabilization [Citation72]. It must be noted that multiple antibacterial mechanisms of Au NPs against Gram-positive and Gram-negative bacteria have been reported. Most commonly, depolarization of membrane potential, cell wall damage, DNA fragmentation, oxidation of cell membrane fatty acids and inhibition of protein synthesis [Citation40,Citation41,Citation73]. Similarly, nonspherical Au NPs were noted to decrease the formation and viability of pathogenic biofilms, which results from Au-NP-mediated ability to decrease the adhesion of microbes to abiotic surfaces [Citation41,Citation42]. Those reports strongly justify the further research on usefulness of these nanomaterials in therapy of drug-resistant pathogens. Because induction of ROS is recognized as one of the mechanisms determining bacterial killing by CSAs [Citation74], we quantified intracellular ROS generation in all otopathogens with a DCFH-DA fluorescent probe [Citation67,Citation75,Citation76]. Interestingly, a dose-dependent increase in ROS generation was recorded only for AuP@CSA-13 and AuP@CSA-44 in S. pneumoniae, whereas no significant ROS levels were measured for other compounds. Normally, the cellular systems within the bacteria maintain a redox state of balance between ROS generation and detoxification through various antioxidant defense strategies. As reported by Zhao et al., oxidative stress intermediates can act as bifunctional factors such that at low stress levels, they potentiate the release of protective enzymes, while cellular dysfunction and bacterial death follows excessive stress production [Citation77]. Therefore, in the latter case, other mechanisms of CSA nanosystems action are likely involved. For instance, it is noteworthy, that in S. pneumoniae the antibacterial effects of CSAs may be at least partially correlated with its natural autolytic behavior, that is, production of LytA autolysin [Citation20]. Likewise, the effect of AuNPs inhibited the viability of S. pneumoniae without modulating the release of ROS but rather through formation of inclusion bodies of Au NPs within the bacteria [Citation78,Citation79]. In contrast, Au NPs enhanced the intracellular release of ROS and inhibited the energy metabolism and transcription process as a mechanism of killing in S. aureus [Citation80]. An accumulation of ROS in response to treatment with CSA compounds, in particular CSA-131, AuP@CSA-13, AuP@CSA-131, AuP@CSA-44, was also recorded in H. influenzae, but surprisingly not in M. catarrhalis. Notably, M. catarrhalis shows relatively high innate level of resistance to exogenous oxidative stress, i.e., killing by exogenous H2O2, compared with H. influenzae or S. pneumoniae [Citation81]. Thus, a presence of robust mechanisms responsible for dealing with this form of stress was suggested for this bacterium [Citation82]. Although the molecular background behind this phenomenon is unknown [Citation82], it may be associated with the nasopharyngeal mucosa niche occupied by M. catarrhalis as well as the lungs of patients with chronic obstructive pulmonary disease, who exhale more H2O2, especially at the time of an exacerbation, than healthy controls [Citation83]. Therefore, differences in activity between the CSAs, aside from the structural diversity of these agents, e.g., lengths of lipid side chains, at least partially may to be attributed to unique traits of some bacterial pathogens. However, it should be noted that ROS measurements using fluorescein based approaches such as DCFDA dye can have some limitations; for example, its lack of direct interaction with intracellular H2O2 is one among others reported [Citation84]. Therefore, ROS assessments using more specific fluorescent probes and alternative detection of lipid peroxidation, SOD/GST enzyme activity can provide more understanding on the possibility of ROS-based killing of the tested compounds. Collectively, the underlying killing mechanism of the tested compounds are not the same for all bacterial species. It appears that the activity of Au NP-based nanosystems is not specific and can have different targets depending on the bacterial species. Therefore, their mechanisms of antimicrobial action remains to be elucidated.
