Abstract
Hearing loss is currently one of the most prevalent sensory disorders worldwide. Because both the blood–labyrinth barrier and the limited blood circulation in the inner ear restrain the effective delivery of most drugs to the inner ear tissues, current treatments for hearing loss are limited to mainly medication, hearing devices and cochlear surgery for therapeutic purposes, whereas treatments lack a noninvasive targeted drug-delivery system. With the continuously rapid development of new nanomaterials, the nanodelivery systems are expected to provide a potentially effective method of clinical treatment for hearing loss. This paper reviews the advantages and disadvantages of the commonly used drug-delivery methods and novel nanomaterials for inner ears as well as advancements in the targeted treatment of hearing loss.
In the past few years, hearing loss has been on the rise globally, especially in older populations and those who are exposed to excessive noise at work or during leisure. According to the latest statistical data from WHO, more than 466 million people (i.e., over 5% of the world population) suffer from severe disabling hearing loss, while the vast majority of these patients live in the middle-income countries. Nearly 50% of people aged from 12 to 35 years old (accounting for ∼1.1 billion young people) are at risk of hearing loss due to long-term overexposure to loud sounds. There are predictions that by the year of 2050, more than 900 million people will suffer from hearing loss [Citation1]. Sudden deafness, sensorineural deafness, Ménière’s disease and autoimmune inner ear diseases (AIEDs) are all common causes of hearing loss. The blood–labyrinth barrier and the complex physiology of the inner ear prevent ordinary therapeutic drugs from reaching it. With the rapid development of new nanobiomaterials and the increased awareness of inner ear diseases, the treatments for inner ear diseases have been significantly improved. The nanoparticle (NP)-based drug-delivery systems would potentially provide a secure and effective approach to the treatment of inner ear diseases due to their advantages: 1) strong specificity and efficient drug targeting, 2) the ability to avoid the blood–labyrinth barrier and to enter the inner ear directly, 3) high drug concentration in the internal and external lymphatic fluids and 4) no side effects of systemic drug delivery. In this study, we review the recent advancements in the drug-delivery systems and novel nanomaterials for the inner ears to provide clinical guidance in the treatment of patients with hearing loss.
Anatomical & physiological characteristics of inner ear
An individual’s ear consists of three parts: the outer, middle and inner ear (). With a complex and sophisticated structure, the inner ear is located in the temporal bone and is composed of a bony vagus and a membranous vagus, which are both filled with lymphatic fluid. The endolymphatic fluid is located within the membranous vagus and is generated by the vascular striae, with no communication between the inner and outer lymphatic fluids. Although the inner and outer lymphs are structurally separated by a barrier, any tissues in the body are connected, with some substances entering the outer (or inner) lymph from the inner (or outer) lymph; in particular, the drug penetrates into the outer lymph via the round window membrane (RWM) and then diffuses into the inner lymph. The labyrinth is divided into three parts, which are spatially arranged from anterior to posterior, including the vestibule, the semicircular canal and the cochlea. The cochlea has the functions of sound transmission, sound perception and balance, while the vestibule and the semicircular canal are inner ear receptors to help maintain both posture and balance [Citation2]. The cochlear spiral apparatus, also known as the Corti’s organ, is an inner ear auditory receptor device located above the basilar membrane and composed of hair cells (i.e., the auditory receptor cells), supporting cells, reticular membrane and capsule (). Because of the blood–labyrinth barrier in the inner ear, conventional drug-delivery methods do not allow the optimal drug concentration in the inner ear, thus greatly reducing the therapeutic effect of the drug [Citation3]. Therefore, the precise delivery of therapeutic drugs to the inner ear in order to achieve the optimal therapeutic effect is now the key to the successful treatment of inner ear diseases. Globally, scientists are increasingly focusing on improving the methods of delivering drugs directly to the inner ear.
The ear is divided into outer ear, middle ear and inner ear. The outer ear consists of the outer auricle and the outer auditory canal. The tympanic membrane is located between the outer ear and the middle ear, containing the tympanum and the auditory ossicle, with the latter composed of three bones, that is, the malleus, the incus and the stapes. The inner ear is mainly composed of labyrinths, including membranous labyrinths and bony labyrinths, which are both filled with lymphatic fluid and are divided into the vestibule, semicircular canal, cochlea and inner ear canal.
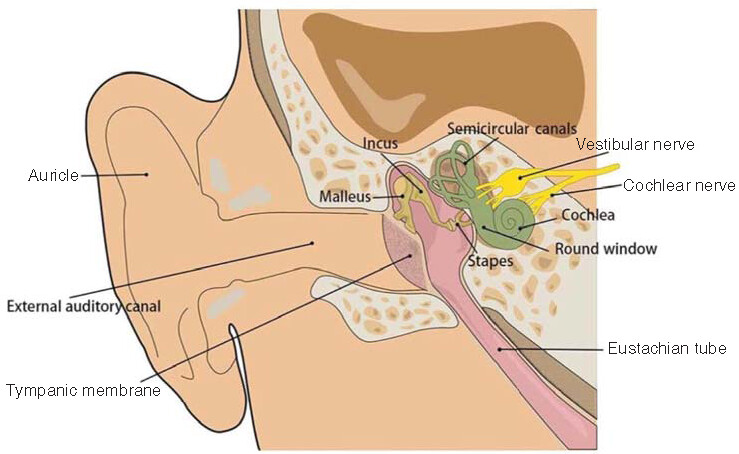
Commonly used methods of inner ear drug delivery
Hearing and balance disorders are generally treated by drugs delivered to the cochlea and vestibule of the inner ear. Although the structure of the inner ear is well understood, the successful delivery of therapeutic drugs to inner ear tissue is currently still challenging. On the one hand, the challenges are derived from the anatomy of the inner ear, that is, firstly, the existence of the blood–labyrinth barrier between the inner ear and the systemic circulation, and secondly, the fact that the arteries of the inner ear are extremely thin terminal arteries with slow blood flow and poor collateral circulation. On the other hand, the pharmacological challenges include the following: 1) the poor permeability of the drug that limits its transport through the RWM into the inner ear, 2) the instability of the drugs, 3) the inability of the drug to effectively aggregate in the internal and external lymphatic fluids as it exits the body via the eustachian tube and 4) the nontargeted drug delivery. As a result, increasing attention is being paid to the research and development of more efficient methods of delivering drugs to the inner ear. Currently, the delivery methods of therapeutic drugs into the inner ear in human subjects is occurring in clinical practice ( & ).
