Abstract
The physiologic process of blood clot formation is well understood and occurs naturally in the setting of tissue injury to achieve hemostasis and begin the process of wound healing. While the investigation of blood clots as a biomaterial is still in the early stages, there has been some research with similar biomaterials made of the components of blood clots that support the innovative idea of using an autologous blood clot as a scaffold or delivery method for therapeutic agents. Here, we review the physiology of blood clots in wound healing and how using blood clots as a biomaterial and delivery system can potentially promote wound healing, provide targeted therapeutic agent delivery and use it as an innovative tool in regenerative medicine.
Plain language summary
Wounds and tissue injuries are unfortunately still common, and research is very active in trying to discover ways to augment the healing process. Various biomaterials have been developed and studied to analyze their therapeutic potential in promoting regeneration of different tissues in the human body. The formation of blood clots is a natural process that occurs in response to tissue injury, and there has been recent work to investigate the utility of blood clots as therapeutic adjuncts. The practicality of obtaining blood clots, along with the feasibility of integrating therapeutic agents into them, make blood clots an attractive tool in regenerative medicine to be explored. This review article synthesizes current research on blood clot biology, physiology and potential therapeutic utility.
Blood clot formation & structure
Bleeding occurs after microvascular damage from tissue injury and is typically stopped naturally after a brief time through primary hemostasis involving platelet aggregation and platelet plug formation, and secondary hemostasis, which refers to the formation of fibrin through the coagulation cascade to strengthen and provide structure to the forming clot as presented in . These complex and intertwined pathways lead to the formation of a clot that stops bleeding at the site of injury, contributes to the wound healing process and prevents infection of the wound.
The process of natural clot formation with associated factors, tissues and molecules.
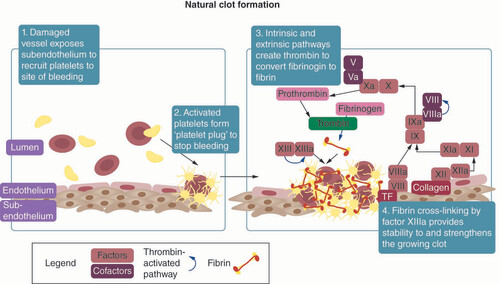
Primary hemostasis
The process of primary hemostasis involves interactions between vessel endothelium and platelets, leading to the formation of a platelet plug. In an uninjured state, the endothelial surface of vessels inhibits clot formation by expressing anticoagulants that keep blood from clotting. Once vascular damage occurs, the highly thrombogenic subendothelial tissue, which contains collagen, von Willebrand factor (vWF) and tissue factors (TFs), is exposed and initiates platelet adhesion to the injured site.
As platelets bind to the surface of the wound, they become activated and undergo a morphological transformation which increases their surface area and exposes binding ligands [Citation1]. After adhering to the exposed subendothelium, platelets secrete platelet-dense granules, thereby releasing ADP, calcium, serotonin and other agonists into the bloodstream that recruit and activate other nearby platelets [Citation2]. This positive feedback system of activated platelets promoting activation and adhesion of other nearby platelets serves to deposit a growing number of platelets on top of the initial platelets that were recruited and activated by the subendothelium. Additionally, this platelet aggregate provides a surface for the activation of other procoagulant factors. Thrombin, the terminal product of the coagulation cascade, also activates platelets by cleaving receptors on the platelet surface [Citation3]. The process of primary hemostasis results in the aggregation of platelets into a platelet plug that covers the wound and stops blood loss [Citation4,Citation5].
Secondary hemostasis
While primary hemostasis results in the formation of a platelet plug, secondary hemostasis concomitantly results in the formation of cross-linked fibrin mesh to stabilize the clot through the coagulation cascade [Citation6]. The coagulation cascade consists of an extrinsic pathway and an intrinsic pathway. The extrinsic pathway starts when vascular damage exposes TF on the subendothelium, which then binds to circulating factor VII in the blood stream to form a TF-VIIa complex. This complex then activates factors IX and X into factors IXa and Xa. Activated factor Xa converts factor II into thrombin (factor IIa), the terminal product of the extrinsic pathway. Even though the extrinsic pathway reactions lead to production of thrombin, this process alone does not generate enough thrombin required for clot formation [Citation5]. In addition, thrombin generation through this process is not considered robust, as this reaction is quickly terminated in the presence of TF pathway inhibitor.
Thus, the intrinsic pathway acts as a positive feedback loop to augment the production of thrombin that was initiated by the extrinsic pathway [Citation7]. This pathway is initiated when circulating factor XII binds to collagen in the subendothelium to become activated factor XIIa. In the presence of calcium, factor XIIa activates factor XI into factor XIa, which then activates factor IX into factor IXa. Factor IXa activates factor X into factor Xa, which again leads to the production of thrombin as in the extrinsic pathway. In the presence of thrombin, platelet-derived factor V is cleaved into factor Va and circulating factor VIII is activated into factor VIIIa. These factors Va and VIIIa serve as cofactors to accelerate the activation of factor Xa by factor IXa and consequently accelerates the activation of factor II into thrombin [Citation6].
Thrombin generation through the extrinsic and intrinsic pathways leads to the conversion of fibrinogen into fibrin, an insoluble fiber which stabilizes the platelet plug that was formed through the process of primary hemostasis. Once fibrin is generated, thrombin also promotes the activation of factor XIII, also known as fibrin stabilizing factor. This provides strength and stability to the growing fibrin network by crosslinking the fibrin fibers into a mesh that is more flexible and resistant to fibrinolysis [Citation7]. Both primary and secondary hemostasis contribute to the formation of a platelet plug and deposition of fibrin, resulting in the final structure of the clot (). Erythrocytes and white blood cells can also be incorporated into the thrombus and contribute to the stability of the clot and help protect the wound against infection [Citation8].