As we presented previously, ceragenins and ceragenin based nanosystems have demonstrated strong antimicrobial activity in the presence of body fluids such as blood plasma, serum, urine, CSF, sputum, abdominal fluid and dental plaque [Citation67,Citation85,Citation86]. Our previous research confirmed the activity of CSAs at the site of infection, and this time we focused on the material for which the influence on the killing abilities of ceragenins and the nanosystems containing them has not yet been determined (i.e., human cerumen). Although cerumen is not commonly associated with middle-ear infections, it should be noted that in the treatment of middle-ear infection, apart from oral antibiotic therapy, an adjuvant therapy in the form of ear drops has also clinical significance. We propose that ceragenins and CSA-based nanosystems possess the great potential to be used as therapeutic agents for local treatment of infections and for this reason, the efficacy of these compounds in the presence of ear secretions should be estimated. Favorably, the bactericidal activity of the ceragenins and their conjugated nanosystems is not only preserved but even augmented in the presence of the human cerumen. This observation possibly may be explained by natural antimicrobial properties of this substance mediated via several AMPs, including hBD1-3, lactoferrin, LL-37, BPI, hSLPI and HNP1-3 [Citation87]. Previous reports demonstrated that ceragenin CSA-13 displays synergistic effects with naturally occurring compounds such as LL-37 peptide, lysozyme and lactoferrin [Citation88]. Most likely, similar effect occurs in our experimental settings, which additionally confirms the utility of ceragenins and CSA-containing nanosystems in the treatment of ear infections. Moreover, slightly acidic pH of cerumen (∼5.4) provides optimal conditions for the synergistic and additive effect of AMPs. However, it is noteworthy that the pH may increase as the result of ear infection, hence acidifying agents such as boric acid are used to support AOM therapy [Citation89].
To introduce novel nanoantibiotics into clinical practice, it is crucial to ensure the adequate biocompatibility against host cells [Citation50]. Notably, AuNPs have emerged as promising candidates for therapeutic and diagnostic applications in oncology owing to their minimal toxic effects [Citation90–92]. To explore whether tested nanoformulations are more selective to bacteria than mammalian cells, we performed cell culture-based experiments using human immortalized keratinocyte HaCaT cell line. HaCaT cells are widely used as a model of eukaryotic system to investigate the toxicity of nanoparticles, with the subsequent ability to release a spectrum of inflammatory cytokines, including IL-8, in the response to infectious factors exposure [Citation50]. Importantly, those cells are also used in a number of in vitro research aiming to understand the tympanic membrane biology [Citation93,Citation94]. In our study, human keratinocytes were used to explore the ability of tested compounds to decrease the release of pro-inflammatory IL-8 in the response to H. influenzae infection. Using this system we demonstrated that an additional benefit from CSAs, in particular their nanosystems, as anti-infective agents is related to their anti-inflammatory activity. Accordingly, collected data present that CSAs on the surface of AuNPs statistically decreased their inflammatory response in comparison to ceragenins alone. This phenomenon might potentially limit the use of other anti-inflammatory drugs, including corticosteroids, which are recognized as an alternative therapy for AOM [Citation95].
Despite these promising preliminary data, there are a few limitations in this research. First, our research was performed using only ATCC strains, and carrying out the experiments with nonsusceptible clinical isolates of S. pneumoniae, H. influenzae and M. catarrhalis would have improved the clinical relevance of the study due to the occurring of genomic variation in antibiotic susceptibility and other virulence genes of these three otopathogens. Second, a biological membrane interaction study with the NPN test was performed only for Gram-negative bacteria. Although the available literature indicates that ceragenins and nanosystems target the membranes of Gram-positive and Gram-negative pathogens to a similar degree, the final confirmation of this issue requires additional experimentation. Finally, to successfully elucidate the antimicrobial mechanisms of CSA and CSA-based nanosystems against OM, in vivo study models or cell lines pertaining to middle-ear epithelial cells mimicking the site of infections should be incorporated. This acknowledges the need for future perspectives on undertaking in vivo efficacy studies of CSA-based nanosystems in OM infection.
Conclusion
Altogether, these observations strongly encourage and justify the development of ceragenins and ceragenin-containing nanoformulations as agents used in treatment of OM. However, a better understanding of the molecular mechanisms implicated in the bactericidal activity of the tested compounds is warranted.
