Abstract
Aim: The Combining Mechanisms for Better Outcomes randomized controlled trial assessed the effectiveness of various spinal cord stimulation (SCS) modalities for chronic pain. Specifically, combination therapy (simultaneous use of customized sub-perception field and paresthesia-based SCS) versus monotherapy (paresthesia-based SCS) was evaluated. Methods: Participants were prospectively enrolled (key inclusion criterion: chronic pain for ≥6 months). Primary end point was the proportion with ≥50% pain reduction without increased opioids at the 3 month follow-up. Patients were followed for 2 years. Results: The primary end point was met (n = 89; p < 0.0001) in 88% of patients in the combination-therapy arm (n = 36/41) and 71% in the monotherapy arm (n = 34/48). Responder rates at 1 and 2 years (with available SCS modalities) were 84% and 85%, respectively. Sustained functional outcomes improvement was observed out to 2 years. Conclusion: SCS-based combination therapy can improve outcomes in patients with chronic pain.
Clinical Trial Registration: NCT03689920 (ClinicalTrials.gov), Combining Mechanisms for Better Outcomes (COMBO)
Plain language summary
Spinal cord stimulation (SCS) is a device-based therapy for chronic pain that delivers electrical impulses to the spinal cord, disrupting pain signals to the brain. Pain relief can be achieved using different SCS techniques that use or do not use paresthesia (stimulation that produces a tingling sensation). These approaches affect patients in different ways, suggesting that different biological processes are involved in enabling pain relief. Research also suggests that better long-term results occur when patients can choose the therapy that is best for their own needs. This clinical study compared pain relief and other functional activities in those receiving combination therapy (simultaneous use of SCS that does and does not produce tingling sensation) against those receiving monotherapy (only SCS therapy producing tingling sensation) for 3 months. In the study, 88% of those receiving combination therapy and 71% with monotherapy alone reported a 50% (or greater) decrease in overall pain (the ‘responder rate’) without an increased dose of opioid drugs at 3 months after the start of therapy. This responder rate was found to be 84% at 1 year and 85% at 2 years (with all SCS therapy options available). Analysis of functional activities or disability showed that patients improved from ‘severely disabled’ at study start to ‘moderately disabled’ after 2 years, indicating that effective long-term (2 year) improvement can be achieved using SCS-based combination therapy for chronic pain.
Chronic neuropathic pain affects millions worldwide and impacts various aspects of the lives of patients. Existing treatments for pain include medications such as antidepressants, antiepileptics, opioids and other therapies. As such, clinicians frequently combine drugs from different classes, and this has been shown to improve effectiveness compared with monotherapy [Citation1,Citation2]. Likewise, use of interventional therapies via a multimodal therapeutic strategy, such as the implementation of comprehensive integrative pain management, has now been publicly recommended by pain care advocates [Citation3]. Treating chronic neuropathic pain using spinal cord stimulation (SCS) is a well-established interventional therapeutic approach. Rapid advances in technology, coupled with the global opioid drug crisis, have reinforced the practical utility of SCS as a treatment approach for chronic neuropathic pain [Citation4,Citation5]. Common application of SCS relies on the generation of paresthesia (i.e., tingling) that masks the perception of pain and disrupts neuronal firing patterns via the use of tonic stimulation frequencies of 40–100 Hz. More recently, novel SCS waveforms that do not rely on the perception of paresthesia have increased therapeutic options so as to improve outcomes and the overall experience implanted patients have with SCS therapy [Citation6–8]. Despite the introduction of these additional approaches, challenges associated with the treatment of chronic pain using SCS exist and opportunities to further improve the efficacy of SCS therapy are needed [Citation9–12].
One aspect thought to play a pivotal role in improving outcomes with regard to treating chronic pain using SCS is the selective localization of neurostimulation for anatomical areas tangibly associated with patients’ perception of chronic pain [Citation13]. Published studies have specified the use of current steering and contoured neurostimulation fields for more directed targeting of dorsal column and dorsal horn elements that are thought to mediate pathological pain signals, while concurrently avoiding side effects associated with off-target stimulation, such as those that can occur at the dorsal root [Citation14,Citation15]. Adjustment of anode–cathode configuration was the first to be reported as an approach to generate greater control over dorsal column versus dorsal root activation [Citation15]. Multiple independent current control (MICC) was subsequently developed as a technique enabling controlled fractionalization of current among different electrodes to further enhance the shaping of neurostimulation fields [Citation16]. Later, anatomically guided neural targeting technology was developed in order to better localize stimulation to the appropriate neural targets, further improving the ability to fine-tune SCS therapy using a programming algorithm designed on the basis of a finite-element model of the spinal canal that accounts for several patient-specific variables (e.g., relative lead positions, distance between leads, cerebrospinal fluid thickness and the electrical conductivities of several tissues) [Citation6].
In parallel to these technological and clinical advancements, a concerted effort was undertaken to obtain greater understanding of potential mechanisms that are thought to mediate the clinical effects of SCS. Various published in vivo animal and in silico modeling studies strongly suggest that not all neuronal responses to SCS can be attributed to mechanisms predicted by the gate control theory (GCT) [Citation17–19]. Evidence gathered from preclinical studies has in particular pointed to specific dorsal horn targets that possesses the neuronal elements capable of playing a role (at least in part) in the transmission of neuropathic pain signals governed by the GCT and/or other concomitant neural circuit mechanisms that, as of yet, are not completely understood but are thought likely to involve the active regulation of the neurotransmitter GABA [Citation20–23]. Moreover, other studies have shown that SCS approaches that utilize sub-perception and/or burst stimulation are likely mediating pain relief through alternative neural mechanisms dissimilar from conventional, paresthesia-based SCS [Citation24–26].