Table 1. Comparison of four different inner ear drug-delivery methods.
Current common methods of inner ear drug delivery include middle ear tympanic injection, round window membrane injection and semicircular canal injection.
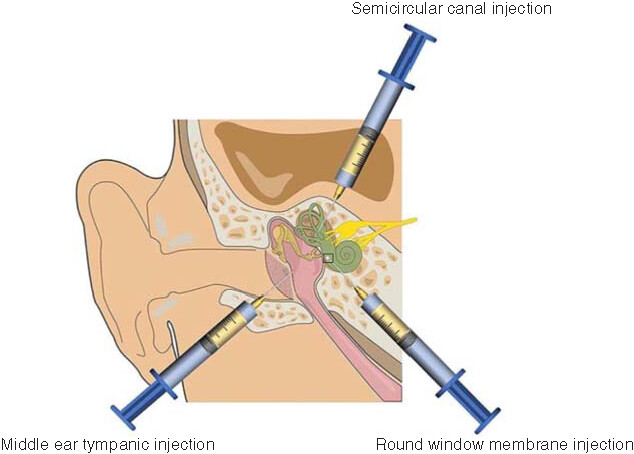
Systemic drug delivery
Systemic administration is generally achieved by oral, intravenous or drip, or intramuscular routes, and has been used to effectively treat inner ear diseases such as AIED, Ménière’s disease and sudden hearing loss. AIED presents with the bilateral asymmetry of a progressive nature of the sensorineural hearing loss and may be associated with vestibular dysfunction [Citation4–6], generally treated with long-term oral corticosteroids. The current treatment regimen for AIED is a high dose of oral prednisone for 4 weeks, followed by a gradual reduction to the lowest possible dose needed to treat the hearing symptoms while maintaining the therapeutic effect. The hearing retention rates have been reported to be between 53% and 70% after using this treatment modality [Citation5]. In 1948, it was discovered that otogenic vertigo could be effectively controlled by intravenous streptomycin, and thereafter, streptomycin has become one of the first drugs to be administered systemically for the treatment of inner-ear-related diseases [Citation7]. Later, streptomycin administered by intramuscular injection also showed evident therapeutic effects on the treatment of Ménière’s disease [Citation8,Citation9]. A study by Wilson et al. demonstrated that sudden sensorineural hearing loss (SSNHL) could be effectively reversed by oral steroids, with an overall cure rate of over 50% [Citation10]. Although the systemic drug delivery is widely used in clinical practice as a noninvasive mode of administration, the blood–labyrinth barrier and low blood supply to the inner ear make it difficult to achieve effective drug concentrations in the inner ear. Therefore, the desired therapeutic effect can only be achieved by increasing the drug concentration in the body circulation, but this risks generating systemic toxic effects. The inadequacy of systemic drug-delivery methods has promoted the development of new drug-delivery methods.
Middle ear intratympanic administration
Intratympanic drug delivery is performed by placing the drug in the middle ear tympanic chamber to pass through the RWM into the extra tympanic lymphatic fluid of the inner ear and subsequently to diffuse into the entire inner ear. Due to its bypass of the vagus artery and the blood–internal-ear barrier, intratympanic administration is a more direct and effective route in comparison to systemic administration and was first successfully attempted by Schuknecht for the treatment of Ménière’s disease with streptomycin applied in the middle ear tympanic cavity [Citation11]. It has been demonstrated that intradrum administration not only increases the drug concentration in the internal and external lymphatic fluids compared with either intravenous or oral administrations, but also avoids the toxic side effects associated with systemic administration. Rauch et al. conducted a randomized prospective trial on the efficacy of oral prednisolone and intradrum injection of methylprednisolone in patients with sudden deafness to reveal that either oral or intradrum injections of corticosteroids given at the beginning of treatment was effective for hearing protection without statistically significant difference, whereas patients who received oral administrations of corticosteroids showed glycemic changes and gastrointestinal reactions [Citation12]. Wang et al. investigated the relationship between SSNHL after intradrum injection of steroids and the degree of hearing recovery [Citation13]. This retrospective investigation identified a positive correlation between the number of intradrum steroid injections and the recovery rate in SSNHL patients. Although the high efficiency of intratympanic delivery reduces the systemic toxicities, the effective concentration of drug accumulated in the inner ear depends on the dose of drug that crosses the RWM of the middle ear, while the differences in RWM permeability may lead to variations in drug retention and elimination rates [Citation14], ultimately making it difficult to standardize the dosage regimens.
Direct inner ear drug delivery
Middle ear intratympanic administration requires the drugs to pass through the RWM barrier, which is not only the necessary route for substances to enter the inner ear from the middle ear, but also a biofilm barrier that prevents substances from entering the inner ear, making the direct inner ear delivery method more effective than middle ear intratympanic administration. Typically, the topical delivery of a drug maximizes its concentration in the inner ear while reducing its side effects. Direct drug delivery includes cochlear or vestibular drug delivery (semicircular canal injection) [Citation20–22]. To date, the trans-RWM injection administration has become the main method of inner ear drug delivery [Citation15–17,Citation20]. Therapeutic drugs encapsulated by nanomaterials have improved solubility, stability and the ability to control drug release, thereby successfully delivering the drugs to the inner ear through RWM [Citation18,Citation19]. However, the inner ear drug delivery is a highly invasive delivery method completed by a surgery, dramatically increasing the risk of cochlear-related diseases.
NP delivery system
NPs are formed by the preparation of different biomaterials, usually ranging from tens to hundreds of nanometers in diameter, capable of encapsulating various types of therapeutic drugs. In comparison to the other types of drug-delivery materials, NPs have shown advantages such as high biocompatibility, increased drug stability and high controllability of drug release and targeting through surface modification in the treatment of inner ear diseases. Based on these advantages, the NP delivery systems have shown significant potential in the treatment of hearing loss. Many nanosystems for inner ear drug delivery have been developed, including polymers, lipid NPs, superparamagnetic iron oxide NPs, silica NPs and nanogels.
