Abstract
Conclusion: The antero-posterior (AP) body tracking test (BTT) showed that the dominant foot could affect the tilt angle of the sway movement, delineated by primary component analysis. Differences associated with the dominant foot could represent the difference in space perception of each person. Objectives: To examine whether the dominant foot could affect the postural control mechanism using the BTT. Methods: Ninety-seven healthy participants enrolled in the study were classified into right-foot and left-foot dominance groups, and their performances were compared. For the BTT, each participant stood on a stabilometer and caught the movement of a visual target moving vertically (anterior-posterior) or horizontally by the center of pressure movement, displayed on a 14-inch screen monitor at 100 cm in front of the subject. The mean displacement angle of the obtained stabilogram was evaluated by principal component analysis. Results: The AP BTT in the right-foot dominance group showed a clockwise tilt with a mean displacement angle of 3.022 ± 3.761°, whereas the group with left-foot dominance had a modest counter-clockwise tilt with a mean displacement angle of –0.694 ± 4.497°. This difference was found to be significant by the independent t test (p < 0.0001). In the lateral BTT, the mean displacement angles were not significant.
Introduction
The righting reflex test is a fundamental test to examine postural control function. Both dynamic and static body balance function tests have been introduced for the clinical evaluation of body balance function. Dynamic body balance tests include Fukuda’s stepping test, the vertical writing test with closed eyes, walking test, and tandem gait test, among others. These dynamic body balance function tests are used in the clinical setting to primarily evaluate the deviation phenomenon and the changes in the distribution of foot pressure during the stance period while walking [Citation1,2].
Stabilometry is used to examine the static postural reflex. There are also reports of stabilometry used in conjunction with the visual stimulation system such as that involving a fixed target and/or a vibrating target at 1/3 Hz [Citation3], a visual target moving at 0.1 or 0.01 Hz to the right and left along with antero-posterior (AP) movements [Citation4], the use of different visual target sizes, and a stimulus using a Nintendo Wii Balance Board (Nintendo, Kyoto, Japan) [Citation5].
Furthermore, there are reports on body balance function testing that involved placing subjects in a tunnel of virtual reality that oscillates in an AP fashion [Citation6], as well as the use of a modified EquiTest® with a dynamic posturography apparatus that uses sudden AP movements on a supporting surface [Citation7].
In addition, some studies have examined walking using vibrotactile feedback of body tilt [Citation8], whereas some studies have investigated the effects of visual deprivation on the center of foot pressure (COP) sway while stepping with eyes closed [Citation9]. Rhythmic weight-shift evaluation using the static posturography test could also be useful in discriminating between normal and pathological subjects [Citation10]. However, the postural balance function during the movement of a visual target that is followed by COP sway has not been studied thus far.
This knowledge will be useful to evaluate overall postural control function, using visual tracking in conjunction with the spinal postural control system because this function is more frequently used during daily social living. Therefore, we developed a new method to assess dynamic body balance, termed the body tracking test (BTT). With this equipment, while the subject is tracking the movement of a visual target that moves on a screen at a constant speed in the AP (vertical movement; AP BTT) or lateral direction (horizontal movement; lateral BTT), the COP is recorded and analyzed in detail. In a previous study, to confirm the manner in which the subject adjusted the COP while tracking a visual target, principal component analysis was employed. As a result, it was determined that the tracking axis of the AP BTT was not parallel to the Y-axis [Citation11]. We thought that this result could be influenced by the dominant foot of the subjects to some extent. Accordingly, the present study was conducted to clarify some changes in the postural control mechanism in relation to the dominant foot.
Material and methods
Participants
The participants were 97 healthy subjects without a history of vertigo or balance disorders. Among them, 64 participants were right-foot dominant and 33 were left-foot dominant. The dominant foot was established by interviewing the subjects to determine which side was used to kick a ball [Citation12]. The age of the participants ranged from 20 to 40 years with an average age of 31.8 years. In particular, the average age (mean ± SD) of the right-foot and left-foot dominance groups was 30.90 ± 7.5 years and 33.5 ± 7.4 years, respectively.
In accordance with the Declaration of Helsinki, the experimental procedures were explained to the participants (volunteers), and each patient provided written informed consent before study participation. The experimental procedure was approved by the local ethics committee.