The collective implications of these studies led to the hypothesis that neurostimulation allowing for the combined implementation of sub-perception- and paresthesia-based SCS in conjunction with optimized stimulation of specific neuronal targets (including at both the dorsal horn and dorsal columns) may help to modulate pain-related neural activity more robustly. This in turn would offer increased opportunity to enhance and/or sustain analgesic outcomes by accessing one or more mechanisms that underpin the development and/or persistence of neuropathic pain. Therefore, an interventional treatment approach that engages multiple underlying mechanisms of action may be able to overcome the challenges associated with the complexity and subjective experience of chronic pain. In this regard, this is analogous to the combined use of multiple classes of drugs within the same patient so as to provide the best opportunity to achieve the most effective clinical outcomes. We thus proposed that the use of combination therapy (i.e., customized sub-perception-based field shape programming algorithm utilized together with paresthesia-based SCS) would elicit significant improvement in pain and functional outcomes. As such, we initiated a prospective, multicenter randomized controlled trial (RCT) which evaluated combination therapy compared with conventional monotherapy (i.e., paresthesia-based SCS only) alone.
Patients & methods
Study design & participants
COMBO (Combining Mechanisms for Better Outcomes) is a multicenter, prospective RCT in subjects with chronic pain of the trunk and/or limbs using an SCS device. This study was approved by the institutional review board for each site and registered at Clinicaltrials.gov (NCT03689920). To participate in the study, subjects were required to meet the following key inclusion criteria: age 22 years or older; chronic pain in the trunk and/or limbs for at least 6 months with low back pain greater than leg pain that was refractory to conservative therapy; no back surgery in the last 6 months; average overall pain intensity of 6 or higher on a 0–10 scale based on 7 day recall; stable prescription use if taking opioids; Oswestry Disability Index (ODI) score of 40–80; eligible for SCS after psychiatric screening and average daily morphine equivalent of 200 mg or less during the 30 days prior to the screening period (). Subjects who met any of the key exclusion criteria were withdrawn from the study: catastrophization based on physician evaluation; leg pain greater than back pain; spinal instability requiring fusion; pain of vascular origin; confounding medical/psychological conditions; cognitive impairment; participation in another drug/device trial; secondary spinal pain; referred visceral pain; current systemic infection; high surgical risk; need for MRI/diathermy or inability to operate the device.
d: Days; Mo.: Month.
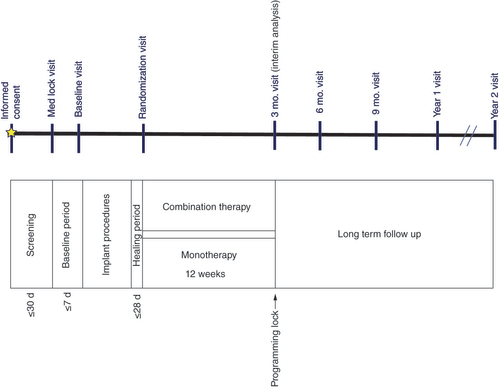
Written informed consent was obtained from each prospective participant prior to enrollment in the study. All data were collected by site personnel and/or completed by subjects only with no sponsor involvement. Pain and other functional outcome measures including disability and satisfaction were collected during in-office visits (no objective measures were used).
Following informed consent and initial screening for eligibility, subjects’ opioid medication was locked for 14 days (baseline period). At the baseline visit (), subjects’ pain intensity (0–10 numerical scale) and overall quality-of-life (including disability-related outcomes) were collected and served as baseline data for the purpose of analysis. All subjects underwent a trial per standard of care (with intraoperative testing as applicable). Following a successful trial (i.e., at least a 50% reduction in overall pain as compared with pain at baseline), subjects proceeded to permanent SCS implantation. All commercially approved SCS leads were available for use in the study, and no restrictions around the use of leads/lead placement were mandated in the study. Stimulation remained off for up to 14 days post-implant. At the randomization visit (day 0), subjects were randomized in a 1:1 ratio to receive either combination therapy (active arm) or monotherapy (control arm) for up to 3 months (90 days) post-randomization. Subjects in the combination therapy arm received simultaneous use of a customized sub-perception field-shape programming algorithm and paresthesia-based SCS, while those in the monotherapy arm received only paresthesia-based stimulation.
Subjects were instructed (informed consent form) that they would receive one of two modalities on their SCS device, and no bias related to the type of therapy they may receive was introduced. Subjects were not blinded in the study. Subjects’ devices were programmed based on treatment assignment to obtain optimal settings until their programming lock visit prior to 3 month follow-up. Following completion of the randomized phase, subjects were programmed with any of the commercially-available SCS parameters (e.g., combination therapy, paresthesia-based therapy and/or sub-perception therapies) as best suited per trial participant. Subjects continued in long-term follow-up out to 2 years () using all available programming modalities as needed.
Outcomes
The primary outcome measure was the proportion of subjects with a 50% or greater reduction in overall pain 3 months after randomization, without an increase in baseline average daily opioid use for pain management. Other measures assessed during study follow-up included percentage pain relief, perception of change as assessed by subjects (Patient Global Impression of Change; [PGIC]) and clinicians (Clinical Global Impression of Change), change in disability as measured by ODI, sleep (Pittsburg Sleep Quality Index) and overall satisfaction with treatment (treatment satisfaction questionnaire).
