Abstract
Prominent amongst the non-classical effects of vitamin D is its interaction with the immune system. Although this has been recognized for many years, it is only through recent studies that we have been able to fully understand the impact of vitamin D on normal innate and adaptive immune function. In particular these studies have illustrated how impaired vitamin D status has important ramifications for dysregulated immune responses to infection and aberrant inflammatory responses associated with autoimmune disease. Indeed it seems likely that the effects of vitamin D will extend beyond these established immune diseases to include additional novel effects, such as interaction with the enteric gut microbiota. Central to this new perspective on vitamin D and immunity has been the elucidation of pivotal mechanisms that underpin the interface between vitamin D and target immune cells. In particular, it is now clear that effects of vitamin D on monocytes, macrophages, dendritic cells, and lymphocytes are not constrained by the metabolic pathways associated with classical endocrine actions of vitamin D. Instead, it is now important to also consider intracrine and paracrine pathways that are subject to a distinct set of modulatory signals, and which may also be influenced by disease-specific dysregulation. The current review will discuss this by comparing the intracrine, paracrine and endocrine metabolic systems that influence the interaction between vitamin D and the immune system.
Introduction
In the last five years there has been a renaissance in vitamin D research, fuelled in large part by a new perspective on vitamin D physiology that has extended our understanding of vitamin D far beyond its established effects on mineral homeostasis and bone metabolism. Prominent amongst these newly proposed functions is the interaction between vitamin D and the immune system, including activities on both innate and adaptive immune responses to infection. Although this is commonly considered to be a new facet of vitamin D physiology, the link between vitamin D and immune function actually stretches back over more than a century to studies that explored the health benefits of light irradiation. In 1903 Niels Finsen received the Nobel Prize for Medicine after demonstrating that exposure to light from an electric arc light could cure Lupus Vulgaris (the epidermal form of tuberculosis,TB) (reviewed in [Citation1]). Cod liver oil, a rich source of dietary vitamin D has also been used as a treatment for TB [Citation2], and one of the earliest recorded non-skeletal applications for vitamin D itself was in the treatment of another disease involving mycobacterial infection, Leprosy [Citation3]. More recent studies have highlighted a link between vitamin D-insufficiency (serum concentrations of 25-hydroxyvitamin D (25(OH)D – the sum of 25(OH)D2 and 25(OH)D3) < 75 nmol/L) and TB risk [Citation4–7]. Several clinical trials of vitamin supplementation have also been reported with varying success [Citation7–9]. The most recent supplementation study was successful in raising serum concentrations of 25(OH)D in TB patients, but showed no overall difference in disease phenotype between treatment and placebo groups [Citation10]. However, the authors did show a significant improvement in a specific subset of TB patients with a Taq1 single nucleotide polymorphism (SNP) within the gene for the receptor for active vitamin D (1,25-dihydroxyvitamin D3 (1,25(OH)2D3 – the sum of 1,25(OH)2D2 and 1,25(OH)2D3)), the vitamin D receptor (VDR) [Citation10]. Thus inherited factors may influence responses to vitamin D supplementation and this facet of vitamin D physiology.
The link between vitamin D and infection is unlikely to be restricted to mycobaterial diseases such as TB and Leprosy. Serum concentrations of 25(OH)D have been shown to correlate with circulating levels of antibacterial proteins and increased risk of critical illness in patients with sepsis [Citation11]. In a similar fashion, low vitamin D status has been linked to risk of infection and mortality in chronic kidney disease [Citation12], and seasonal variations of infections such as influenza, the latter highlighting a potential role for vitamin D in counteracting infection in the upper respiratory tract [Citation13]. Increasing numbers of studies have linked vitamin D insufficiency to aberrant adaptive immune function and increased risk or severity of autoimmune disease [Citation14,Citation15]. Low vitamin D status has been linked type 1 diabetes [Citation16,Citation17], and supplementation with vitamin D has been reported to protect against this disease [Citation18]. At a genetic level, specific VDR gene haplotypes appear to protect against diabetes [Citation19], and polymorphisms in the CYP27B1 gene have also been shown to affect diabetes susceptibility [Citation20]. Other autoimmune diseases linked to vitamin D insufficiency include Multiple Sclerosis (MS) (reviewed in [Citation21]), and Crohn's disease, a form of inflammatory bowel disease (IBD) [Citation22–24]. Crohn's disease is considered to be an autoimmune disease due to aberrant colonic immune responses to enteric bacteria. Intriguingly, current studies have implicated aberrant innate immune handling of enteric microbiota as an initiator of the adaptive immune damage associated with Crohn's disease [Citation25]. Consequently, it is possible that the effects of vitamin D on IBD may involve both the activation of innate immunity, together with the suppression of adaptive immunity and associated inflammation.
Epidemiological reports have highlighted association between vitamin D status and immune abnormalities. However, the link between vitamin D and normal human immunity is perhaps best illustrated by the functional analyses that have defined the mechanisms by which cells from the immune system handle and utilize vitamin D. These studies have revealed pathways for vitamin D metabolism and signal transduction that are both similar to, but distinct from, the vitamin D endocrine system associated with classical calciotropic and skeletal actions of vitamin D. The aim of the following review will be to summarize our current knowledge of the intracrine, paracrine, and endocrine systems that mediate immune responses to vitamin D. In doing so, the review will also describe the potential for dysregulation of these vitamin D pathways, and associated effects on immune diseases.