Due to a high prevalence of otitis media (OM) and the continuous emergence of drug-resistant OM-causing pathogens, new therapeutic options are required.
Ceragenins exert potent bactericidal activity against bacterial pathogens causing ear infections.
Immobilization of ceragenins on the surface of nonspherical gold nanoparticles intensifies their antibacterial effects, against both planktonic and biofilm form of bacteria.
Activity of both ceragenins and cationic steroid antimicrobial (CSA)-based nanosystems is enhanced in the presence of human cerumen.
Nonspherical, ceragenin-based nanosystems developed in the course of our research exert potent bactericidal activity at concentrations which are recognized as nontoxic to host cells.
Killing properties of developed nanosystems include the induction of oxidative stress in bacterial cells resulting in permeabilization of bacterial membranes and leakage of intracellular content.
Author contributions
E Piktel and R Bucki designed and supervised the research and preparation of the manuscript. SV Prasad, E Piktel, L Suprewicz, T Daniluk and U Wnorowska conducted the experiments and prepared the figures. J Depciuch, A Maximenko, P Zielinski and M Parlińska-Wojtan carried out the synthesis of the tested nanosystems, performed physicochemical analysis of the tested compounds and analyzed the collected data. J Spałek and S Okła collected the human cerumen from hospital patients. SV Prasad, E Piktel, J Depciuch, A Maximenko, T Daniluk and K Fiedoruk collected and analyzed the data. J Spałek, S Okła, K Fiedoruk and R Bucki made recommendations on the experiments. PB Savage carried out the synthesis of ceragenins. SV Prasad, E Piktel, J Depciuch, A Maximenko, L Suprewicz, U Wnorowska, M Parlińska-Wojtan, PB Savage, K Fiedoruk and R Bucki participated in the writing of the manuscript. All authors reviewed the manuscript. All authors have read and agreed to the published version of the manuscript.
Ethical conduct of research
Cerumen samples were collected from adult healthy volunteers under approval of the Bioethics Committee at the Jan Kochanowski University in Kielce, Faculty of Medicine and Health Sciences (no. 20/2019). Informed written consent has been obtained from the participants involved.
supplementary Text 1
Download PDF (67.7 KB)Supplementary data
To view the supplementary data that accompany this paper please visit the journal website at: www.tandfonline.com/doi/suppl/10.2217/nnm-2021-0370
Financial & competing interests disclosure
This work was financially supported by grants from the National Science Centre, Poland, UMO-2018/31/B/NZ6/02476 (to R Bucki). Part of the study was conducted with the use of equipment purchased by the Medical University of Białystok as part of the RPOWP 2007–2013 funding, Priority I, Axis 1.1, contract no. UDA-RPPD.01.01.00-20-001/15-00 dated 26 June 2015. This research was conducted within the project which has received funding from the European Union’s Horizon 2020 research and innovation programme under the Marie Skłodowska-Curie grant agreement no. 754432 and the Polish Ministry of Science and Higher Education, from financial resources for science in 2018–2023 granted for the implementation of an international co-financed project. The funders had no role in study design, data collection and interpretation, or the decision to submit the work for publication. E Piktel, Depciuch, U Wnorowska, M Parlińska-Wojtan and R Bucki have patents pending for synthesis and biological activity of gold nanoparticles in the shape of nanopeanuts and their utility as drug nanocarriers. The authors have no other relevant affiliations or financial involvement with any organization or entity with a financial interest in or financial conflict with the subject matter or materials discussed in the manuscript apart from those disclosed.
No writing assistance was utilized in the production of this manuscript.
Additional information
Funding
References
- Rettig E , TunkelDE. Contemporary concepts in management of acute otitis media in children. Otolaryngol. Clin. N. Am.47(5), 651–672 (2014).
- Paul CR , MorenoMA. Acute otitis media. JAMA Pediatr.174(3), 308 (2020).
- Pichichero ME . Immunologic dysfunction contributes to the otitis prone condition. J. Infect.80(6), 614–622 (2020).