Statistical analysis
The primary statistical hypothesis in this study was that the proportion of subjects with ≥50% reduction from baseline in average daily overall pain intensity with no increase in opioid medications at 3 months post-randomization in the active group (combination therapy) would be non-inferior compared with the control group (i.e., monotherapy). Pain relief was measured by percentage pain relief from baseline. A total sample of 148 randomized subjects was required to show that the responder rate in the active group (combination therapy) is non-inferior (with a margin 20%) to that in the control group (monotherapy), assuming the responder rates in the active (combination therapy) and control (monotherapy) groups are 65 and 60%, respectively. The calculation was based on a two-arm parallel study of three interim locks, 1:1 randomization, one-sided with an α level of 0.025 and an 80% power (5% attrition rate). The evaluation of efficacy and safety end points was based on the intent-to-treat and per-protocol sets. These assessments and their methods were predefined in the statistical analysis plan prior to performing any of the analysis. Continuous variables were summarized using descriptive statistics, which include number of non-missing observations, mean, median, standard deviation (SD), minimum, maximum or 95% CI. The study’s non-inferiority margin is 0.20. The 95% CI of πt – πc was computed where πt and πc are responder rates in the treatment and control groups, respectively. The study was considered a success if, using the intent-to-treat analysis, the lower bound of the two-sided 95% CI for the difference was greater than -0.20. All statistical analysis was completed using software SAS v. 9.4 (SAS Institute, Inc., NC, USA). Randomization took place with a computer-generated data capture system using a pre-generated randomization table, stratified by site with various block sizes to ensure approximate balance of active versus control treatment allocation within each site.
Device description
Participating trial subjects were implanted with an SCS system (Spectra WaveWriter, Boston Scientific, CA, USA) that has capability for MICC, anatomically-guided neural targeting and patient-selectable neurostimulative programming options, as described previously [Citation6,Citation27]. All commercially approved SCS leads were available for use in the study. The system was specifically designed to provided optimized combination therapy by simultaneously delivering multiple stimulation waveforms. Additionally, a personalized field shape explicitly engineered for sub-perception SCS was utilized in this study (Contour, Boston Scientific) in order to modulate synaptic terminals in the superficial dorsal horn with high specificity while lessening dorsal column activation. As reported previously, this customized field shape programming algorithm establishes a constant electric field along the rostrocaudal axis that is thought to selectively engage the synaptic terminals of similarly aligned inhibitory interneurons and inhibitory descending pathways in the dorsal horn [Citation28]. This is achieved by distributing the anodic and cathodic charges over multiple electrodes used to shape the field with appropriate fractionalization using MICC. The fractionalization of the current on each electrode (anode or cathode) is determined using a proprietary computational model that considers the lead array geometry, the anatomical and physiological properties of spinal cord, and the desired electrical field. Moreover, the current fractionalization is personalized and calibrated to customize the field for each individual patient, based on the stimulation threshold evaluated for individual contacts. Compared with more conventionally used 8 mm bipolar electrode configurations, this algorithm produces a broader field over the spinal cord surface, so that a larger population of neural elements may be engaged without producing the sensation of paresthesia (). This is designed to thereby allow for selective modulation of inhibitory interneuron terminals and descending projections over that of dorsal column fibers [Citation29,Citation30].
(A) Produced by an 8 mm bipole configuration typical of conventional spinal cord stimulation and (B) algorithmic-based customized spinal cord stimulation configuration designed to more optimally provide for stimulation of the spinal dorsal horn.
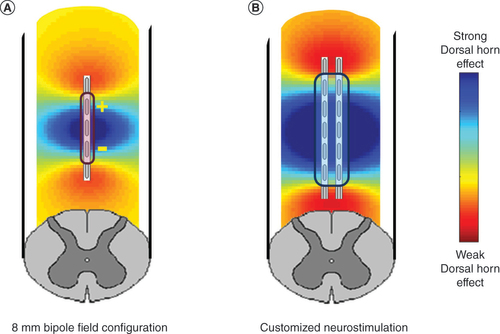
Role of funding source
Boston Scientific provided funding for the study and contributed to the study design, monitoring and data management. The sponsor had no role in data collection for the study. Boston Scientific personnel conducted the analysis and provided administrative and technical support as needed during the study. All content was reviewed and approved by all study authors.
Results
Between October 2018 and November 2019, 174 patients were enrolled across 14 sites. Of these 174 patients, a total of 124 proceeded to receive SCS trial (), of whom 85% (105/124) had a successful trial (≥50% pain reduction) and 100 were implanted with an SCS device. 99 patients were randomized and a prespecified cohort of 89 randomized patients were included in the analysis. 41 subjects were randomized to the active arm (combination therapy) and 48 to the control arm (monotherapy) (). Baseline clinical characteristics and demographics of those randomized are summarized in . The mean age of study participants at time of consent was 57.3 ± 11.86 (SD) years, and 65.2% (58 of 89) subjects were females. Reported average duration of low back and/or leg pain prior to study enrollment among all included subjects was determined to be 16.2 ± 13 (SD) years. The most prevalent subject diagnosis was failed back surgery syndrome (60.7%), followed by radiculopathy (22.5%). At baseline, subjects were classified as ‘severely disabled’ as assessed by a mean ODI score of 54.2 ± 8.9 (SD) with a mean overall Verbal Rating Scale (VRS) pain score of 7.4 ± 1.0 (SD) and mean low back VRS pain score of 7.6 ± 1.0 (SD).
A total of 174 subjects were enrolled, of whom 100 were implanted. A prespecified cohort of 89 randomized subjects were included in the interim analysis.
*Pre-specified cohort of 89 randomized subjects for analysis.
SCS: Spinal cord stimulation.