Polymeric NP drug-delivery system
The poly(lactic-co-glycolic acid) (PLGA) is a negatively charged, biodegradable polymer formed by the random polymerization of two monomers, that is, lactic acid and hydroxyacetic acid, with enhanced biocompatibility and hydrophilicity and controlled degradation. The polymer assists in the timely and quantitative delivery of drugs and other bioactive molecules to the target area. The PLGA NPs are targeted to the inner ear scala tympani after the trans-RWM injection administration compared with the systemic administration. The successful topical application of this NP to the RWM demonstrates an effective targeting and sustained delivery strategy for inner ear treatment. Zhang et al. reported for the first time the diffusion route of PLGA NPs after the intratympanic injections, providing guidance for the appropriate preparation of inner ear NP carriers with increased therapeutic effects [Citation23]. Dai et al. developed a novel drug-delivery system with the PLGA NPs encapsulating IFN α-2 b before loading into the thermosensitive hydrogel chitosan (CS)/glycerol phosphate, allowing longer exposure and residence time of the drug in guinea pig cochlea without causing the loss of inner ear cells [Citation24]. This biodegradable and thermosensitive hydrogel system could successfully deliver drugs to the inner ear and may become a novel vehicle for the treatment of inner ear diseases. Kuang et al. designed a new mitochondria-targeted drug-delivery system by successfully constructing the PLGA NPs loaded with SS-31 peptide-conjugated geranyl geranyl acetone based on the emulsion-solvent evaporation method [Citation25]. The experimental results showed that the system could protect zebrafish hair cells from ototoxic drugs (i.e., gentamicin) with potential clinical applications. In order to increase the circulation time of NPs in the body and delay the elimination of NPs by the mononuclear phagocytic system, the surface modification of NPs is performed by using the surfactants, that is, the hydrophilic biomaterials attached to the NP surface for prolongation, thus promoting the targeted delivery of NPs as well as preventing the rapid elimination of NPs. Wen et al. performed the surface modification of PLGA NPs with poloxamer 407 (P407), CS and methoxypolyethylene glycol, respectively, and compared the properties of PLGA NPs before and after the surface modification [Citation26]. The results showed that the PLGA NPs in hydrophilic molecules, especially those modified with P407, could significantly improve the efficiency of NP delivery to the outer hair cells (OHCs) in the cochlea. In addition to the PLGA NPs in polymeric NP drug-delivery systems, other types of polymeric NPs, including polylactic acid, polycaprolactone and PEG, are also used for the treatment of inner ear diseases by encapsulating drugs through electrostatic interactions or hydrophobic—hydrophobic interactions [Citation27–29]. Zhao et al. successfully synthesized reactive oxygen-responsive NPs (PL-PPS/BBR) and modified the NPs with prestin-targeting peptide 2 to target OHCs for the treatment of noise-induced hearing loss (NIHL) [Citation30]. The NPs were internally wrapped with flavopiridol (BBR) and polypropyl sulfide 120 to provide the synthesized NPs with increased anti-inflammatory and antioxidant effects. The results of auditory brainstem response revealed a significant improvement by the PL-PPS/BBR on the hearing of guinea pigs after noise exposure, suggesting that the application of PL-PPS/BBR has the potential to become a new therapeutic treatment for noise-related injury.
Liposomal NP-based drug-delivery system
Lipid NPs
Lipid NPs are biodegradable and can deliver both hydrophilic and lipophilic drugs, depending on their molecular structures and compositions [Citation31,Citation32]. Solid lipid NPs (SLNs) are a type of novel submicron-scale lipid particle [Citation33] and have been tested as novel intelligent drug-delivery systems in various cell types to treat different diseases [Citation34–36]. SLNs can be loaded with various types of drugs such as Adriamycin, paclitaxel or trans-retinoic acid used in the treatment of cancer [Citation37–39]. As a potential type of drug-delivery system, SLNs have shown several advantages, including low cost, easy scale-up of SLNs production and proven production method, that is, microemulsions, without the use of organic solvents [Citation40]. These advantageous features demonstrate a promising potential for SLNs to be used in clinical treatments. Cervantes et al. reported that SLNs based on stearic acid have appropriate particle size, polydispersity index and ζ-potential, and are considered as one of the best nanocarriers for drug delivery [Citation40]. To test the drug delivery efficiency of SLNs, they loaded either dexamethasone or hydrocortisone alone into SLNs to perform the protection tests against cisplatin ototoxicity in vitro. The experimental results showed that both medicines wrapped in SLNs significantly increased the drug protection and prolonged the survival time of HEI-OC1 cells compared with the free mode of administration. Gao et al. investigated the otoprotective effect of edaravone on noise-exposed guinea pigs using SLNs encapsulated with a slow-release dosage form of edaravone [Citation41]. These results evidently demonstrated that the edaravone-SLNs exhibited a significant slow-release effect and enhanced protection against NIHL. Gu et al. established a lipid–polymer NP (LPN) to load astaxanthin (ATX), which has a unique antioxidant capacity and is a candidate for new therapies against oxidative-stress-related diseases [Citation19]. LPN assists ATX in penetrating the RWM and maintaining its effective drug concentration in the outer lymphatic fluid of the inner ear for 24 h after injection. The authors further analyzed the protective effect of ATX against cisplatin ototoxicity in HEI-OC1 cells, zebrafish and guinea pigs. The experimental results demonstrated that ATX–LPN could effectively scavenge cisplatin-induced reactive oxygen species (ROS) production, reduce the expression of proapoptotic proteins and enhance the antiapoptotic expression, ultimately attenuating the hair cell loss. These results suggested that ATX–LPN may be a potential drug to alleviate cisplatin-induced hearing loss.