- Frost HM , BeckerLF, KnepperBCet al. Antibiotic prescribing patterns for acute otitis media for children 2 years and older. J. Pediatr.220, 109–115.e1 (2020).
- Williams CJ , JacobsAM. The impact of otitis media on cognitive and educational outcomes. Med. J. Aust.191(S9), S69–S72 (2009).
- Schilder AG , ChonmaitreeT, CrippsAWet al. Otitis media. Nat. Rev. Dis. Primers.2, 16063 (2016).
- Ngo CC , MassaHM, ThorntonRB, CrippsAW. Predominant bacteria detected from the middle ear fluid of children experiencing otitis media: a systematic review. PLoS ONE11(3), e0150949 (2016).
- Bair KL , CampagnariAA. Moraxella catarrhalis promotes stable polymicrobial biofilms with the major otopathogens. Front. Microbiol.10, 3006 (2019).
- Silva MD , SillankorvaS. Otitis media pathogens – a life entrapped in biofilm communities. Crit. Rev. Microbiol.45(5-6), 595–612 (2019).
- Vermee Q , CohenR, HaysCet al. Biofilm production by Haemophilus influenzae and Streptococcus pneumoniae isolated from the nasopharynx of children with acute otitis media. BMC Infect. Dis.19(1), 44 (2019).
- Obolski U , LourençoJ, ThompsonCet al. Vaccination can drive an increase in frequencies of antibiotic resistance among nonvaccine serotypes of. Proc. Natl. Acad. Sci. USA115(12), 3102–3107 (2018).
- Cleary D , DevineV, MorrisDet al. Pneumococcal vaccine impacts on the population genomics of non-typeable Haemophilus influenzae. Microb. Genom.4(9), (2018).
- Kaur R , PhamM, YuKOA, PichicheroME. Rising pneumococcal antibiotic resistance in the post-13-valent pneumococcal conjugate vaccine era in pediatric isolates from a primary care setting. Clin. Infect. Dis.72(5), 797–805 (2021).
- Marom T , Nokso-KoivistoJ, ChonmaitreeT. Viral-bacterial interactions in acute otitis media. Curr. Allergy Asthma Rep.12(6), 551–558 (2012).
- Qureishi A , LeeY, BelfieldKet al. Update on otitis media – prevention and treatment. Infect. Drug Resist.7, 15–24 (2014).
- Yang R , SabharwalV, OkonkwoOSet al. Treatment of otitis media by transtympanic delivery of antibiotics. Sci. Transl. Med.8(356), 356ra120 (2016).
- Surel U , NiemirowiczK, MarzecMet al. Ceragenins – a new weapon to fight multidrug resistant bacterial infections. Med. Stud.30(3), 207–213 (2014).
- Lai XZ , FengY, PollardJet al. Ceragenins: cholic acid-based mimics of antimicrobial peptides. Acc. Chem. Res.41(10), 1233–1240 (2008).
- Leszczynska K , NamiotD, ByfieldFJet al. Antibacterial activity of the human host defence peptide LL-37 and selected synthetic cationic lipids against bacteria associated with oral and upper respiratory tract infections. J. Antimicrob. Chemother.68(3), 610–618 (2013).
- Moscoso M , Esteban-TorresM, MenendezM, GarciaE. In vitro bactericidal and bacteriolytic activity of ceragenin CSA-13 against planktonic cultures and biofilms of Streptococcus pneumoniae and other pathogenic streptococci. PLoS ONE9(7), e101037 (2014).
- Hacioglu M , HaciosmanogluE, Birteksoz-TanASet al. Effects of ceragenins and conventional antimicrobials on Candida albicans and Staphylococcus aureus mono and multispecies biofilms. Diagn. Microbiol. Infect. Dis.95(3), 114863 (2019).
- Howell MD , StreibJE, KimBEet al. Ceragenins: a class of antiviral compounds to treat orthopox infections. J. Invest. Dermatol.129(11), 2668–2675 (2009).
- Piktel E , PogodaK, RomanMet al. Sporicidal activity of ceragenin CSA-13 against Bacillus subtilis. Sci. Rep.7, 44452 (2017).