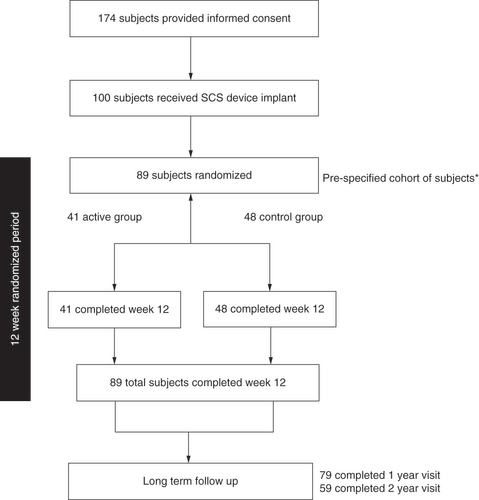
Table 1. Patient baseline characteristics and demographics.
The primary end point of the study (i.e., proportion of subjects with 50% or greater reduction in overall pain at 3 months post-randomization with no increase in baseline average daily opioid medications) was successfully met based on the prespecified cohort of 89 randomized subjects (p < 0.0001). A responder rate of 88% (36/41) was noted in those subjects receiving combination therapy compared with 71% (34/48) for the monotherapy group () at 3 month follow-up. A significant improvement in disability (as assessed by ODI) was noted in both groups (26-point improvement compared with baseline; p < 0.0001). Additionally, at 3 months, 90% of patients reported being ‘very much improved’ (39%) or ‘much improved’ (51%) with the use of combination therapy, compared with 79% with monotherapy (27% reported ‘very much improved’ and 52% reported ‘much improved’) based on PGIC scores, as shown in .
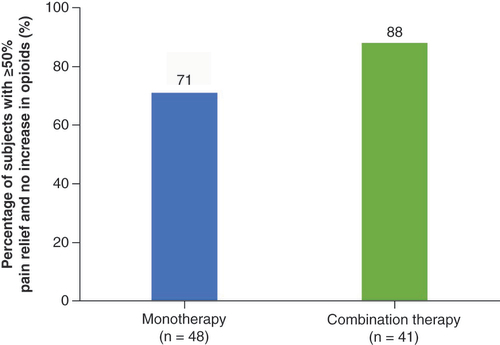
(A) Mean improvement in Oswestry Disability Index score at 3 month follow-up. (B) Proportion of subjects who reported being ‘much’ or ‘very much’ improved at 3 month follow-up.
ODI: Oswestry Disability Index; PGIC: Patient Global Impression of Change.
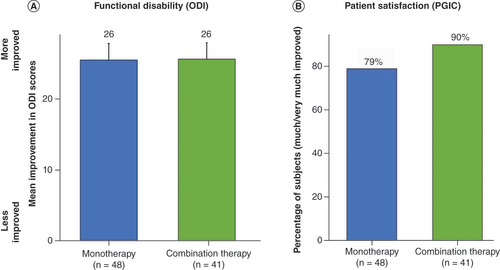
During long-term follow-up of overall pain (up to 2 years), 84 and 85% of patients were responders at 1 and 2 years, respectively (). For back pain, 83 and 85% of patients were responders at 1 and 2 years, respectively. In addition, mean improvement in disability (ODI) was sustained at these time points (25-point improvement at 2 years) as well as patient satisfaction as determined by PGIC: 85% reported being ‘very much improved’ (44%) or ‘much improved’ (41%) at 2 years.
Discussion
COMBO is the first multicenter, prospective RCT assessing the use of multiple SCS modalities delivered simultaneously (sub-perception-based and paresthesia-based SCS) with patient-specific optimization of customized neurostimulation fields (i.e., combination therapy) compared with monotherapy alone (paresthesia-based SCS only). At 3 months post-randomization, analysis of the primary end point demonstrated a higher responder rate in those allocated to receive combination therapy versus conventional monotherapy, and evaluation of secondary outcomes displayed a trend toward improved outcomes in the active group (vs the control group) randomly assigned to the combination therapy arm. During long-term follow-up, where all SCS modalities were available, subjects continued to experience highly effective outcomes out to 1 and 2 years, including a high responder rate, improved disability and substantial patient satisfaction.
The assessment of responder rate (as defined by a ≥50% reduction in pain at 3 month follow-up) is a key benchmark for clinical effectiveness and has been utilized to determine study success in previously reported RCTs evaluating SCS for chronic pain. In the SENZA clinical trial, an 84% responder rate at 3 months was reported with 10 kHz SCS compared with 44% with conventional (paresthesia-based only) SCS [Citation31]. The responder rate at 3 month follow-up (with no increase in opioids) of the monotherapy (paresthesia-based SCS) arm in the COMBO RCT was considerably higher (71%) than that reported in the SENZA study. The use of updated SCS systems with advanced neural targeting capabilities is likely key to these improved outcomes. However, with the use of SCS-based combination therapy, an even greater proportion of COMBO study participants achieved clinically effective pain relief, as demonstrated by a high responder rate (88%) at 3 month follow-up. As such, these combination therapy-associated response outcomes, as obtained in the COMBO RCT, represent some of the highest responder rates to be reported in the peer-reviewed literature when compared with other published RCTs assessing SCS for chronic pain [Citation31–36]. This would seem to be supportive of the general trend seen throughout much of the recent published literature indicating that SCS-implanted patients not only prefer therapy that allows for a greater level of ‘customizable’ treatment but are likely to clinically benefit from such an approach that addresses their needs on an individualized basis, in contrast to methodologies that place singular importance on application of a ‘monotherapeutic’ neurostimulative modality alone across all patients alike [Citation7,Citation37,Citation38].