Liposomes
Liposomes are miniature vesicles formed by encapsulating drugs in lipid-like bilayers, which can fuse with cell membranes and enter the inner ear cells to release drugs and then degrade automatically, with the characteristics of nontoxicity, high biocompatibility, and improved stability and utilization of drugs [Citation42]. The encapsulation of liposomes allows for the sustained release and prolonged circulation time of the drug in the body, resulting in a significantly increased drug efficacy. Studies have shown that modifications of targeting ligands reduce the off-targeting of lipid NPs and enhance the cellular uptake, while recent advancements in targeting ligand design have yielded clinically promising and efficient drug-delivery systems with targeting ligands, including peptides [Citation43,Citation44], antibodies [Citation45,Citation46], oligonucleotide aptamers [Citation47,Citation48] and folic acid [Citation49]. It has been demonstrated that liposomes can carry drugs to transport across the RWM, ultimately delivering therapeutic drugs into the inner ear cells [Citation50,Citation51]. Hou et al. developed a liposome nanodelivery system for loading the water-soluble drug minocycline, which has been approved for clinical application. Meanwhile, they used the mitochondrial targeted peptide SS-31 to modify the surface of NPs [Citation51]. The results showed that liposome NPs modified by SS-31 significantly improved the survival rate of zebrafish hair cells after long-term exposure to gentamicin and reduced the hearing loss caused by ototoxic drugs. The liposome drug-delivery system has also the characteristics of small particle size, high drug encapsulation rate and slow drug release. Therefore, the liposome drug-delivery system shows a high potential in clinical application in the treatment of inner ear diseases. Multivesicular vesicle liposomes (MVLs) have a larger particle size (1–100 μm) with strongly sustained release properties and drug release compared with conventional liposomes [Citation52]. MVLs were first prepared in the early 1980s and are now widely used in antitumor drugs, antibiotics and other related fields [Citation53–55]. However, MVLs are rarely used for topical administration in the ear. Li et al. established a dexamethasone sodium phosphate multicapsular liposomal thermosensitive hydrogel (DEX-MVLs-Gel) delivery system to detect hearing, drug concentration in peripheral lymph fluid and hair cell morphology in guinea pigs by intra-drum injection [Citation56]. The pharmacokinetics and biosafety of this drug-delivery system in the inner ear were further analyzed and its protective effect on the hearing of guinea pigs after noise exposure was also investigated. The results revealed a significant protective effect of DEX-MVLs-Gel with a high concentration (20 mg/ml) on NIHL in guinea pigs, and the prepared DEX-MVLs-Gel could be effectively preserved in the peripheral lymphatic fluid of guinea pigs for 3–7 days, while the MVLs-Gel showed no significant ototoxicity. These results indicated that DEX-MVLs-Gel could be used as a novel drug-delivery system with high stability and safety, and evident protective and therapeutic effects on NIHL, providing a potential treatment for inner ear diseases.
Inorganic NP drug-delivery system
Magnetic NPs
With the advantages of low toxicity, high biocompatibility and convenient manipulation by applying magnetic fields, the magnetic NPs have shown significant promise for medical applications in recent years. The representative superparamagnetic iron oxide NPs (SPION) are a type of emerging functional material with a diameter of less than 100 nm and superparamagnetic properties. SPION has shown advantages in the following aspects:
Magnetic responsiveness. When SPION is in suspension, the NPs are magnetized by the external magnetic field and can be separated from the medium, while the magnetism disappears when the external magnetic field is removed and the NPs are in suspension again [Citation57].
Superparamagnetism. For certain magnetic particles, when the diameter of the magnetic particle reaches its magnetic single-domain size – that is, the size of the magnetic particle is less than the critical value – then the original domain structure disappears and all the magnetic movements are only aligned in a magnetic state parallel in one direction, allowing the particles to generate larger magnetism in the weaker outer magnetic field, while the particle’s magnetism disappears when the outer magnetic field is removed [Citation58].
Small particle size. The SPION are generally 10–200 nm in diameter, smaller than or similar to cells, viruses or genes, and easy to interact with, showing high biocompatibility [Citation59].
Because of these advantages, SPION have been widely used in biomedical experiments and clinical treatments in recent years. Mondalek et al. designed an in vitro RWM model similar to RWM to demonstrate that SPION could be magnetically transported through the RWM model, allowing the quantitative assessment of the expected targeted drug or gene delivery [Citation60]. Cisplatin is widely used as a first-line chemotherapeutic agent for epithelial tumors such as those of the head and neck, with the main side effect of irreversible and bilateral sensorineural deafness, occurring in 50–70% of patients treated with cisplatin [Citation61,Citation62]. To address this side effect, Kayyali et al. designed a novel nanostructure composed of SPION encapsulated in polymeric micelles formed by amphiphilic block copolymers coupled to diethyl glutathione, which were capable of isolating extracellular cisplatin [Citation63]. The biosafety of glutathione micelles was evaluated at both the cellular and organological levels, indicating that the glutathione micelles cause neither cytotoxicity nor organ damage. Subsequently, the nanostructure was verified in an in vitro model to protect cells from the cytotoxicity of cisplatin, suggesting that this novel nanostructure may have a protective effect against cisplatin-induced ototoxicity.
Silicon dioxide NPs
In comparison with the organic nanocarriers, inorganic nanocarriers have shown unique advantages, including high drug-loading capacity, high yield and low production cost, with silica commonly used as the material of choice in both inorganic nanomaterial and biomedical applications. Mesoporous silica NPs (MSNs) with pore sizes of 2–50 nm are optimal candidates for drug delivery and medical applications compared with other porous silica NPs. MSNs play a critical role in NP drug-delivery systems due to their favorable biocompatibility, large specific surface area and volume, high particle-size adjustability and ease of surface modification. Therefore, MSNs open up new avenues for clinical applications in various biomedical fields such as delivery vehicles for drugs, genes and proteins [Citation64–69], medical imaging [Citation70,Citation71], and cancer treatment [Citation72,Citation73]. Pritz et al. investigated the endocytic transport of silica NPs by HEI-OC1 cells [Citation74] . Specifically, both light and electron microscopies were used to study the interactions between silica NPs of three different sizes (50, 70 and 100 nm) and HEI-OC1 cells. The results showed that NPs of 50 nm had the highest internalization efficiency in HEI-OC1 cells, indicating that smaller silica NPs are effective drug and gene carriers. Furthermore, it was demonstrated that macrocytic drinking action was the key to the internalization of 50 nm silica NPs. It was noted that if silica was transported to the inner ear as a carrier of drugs or genes, then the effect of in vivo kinetics should be further investigated. Gunewardene et al. developed a highly biocompatible inner ear drug-delivery system, that is, the mesoporous silica supraparticulate delivery systems, to evaluate the pharmacokinetics and biodistribution of exogenous neurotrophin-3 delivered by this system in the inner ear [Citation75]. They used the radioactive iodine-125 as a tracer to observe the load, retention and distribution of neurotrophin-3. Due to its high loading capacity and wide distribution in the guinea pig cochlea after administration, this system has become a viable method for effective, long-term delivery of neurotrophins to the cochlea.