Factors affecting clot structure & stability
The structure and quality of the clot itself can depend on many variables, including fibrin thickness, length, and density, the concentrations of various components like thrombin, calcium and fibrinogen and physical conditions like pH and shear rate [Citation9–Citation10].
Biological factors
Fibrin fiber thickness and density within a blood clot is highly dependent on the concentration of thrombin present during clot formation. When low thrombin concentrations are present during clot formation, highly permeable and turbid (low optical density) fibrin clots composed of thick, loosely woven fibrin strands are produced. This fibrin pattern is correlated with weak, fragile clots that are prone to premature breakage and inadequate hemostasis. Conversely, when high concentrations of thrombin are present during clot development, clot formation time is significantly decreased and fibrinogen is converted into fibrin that exhibits a denser pattern of more thin strands, resulting in relatively non-turbid, less permeable clots. This fibrin pattern is correlated with stronger, more stable clots that are also more resistant to fibrinolysis [Citation9,Citation11].
Current understanding of clot formation and structure suggests that fibrin thickness varies throughout the clot. As the clot is in earlier stages of formation, lower thrombin concentrations result in a looser, more open pattern of fibrin being deposited. During later stages of clot formation, positive feedback from the coagulation cascade leads to a higher concentration of thrombin, which allows for thinner strands of fibrin to be densely deposited and further stabilize the clot [Citation12]. This process results in a blood clot that has a diverse range of fibrin fiber thicknesses throughout the clot.
A key consideration for the application of blood clots as therapeutics is their viscoelastic properties and the determinants that govern these structural aspects. Variables in the process of clotting can affect the viscoelastic properties of formed blood clots. One study found that the TF concentration and rate of clotting are determinants of clot microstructure, which then defines the rigidity and viscoelastic properties of clots [Citation13]. Additionally, clot elasticity is specifically determined by relative amounts of red blood cells and fibrin, with higher hematocrit clots having lower shear moduli and less rigidity than lower hematocrit clots [Citation14]. When considering the application of blood clots as a biomaterial, it is also important to consider how the blood clot will fare under stress and compression loads. Clots will lose water under deformation because they are porous, and relaxation of the clot subjected to mechanical stress after the stress is removed is inversely related to clot diameter [Citation15]. Along with consideration of the stresses to which blood clots will be subjected when used as a biomaterial, the size of clots chosen for therapeutic purposes must be optimized. Understanding these properties of blood clots is crucial to ensure the efficacy of blood clots developed as biomaterials for tissue healing.
Physiological factors
In addition, changes in blood pH can also influence coagulation dynamics. In the case of large traumatic injuries, oxygen delivery to tissue is severely impaired due to significant blood loss that leads to vasoconstriction and decreased cardiac output. Cells exposed to hypoxia undergo anerobic metabolism resulting in a lactic acidosis [Citation16]. Research has shown that acidosis as an outcome of traumatic injury has been linked to impaired coagulation efficiency by disrupting hemostasis and inhibiting the function of enzymatic reactions critical to the coagulation process. In one experiment, researchers examined the effects of a range of pH from 5.8 to 8.0 on clot formation dynamics while holding fibrinogen and thrombin concentrations constant [Citation17]. They discovered that increasing pH resulted in the formation of thinner fibrin fiber, leading to decreased clot turbidity and increased clot strength. By contrast, in clots formed at lower pH (<7.3), fibrin formation was significantly impacted, leading to higher overall turbidity, and decreased structural integrity of the fibrin. Clot formation pH may be an important factor to consider as patients experiencing severe trauma and acidosis may have decreased ability to form robust clots to stop bleeding. Varying pH conditions during clot formation may lead to an optimized clot structure for conjugation with other therapeutics.
Blood clot composition must be conducive to proper physiological functioning, and therefore the amounts of clot components must be optimal. Fracture mechanics of blood clots depend on the relative proportion of red blood cells to fibrin. It has been established that resistance to fracture increases with the amount of fibrin and decreases with the amount of red blood cells in the clot [Citation18]. However, a study that investigated fracture mechanics of whole blood clots concluded the contrary, and also determined that fracture resistance is independent of geometry and stress load [Citation19]. Possibly contributing to these conflicting reports is the fact that blood clot stiffness and fracture behavior is very difficult to quantify. The wet, fragile and soft nature of clots makes handling and testing them nontrivial, but further research on fracture behavior of clots is necessary to optimize their potential as therapeutic biomaterials.
Blood factors
Platelets are another essential component of blood clots that contribute to the structure of the overall blood clot, but even though much research has been conducted investigating platelet activation in diseased states, for example, hypercoagulable states and undesired thrombosis, not much is known about how changes in platelet activation affect overall clot quality and contribution to wound healing. Preliminary studies suggest that plasma may have an inhibitory effect on platelet aggregation and the release of PGFs [Citation20], but more research is needed to fully understand the impact of plasma and other factors influencing platelet activity within blood clots.
Fluid mechanics of the blood at the site of injury can also affect clot formation. The shear rate is the rate of change in velocity as one layer of fluid passes over an adjacent layer of fluid, in this case, blood. Under normal physiological conditions, the wall shear rate increases from roughly 10 s-1 in veins to about 2000 s-1 in the smallest arteries [Citation21]. This difference in blood shear rate results in different morphologies between venous and arterial blood clots. As the shear rate within a vessel increases, more platelets are recruited and deposited at the surface of the vessel due in part to the margination effect, which refers to the increase in platelet concentration at the vessel periphery under high shear conditions. As well as promoting platelet concentration near the vessel wall, high shear also induces a conformational change in von Willebrand factor to expose more platelet binding sites and ‘trap’ platelets at the clot [Citation22]. Higher shear rates also promote activation of platelets leading to increased platelet adhesion [Citation21]. As a result, arterial clots have higher concentrations of platelets and significantly less fibrin concentrations than venous clots. Thus, depending on whether an artery or vein is injured, the resulting clot will have differing concentrations of fibrin and platelets. These results have primarily been observed in cases of thrombosis [Citation10], but may also be applicable in cases of external injuries to arteries and veins.