Though advances in SCS technology have proliferated greatly in recent years, considerable emphasis has been placed on the utilization of specific waveforms applied as a monotherapy using less traditional frequencies (e.g., 1 kHz) and stimulation patterns (e.g., burst) for treatment of neuropathic pain [Citation34,Citation39–41]. Clinical implementation of monotherapy approaches has in part been supported on the basis of positive outcomes derived from various RCTs. Other published RCTs, though, have provided evidence suggesting that not all patients respond to a given monotherapy. Furthermore, some patients have noted reduced benefit over time with the use of monotherapies [Citation34,Citation35]. As such, different neurostimulative modalities likely do affect patients in variable ways via distinct underlying mechanisms, thereby supporting the use of SCS devices that allow for patient-selective use of multiple therapeutic options in order to provide effective pain relief [Citation35,Citation42,Citation43]. Furthermore, while improvement in pain relief will always be a key measure in SCS clinical studies, it is commonly held that assessment of SCS therapy should ideally include comprehensive measures beyond pain relief alone [Citation43]. As provided in this report, results obtained from the COMBO RCT with regard to changes in the level of functional disability and patient satisfaction reflect a substantial improvement in the overall disposition of SCS-implanted patients using combination therapy (). In particular, the derived magnitude of these improvements was higher than originally expected, per these same data measures reported in previously published monotherapy RCTs (i.e., change in disability improvement as measured by ODI: 25 points [COMBO RCT] vs 17 points [Citation44], 14 points [Citation45]; percentage patient satisfaction as measured by PGIC: 85% [COMBO RCT] vs 64% [Citation39], 54% [Citation46] and 60% [Citation47]).
The clinical trial results described in this report are the first to be obtained from a prospective RCT carried out on the basis of mounting data from preclinical animal model work suggesting that combining multiple modalities may have additional clinical benefit. Notably, though, one previously published study in human patients reported a restoration in pain relief in those with neuropathic pain that no longer responded to SCS treatment who subsequently then underwent intrathecal administration of baclofen (a derivative of GABA), thereby indicating that local spinal GABAergic mechanisms (which are known to occur in neurons within the dorsal horn) are seemingly involved in at least some of the analgesic capabilities of SCS [Citation48]. However, these mechanisms are likely occurring according to a complex interplay of neural circuits that extend beyond that of the canonical GCT. As shown in preclinical studies, SCS can inhibit a subpopulation of nociceptive-specific spinothalamic tract neurons found at the dorsal horn, and these neurons were observed in a rat model of neuropathic pain to exhibit responses to SCS not consistent with the canonical GCT [Citation18,Citation49]. In turn, this has led some researchers to propose various theories regarding how the clinical use of SCS (both sub-perception-based and paresthesia-based modalities) might elicit analgesic effects via circuits located at the dorsal horn [Citation49–51]. Collectively, these various studies help to provide motivation for the use of customized neurostimulation fields that are configured to preferentially stimulate specific neuronal targets, while also combining sub-perception- and paresthesia-based SCS modalities in order to more effectively treat patients with chronic neuropathic pain as conducted in the COMBO RCT.
Combination therapy was initially conceived to improve outcomes by combining various modalities to maximize their complementary effects. Additionally, it was also developed to enable more personalized therapy so as to help address potential complications such as lead migration, patient-to-patient variability and the loss of therapeutic effectiveness which can precede revision or explant and in turn result in increased healthcare costs [Citation52–60]. A customized stimulation field targeting algorithm, as available on the device under study as described in this report, is one such example of novel technology engineered to help address such concerns and so enable a more patient-specific approach toward the utilization of SCS for treatment of chronic pain. We therefore submit that SCS devices with robust flexibility for neurostimulative manipulation in the context of combination therapy are suitably equipped to help manage unexpected treatment complications and patient-to-patient variation. As such, SCS-based combination therapy represents a fitting addition to the comprehensive integrative pain management-based ‘toolbox’ of pain care therapies, so as to support the treatment of pain, to the furthest extent possible, in a ‘person-centered’ manner.
It should be noted that this trial is not without limitations. First, blinding of participating subjects and clinicians was not implemented. Moreover, a comparator to a sub-perception-based therapy or sham was not included. As a result, it was not possible to tease apart the relative contribution of the different SCS modalities utilized in combination therapy in this trial.
Conclusion
The clinical results derived from this multicenter, prospective RCT demonstrate that an SCS system capable of simultaneous delivery of multiple modalities enables more patients to achieve a successful outcome than monotherapy alone, per the positive outcomes attained including improvement in overall pain, back pain, disability and satisfaction out to 2 years’ follow-up. The COMBO RCT thus provides evidence for the inclusion of SCS-based combination therapy as an important part of the therapeutic armamentarium now available in the pursuit of improving the lives of patients in need, reducing healthcare costs and decreasing the overall societal burden of chronic pain.
A multimodal approach is now widely thought to be key to achieving successful clinical outcomes in patients suffering from chronic pain.
Patients implanted with a spinal cord stimulation (SCS) device exhibit substantial variability in their experience of chronic pain and often show a preference for the availability of multiple modalities within a single device.
Various SCS approaches classified as either paresthesia-based or sub-perception-based are implicated to mediate pain relief using different mechanisms of action.
SCS-based combination therapy, similar to the combined use of different classes of therapeutic drugs, provides for the simultaneous delivery of paresthesia-based and sub-perception-based neurostimulation in patients with chronic pain.
The COMBO randomized controlled trial compares outcomes with the use of combination therapy (paresthesia-based SCS and sub-perception-based SCS applied simultaneously) versus monotherapy (paresthesia-based SCS alone).
Primary end point analysis (responder rate [≥50% pain relief] with no increase in opioids) showed that patients using combination therapy had a higher responder rate (88%) versus those using monotherapy alone (71%).
The responder rates at 1 and 2 years, during which subjects were able to use all available programming modalities, were 84 and 85%, respectively (low back pain responder rate at 2 years: 85%).