Gold NPs
Gold NPs (AuNPs) are simple to prepare with various morphologies and have been widely used in biomedical fields in recent years because of their unique optical properties and high biocompatibility, showing promising applications in bioimaging and tumor therapy [Citation76]. AuNPs have shown significant potential as an inner ear imaging contrast agent due to their characteristics of easy targeting and less cytotoxicity [Citation77]. Lin et al. investigated the efficiency of ultrasound microbubble (USMB)-assisted delivery of CS-coated gold NPs (CS-AuNPs) [Citation78]. They exposed the inner ear of mice to USMBs with an intensity of 2 W/cm2 and then injected the CS-AuNPs or fluorescein isothiocyanate-modified CS-AuNPs into the tympanic chamber. The experimental results showed that USMBs enhanced the permeability of RWM and markedly increased the delivery of fluorescein isothiocyanate-modified CS-AuNPs to the inner ear. Their study suggested that the combination of USMBs and CS-AuNPs could be used as a promising and effective drug- and gene-delivery vehicle for the treatment of inner ear diseases via the RWM pathway.
Nanogel drug-delivery system
As a type of hydrogel-based polymer with a 3D mesh structure, nanogel is considered as a potential drug-delivery carrier due to its unique hydrogel properties and nanoporous structure [Citation79]. Nanogels have the advantages of small particle size, long circulation time, high biocompatibility, biodegradability and drug-loading capacity. The carrier materials commonly used for the preparation of nanogels include polyacrylic acid, polyacrylamide, Pluronic and polysaccharide polymers and their derivatives, formed by amino-based crosslinking, ‘click’ reaction crosslinking, physical crosslinking, photocrosslinking and multiphase monomer polymerization [Citation80]. Nanogels show great promise as an ideal new drug-delivery system for chemotherapy drugs, protein-based drugs and gene delivery. In 2015, Lajud et al. developed a minimally invasive nanohydrogel drug-delivery system [Citation28]. In 2018, Kayyali et al performed further functionalized modifications based on the drug-delivery system developed previously to introduce a targeting peptide to specifically recognize prestin [Citation81]. Injected via the RWM pathway, this drug-delivery system could improve the protection against NIHL through targeted delivery of c-Jun N-terminal kinase inhibitor to OHCs via targeted NPs, suggesting that this novel, safe and controlled targeted nanohydrogel delivery system has shown great potential for the treatment of inner ear diseases. Chen et al. designed a dextran–PEG hydrogel delivery system (DEX-SILK) loaded with dexamethasone to investigate the protective effect of the system in mouse model with cisplatin ototoxicity [Citation82]. Specifically, they explored the otoprotective effect of dexamethasone hydrogel on cisplatin by using in vitro cultured cells of HEI-OC1, Corti’s explant organs and a mouse model of cisplatin-induced deafness. The results showed that DEX-SILK could release dexamethasone slowly in the inner ear for up to 21 days with a sustained release, while the slow release and prolonged retention of DEX-SILK in inner ear cells enhanced the cytoprotective effect on cisplatin-exposed inner ear cells by inhibiting the regeneration of ROS. Compared with the free dexamethasone administration, the DEX-SILK administration via RWM showed significant protection against 4, 8 and 16 kHz cisplatin-induced hearing loss in mice. Chen et al. designed an injectable liposomal hydrogel microsphere nanodelivery system by first encapsulating the liposoluble drug ebselenoside, which was a type of organoselenium compound with both anti-inflammatory and antioxidant effects, with liposomes, and then constructing the homogeneous photocrosslinked gelatin methacrylamide microspheres based on the microfluidic techniques and the surface modification with polydopamine on the surface of the microspheres to increase the adhesion of the hydrogel microspheres to the circular window membrane [Citation18]. The in vitro experiments demonstrated that the microspheres exhibited significant oxidation resistance and adhesion, showing a long residence time on the RWM, while the hydrogel microspheres remained adhered to the RWM after the rotation and inversion treatments. Hydrogel microspheres were demonstrated to have significant protection of hearing in a mouse model of NIHL. Therefore, the mucoadhesive injectable hydrogel microspheres developed in this study have provided a potentially effective treatment of NIHL.
Conclusion
Due to the blood–labyrinth barrier, it is difficult for drugs to reach the inner ear through the blood circulation to achieve the optimal therapeutic effect. In recent years, novel nano drug-delivery systems have become a research hotspot for the treatment of inner ear diseases, achieving significantly improved therapeutic effects in various animal models, and the rapid progress of studies has provided novel insights into the medical treatment of hearing loss. This review describes the anatomical and physiological characteristics of the inner ear, compares common inner ear drug-delivery methods and further discusses recent advancements in various types of nanodelivery systems. Many NP delivery systems, such as polymeric, liposome, inorganic and nanogel delivery systems, have been comprehensively reviewed for targeted drug delivery to the inner ear and for the treatment of hearing loss. However, there are still many shortcomings in the existing inner ear drug-delivery methods: for example, the pharmacokinetic profile is not well defined, while the drug dose in the inner ear is difficult to control precisely and may damage the hearing and balance function of the inner ear at high concentrations. These deficiencies have resulted in NP-based drug release systems that are still in the experimental stage and rarely used in the clinical setting.
Future perspective
In the future, the novel NPs should have improvements in high biosafety, efficient targeting and high stability and universality, to further explore the effective and noninvasive ways of drug delivery to the inner ear in order to achieve the precise and effective treatment of hearing loss. It is reasonably predicted that with a deeper understanding of the physiological structure of the inner ear and the emergence of new biomaterials, the nanomaterials will have a broader application prospect and greater clinical potential in the field of treatment of hearing loss.
Anatomical & physiological characteristics of the inner ear
The special anatomical and physiological characteristics of the inner ear are the key factors limiting the treatment of inner ear diseases. The most important of these is the presence of the blood–labyrinth barrier, which limits the passage of drugs into the inner ear tissues to achieve its effective therapeutic concentrations.
Commonly used methods of inner ear drug delivery
Methods of inner ear administration include systemic administration, “internal administration to the middle ear drum and direct administration to the inner ear. Direct inner ear administration is more effective than the first two methods, maximizing the concentration of the drug in the inner ear while reducing the systemic toxic side effects.