As more research is being conducted into the applications of blood clots in regenerative medicine, conditions affecting clot formation must be well understood in order to optimize their use as biomaterials conjugated with antibiotics, stem cells and other therapeutic agents.
Blood clot formation in vitro
Clot formation efficiency
Using blood clots as a vehicle for therapeutics is a recent development and there are many ways to modulate the formation and process of clot formation. Typical blood clot formation utilizes blood from a donor that then clots naturally in vitro, in combination with the desired added therapeutic agents. This process can be slow, and several agents have been investigated to make this process more efficient. For cases of blood clotting disorders or intentional formation of blood clots for therapeutic use, the addition of clotting related ions or proteins and molecules that act on the clotting cascade can enhance the blood clot formation process. Calcium, magnesium, fibrinogen, activated prothrombin complex concentrate and thrombin activatable fibrinolysis inhibitor (TAFI) have been approved treatments. Gessmann and colleagues demonstrated that autologous plasma clot formation with varying concentrations calcium affected the visual clot structure and the intra-clot mesenchymal stem cell (MSC) viability, with 5% calcium chloride by plasma volume allowing for the highest intra-clot cell proliferation [Citation23]. Calcium has been well studied and emphasized in relation to clotting, yet the role of magnesium in clotting has been observed but not well studied. Hence, Schumann et al. demonstrated that the addition of magnesium to a combination of Lactated Ringer’s solution with coagulation factor-containing blood products consistently formed blood clots without adding calcium [Citation24]. Shenkman and colleagues demonstrated that adding activated prothrombin complex concentrate and fibrinogen to blood can speed clot formation and enhance clot firmness in vitro. The strength of the blood clot formed can also be enhanced with the addition of TAFI, which inhibits fibrinolysis [Citation25]. In addition to adding agents to the clots, Perot et al. engineered modified Factor IX proteins, which enhanced clotting activity in vitro and in vivo and thrombin generating capacity in vivo when compared with wild type Factor IX protein in hemophilia B mice [Citation26]. Though shown to be effective in enhancing clot formation, modified Factor IX proteins warrant further studies to examine immunogenicity concerns. Despite several promising additive agents, improvement in the efficiency of clot formation remains an area that can be further explored.
Structural modifications
In addition to the speed of formation, blood clots formed in vitro have several other components that should be considered, including the properties and structure. To form autologous plasma clots, Gessmann and colleagues centrifuged blood from human volunteers and extracted only the plasma component [Citation27]. To modify the structure of these clots, Gessmann et al. utilized electric fields, magnetic fields, or orientated flow to alter the fibrin network in a particular alignment. The specific alignment of the fibrin network directed mesenchymal stem cell migration when cells were loaded onto the clots for longitudinal axonal regrowth for traumatic peripheral nerve injuries [Citation27]. In a following study, they were also able to load antibiotics and silver ions in plasma clots, which allowed for a graded release without cytotoxic effects [Citation28]. Hence autologous platelet-free plasma clots formed in vitro have been shown to be effective in harnessing therapeutic modalities including antibiotics and stem cells.
Whole blood clot formation
In addition to plasma clot formation, studies have emerged utilizing whole blood clots to harness the photosensitive properties that red blood cells contribute to the clot. Fan et al. utilized the thermotherapeutic and photosensitive property of whole blood clots to absorb radiation in the infrared range to aid in the blood clot delivery of cancer therapeutics. Mild hyperthermia from irradiation with light in the near infrared wavelength range has also been shown to enhance the healing potential for bones concurrently with the delivery of BMP-2 loaded onto the clot [Citation29]. Fan et al. formed their whole blood clots in a sterile mold that was held at room temperature and then transferred to a drying cabinet with phosphorus pentoxide at 37°C to ensure that serum and agents will not seep out of the clot. In contrast to whole blood clot formation, Zhu et al. conjugated red blood cells directly with photoactivatable molecular triggers, like 2-(1-hexyloxyethyl)-2-devinyl pyropheophorbide-α (HPPH), as well as various cancer therapeutics. These modified red blood cells had long-term stability in systemic circulation in vivo and were able to precisely release their therapeutic agents when irradiated at tumors [Citation30]. Whole blood clots have a wide range of therapeutic potential due to the integration of red blood cells and their native photosensitive properties.
Physiologic role of blood clots during wound healing of various tissue types
A deep understanding of blood clot structure is a critical prerequisite for determining how to properly apply them in the context of tissue regenerative and wound healing. The properties of a fibrin blood clot, namely porosity, malleability, elasticity and biodegradability determine the clot’s effectiveness in serving as a scaffold for tissue regeneration and optimization of cellular activity [Citation31]. Therefore, modification of blood clot structure can directly affect local cellular activity. Fibrin clots with thinner fibers and smaller pores are structurally more compact and are less permeable, hindering the diffusion of cytokines and cells [Citation32]. A more porous clot composed of thicker fibers may have better therapeutic efficacy given the involvement of various cellular and molecular mediators of tissue regeneration. For instance, Wang et al. demonstrated how modulation of clot structure can affect bone healing. IL-1β was shown to facilitate fibrin cross-linking, promote coagulation and increase clot fiber density in human blood, and this led to impaired and delayed healing of bone lesions [Citation33]. However, the addition of S-nitrosoglutathione contributed to the formation of clots with lower fiber density and promoted more rapid bone healing, corroborating the notion that clot structure is a critical determinant of tissue regeneration. Additionally, chronic disease states can affect clot structure, which consequently affects tissue regeneration. In patients with diabetes, blood clots have been shown to have thicker fibers, fewer pores, and higher fibrinogen concentration, all properties that contribute to the impaired wound healing in these patients [Citation34]. Erythrocytes also play a role in the clot structure and consequent function. Namely, these cells reduce clot permeability, decrease fibrin diameter, hinder the diffusion of cells and molecules, and limit the process of clot contraction, suggesting that a blood clot that is overly concentrated with erythrocytes is not optimal for wound healing [Citation35].