- Durnas B , WnorowskaU, PogodaKet al. Candidacidal activity of selected ceragenins and human cathelicidin LL-37 in experimental settings mimicking infection sites. PLoS ONE11(6), e0157242 (2016).
- Bucki R , NamiotDB, NamiotZet al. Salivary mucins inhibit antibacterial activity of the cathelicidin-derived LL-37 peptide but not the cationic steroid CSA-13. J. Antimicrob. Chemother.62(2), 329–335 (2008).
- Bucki R , SostareczAG, ByfieldFJet al. Resistance of the antibacterial agent ceragenin CSA-13 to inactivation by DNA or F-actin and its activity in cystic fibrosis sputum. J. Antimicrob. Chemother.60(3), 535–545 (2007).
- Hashemi MM , RovigJ, WeberSet al. Susceptibility of colistin-resistant, gram-negative bacteria to antimicrobial peptides and ceragenins. Antimicrob. Agents Chemother.61(8), e00292 (2017).
- Pollard JE , SnarrJ, ChaudharyVet al. In vitro evaluation of the potential for resistance development to ceragenin CSA-13. J. Antimicrob. Chemother.67(11), 2665–2672 (2012).
- Wnorowska U , FiedorukK, PiktelEet al. Nanoantibiotics containing membrane-active human cathelicidin LL-37 or synthetic ceragenins attached to the surface of magnetic nanoparticles as novel and innovative therapeutic tools: current status and potential future applications. J. Nanobiotechnol.18(1), 3 (2020).
- Alkilany AM , MurphyCJ. Toxicity and cellular uptake of gold nanoparticles: what we have learned so far?J. Nanopart. Res.12(7), 2313–2333 (2010).
- Shamaila S , ZafarN, RiazSet al. Gold nanoparticles: an efficient antimicrobial agent against enteric bacterial human pathogen. Nanomaterials (Basel)6(4), 71 (2016).
- Mohamed MM , FouadSA, ElshokyHAet al. Antibacterial effect of gold nanoparticles against Corynebacterium pseudotuberculosis. Int. J. Vet. Sci. Med.5(1), 23–29 (2017).
- Khan MA , KhanMJ. Nano-gold displayed anti-inflammatory property via NF-κB pathways by suppressing COX-2 activity. Artif. Cells Nanomed. Biotechnol.46(Suppl 1.), 1149–1158 (2018).
- Lee B , LeeDG. Synergistic antibacterial activity of gold nanoparticles caused by apoptosis-like death. J. Appl. Microbiol.127(3), 701–712 (2019).
- Rad MR , KazemianH, YazdaniFet al. Antibacterial activity of gold nanoparticles conjugated by aminoglycosides against A. baumannii isolates from burn patients. Recent Pat. Antiinfect. Drug Discov.13(3), 256–264 (2018).
- Kalita S , KandimallaR, SharmaKKet al. Amoxicillin functionalized gold nanoparticles reverts MRSA resistance. Mater. Sci. Eng. C Mater. Biol. Appl.61, 720–727 (2016).
- Díez-Martínez R , García-FernándezE, ManzanoMet al. Auranofin-loaded nanoparticles as a new therapeutic tool to fight streptococcal infections. Sci. Rep.6, 19525 (2016).
- Slomberg DL , LuY, BroadnaxADet al. Role of size and shape on biofilm eradication for nitric oxide-releasing silica nanoparticles. ACS Appl. Mater. Interfaces5(19), 9322–9329 (2013).
- Piktel E , OściłowskaI, SuprewiczŁet al. ROS-mediated apoptosis and autophagy in ovarian cancer cells treated with peanut-shaped gold nanoparticles. Int. J. Nanomedicine16, 1993–2011 (2021).
- Penders J , StolzoffM, HickeyDJet al. Shape-dependent antibacterial effects of non-cytotoxic gold nanoparticles. Int. J. Nanomedicine12, 2457–2468 (2017).