Evaluation
The BTT equipment, as shown in , was composed of a stabilometer (Anima-G620, Anima Co., Tokyo, Japan) and a visual stimulus display. Although various recording conditions can be set for performing a detailed examination, we only applied the BTT to evaluate tracking function in the AP and lateral directions in the present study.
The monitor screens for the AP BTT and lateral BTT are shown in . The width of the moving stimulus was set at 15 cm for both the AP BTT and the lateral BTT, and it was presented on a 14-inch display screen. On the monitor screen, the moving visual stimulus was shown in green, whereas the position of the subject’s COP was shown in red. The moving visual stimulus and the subject’s COP appeared side-by-side on the display, as shown in . Each subject had to move his/her COP according to the movement of the target, and the position of each movement was assessed. An upward movement on the AP BTT display reflected a forward direction of the COP, whereas a downward movement reflected a posterior movement. In the lateral BTT, right and left movements on the display reflected the same corresponding movements of the COP.
Figure 2. Monitor screen for the body tracking test (BTT) system. The moving visual target is shown in green and the subject’s center of pressure (tracking graphic position) is shown in red. AP, antero-posterior.
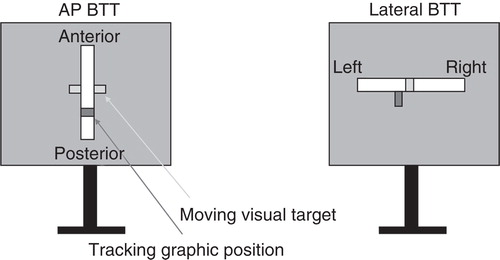
Visual stimulation was given at a constant speed of 0.125 Hz, which was determined by previous experimental results. This stimulus speed was selected to avoid making a rhythmic movement of the subject’s COP. The analog/digital (A/D) converter recorded samples every 50 ms (20 Hz) and the AP BTT and lateral BTT were performed for 60 s, respectively. The distance between the target and the subject was set at 100 cm, and subjects stood with their feet close together and in parallel, while maintaining an upright posture. The height of the target on the monitor screen was set at the subject’s eye level and the subject was allowed to wear glasses or contact lenses, if required.
These test conditions are those recommended by the Japan Society for Equilibrium Research. Based on a previous study, the gain of the COP movement displayed on the screen was two times greater than the actual COP movement value. This gain was set to avoid discomfort for the subject after repeated testing.
Subjects were instructed to set their COP at the center of the coordinate, maintain foot position, and track the target with an upright posture. The actual recording started 7 s after the exercise began.
Analysis
Evaluation of tilt angles
In the AP BTT and lateral BTT, the tracking of the visual stimulus for movement was displayed on a straight line, and the subject’s COP tracked the location of the target.
The two-dimensional coordinates of the COP (X-axis, lateral direction; Y-axis, AP direction) sampled in each experimental session were analyzed by principal component analysis. The main axis of the tracking performance was evaluated as an eigenvector corresponding to the first principal component. In the AP BTT, the subject’s tilt during the task was expressed as a displacement angle of the main axis from the Y-axis, with clockwise tilt considered as positive and a counter-clockwise tilt considered as negative. In the lateral BTT, the subject’s tilt during the task was expressed as a displacement angle of the main axis from the X-axis, with clockwise tilt considered as positive and counter-clockwise considered as negative ().
Figure 3. Representative schemata of center of pressure movement obtained by the antero-posterior (AP) body tracking test (BTT) (a) and lateral BTT (b) in a case with right-foot dominance. The tilt angle calculated by principal component analysis in each figure is indicated by a grey line. In this case, clockwise tilt is shown in both AP BTT and lateral BTT.
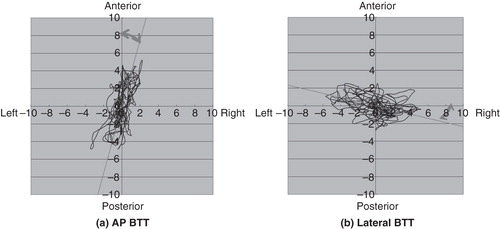
Statistical analysis
Statistical comparisons were conducted using the independent t test. A p value of < 0.05 was considered statistically significant.