Autologous biomaterials
The formation of a hematoma is intrinsic to physiological wound healing and tissue regeneration, which is why blood clots have been investigated as a potential biomaterial to promote these processes. Hematoma formation that occurs as part of cartilage injury repair involves the release of many PDGFs as well as chemotactic molecules that promote cellular differentiation and proliferation; this is the rationale for microfracture surgery to promote healing of areas of cartilage that are poorly vascularized [Citation36]. Bone injury is another context in which hematoma formation is critical to promote angiogenesis, periosteal cell proliferation, and introduction of MSCs that can differentiate into various cellular components of bone [Citation37]. Considering the potential utility of peripheral blood clots in augmenting bone healing, it is important to note that peripheral blood clots contain approximately half as many granulocytes and monocytes with respect to lymphocytes as fracture hematomas, as well as more CD4+ and CD8+ T cells [Citation37]. The lack of granulocytes can limit infection prevention and extracellular matrix deposition, while greater amounts of helper T cells can promote excessive inflammation, which may damage tissues, demonstrating a potential limitation to using peripheral blood clots to augment bone healing.
In addition to therapeutic potential, infection prevention is another component of the utility of blood clots as a biomaterial in tissue regeneration. Fibrin cross-linking in blood clots is mediated by Factor XIII, and this aspect of blood clot structure contributes to the immobilization and reduced dissemination of bacteria in wounds [Citation38]. Macrae et al. demonstrated that a fibrin biofilm with a uniform structure covers the exterior surface of blood clots and greatly limits clot invasion of Escherichia coli, Staphylococcus epidermidis, and Staphylococcus aureus in vitro for 12–27 hours, and limits Pseudomonas aeruginosa proliferation in a murine dermal injury model for over 12 hours [Citation39]. Additionally, the adaptive immune system, namely regulatory T cells (Tregs) are crucial in optimizing tissue regeneration. For instance, it has been demonstrated that MSCs can induce the formation of Tregs, which limit excessive inflammatory actions by neutrophils and M1 macrophages and promote M2 macrophage differentiation, which leads to tissue reconstruction and regeneration [Citation40]. Additionally, the effects of Tregs in various tissues include enhancing satellite cell proliferation in muscles, promotion of epidermal growth factor receptor-mediated healing in cutaneous wounds, and inhibition of osteoclastogenesis in bones to prevent bone degradation [Citation40]. This demonstrates the importance of optimizing blood clot structure to allow for effective conjugation with cells, such as MSCs, and therapeutic molecules to promote tissue regeneration.
Paracrine release
Other autologous blood products have been used to generate clots with therapeutic potential. For instance, platelet-rich fibrin (PRF) clots may serve as a therapeutic biomaterial and improve bone morphogenetic protein (BMP) function when conjugated with VEGF, thus improving angiogenesis and tissue regeneration [Citation41]. However, peripheral blood clots may not require the addition of exogenous molecules to promote these healing processes, but a comparative analysis of the therapeutic efficacy of peripheral blood versus PRF clots is warranted to verify this. On the other hand, bone marrow aspirate clots contain MSCs and higher concentration of a variety of growth factors such as VEGF, TGF-β, BMP, and others than in peripheral blood clots, with the overall effects of limiting harmful inflammation [Citation42] and promoting regeneration when applied to injured tissues [Citation43] (see ). Considering the relative difficulty in obtaining bone marrow aspirate compared with peripheral blood to generate a clot to use as a therapeutic biomaterial, a peripheral blood clot appears to be a more practical biomaterial despite having less therapeutic potential than bone marrow aspirate. Therefore, conjugating blood clots with therapeutic molecules or cells are attractive options for improving tissue regeneration.
Table 1. Comparative effects of blood clots versus blood clots conjugated with mesenchymal stem cells.
Potential applications of blood clots to improve tissue healing in regenerative medicine
Orthobiologics are biologically derived materials and substances used to promote orthopaedic tissue regeneration and repair. Current orthobiologics include hyaluronic acid, PRP, bone marrow aspirate, bone grafts (autografts, allografts, graft substitutes), mesenchymal stem cells and growth factors (VEGF, BMP and PDGF) [Citation44,Citation45]. Many orthobiologics lack convincing evidence of efficacy, have immunogenicity concern for rejection, lack US FDA approval, or may not be covered by insurance, and are thus an expensive option for patients [Citation44]. A novel orthobiologic is blood clots, which can be used as a vehicle of delivery for medications, growth factors or stem cells, and as a scaffold or biomaterial. Blood clots are part of the natural healing process of many tissues and regulate immune cells to create the appropriate environment for tissue healing [Citation46]. As depicted in , clots can be prepared quickly, combined with therapeutic agents, and be applied to many different tissue types for a wide variety of applications. Due to their ease of preparation ex vivo, autologous origin, immunomodulatory effects that favor tissue repair, and malleable gel-like consistency, blood clots hold great potential as an orthobiologic, and more widely as a therapeutic biomaterial in regenerative medicine.
The strategy to use autologous blood clots in regenerative medicine, including: 1. blood preparation after being drawn from patients; 2. blood clot forms in vitro and can be conjugated with others, such as drugs, antibiotics, and stem cells; 3. use the conjugated blood clots to repair these injured tissues, such as skin wounds, soft tissues, bone defects, muscle, and tendon tears.