Nanoparticle delivery system
Nanoparticle delivery systems have been proven to show high biocompatibility, stability, controllability and targeting in the treatment of inner ear diseases. Many nanosystems for inner ear drug delivery have been developed, including polymers, lipid nanoparticles, superparamagnetic iron oxide nanoparticles, silica nanoparticles and nanogels. With the continuous improvement of new nanomaterials, these nanomaterials will have a broader application prospect and greater clinical transformation potential in the treatment of hearing loss.
Author contributions
X Bai, L Zhang and L Xu conducted the initial literature search and completed the outline of the manuscript. L Zhang wrote the original manuscript. All authors reviewed and critically revised previous versions of the manuscript. All authors read and approved the final manuscripts.
Financial & competing interests disclosure
This work was supported by National Natural Science Foundation of China (grant no. 81972057, 81670942), the Natural Science Foundation of Shandong Province (grant no. ZR2017MH004), and the Science and Technology Development Foundation of Jinan (grant no. 201704123), and Special Funds for Taishan Scholar Project (tsqn202103180). The authors have no other relevant affiliations or financial involvement with any organization or entity with a financial interest in or financial conflict with the subject matter or materials discussed in the manuscript apart from those disclosed.
No writing assistance was utilized in the production of this manuscript.
Additional information
Funding
References
- WHO . Deafness and hearing loss (2022). www.who.int/en/news-room/fact-sheets/detail/deafness-and-hearing-loss
- Zhang Z , LiX, ZhangW, KohaneDS. Drug delivery across barriers to the middle and inner ear. Adv. Funct. Mater.31(44), 2008701 (2021).
- Nyberg S , AbbottNJ, ShiX, SteygerPS, DabdoubA. Delivery of therapeutics to the inner ear: the challenge of the blood–labyrinth barrier. Sci. Transl. Med.11(482), eaao0935 (2019).
- Ruckenstein MJ . Autoimmune inner ear disease. Curr. Opin. Otolaryngol. Head Neck Surg.12(5), 426–430 (2004).
- Buniel MC , Geelan-HansenK, WeberPC, TuohyVK. Immunosuppressive therapy for autoimmune inner ear disease. Immunotherapy1(3), 425–34 (2009)
- Alexander TH , WeismanMH, DereberyJMet al. Safety of high-dose corticosteroids for the treatment of autoimmune inner ear disease. Otol. Neurotol.30(4), 443–448 (2009).
- Fowler EP Jr . Streptomycin treatment of vertigo. Trans. Am. Acad. Ophthalmol. Otolaryngol.52, 293–301 (1948).
- Sataloff RT , McCarterA, SpiegelJR. Very high-dose streptomycin labyrinthectomy. Ear Nose Throat J.75(4), 239–243 (1996).
- Berryhill WE , GrahamMD. Chemical and physical labyrinthectomy for Meniere’s disease. Otolaryngol. Clin. North Am.35(3), 675–682 (2002).
- Wilson WR , BylFM, LairdN. The efficacy of steroids in the treatment of idiopathic sudden hearing loss. A double-blind clinical study. Arch. Otolaryngol.106(12), 772–776 (1980).
- Schuknecht HF . Ablation therapy for the relief of Ménière’s disease. Laryngoscope66(7), 859–870 (1956).
- Rauch SD , HalpinCF, AntonelliPJet al. Oral vs intratympanic corticosteroid therapy for idiopathic sudden sensorineural hearing loss: a randomized trial. JAMA305(20), 2071–2079 (2011).
- Wang Y , GaoG, WangL, MaX, YuL, YeF. Association between the number of intratympanic steroid injections and hearing recovery in sudden sensorineural hearing loss. Front. Neurol.12, 798569 (2021).
- Lehner E , LiebauA, SyrowatkaF, KnolleW, PlontkeSK, MäderK. Novel biodegradable round window disks for inner ear delivery of dexamethasone. Int. J. Pharm.594, 120180 (2021).
- Yang KJ , SonJ, JungSYet al. Optimized phospholipid-based nanoparticles for inner ear drug delivery and therapy. Biomaterials171, 133–143 (2018).
- Jeong SH , KimY, LyuARet al. Junctional modulation of round window membrane enhances dexamethasone uptake into the inner ear and recovery after NIHL. Int. J. Mol. Sci.22(18), 10061 (2021).
- Arriaga M , ArteagaDN, FafalisDet al. Membrane curvature and connective fiber alignment in guinea pig round window membrane. Acta Biomater.136, 343–362 (2021).
- Chen K , WangF, DingRet al. Adhesive and injectable hydrogel microspheres for inner ear treatment. Small18(36), e2106591 (2022).
- Gu J , ChenY, TongL, WangX, YuD, WuH. Astaxanthin-loaded polymer-lipid hybrid nanoparticles (ATX-LPN): assessment of potential otoprotective effects. J. Nanobiotechnol.18(1), 53 (2020).
- Husseman J , RaphaelY. Gene therapy in the inner ear using adenovirus vectors. In: Gene Therapy of Cochlear Deafness.RyanAF ( Ed.). Karger AG, Basel, Switzerland, 37–51 (2009).
- An X , ZhaD. Development of nanoparticle drug-delivery systems for the inner ear. Nanomedicine (Lond.)15(20), 1981–1993 (2020).
- Mittal R , PenaSA, ZhuAet al. Nanoparticle-based drug delivery in the inner ear: current challenges, limitations and opportunities. Artif. Cells Nanomed. Biotechnol.47(1), 1312–1320 (2019).
- Zhang L , XuY, CaoW, XieS, WenL, ChenG. Understanding the translocation mechanism of PLGA nanoparticles across round window membrane into the inner ear: a guideline for inner ear drug delivery based on nanomedicine. Int. J. Nanomed.13, 479–492 (2018).
- Dai J , LongW, LiangZ, WenL, YangF, ChenG. A novel vehicle for local protein delivery to the inner ear: injectable and biodegradable thermosensitive hydrogel loaded with PLGA nanoparticles. Drug Dev. Ind. Pharm.44(1), 89–98 (2018).
- Kuang X , ZhouS, GuoW, WangZ, SunY, LiuH. SS-31 peptide enables mitochondrial targeting drug delivery: a promising therapeutic alteration to prevent hair cell damage from aminoglycosides. Drug Deliv.24(1), 1750–1761 (2017).
- Wen X , DingS, CaiHet al. Nanomedicine strategy for optimizing delivery to outer hair cells by surface-modified poly(lactic/glycolic acid) nanoparticles with hydrophilic molecules. Int. J. Nanomed.11, 5959–5969 (2016).