- Piktel E , SuprewiczŁ, DepciuchJet al. Varied-shaped gold nanoparticles with nanogram killing efficiency as potential antimicrobial surface coatings for the medical devices. Sci. Rep.11(1), 12546 (2021).
- Piktel E , SuprewiczŁ, DepciuchJet al. Rod-shaped gold nanoparticles exert potent candidacidal activity and decrease the adhesion of fungal cells. Nanomedicine (Lond.)15(28), 2733–2752 (2020).
- Chmielewska SJ , SkłodowskiK, DepciuchJet al. Bactericidal properties of rod-, peanut-, and star-shaped gold nanoparticles coated with ceragenin CSA-131 against multidrug-resistant bacterial strains. Pharmaceutics13(3), 425 (2021).
- Ding B , GuanQ, WalshJPet al. Correlation of the antibacterial activities of cationic peptide antibiotics and cationic steroid antibiotics. J. Med. Chem.45(3), 663–669 (2002).
- Rodriguez-Carvajal J . FULLPROF: a program for rietveld refinement and pattern matching analysis. In: Abstracts of the Satellite Meeting on Powder Diffraction of the XV Congress of the IUCr.Toulouse, France, 127 (1990).
- Jorgensen JH , MaherLA, HowellAW. Use of Haemophilus test medium for broth microdilution antimicrobial susceptibility testing of Streptococcus pneumoniae. J. Clin. Microbiol.28(3), 430–434 (1990).
- Durnas B , PiktelE, WatekMet al. Anaerobic bacteria growth in the presence of cathelicidin LL-37 and selected ceragenins delivered as magnetic nanoparticles cargo. BMC Microbiol.17(1), 167 (2017).
- Chai TJ , ChaiTC. Bactericidal activity of cerumen. Antimicrob. Agents Chemother.18(4), 638–641 (1980).
- Wnorowska U , PiktelE, DurnaśBet al. Use of ceragenins as a potential treatment for urinary tract infections. BMC Infect Dis.19(1), 369 (2019).
- Piktel E , WnorowskaU, CieślukMet al. Inhibition of inflammatory response in human keratinocytes by magnetic nanoparticles functionalized with PBP10 peptide derived from the PIP2-binding site of human plasma gelsolin. J. Nanobiotechnol.17(1), 22 (2019).
- Suh I , OhtaH, WasedaY. High-temperature thermal expansion of six metallic elements measured by dilatation method and x-ray diffraction. J. Mater. Sci.23, 757–760 (1988).
- Sneba K , SathishkumarM, SokK, YeoungSangY. Counter ions and temperature incorporated tailoring of biogenic gold nanoparticles. Process Biochem.45(9), 1450–1458 (2010).
- Levin CS , JaneskoBG, BardhanRet al. Chain-length-dependent vibrational resonances in alkanethiol self-assembled monolayers observed on plasmonic nanoparticle substrates. Nano Lett.6(11), 2617–2621 (2006).
- Bazylewski P , DivigalpitiyabR, FanchiniacG. In situ Raman spectroscopy distinguishes between reversible and irreversible thiol modifications in l-cysteine. RCS Adv.7, 2964–2970 (2017).
- Deręgowska A , DepciuchJ, WojnarowskaRet al. Study of optical properties of a glutathione capped gold nanoparticles using linker (MHDA) by Fourier transform infra red spectroscopy and surface enhanced Raman scattering. Int. J. Biol. Biomol. Agricult. Food Biotechnol. Eng.7(1), 80–83 (2013).
- Charlé K , FrankF, SchulzeW. The optical properties of silver microcrystallites in dependence on size and the influence of the matrix environment. Berichte Bunsengesellschaft Phys. Chem.88(4), 350–354 (1984).
- Chandekar A , SenguptaSK, WhittenJE. Thermal stability of thiol and silane monolayers: A comparative study. Appl. Surf. Sci.256(9), 2742–2749 (2010).
- Frost HM , GerberJS, HershAL. Antibiotic recommendations for acute otitis media and acute bacterial sinusitis. Pediatr. Infect. Dis. J.38(2), 217 (2019).