Results
The AP BTT in the right-foot dominance group presented a clockwise tilt with a mean displacement angle of 3.022° ± 3.761°. In contrast, the group with left-foot dominance presented a somewhat mild counter-clockwise tilt, with a mean displacement angle of –0.694° ± 4.497° (). This difference was found to be statistically significant according to the independent t test (p < 0.0001). For the lateral BTT, mean displacement angles of 0.483° ± 4.819° and –0.703° ± 5.891° were observed in the right-foot and left-foot dominance groups, although the difference was not significant ().
Discussion
Few studies have investigated the methods for evaluating dynamic body balance function by using moving visual stimuli and COP assessment. Recent reports have described the utilization of computerized dynamic posturography, using a visual stimulus, to evaluate body balance function [Citation13].
Kobori and Yoneda [Citation14] described a test to evaluate the movement of COP to the left or right in accordance with target movement. However, this test is not useful for motion tracking, because it only measures stepping movements. To our knowledge, no report has described the use of a moving visual stimulus for the evaluation of COP sway, such as the one performed in the present BTT study.
The subject’s COP tracking function of the moving visual stimulus is important for the evaluation of dynamic balance. The ability to maintain postural balance while staring at a moving target is usually performed, consciously or unconsciously, during activities of daily living. For the practical use of the BTT, it is essential to clearly understand how visual tracking function is controlled by COP in healthy subjects in relation to individual variation.
In the present BTT study, the visual input comprising the visual target and COP was presented on the same screen. These data were integrated in the cerebrum, especially in the posterior lobe, to maintain postural balance through the actions of the cerebellum and brainstem, with the spinal ascending feedback system. In addition, subjects could track the movement of the visual target with COP under the most stable and tractable conditions. Because the movements of the visual target were only displayed on X- or Y-axes, (i.e. purely vertical and horizontal planes), the subjects could not recognize the tilt of their own movement axis.
Despite individual variations among subjects, we initially considered that the tilt of COP movement occurs in parallel with the X- and Y-axes. However, in a foregoing experiment, it was shown that the AP BTT in healthy subjects had a clockwise tilt [Citation11].
Conversely, Boonstra et al. reported that no significant change was shown on static postural function between both feet [Citation15]. However, it has generally been recognized that the dominant hand or foot could affect the postural compensation process after vestibular neuronitis; in addition, it could affect the final outcome after gonarthrosis surgery [Citation16].
Therefore, it should be clarified whether or not the dominant foot could influence the postural control mechanism as well. Accordingly, we performed the present study by dividing the participants into left-foot and right-foot dominance groups. We noted that there is a different postural control mechanism in accordance with the dominant foot. Habitual postural movements using the dominant foot might significantly be associated with developing those different postural mechanisms. In addition, it could also be inferred that spatial perception using the dominant foot might influence this phenomenon, and that the function of the posterior parietal cortex – involved in the spatial perception process to coordinate axial displacement using positional information – may play an important role in this regard [Citation17,18].
To maintain balance, two major strategies are recognized. One is the hip strategy, which is related to robust control. The other is the ankle strategy, which is used for delicate postural control [Citation15]. During BTT, the ankle strategy could be used mainly for maintenance of a stable posture, especially in AP BTT, which is affected by the dominant foot during fine arrangement of postural control. In other words, postural control in accordance with the movement of the visual target, especially in a vertical plane, will be influenced by differences in spatial perception based on the differences in the dominant foot. This difference could lead to control of the anterior displacement of COP by the dominant foot and posterior displacement of COP by the other non-dominant foot. This may be a reason why the tilt angle is observed in AP BTT. With regard to lateral BTT, these differences may be minimal. It has been reported that footedness effects could be found significantly under moving conditions, such as single-foot circular tracking task, rather than in stable conditions [Citation19]. However, it was shown in the present study that the dominant foot effect could be hindered by the direction of the moving target in lateral BTT.
De Agonostini and Dellatolas [Citation20] showed that the dominant foot, hand, and eye had no significant effect on cognitive performance as determined by the use of the computerized version of the Peg-Moving Task proposed by Annett. Because the postural control along with space orientation are attained during learning processes while an individual grows older, the following points should be clarified in the future: (1) whether or not the tilt of the main axis of the AP BTT is produced in accordance with physical growth, and (2) whether or not the main axis is influenced by aging. Additionally, further understanding of the postural mechanism of the dominant foot in pathological cases might influence the modality of balance rehabilitation.