At 2-year follow-up, significant improvement in functional outcomes was observed, including the level of disability (25-point reduction in Oswestry Disability Index score) and patient satisfaction (85% of patients reported being either ‘very much improved’ [44%] or ‘much improved’ [41%] when assessed with the Patient Global Impression of Change questionnaire).
The COMBO randomized controlled trial provides evidence that SCS-based combination therapy can provide better clinical outcomes than paresthesia-based SCS alone, thereby supporting SCS-based combination therapy as an important tactic to consider when treating patients using SCS for chronic neuropathic pain.
Author contributions
Conception and design: M Wallace, L Chen and R Jain; Patient enrollment and data collection: all authors; data analysis: L Chen; manuscript preparation: M Wallace and R Jain. All authors have critically reviewed and approved the published version of the submitted manuscript.
Ethical conduct of research
The authors state that they have obtained appropriate institutional review board approval or have followed the principles outlined in the Declaration of Helsinki for all human or animal experimental investigations. In addition, for investigations involving human subjects, informed consent has been obtained from the participants involved.
Acknowledgments
The authors wish to express their great appreciation to D Halperin for providing substantial contribution to the writing and editing of this published manuscript, and to R Carbunaru and N Patel for their intellectual input and editorial review.
Financial & competing interests disclosure
M Wallace reports a consulting agreement from Boston Scientific; J North reports a consulting agreement with Boston Scientific; G Phillips reports a consulting agreement with Boston Scientific; A Calodney reports consultant fees or receives research support from Medtronic, Nevro, Stryker, Saluda, Nalu, Boston Scientific, Vertos, Painteq, Stimgenics, Spine BioPharma, Saol Therapeutics, Tissuetech, BioRestorative, FUSMobile and APEX Biologix; J Scowcroft reports research support from Boston Scientific, Nevro and Saluda Medical; B Popat-Lewis reports no conflicts; J Lee reports a consulting agreement with Boston Scientific; E Washabaugh III reports a consulting agreement and research funding from Boston Scientific, and research funding from Vertos; J Paez reports no conflicts; R Bolash reports a consulting agreement with AcelRx, Lilly, Medtronic, Nuvectra, Premier, Salix, TerCera, Jazz Pharmaceuticals and Pfizer; J Noles reports no conflict; J Atallah reports a consulting agreement with Boston Scientific; B Shah reports no conflicts; F Ahadian reports no conflicts; D Trainor reports a consulting agreement with Boston Scientific; L Chen reports employment with Boston Scientific; R Jain reports employment with Boston Scientific. The authors have no other relevant affiliations or financial involvement with any organization or entity with a financial interest in or financial conflict with the subject matter or materials discussed in the manuscript apart from those disclosed.
No writing assistance was utilized in the production of this manuscript.
Data sharing statement
The authors certify that this manuscript reports original clinical trial data of NCT01719055. Individual and deidentified participant data will not be available. The data, analytical methods and study materials for this clinical study will be made available to other researchers in accordance with Boston Scientific’s data sharing policy (https://www.bostonscientific.com).
Additional information
Funding
References
- Gilron I , JensenTS , DickensonAH. Combination pharmacotherapy for management of chronic pain: from bench to bedside. Lancet Neurol.12(11), 1084–1095 (2013).
- Chaparro LE , WiffenPJ , MooreRA , GilronI. Combination pharmacotherapy for the treatment of neuropathic pain in adults. Cochrane Database Syst. Rev.2012(7), CD008943 (2012).
- US Department of Health and Human Services, Alliance to Advance Comprehensive Integrative Pain Management . Pain Management Best Practices Inter-Agency Task Force Report (2019). https://painmanagementalliance.org/resources/hhs-report-2019/
- Bergen-Cico D , SchollS , IvanashviliN , CicoR. Opioid prescription drug abuse and its relation to heroin trends. In: Neuropathology of Drug Addictions and Substance Misuse (Volume 1).PreedyVR ( Ed.). 878–887 (2016). www.researchgate.net/publication/305176315_Opioid_Prescription_Drug_Abuse_and_Its_Relation_to_Heroin_Trends
- Pollard EM , LamerTJ , MoeschlerSMet al. The effect of spinal cord stimulation on pain medication reduction in intractable spine and limb pain: a systematic review of randomized controlled trials and meta-analysis. J. Pain Res.12, 1311–1324 (2019).
- Veizi E , HayekSM , NorthJet al. Spinal cord stimulation (SCS) with anatomically guided (3D) neural targeting shows superior chronic axial low back pain relief compared to traditional SCS – LUMINA study. Pain Med.18(8), 1534–1548 (2017).
- Head J , MazzaJ , SabourinVet al. Waves of pain relief: a systematic review of clinical trials in spinal cord stimulation waveforms for the treatment of chronic neuropathic low back and leg pain. World Neurosurg.131, 264–274.e3 (2019).
- Maheshwari A , PopeJE , DeerTR , FalowskiS. Advanced methods of spinal stimulation in the treatment of chronic pain: pulse trains, waveforms, frequencies, targets, and feedback loops. Expert Rev. Med. Devices16(2), 95–106 (2019).
- Russo M , Van BuytenJP. 10-kHz High-frequency SCS therapy: a clinical summary. Pain Med.16(5), 934–942 (2015).
- Holsheimer J , KhanYN , RazaSS , KhanEA. Effects of electrode positioning on perception threshold and paresthesia coverage in spinal cord stimulation. Neuromodulation10(1), 34–41 (2007).
- Kumar K , CarawayDL , RizviS , BishopS. Current challenges in spinal cord stimulation. Neuromodulation17(Suppl. 1), 22–35 (2014).