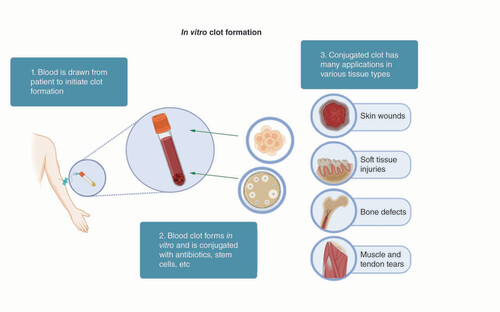
Blood clots as orthobiologic scaffolds
Blood clots are versatile as a biomaterial because they can be conjugated with many different therapeutic agents depending on desired function and target tissue. For example, blood clots have been used as a scaffold to deliver cancer vaccinations and chemotherapeutic agents and have been shown in this setting to modulate the immune cell environment to promote cancer cell destruction [Citation30,Citation46]. Additionally, the structure of the fibrin within a blood clot creates an environment that promotes the extended release of therapeutic agents. When combined with drug-eluting fibrin hydrogels, the antibiotic cefazolin has been found to have an extended release in vitro [Citation47]. Growth factors that promote angiogenesis and tissue repair, for example VEGF, FGF-2 and BMP2, have short half-lives and direct injection does not achieve adequate concentrations to impact tissue healing [Citation48]. This is an area of potential investigation as blood clots have the potential to provide extended release of these factors, and thus may achieve therapeutic concentrations that can improve tissue regeneration. Another therapeutic agent traditionally used in regenerative medicine includes stem cells, which can also be conjugated with blood clots to create an innovative delivery method. While few studies have combined stem cells with blood clots, a fibrin hydrogel scaffold has been shown to enable stable differentiation of adipose-derived mesenchymal stem cells and improved inflammation of neural tissue in vivo [Citation49]. Another immunocompatible scaffold made of acellular amniotic membrane, fibrin, and hyaluronic acid has also been used as a delivery mechanism for human-derived adipose stem cells in burn wound models [Citation50]. While several of these studies utilized fibrin hydrogels or similar biomaterials, blood clots also contain a fibrin network that has the potential to extend the release of therapeutic agents and should be further explored as a scaffold and delivery system.
The use of blood clots, rather than a fibrin gel or other similar material, showed that blood clots could be used as a scaffold to improve bone repair when combined with growth factors [Citation29]. Fan et al. formed a hydrogel by incorporating BMP-2 into drying fresh blood [Citation29]. They found this BMP-2-infused clot was able to be adjusted to fit the bone defect, and it successfully improved osteogenic differentiation and regeneration in vitro and in vivo. In addition to being a moldable, biocompatible vehicle of delivery for growth factors, they found the blood clot was also able to retain heat and act as a photothermal agent when irradiated with an 808 nm laser, which better promoted bone formation [Citation29]. Given the gel-like consistency of blood clots and their early preliminary success in regenerative medicine, the use of blood clots has the potential to enhance the healing of many different tissue types.
One example of blood clots (BC) currently being used as a regenerative agent is in the endodontics field, during an operation known as a regenerative endodontic procedure (REP) in which blood clots can be used as a scaffold to develop the immature necrotic tooth root into a mature root after pulp tissue damage [Citation51]. Autologous BC have been the early staple implant for endodontic patients for regenerative procedures [Citation52–55]. New developments in this regard are being compared with BC, focusing in on the platelet, plasma or fibrin components of the BC. Platelet-rich plasma (PRP) and platelet-rich fibrin (PRF) scaffold implants are among the more recent developments and have been shown to induce apical closure more frequently than BC for revascularization to regenerate immature permanent teeth [Citation56,Citation57] and revascularize necrotic teeth [Citation58]. When utilized in combination with hyaluronic acid derivatives, BC seem to improve outcomes of REP in non-infected, pulpless, immature teeth in a canine model [Citation59]. Clinically, outcomes of PRF seem to be comparable to BC and both adequately induce continued root development with regenerative endodontic treatment [Citation60]. However, the degree of healing in some cases may vary and the original pulp tissue may not be regenerated; It may be considered clinically successful if the entire length of the root canal space can either be filled with vital tissue with apical closure and continued root development, or the more coronal parts of the root canal may be at a standstill [Citation61].
Blood clots as wound dressings
Another potential application of blood clots as a biomaterial is their conjugation with antibiotics to create a wound covering that has the potential to both improve wound healing and prevent or eliminate infections of both clean and contaminated wounds and surgical incisions. Surgical site infections (SSIs) occur in 2–5% of surgeries and are a major cause of morbidity and mortality in USA [Citation62,Citation63]. Several techniques and products have been investigated and recommended for surgical site infection prevention, like perioperative antibiotic prophylaxis and antimicrobial coated sutures, while other techniques are more controversial, including negative pressure wound therapy, standard wound dressings, and incisional wound irrigation with antibiotics [Citation64]. Conversely, several techniques have also been explored to improve wound healing and hemostasis [Citation65], but few materials or techniques have been explored to achieve both infection prevention and improved wound healing. One current biomaterial under investigation is a biofilm-dispersing wound gel (BDWG); when placed over the full thickness skin wounds in mice it has been shown to prevent infection in clean wounds and improve wound healing on the microscopic level but showed no difference in the rate of wound healing [Citation66]. Fibrin hydrogels conjugated with cefazolin have also been explored as a method for postoperative extended antibiotic release and were shown to be effective in vitro [Citation47]. While still hypothetical, blood clots, when combined with antibiotics, could offer antimicrobial protection while also improving wound healing due to the presence of growth factors naturally abundant in blood as described previously [Citation48]. Due to the rapidity and ease of formation of blood clots ex vivo, the low financial cost, the autologous nature, and the potential to both improve SSI rate and wound healing, blood clots are a promising regenerative therapy for cutaneous wounds, surgical and otherwise.
Overall, blood clots hold great potential in regenerative medicine and are still in their early stages of investigation as a biomaterial. They have been successfully combined with several different therapeutic agents and show great promise for further investigation due to their intrinsic properties, natural composition of growth factors, fibrin structure and gel-like consistency.