- Gerber JS , RossRK, BryanMet al. Association of broad- vs narrow-spectrum antibiotics with treatment failure, adverse events, and quality of life in children with acute respiratory tract infections. JAMA318(23), 2325–2336 (2017).
- Leso V , FontanaL, ErcolanoML, RomanoR, IavicoliI. Opportunities and challenging issues of nanomaterials in otological fields: an occupational health perspective. Nanomedicine(Lond.)14(19), 2613–2629 (2019).
- Ziąbka M , DziadekM, MenaszekEet al. Middle ear prosthesis with bactericidal efficacy-in vitro investigation. Molecules22(10), 1681 (2017).
- Yu Q , LiJ, ZhangY, WangYet al. Inhibition of gold nanoparticles (AuNPs) on pathogenic biofilm formation and invasion to host cells. Sci. Rep.6, 26667 (2016).
- Kayyali MN , BrakeL, RamseyAJet al. A novel nano-approach for targeted inner ear imaging. J. Nanomed. Nanotechnol.8(4), 2017).
- Ahangari A , SaloutiM, HeidariZet al. Development of gentamicin-gold nanospheres for antimicrobial drug delivery to Staphylococcal infected foci. Drug Deliv.20(1), 34–39 (2013).
- Rai A , PrabhunebA, PerryC. Antibiotic mediated synthesis of gold nanoparticles with potent antimicrobial activity and their application in antimicrobial coatings. J. Mater. Chem.20, 6789–6798 (2010).
- Brown AN , SmithK, SamuelsTAet al. Nanoparticles functionalized with ampicillin destroy multiple-antibiotic-resistant isolates of Pseudomonas aeruginosa and Enterobacter aerogenes and methicillin-resistant Staphylococcus aureus. Appl. Environ. Microbiol.78(8), 2768–2774 (2012).
- Niemirowicz K , DurnaśB, TokajukGet al. Formulation and candidacidal activity of magnetic nanoparticles coated with cathelicidin LL-37 and ceragenin CSA-13. Sci. Rep.7(1), 4610 (2017).
- Piktel E , ProkopI, WnorowskaUet al. Ceragenin CSA-13 as free molecules and attached to magnetic nanoparticle surfaces induce caspase-dependent apoptosis in human breast cancer cells via disruption of cell oxidative balance. Oncotarget9(31), 21904–21920 (2018).
- Chatterjee T , ChatterjeeBK, ChakrabartiP. Modelling of growth kinetics of Vibrio cholerae in presence of gold nanoparticles: effect of size and morphology. Sci. Rep.7(1), 9671 (2017).
- Perez AC , PangB, KingLBet al. Residence of Streptococcus pneumoniae and Moraxella catarrhalis within polymicrobial biofilm promotes antibiotic resistance and bacterial persistence in vivo. Pathog. Dis.70(3), 280–288 (2014).
- Epand RF , PollardJE, WrightJOet al. Depolarization, bacterial membrane composition, and the antimicrobial action of ceragenins. Antimicrob. Agents Chemother.54(9), 3708–3713 (2010).
- Slavin YN , AsnisJ, HafeliUO, BachH. Metal nanoparticles: understanding the mechanisms behind antibacterial activity. J. Nanobiotechnol.15(1), 65 (2017).
- Lee H , LeeDG. Gold nanoparticles induce a reactive oxygen species-independent apoptotic pathway in Escherichia coli. Colloids Surf. B Biointerfaces167, 1–7 (2018).
- Rowe-Magnus DA , KaoAY, PrietoACet al. Cathelicidin peptides restrict bacterial growth via membrane perturbation and induction of reactive oxygen species. mBio10(5), e02021 (2019).
- Eruslanov E , KusmartsevS. Identification of ROS using oxidized DCFDA and flow-cytometry. Methods Mol. Biol.594, 57–72 (2010).
- Dwyer DJ , BelenkyPA, YangJHet al. Antibiotics induce redox-related physiological alterations as part of their lethality. Proc. Natl. Acad. Sci. USA111(20), E2100–E2109 (2014).