- Fama CA , ChenN , PrusikJet al. The use of preoperative psychological evaluations to predict spinal cord stimulation success: our experience and a review of the literature. Neuromodulation19(4), 429–436 (2016).
- Molnar G , BarolatG. Principles of cord activation during spinal cord stimulation. Neuromodulation17(Suppl. 1), 12–21 (2014).
- Holsheimer J , WesselinkWA. Optimum electrode geometry for spinal cord stimulation: the narrow bipole and tripole. Med. Biol. Eng. Comput.35(5), 493–497 (1997).
- Holsheimer J , WesselinkWA. Effect of anode cathode configuration on paresthesia coverage in spinal cord stimulation. Neurosurgery41(3), 654–659 discussion 659–660 (1997).
- Bradley K . The technology: the anatomy of a spinal cord and nerve root stimulator: the lead and the power source. Pain Medicine7(Suppl. 1), S27–S34 (2006).
- Zhang TC , JanikJJ , PetersRVet al. Spinal sensory projection neuron responses to spinal cord stimulation are mediated by circuits beyond gate control. J. Neurophysiol.114(1), 284–300 (2015).
- Idlett S , HalderM , ZhangTCet al. Assessment of axonal recruitment using model-guided preclinical spinal cord stimulation in the ex vivo adult mouse spinal cord. J. Neurophysiol.122(4), 1406–1420 (2019).
- Guan Y , WacnikPW , YangFet al. Spinal cord stimulation-induced analgesia: electrical stimulation of dorsal column and dorsal roots attenuates dorsal horn neuronal excitability in neuropathic rats. Anesthesiology113(6), 1392–1405 (2010).
- Stiller CO , CuiJG , O’ConnorWT , BrodinE , MeyersonBA , LinderothB. Release of gamma-aminobutyric acid in the dorsal horn and suppression of tactile allodynia by spinal cord stimulation in mononeuropathic rats. Neurosurgery39(2), 367–374 discussion 374–375 (1996).
- Janssen SP , TruinM , Van KleefMet al. Differential GABAergic disinhibition during the development of painful peripheral neuropathy. Neuroscience184, 183–194 (2011).
- Janssen SP , GerardS , RaijmakersMEet al. Decreased intracellular GABA levels contribute to spinal cord stimulation-induced analgesia in rats suffering from painful peripheral neuropathy: the role of KCC2 and GABA(A) receptor-mediated inhibition. Neurochem. Int.60 (1), 21–30 (2012).
- Meuwissen KPV , de VriesLE , GuJWet al. Burst and tonic spinal cord stimulation both activate spinal GABAergic mechanisms to attenuate pain in a rat model of chronic neuropathic pain. Pain Pract.20(1), 75–87 (2020).
- Lee KY , BaeC , LeeDet al. Low-intensity, kilohertz frequency spinal cord stimulation differently affects excitatory and inhibitory neurons in the rodent superficial dorsal horn. Neuroscience428, 132–139 (2020).
- Chen Z , HuangQ , YangFet al. The impact of electrical charge delivery on inhibition of mechanical hypersensitivity in nerve-injured rats by sub-sensory threshold spinal cord stimulation. Neuromodulation22(2), 163–171 (2019).
- Arle JE , MeiL , CarlsonKW. Fiber threshold accommodation as a mechanism of burst and high-frequency spinal cord stimulation. Neuromodulation23(5), 582–593 (2019).
- Metzger CS , HammondMB , PylesSTet al. Pain relief outcomes using an SCS device capable of delivering combination therapy with advanced waveforms and field shapes. Expert Rev. Med. Devices17(9), 951–957 (2020).
- Paz-Solís J , ThomsonS , JainRet al. Exploration of high- and low-frequency options for subperception spinal cord stimulation using neural dosing parameter relationships: the HALO study. Neuromodulation25(1), 94–102 (2022).
- McIntyre CC , GrillWM. Selective microstimulation of central nervous system neurons. Ann. Biomed. Eng.28(3), 219–233 (2000).
- Radman T , RamosRL , BrumbergJC , BiksonM. Role of cortical cell type and morphology in subthreshold and suprathreshold uniform electric field stimulation in vitro. Brain Stimul.2(4), 215–228 228.e1-3 (2009).
- Kapural L , YuC , DoustMWet al. Novel 10-kHz high-frequency therapy (HF10 therapy) is superior to traditional low-frequency spinal cord stimulation for the treatment of chronic back and leg pain: the SENZA-RCT randomized controlled trial. Anesthesiology123(4), 851–860 (2015).
- Mekhail N , LevyRM , DeerTRet al. Long-term safety and efficacy of closed-loop spinal cord stimulation to treat chronic back and leg pain (Evoke): a double-blind, randomised, controlled trial. Lancet Neurol.19(2), 123–134 (2020).
- Rigoard P , BasuS , DesaiMet al. Multicolumn spinal cord stimulation for predominant back pain in failed back surgery syndrome patients: a multicenter randomized controlled trial. Pain160 (6), 1410–1420 (2019).
- North JM , HongKJ , ChoPY. Clinical outcomes of 1 kHz subperception spinal cord stimulation in implanted patients with failed paresthesia-based stimulation: results of a prospective randomized controlled trial. Neuromodulation19(7), 731–737 (2016).
- North J , LoudermilkE , LeeAet al. Outcomes of a multicenter, prospective, crossover, randomized controlled trial evaluating subperception spinal cord stimulation at ≤1.2 kHz in previously implanted subjects. Neuromodulation23(1), 102–108 (2020).