Conclusion
Blood clots form naturally to achieve hemostasis upon injury and are the first step in the healing of many tissue types. While the physiologic formation of blood clots is well understood, their use in regenerative medicine is just beginning to be explored. Blood clots offer the gel-like consistency and drug-eluting properties similar to other scaffolds but have additional growth factors and immunomodulatory effects. Given the similarity to other scaffolds, the accessibility, the immunocompatibility, and the ease of formation and conjugation with other therapeutic agents, blood clots must be investigated further as a potential biomaterial for many tissue types in regenerative medicine.
Future perspective
The use of blood clots in regenerative medicine is a novel concept and is currently understudied. Several components of blood clots have been investigated, such as fibrin hydrogels or platelet rich plasma, but the whole blood clot has been overlooked. Over the next several years, we anticipate more work will be done to fully elucidate the role of blood clots as a simple, versatile tool to enhance wound healing and decrease infection rates in several different tissue types. First, studies to determine the most efficient way to develop therapeutic autologous blood clots at the patients’ bedside are needed to make blood clots a practical tool. Additional studies are also needed to determine the most appropriate properties of blood clots for each desired therapeutic indication. One major advantage of blood clots, however, is since they already contain the requisite molecules for healing, they can be tested in clinical trials while individual components are further studied. After the basic science has been thoroughly investigated, we expect studies using blood clots to deliver therapeutic agents to show improved wound healing and decreased infection rates in vitro and in vivo and then eventually expand to clinical studies. Overall, we anticipate blood clots will eventually provide patients an effective, inexpensive biologic option that will improve wound healing and decrease infection rates in many different tissue types.
Blood clot formation & structure
Primary hemostasis
The purpose of primary hemostasis is to generate a platelet aggregate that plugs the site of tissue injury, prevents blood loss, and provides a surface for activation of coagulation factors.
Secondary hemostasis
The purpose of secondary hemostasis is to create a cross-linked fibrin structure that stabilizes the platelet aggregates formed in primary hemostasis.
Complete clot formation necessitates stabilization by fibrin deposition, and cellular components such as erythrocytes and leukocytes can be incorporated into the structure as well.
Factors affecting clot structure & stability
Biological factors
The concentration of thrombin determines fibrin thickness and density within a blood clot, which ultimately determines the clots stability and resistance to fibrinolysis.
Fibrin thickness is variable within blood clots.
Physiological factors
The physiological context within which blood clots form, such as during trauma and subsequent acidotic states, must be considered when analyzing clot structure.
Blood pH affects clot strength and structure by influencing coagulation dynamics and fibrin fiber thickness.
Blood factors
Shear rate and blood flow mechanics impact clot formation and platelet activity and fibrin concentration.
Blood clot formation in vitro
Clot formation efficiency
Various additives can improve the efficiency of in vitro formation of blood clots and enhance their physical properties and therapeutic potential.
Structural modifications
Clot structure can be modified to regulate fibrin orientation, which can direct cellular trafficking and be beneficial for localized tissue healing.
Whole blood clot formation
Whole blood clots have photosensitive properties that allow absorption of radiation and are conducive to delivery of cancer-treating substances and other molecular healing factors, demonstrating their therapeutic potential.
Physiologic role of blood clots during wound healing of various tissue types
Autologous biomaterials
Peripheral blood clots can promote tissue healing and serve as a vehicle to deliver therapeutics such as MSCs.
A fibrin biofilm that covers blood clots contributes to infection prevention at the site of tissue healing.
Paracrine release
Various autologous blood products have been developed as therapeutics, supporting the potential utility of peripheral blood clots, which are more practical to generate.
Potential applications of blood clots to improve tissue healing in regenerative medicine
Blood clots as orthobiologic scaffolds
Blood clots can serve as a scaffold that allows for local delivery and extended release of therapeutics such as chemotherapy, antibiotics and growth factors.
Blood clots as wound dressings
Conjugation of blood clots with antibiotics is being explored as a rationale for developing novel methods to prevent wound infection.
Author contributions
E VanZweden: drafted the manuscript and created figures, R Tolsma: drafted the manuscript and created figures, V Hung: drafted the manuscript and created figures, P Awad: drafted the manuscript and created figures, R Sawyer: discussed and edited the manuscript, Y Li: supervised, structured, drafted and editing of the manuscript.
All authors approve of the final version of the manuscript.
Financial & competing interests disclosure
This review is partially supported by a pioneer grant from the Western Michigan University Homer Stryker MD School of Medicine. The authors have no other relevant affiliations or financial involvement with any organization or entity with a financial interest in or financial conflict with the subject matter or materials discussed in the manuscript apart from those disclosed.
No writing assistance was utilized in the production of this manuscript.
Additional information
Funding
References
- YauJW , TeohH , VermaS. Endothelial cell control of thrombosis. BMC Cardiovasc. Disord.15(1), 130–141 (2015).
- GolebiewskaEM , PooleAW. Platelet secretion: from haemostasis to wound healing and beyond. Blood Rev.29(3), 153–162 (2015).
- BrassLF. Thrombin and platelet activation. Chest124(Suppl. 3), S18–S25 (2003).
- GaleAJ. Continuing Education Course #2: current understanding of hemostasis. Toxicol. Pathol.39(1), 273–280 (2011).
- PaltaS , SaroaR , PaltaA. Overview of the coagulation system. Indian J. Anaesth.58(5), 515–523 (2014).
- ChaudhryR , UsamaSM , BabikerHM. Physiology, coagulation pathways. Treasure Island (FL): StatPearl Publishing NBK482253 (2022).
- RijkenDC , Uittede Willige S. Inhibition of fibrinolysis by coagulation factor XIII. BioMed Res. Int.2017, 1209676 (2017).
- AriensRAS. Contribution of red blood cells and clot structure to thrombosis. Blood126(23), SCI-15-SCI-15 (2015).
- WolbergAS , CampbellRA. Thrombin generation, fibrin clot formation and hemostasis. Transfus. Apher. Sci.38(1), 15–23 (2008).