- Zhao X , DrlicaK. Reactive oxygen species and the bacterial response to lethal stress. Curr. Opin. Microbiol.21, 1–6 (2014).
- Ortiz-Benitez EA , Carrillo-MoralesM, Velázquez-GuadarramaNet al. Inclusion bodies and pH lowering: as an effect of gold nanoparticles in Streptococcus pneumoniae. Metallomics7(7), 1173–1179 (2015).
- Ortiz-Benítez EA , Velázquez-GuadarramaN, DuránFigueroa NVet al. Antibacterial mechanism of gold nanoparticles on Streptococcus pneumoniae. Metallomics11(7), 1265–1276 (2019).
- Zheng K , SetyawatiMI, LeongDT, XieJ. Antimicrobial gold nanoclusters. ACS Nano11(7), 6904–6910 (2017).
- Pericone CD , OverwegK, HermansPW, WeiserJN. Inhibitory and bactericidal effects of hydrogen peroxide production by Streptococcus pneumoniae on other inhabitants of the upper respiratory tract. Infect. Immun.68(7), 3990–3997 (2000).
- Hoopman TC , LiuW, JoslinSNet al. Identification of gene products involved in the oxidative stress response of Moraxella catarrhalis. Infect. Immun.79(2), 745–755 (2011).
- Borrill ZL , RoyK, SinghD. Exhaled breath condensate biomarkers in COPD. Eur. Respir. J.32(2), 472–486 (2008).
- Kalyanaraman B , Darley-UsmarV, DaviesKJet al. Measuring reactive oxygen and nitrogen species with fluorescent probes: challenges and limitations. Free Radic. Biol. Med.52(1), 1–6 (2012).
- Niemirowicz K , SurelU, WilczewskaAZet al. Bactericidal activity and biocompatibility of ceragenin-coated magnetic nanoparticles. J. Nanobiotechnology13(1), 32 (2015).
- Durnaś B , WnorowskaU, PogodaKet al. Candidacidal activity of selected ceragenins and human cathelicidin LL-37 in experimental settings mimicking infection sites. PLoS ONE11(6), e0157242 (2016).
- Schwaab M , GurrA, NeumannAet al. Human antimicrobial proteins in ear wax. Eur. J. Clin. Microbiol. Infect. Dis.30(8), 997–1004 (2011).
- Leszczyńska K , NamiotA, CruzKet al. Potential of ceragenin CSA-13 and its mixture with pluronic F-127 as treatment of topical bacterial infections. J. Appl. Microbiol.110(1), 229–238 (2011).
- Kim JK , ChoJH. Change of external auditory canal pH in acute otitis externa. Ann. Otol. Rhinol. Laryngol.118(11), 769–772 (2009).
- Sulaiman GM , WaheebHM, JabirMSet al. Hesperidin loaded on gold nanoparticles as a drug delivery system for a successful biocompatible, anti-cancer, anti-inflammatory and phagocytosis inducer model. Sci. Rep.10(1), 9362 (2020).
- Farooq MU , NovosadV, RozhkovaEAet al. Gold nanoparticles-enabled efficient dual delivery of anticancer therapeutics to HeLa cells. Sci. Rep.8(1), 2907 (2018).
- Cheheltani R , EzzibdehRM, ChhourPet al. Tunable, biodegradable gold nanoparticles as contrast agents for computed tomography and photoacoustic imaging. Biomaterials102, 87–97 (2016).
- Raynov AM , ChoungYH, ParkHYet al. Establishment and characterization of an In vitro model for cholesteatoma. Clin. Exp. Otorhinolaryngol.1(2), 86–91 (2008).
- von Witzleben M , StoppeT, AhlfeldTet al. Biomimetic tympanic membrane replacement made by melt electrowriting. Adv. Healthc. Mater.10(10), e2002089 (2021).
- Ranakusuma RW , McCulloughAR, SafitriEDet al. Oral prednisolone for acute otitis media in children: a pilot, pragmatic, randomised, open-label, controlled study (OPAL study). Pilot Feasibility Stud.4, 146 (2020).