- De Andres J , Monsalve-DolzV , Fabregat-CidGet al. Prospective, randomized blind effect-on-outcome study of conventional vs high-frequency spinal cord stimulation in patients with pain and disability due to failed back surgery syndrome. Pain Med.18(12), 2401–2421 (2017).
- Liang L , DamianiA , BroccoMDet al. A systematic review of computational models for the design of spinal cord stimulation therapies: from neural circuits to patient-specific simulations. J. Physiol. doi: 10.1113/JP282884 (2022) ( Epub ahead of print).
- Ranjan M , KumarP , KonradP , RezaiAR. Finding optimal neuromodulation for chronic pain: waves, bursts, and beyond. Neurol. India68(Suppl.), S218–S223 (2020).
- Kapural L , YuC , DoustMWet al. Comparison of 10-kHz high-frequency and traditional low-frequency spinal cord stimulation for the treatment of chronic back and leg pain: 24-month results from a multicenter, randomized, controlled pivotal trial. Neurosurgery79(5), 667–677 (2016).
- Deer T , SlavinKV , AmirdelfanKet al. Success using neuromodulation with BURST (SUNBURST) study: results from a prospective, randomized controlled trial using a novel burst waveform. Neuromodulation21(1), 56–66 (2018).
- Wille F , BreelJS , BakkerEWet al. Altering conventional to high density spinal cord stimulation: an energy dose–response relationship in neuropathic pain therapy. Neuromodulation20(1), 71–80 (2017).
- Perruchoud C , EldabeS , BatterhamAMet al. Analgesic efficacy of high-frequency spinal cord stimulation: a randomized double-blind placebo-controlled study. Neuromodulation16(4), 363–369 discussion 369 (2013).
- Thomson SJ , TavakkolizadehM , Love-JonesSet al. Effects of rate on analgesia in kilohertz frequency spinal cord stimulation: results of the PROCO randomized controlled trial. Neuromodulation21(1), 67–76 (2018).
- Amirdelfan K , YuC , DoustMWet al. Long-term quality of life improvement for chronic intractable back and leg pain patients using spinal cord stimulation: 12-month results from the SENZA-RCT. Qual. Life Res.27(8), 2035–2044 (2018).
- US Food and Drug Administration . Senza Spinal Cord Stimulation (SCS) system: summary of safety and effectiveness data (SSED). www.accessdata.fda.gov/cdrh_docs/pdf13/P130022b.pdf
- St Jude Medical . Proclaim™ implantable pulse generator: clinician’s manual. https://manuals.sjm.com/~/media/manuals/product-manual-pdfs/1/5/15be12c6-7049-47a8-8d93-2277601dc99c.pdf
- Medtronic Lunch Symposium . ‘A mission to alleviate pain and restore life: past, present, and future of SCS’. Virtual Meeting of the North American Neuromodulation Society. 16 January 2021.
- Lind G , SchechtmannG , WinterJet al. Baclofen-enhanced spinal cord stimulation and intrathecal baclofen alone for neuropathic pain: long-term outcome of a pilot study. Eur. J. Pain12 (1), 132–136 (2008).
- Shiying L , FarberJP , LinderothBet al. Spinal cord stimulation with ‘conventional clinical’ and higher frequencies on activity and responses of spinal neurons to noxious stimuli: an animal study. Neuromodulation21(5), 440–447 (2018).
- Uno T . Possible mechanisms of spinal cord stimulation: disinhibition of the dorsal horn circuits and ascending nociceptive control. Neuromodulation23(3), 407–408 (2020).
- Taghipour M , GhaffarpasandF. Antinocioceptive effects of spinal cord stimulation by activation of periaqueductal gray matter and rostral ventromedial medulla: a mechanism beyond the gate control theory. Neuromodulation21(5), 520–521 (2018).
- Wang VC , BounkousohnV , FieldsK , BernsteinC , PaiciusRM , GilliganC. Explantation rates of high frequency spinal cord stimulation in two outpatient clinics. Neuromodulation24(3), 507–511 (2021).
- Hagedorn JM , LamCM , D’SouzaRSet al. Explantation of 10 kHz spinal cord stimulation devices: a retrospective review of 744 patients followed for at least 12 months. Neuromodulation24(3), 499–506 (2021).
- Horan M , JacobsenAH , SchererCet al. Complications and effects of dorsal root ganglion stimulation in the treatment of chronic neuropathic pain: a nationwide cohort study in Denmark. Neuromodulation24(4), 729–737 (2021).
- Fishman MA , AntonyA , EspositoM , DeerT , LevyR. The evolution of neuromodulation in the treatment of chronic pain: forward-looking perspectives. Pain Med.20(Suppl. 1), S58–S68 (2019).
- European Migraine and Headache Alliance . Marking 50 years of spinal cord stimulation (2017). www.emhalliance.org/news/curabitur-facilisis-pellentesque-pharetra-donec-justo-urna/
- Hayek SM , VeiziE , HanesM. Treatment-limiting complications of percutaneous spinal cord stimulator implants: a review of eight years of experience from an academic center database. Neuromodulation18(7), 603–608 (2015).
- Antonovich DD , GamaW , RitterAet al. Reoperation rates of percutaneous and paddle leads in spinal cord stimulator systems: a single-center retrospective analysis. Pain Med.22(1), 34–40 (2020).
- Negoita S , DuyPQ , MahajanUV , AndersonWS. Timing and prevalence of revision and removal surgeries after spinal cord stimulator implantation. J. Clin. Neurosci.62, 80–82 (2019).
- Chakravarthy K , RichterH , ChristoPJet al. Spinal cord stimulation for treating chronic pain: reviewing preclinical and clinical data on paresthesia-free high-frequency therapy. Neuromodulation21(1), 10–18 (2018).