- CasaLDC , DeatonDH , KuDN. Role of high shear rate in thrombosis. J. Vasc. Surg.61(4), 1068–1080 (2015).
- WolbergAS. Thrombin generation and fibrin clot structure. Blood Rev.21(3), 131–142 (2007).
- ZengZ , FagnonM , NallanChakravarthula T , AlvesNJ. Fibrin clot formation under diverse clotting conditions: comparing turbidimetry and thromboelastography. Thromb. Res.187, 48–55 (2020).
- LakshmananRS , EfremovV , O’DonnellJS , KillardAJ. Measurement of the viscoelastic properties of blood plasma clot formation in response to tissue factor concentration-dependent activation. Anal. Bioanal. Chem.408(24), 6581 (2016).
- HuangCC , ShihCC , LiuTY , LeePY. Assessing the viscoelastic properties of thrombus using a solid-sphere-based instantaneous force approach. Ultrasound Med. Biol.37, 1722–1733 (2011).
- GhezelbashF , LiuS , Shirazi-AdlA , LiJ. Blood clot behaves as a poro-visco-elastic material. J. Mech. Behav. Biomed. Mater.128, 105101 (2022).
- MooreEE , MooreHB , KornblithLZet al.Trauma-induced coagulopathy. Nat. Rev. Dis. Primers7(1), 30–43 (2021).
- GisselM , Brummel-ZiedinsKE , ButenasS , PusateriAE , MannKG , OrfeoT. Effects of an acidic environment on coagulation dynamics. J. Thromb. Haemost.14(10), 2001–2010 (2016).
- FereidoonnezhadB , DwivediA , JohnsonS , McCarthyR , McGarryP. Blood clot fracture properties are dependent on red blood cell and fibrin content. Acta Biomater.127, 213–228 (2021).
- LiuS , BaoG , MaZ , KastrupCJ , LiJ. Fracture mechanics of blood clots: measurements of toughness and critical length scales. Extreme Mech. Lett.48, 101444 (2021).
- CattaneoM , LecchiA , ZighettiML , LussanaF. Platelet aggregation studies: autologous platelet-poor plasma inhibits platelet aggregation when added to platelet-rich plasma to normalize platelet count. Haematologica92(5), 694–697 (2007).
- SakariassenKS , OrningL , TurittoVT. The impact of blood shear rate on arterial thrombus formation. Future Sci. OA1(4), FSO30 (2015).
- FogelsonAL , NeevesKB. Fluid mechanics of blood clot formation. Annu. Rev. Fluid Mech.47, 377–403 (2015).
- GessmannJ , SeyboldD , PeterE , SchildhauerTA , KöllerM. Plasma clots gelled by different amounts of calcium for stem cell delivery. Langenbeck’s Arch. Surg.398(1), 161–167 (2013).
- SchumannR , ZaimiI , ShebacloK , GuptaA. Blood products, crystalloids, and rapid infusion: an experimental study with magnesium. J. Cardiothorac. Vasc. Anesth.36(4), 1040–1046 (2022).
- ShenkmanB , EinavY , LivnatT , BudnikI , MartinowitzU. Rotation thromboelastometry analysis of clot formation and fibrinolysis in severe thrombocytopenia: effect of fibrinogen, activated prothrombin complex concentrate, and thrombin- activatable fibrinolysis inhibitor. Int. J. Lab. Hematol.37(4), 521–529 (2015).
- PerotE , EnjolrasN , le QuellecSet al.Expression and characterization of a novel human recombinant factor IX molecule with enhanced in vitro and in vivo clotting activity. Thromb. Res.135(5), 1017–1024 (2015).
- GessmannJ , SeyboldD , PeterE , SchildhauerTA , KöllerM. Alignment of the fibrin network within an autologous plasma clot. Tissue Eng. Part C Methods22(1), 30–37 (2016).
- GessmannJ , SeyboldD , AyamiFet al.Peripheral blood plasma clot as a local antimicrobial drug delivery matrix. Tissue Eng. Part A24(9–10), 809–818 (2018).
- FanQ , BaiJ , ShanHet al.Implantable blood clot loaded with BMP-2 for regulation of osteoimmunology and enhancement of bone repair. Bioact. Mater.6(11), 4014–4026 (2021).
- ZhuYX , JiaHR , GuoYet al.Repurposing erythrocytes as a “photoactivatable bomb”: a general strategy for site-specific drug release in blood vessels. Small17(34), 2100753 (2021).
- WeiselJW , LitvinovRI. Fibrin formation, structure and properties. Subcell. Biochem.82, 405–456 (2017).
- MihalkoE , BrownAC. Clot structure and implications for bleeding and thrombosis. Semin. Thromb. Hemost.46(1), 96–97 (2020).
- WangX , FriisTE , MasciPP , CrawfordRW , LiaoW , XiaoY. Alteration of blood clot structures by interleukin-1 beta in association with bone defects healing. Sci. Rep.6, 35645–35656 (2016).
- de VriesJJ , HoppenbrouwersT , Martinez-TorresCet al.Effects of diabetes mellitus on fibrin clot structure and mechanics in a model of acute neutrophil extracellular traps (Nets) formation. Int. J. Mol. Sci.21(19), 1–15 (2020).
- WeiselJW , LitvinovRI. Red blood cells: the forgotten player in hemostasis and thrombosis. J. Thromb. Haemost.17(2), 271–282 (2019).
- ZadehLG , ChevrierA , FarrJ , RodeoSA , BuschmannMD. Augmentation techniques for meniscus repair. J. Knee Surg.31(1), 99–116 (2018).
- KolarP , Schmidt-BleekK , SchellHet al.The early fracture hematoma and its potential role in fracture healing. Tissue Eng. Part B Rev.16(4), 427–434 (2010).
- AntoniakS. The coagulation system in host defense. Res. Pract. Thromb. Haemost.2(3), 549–557 (2018).