- El Kechai N , AgnelyF, MamelleE, NguyenY, FerraryE, BochotA. Recent advances in local drug delivery to the inner ear. Int. J. Pharm.494(1), 83–101 (2015).
- Lajud SA , NagdaDA, QiaoPet al. A novel chitosan-hydrogel-based nanoparticle delivery system for local inner ear application. Otol. Neurotol.36(2), 341–7 (2015).
- Vigani B , RossiS, SandriG, BonferoniMC, CaramellaCM, FerrariF. Hyaluronic acid and chitosan-based nanosystems: a new dressing generation for wound care. Expert Opin Drug Deliv.16(7), 715–740 (2019).
- Zhao Z , HanZ, NaveenaKet al. ROS-responsive nanoparticle as a berberine carrier for OHC-targeted therapy of noise-induced hearing loss. ACS Appl. Mater. Interfaces13(6), 7102–7114 (2021).
- Dawoud MZ , NasrM. Comparison of drug release from liquid crystalline monoolein dispersions and solid lipid nanoparticles using a flow cytometric technique. Acta Pharm. Sin. B6(2), 163–169 (2016).
- Teixeira MC , CarboneC, SoutoEB. Beyond liposomes: recent advances on lipid based nanostructures for poorly soluble/poorly permeable drug delivery. Prog. Lipid Res.68, 1–11 (2017).
- Danaei M , DehghankholdM, AtaeiSet al. Impact of particle size and polydispersity index on the clinical applications of lipidic nanocarrier systems. Pharmaceutics10(2), 57 (2018).
- Arana L , SaladoC, VegaSet al. Solid lipid nanoparticles for delivery of Calendula officinalis extract. Colloids Surf. B Biointerfaces135, 18–26 (2015).
- Arana L , Bayón-CorderoL, SarasolaLI, BerasategiM, RuizS, AlkortaI. Solid lipid nanoparticles surface modification modulates cell internalization and improves chemotoxic treatment in an oral carcinoma cell line. Nanomaterials (Basel)9(3), 464 (2019).
- Raza A , SimeFB, CabotPJ, MaqboolF, RobertsJA, FalconerJR. Solid nanoparticles for oral antimicrobial drug delivery: a review. Drug Discov. Today24(3), 858–866 (2019).
- Zhang M , XiaoB, WangHet al. Edible ginger-derived nano-lipids loaded with doxorubicin as a novel drug-delivery approach for colon cancer therapy. Mol. Ther.24(10), 1783–1796 (2016).
- Serpe L , CatalanoMG, CavalliRet al. Cytotoxicity of anticancer drugs incorporated in solid lipid nanoparticles on HT-29 colorectal cancer cell line. Eur. J. Pharm. Biopharm.58(3), 673–680 (2004).
- Shegokar R , MüllerRH. Nanocrystals: industrially feasible multifunctional formulation technology for poorly soluble actives. Int. J. Pharm.399(1-2), 129–139 (2010).
- Cervantes B , AranaL, Murillo-CuestaSet al. Solid lipid nanoparticles loaded with glucocorticoids protect auditory cells from cisplatin-induced ototoxicity. J. Clin. Med.8(9), 1464 (2019).
- Gao G , LiuY, ZhouCH, JiangP, SunJJ. Solid lipid nanoparticles loaded with edaravone for inner ear protection after noise exposure. Chin. Med. J. (Engl.)128(2), 203–209 (2015).
- Panahi Y , FarshbafM, MohammadhosseiniMet al. Recent advances on liposomal nanoparticles: synthesis, characterization and biomedical applications. Artif. Cells Nanomed. Biotechnol.45(4), 788–799 (2017).
- Shin MC , ZhangJ, MinKAet al. Cell-penetrating peptides: achievements and challenges in application for cancer treatment. J. Biomed. Mater. Res. A102(2), 575–587 (2014).
- Qin L , WangCZ, FanHJet al. A dual-targeting liposome conjugated with transferrin and arginine-glycine- aspartic acid peptide for glioma-targeting therapy. Oncol. Lett.8(5), 2000–2006 (2014).
- Saeed M , van BrakelM, ZalbaSet al. Targeting melanoma with immunoliposomes coupled to anti-MAGE A1 TCR-like single-chain antibody. Int. J. Nanomed.11, 955–975 (2016).
- Yue PJ , HeL, QiuSWet al. OX26/CTX-conjugated PEGylated liposome as a dual-targeting gene delivery system for brain glioma. Mol. Cancer13, 191 (2014).
- Esposito CL , CatuognoS, de FranciscisV. Aptamer-mediated selective delivery of short RNA therapeutics in cancer cells. J. RNAi Gene Silencing10, 500–506 (2014).
- Wu YY , HsiehIS, TungCHet al. A novel DNA aptamer targeting lung cancer stem cells exerts a therapeutic effect by binding and neutralizing annexin A2. Mol. Ther. Nucleic Acids27, 956–968 (2022).
- Cui SH , ZhiDF, ZhaoYNet al. Cationic lioposomes with folic acid as targeting ligand for gene delivery. Bioorg. Med. Chem. Lett.26(16), 4025–4029 (2016).
- Meyer H , StöverT, FouchetFet al. Lipidic nanocapsule drug delivery: neuronal protection for cochlear implant optimization. Int. J. Nanomed.7, 2449–2464 (2012).
- Hou S , YangY, ZhouSet al. Novel SS-31 modified liposomes for improved protective efficacy of minocycline against drug-induced hearing loss. Biomater. Sci.6(6), 1627–1635 (2018).
- Chaurasiya A , GorajiyaA, PanchalK, KatkeS, SinghAK. A review on multivesicular liposomes for pharmaceutical applications: preparation, characterization, and translational challenges. Drug Deliv. Transl. Res.12(7), 1569–1587 (2022).
- Abuzar SM , ParkEJ, SeoY, LeeJ, BaikSH, HwangSJ. Preparation and evaluation of intraperitoneal long-acting oxaliplatin-loaded multi-vesicular liposomal depot for colorectal cancer treatment. Pharmaceutical12(8), 736 (2020).
- Luo Y , LiuZ, ZhangXet al. Effect of a controlled-release drug delivery system made of oleanolic acid formulated into multivesicular liposomes on hepatocellular carcinoma in vitro and in vivo. Int. J. Nanomed.11, 3111–3129 (2016).