In conclusion, we analyzed the main axis by using the first principal component in the BTT, which is a dynamic body balance test. The performance was then compared by grouping the subjects according to their dominant foot. Significant differences between the dominant feet were found in AP BTT but not in lateral BTT.
Declaration of interest: The authors report no conflicts of interest. The authors alone are responsible for the content and writing of the paper.
References
- Angunsri N, Ishikawa K, Yin M, Omi E, Shibata Y, Saito T, et al. Gait instability caused by vestibular disorders – analysis by tactile sensor. Auris Nasus Larynx 2011;38:462–8.
- Ishikawa K, Wang Y, Wong WH, Shibata Y, Itasaka Y. Gait instability in patients with acoustic neuroma. Acta Otolaryngol 2004;124:486–9.
- Laurens J, Awai L, Bockisch CJ, Hegemann S, van Hedel HJ, Dietz V, et al. Visual contribution to postural stability: interaction between target fixation or tracking and static or dynamic large-field stimulus. Gait Posture 2010;31:37–41.
- Bobrova EV, Kucher VI, Levik IuS, Bogacheva IN. [Nonlinear analysis of the dynamics of the human balance control system during fixation and smooth pursuit of visual target]. Biofizika 2007;52:355–61; in Russian.
- Koslucher F, Wade MG, Nelson B, Lim K, Chen FC, Stoffregen TA. Nintendo Wii Balance Board is sensitive to effects of visual tasks on standing sway in healthy elderly adults. Gait Posture 2012;36:605–8.
- Greffou S, Bertone A, Hanssens JM, Faubert J. Development of visually driven postural reactivity: a fully immersive virtual reality study. J Vis 2008;8:15.1–10.
- Ledin T, Odkvist LM. Visual influence on postural reactions to sudden antero-posterior support surface movements. Acta Otolaryngol 1991;111:813–19.
- Wall C 3rd, Wrisley DM, Statler KD. Vibrotactile tilt feedback improves dynamic gait index: a fall risk indicator in older adults. Gait Posture 2009;30:16–21.
- Aoki H, Demura S, Kawabata H, Sugiura H, Uchida Y, Xu N, et al. Evaluating the effects of open/closed eyes and age-related differences on center of foot pressure sway during stepping at a set tempo. Adv Aging Res 2012;1:72–7.
- Balaguer García R, Pitarch Corresa S, Baydal Bertomeu JM, Morales Suárez-Varelaca MM. Static posturography with dynamic tests. Usefulness of biomechanical parameters in assessing vestibular patients. Acta Otorhinolaryngol Esp 2012;63:332–8.
- Yoshida T, Ikemiyagi F, Ikemiyagi Y, Tnaka T, Takanami T, Tamura Y, et al. The tracking axis of the body tracking test. Nihon Jibiinkoka Gakkai Kaiho 2013;116:1308–13.
- Schneiders AG, Sullivan SJ, O’Malley KJ, Clarke SV, Knappstein SA, Taylor LJ. A valid and reliable clinical determination of footedness. PM R 2010;2:835–41.
- Black FO. Clinical status of computerized dynamic posturography in neurology. Curr Opin Otolaryngol Head Neck Surg 2001;9:314–18.
- Kobori S, Yoneda T. Analysis of tracking motion in standing posture using multiple regression analysis method. IEICE Trans 1990;E73:1824–6.
- Boonstra TA, Schouten AC, van der Kooij H. Identification of the contribution of the ankle and hip joints to multi-segmental balance control. J Neuroeng Rehabil 2013;10:23.
- Yoshida T. The view of a right-handed and a left-handed person. Equilibrium Res 2010;69:147–50.
- Buneo CA, Andersen RA. Integration of target and hand position signals in the posterior parietal cortex: effects of workspace and hand vision. J Neurophysiol 2012;108:187–99.
- Vesia M, Yan X, Henriques DY, Sergio LE, Crawford JD. Transcranial magnetic stimulation over human dorsal-lateral posterior parietal cortex disrupts integration of hand position signals into the reach plan. J Neurophysiol 2008;100:2005–14.
- Wang Z1, Newell KM. Footedness exploited as a function of postural task asymmetry. Laterality 2013;18:303–18.
- De Agostini M, Dellatolas G. Lateralities in normal children ages 3 to 8 and their role in cognitive performances. Dev Neuropsychol 2001;20:429–44.