- MacraeFL , DuvalC , PapareddyPet al.A fibrin biofilm covers blood clots and protects from microbial invasion. J. Clin. Invest.128(8), 3356–3368 (2018).
- LiJ , TanJ , MartinoMM , LuiKO. Regulatory T cells: potential regulator of tissue repair and regeneration. Front. Immunol.9, 585–596 (2018).
- MironRJ , ZhangY. Autologous liquid platelet rich fibrin: a novel drug delivery system. Acta Biomaterialia75, 35–51 (2018).
- QiK , LiN , ZhangZ , MelinoG. Tissue regeneration: the crosstalk between mesenchymal stem cells and immune response. Cell. Immunol.326, 86–93 (2018).
- LanaJF , da FonsecaLF , AzziniGet al.Bone marrow aspirate matrix: a convenient ally in regenerative medicine. Int. J. Molec. Sci.22(5), 1–12 (2021).
- CalceiJG , RodeoSA. Orthobiologics for bone healing. Clin. Sports Med.38(1), 79–95 (2019).
- KruelAVS , RibeiroLL , GusmãoPD , HuberSC , LanaJFSD. Orthobiologics in the treatment of hip disorders. World J. Stem Cells13(4), 304–316 (2021).
- FanQ , MaQ , BaiJet al.An implantable blood clot-based immune niche for enhanced cancer vaccination. Sci. Adv.6(39), eabb4639 (2020).
- TredwellS , JacksonJK , HamiltonD , LeeV , BurtHM. Use of fibrin sealants for the localized, controlled release of cefazolin. Can. J. Surg.49(5), 347–352 (2006).
- CalabrisoN , StancaE , RochiraAet al.Angiogenic properties of concentrated growth factors (CGFs): the role of soluble factors and cellular components. Pharmaceutics13(5), (2021).
- ChandrababuK , SreelathaHV , SudhadeviT , AnilA , ArumugamS , KrishnanLK. In vivo neural tissue engineering using adipose-derived mesenchymal stem cells and fibrin matrix. J. Spinal Cord Med.1, 1–15 (2021).
- RamakrishnanR , SreelathaHV , AnilAet al.Human-derived scaffold components and stem cells creating immunocompatible dermal tissue ensuing regulated nonfibrotic cellular phenotypes. ACS Biomat. Sci. Eng.6(5), 2740–2756 (2020).
- LeeBN , MoonJW , ChangHS , HwangIN , OhWM , HwangYC. A review of the regenerative endodontic treatment procedure. Restor. Dent. Endod.40(3), 179 (2015).
- ÖstbyBN. The role of the blood clot in endodontic therapy an experimental histologic study. Acta Odontol. Scand.19(3–4), 324–353 (1961).
- BanchsF , TropeM. Revascularization of immature permanent teeth with apical periodontitis: new treatment protocol?J. Endod.30(4), 196–200 (2004).
- ReynoldsK , JohnsonJD , CohencaN. Pulp revascularization of necrotic bilateral bicuspids using a modified novel technique to eliminate potential coronal discolouration: a case report. Int. Endod. J.42(1), 84–92 (2009).
- LovelaceTW , HenryMA , HargreavesKM , DiogenesA. Evaluation of the delivery of mesenchymal stem cells into the root canal space of necrotic immature teeth after clinical regenerative endodontic procedure. J. Endod.37(2), 133–138 (2011).
- UlusoyAT , TurediI , CimenM , CehreliZC. Evaluation of blood clot, platelet-rich plasma, platelet-rich fibrin, and platelet pellet as scaffolds in regenerative endodontic treatment: a prospective randomized trial. J. Endod.45(5), 560–566 (2019).
- MurrayPE. Platelet-rich plasma and platelet-rich fibrin can induce apical closure more frequently than blood-clot revascularization for the regeneration of immature permanent teeth: a meta-analysis of clinical efficacy. Front. Bioeng. Biotechnol.6(OCT), 139 (2018).
- RizkHM , SalahAl-Deen MS , EmamAA. Pulp revascularization/revitalization of bilateral upper necrotic immature permanent central incisors with blood clot vs platelet-rich fibrin scaffolds—a split-mouth double-blind randomized controlled trial. Int. J. Clin. Pediatr. Dent.13(4), 337 (2020).
- AlHowaishNA , AlSudaniDI , KhounganianR , AlMuraikhiN. Histological evaluation of restylane lyft used as a scaffold for dental pulp regeneration in non-infected immature teeth in dogs. Materials (Basel)15(12), 4095 (2022).
- LvH , ChenY , CaiZet al.The efficacy of platelet-rich fibrin as a scaffold in regenerative endodontic treatment: a retrospective controlled cohort study. BMC Oral Health18(1), 1–8 (2018).
- FarhadAR , ShokranehA , ShekarchizadeN. Regeneration or replacement? A case report and review of literature. Dent. Traumatol.32(1), 71–79 (2016).
- KorolE , JohnstonK , WaserNet al.A systematic review of risk factors associated with surgical site infections among surgical patients. PLOS ONE8(12), 83743 (2013).
- AvsarP , PattonD , OuseyK , BlackburnJ , O’ConnorT , MooreZ. The impact of surgical site infection on health-related quality of life: a systematic review. Wound Manag. Prev.67(6), 10–19 (2021).
- Allegranzib , ZayedB , BischoffPet al.New WHO recommendations on intraoperative and postoperative measures for surgical site infection prevention: an evidence-based global perspective. The Lancet Infect. Dis.16(12), e288–e303 (2016).
- ZhongY , HuH , MinN , WeiY , LiX , LiX. Application and outlook of topical hemostatic materials: a narrative review. Ann. Transl. Med.9(7), 577–577 (2021).
- BoundsK , Colmer-HamoodJA , MynttiM , JeterRM , HamoodAN. The influence of a biofilm-dispersing wound gel on the wound healing process. Int. Wound J.19(3), 553–572 (2021).