- Yang D , XuY, LiF, LiuH, HeX. [Preparation of cationic vancomycin hydrochloride multivesicular liposomes and its quality]. Zhongguo Xiu Fu Chong Jian Wai Ke Za Zhi27(4), 443–448 (2013).
- Li Y , ZhangR, LiX, LiW, LuY, DaiC. The preparation of dexamethasone sodium phosphate multivesicular liposomes thermosensative hydrogel and its impact on noise-induced hearing loss in the Guinea pigs. Exp. Cell Res.387(1), 111755 (2020).
- Obireddy SR , LaiWF. ROS-generating amine-functionalized magnetic nanoparticles coupled with carboxymethyl chitosan for pH-responsive release of doxorubicin. Int. J. Nanomed.17, 589–601 (2022).
- Martín MJ , SpitzmaulG, LassalleV. Novel insights and perspectives for the diagnosis and treatment of hearing loss through the implementation of magnetic nanotheranostics. ChemMedChem17(5), e202100685 (2022).
- Hu Y , LiD, WeiHet al. Neurite extension and orientation of spiral ganglion neurons can be directed by superparamagnetic iron oxide nanoparticles in a magnetic field. Int. J. Nanomed.16, 4515–4526 (2021).
- Mondalek FG , ZhangYY, KroppBet al. The permeability of SPION over an artificial three-layer membrane is enhanced by external magnetic field. J. Nanobiotechnol.4, 4 (2006).
- Knight KR , ChenL, FreyerDet al. Group-wide, prospective study of ototoxicity assessment in children receiving cisplatin chemotherapy (ACCL05C1): a report from the children’s oncology group. J. Clin. Oncol.35(4), 440–445 (2017).
- Sheth S , MukherjeaD, RybakLP, RamkumarV. Mechanisms of cisplatin-induced ototoxicity and otoprotection. Front. Cell. Neurosci.11, 338 (2017).
- Kayyali MN , RamseyAJ, Higbee-DempseyEMet al. The development of a nano-based approach to alleviate cisplatin-induced ototoxicity. J. Assoc. Res. Otolaryngol.19(2), 123–132 (2018).
- Lohiya G , KattiDS. Carboxylated chitosan-mediated improved efficacy of mesoporous silica nanoparticle-based targeted drug delivery system for breast cancer therapy. Carbohydr. Polym.277, 118822 (2022).
- Shen Y , LiM, LiuTet al. A dual-functional HER2 aptamer-conjugated, pH-activated mesoporous silica nanocarrier-based drug delivery system provides in vitro synergistic cytotoxicity in HER2-positive breast cancer cells. Int. J. Nanomed.14, 4029–4044 (2019).
- Pinese C , LinJ, MilbretaUet al. Sustained delivery of siRNA/mesoporous silica nanoparticle complexes from nanofiber scaffolds for long-term gene silencing. Acta Biomater.76, 164–177 (2018).
- Cheang TY , TangB, XuAWet al. Promising plasmid DNA vector based on APTES-modified silica nanoparticles. Int. J. Nanomed.7, 1061–1067 (2012).
- Liu T , LiuH, FuCet al. Silica nanorattle with enhanced protein loading: a potential vaccine adjuvant. J. Colloid Interface Sci.400, 168–174 (2013).
- Mody KT , PopatA, MahonyD, CavallaroAS, YuC, MitterN. Mesoporous silica nanoparticles as antigen carriers and adjuvants for vaccine delivery. Nanoscale5(12), 5167–5179 (2013).
- Lu J , NiC, HuangJet al. Biocompatible mesoporous silica-polydopamine nanocomplexes as MR/fluorescence imaging agent for light-activated photothermal–photodynamic cancer therapy in vivo. Front. Bioeng. Biotechnol.9, 752982 (2021).
- Guo X , ZhuM, YuanPet al. The facile formation of hierarchical mesoporous silica nanocarriers for tumor-selective multimodal theranostics. Biomater. Sci.9(15), 5237–5246 (2021).
- Baek S , SinghRK, KhanalDet al. Smart multifunctional drug delivery towards anticancer therapy harmonized in mesoporous nanoparticles. Nanoscale7(34), 14191–14216 (2015).
- Cao M , WangP, KouYet al. Gadolinium (III)-chelated silica nanospheres integrating chemotherapy and photothermal therapy for cancer treatment and magnetic resonance imaging. ACS Appl. Mater. Interfaces7(45), 25014–25023 (2015).
- Pritz CO , BitscheM, SalvenmoserW, DudásJ, Schrott-FischerA, GlueckertR. Endocytic trafficking of silica nanoparticles in a cell line derived from the organ of Corti. Nanomedicine (Lond.)8(2), 239–252 (2013).
- Gunewardene N , LamP, MaYet al. Pharmacokinetics and biodistribution of supraparticle-delivered neurotrophin 3 in the guinea pig cochlea. J. Control Release342, 295–307 (2022).
- Yang Z , WangD, ZhangCet al. The applications of gold nanoparticles in the diagnosis and treatment of gastrointestinal cancer. Front. Oncol.11, 819329 (2022).
- Kayyali MN , BrakeL, RamseyAJ, WrightAC, O’MalleyBW, LiDD. A novel nano-approach for targeted inner ear imaging. J. Nanomed. Nanotechnol.8(4), 456 (2017).
- Lin YC , ShihCP, ChenHCet al. Ultrasound microbubble-facilitated inner ear delivery of gold nanoparticles involves transient disruption of the tight junction barrier in the round window membrane. Front. Pharmacol.12, 689032 (2021).
- Merino S , MartínC, KostarelosK, PratoM, VázquezE. Nanocomposite hydrogels: 3D polymer-nanoparticle synergies for on-demand drug delivery. ACS Nano9(5), 4686–4697 (2015).
- Li C , ObireddySR, LaiWF. Preparation and use of nanogels as carriers of drugs. Drug Deliv.28(1), 1594–1602 (2021).
- Kayyali MN , WooltortonJRA, RamseyAJet al. A novel nanoparticle delivery system for targeted therapy of noise-induced hearing loss. J. Control Release279, 243–250 (2018).
- Chen Y , GuJ, LiuJet al. Dexamethasone-loaded injectable silk-polyethylene glycol hydrogel alleviates cisplatin-induced ototoxicity. Int. J. Nanomed.14, 4211–4227 (2019).