Intracrine vitamin D – a mechanism for innate antibacterial activity
One of the earliest studies to describe a potential mechanism for the beneficial effects of vitamin D on TB, showed that the active form of vitamin D, 1,25(OH)2D, potently suppressed proliferation of the infectious pathogen associated with TB, Mycobacterium tuberculosis (M. tb), in human monocytes [Citation26]. Based on these data, it was assumed that therapeutic administration of 1,25(OH)2D or synthetic non-calcemic analogs of 1,25(OH)2D would provide the most effective strategy for translational use of vitamin D in patients with TB. This was somewhat surprising as it had been recognized for some time prior to the M. tb study that macrophages were a potential extra-renal source of 1,25(OH)2D. For example, extra-renal activity of the enzyme 25-hydroxyvitamin D-1α-hydroxylase (1α-hydroxylase) was detectable in macrophages associated with the granulomatous disease sarcoidosis [Citation27]. In this instance the localized production of 1,25(OH)2D by 1α-hydroxylase in macrophages was sufficient to spill-over into the general circulation and, in some instances, cause dysregulation of calcium homeostasis [Citation28]. Subsequent studies showed that macrophage 1α-hydroxylase activity is common to granulomatous diseases in general, as well as several types of tumor involving significant macrophage infiltration [Citation29]. Other studies went on to show that synthesis of 1,25(OH)2D was also detectable in normal, non-disease macrophages following activation with inflammatory cytokines such as interferon γ (IFNγ) [Citation30], or an immunogen such as lipopolysaccharide (LPS) [Citation31]. Indeed, it was subsequently shown that expression of 1α-hydroxylase was common to many tissues [Citation32], and involved the same gene (CYP27B1) associated with 1α-hydroxylase expression in the kidney [Citation33]. However, despite these early observations, it was only much later that the significance of macrophage 1,25(OH)2D production on normal human immune function was clarified.
In a series of seminal experiments Liu et al. showed for the first time that monocyte synthesis of 1,25(OH)2D was an integral part of innate immune responses to the pathogen M. tb [Citation34]. In this study, gene array analyses revealed that monocyte expression of CYP27B1 and the nuclear receptor for 1,25(OH)2D, VDR, was induced following activation of toll-like receptor 2/1 (TLR2/1), a pathogen recognition receptor for gram-positive bacteria and M. tb [Citation34]. As a consequence, TLR2/1-activated cells treated with 25(OH)D showed increased local synthesis of 1,25(OH)2D, which could then bind to and signal via the VDR. This represented a potential vitamin D intracrine system for localized, VDR mediated regulation of gene expression. Amongst the potential targets for VDR in this setting is the gene for cathelicidin (LL37), which encodes a protein known to be involved in promoting intracellular killing of bacteria [Citation35,Citation36]. Prior studies had shown that transcription of LL37 is stimulated in a direct fashion by the 1,25(OH)2D-VDR complex [Citation37] acting via specific vitamin D response elements (VDRE) within the LL37 gene promoter [Citation38]. This VDRE appears to be specific for primates, as the VDRE is not observed in the LL37 gene promoter of lower mammals, and cells from animals such as mice show no induction of LL37 when treated with 1,25(OH)2D [Citation38,Citation39].
The TLR2/1-CYP27B1-VDR-mediated induction of monocyte LL37 was the first example of an intracrine system in which enhanced killing of a pathogen such as M. tb could be achieved simply by increasing localized synthesis of 1,25(OH)2D. As this mechanism was exquisitely sensitive to the availability of substrate 25(OH)D, it was proposed that simple variations in vitamin D status could enhance or impair this intracrine response to infection. Indeed monocytes cultured in medium supplemented with serum from vitamin D-insufficient donors showed lower concentrations of LL37 expression following TLR2/1 activation when compared to cells cultured in serum from vitamin D-sufficient donors [Citation34]. Conversely TLR2/1-induced monocyte expression of LL37 was increased in serum from vitamin D-insufficient subjects after a program of in vivo supplementation with vitamin D [Citation40]. The over-arching conclusion from these reports was that intracrine metabolism of 25(OH)D is a pivotal component of antibacterial activity in monocytes that is independent of the classical endocrine systems for 25(OH)D metabolism. In the basic model shown in , synthesis of 1,25(OH)2D is more likely to be compromised by decreased availability of its precursor 25(OH)D, indicating that vitamin D-insufficiency may lead to impaired innate immune response to infection. Nevertheless, it is now clear that other factors can also influence the efficacy of intracrine vitamin D metabolism and associated immune activity, and these will be discussed in the remainder of the review.
Figure 1. Intracrine metabolism of vitamin D and monocyte antibacterial immunity. Bacterial pathogens are phagocytosed by cells such as monocytes but are the able to undergo intracellular replication that may threaten the host cell. Pattern recognition receptors such as the toll-like receptor (TLR) family can also act as monocyte sensors of pathogens, with concomitant effects on expression of genes such as CYP27B1 and VDR. Induction of these components of the vitamin D system provides an mechanism for conversion of 25(OH)D (25D) to 1,25(OH)2D (1,25D) and subsequent intracrine nuclear signaling via the VDR. Amongst the genes induced by the intracrine system is cathelicidin (LL37) which acts as an antibacterial protein when incorporated into the phagosome. Other antibacterial factors such as β-defensin 2 (DEFB4) are also induced by intracrine 1,25D, but require additional stimulation by nuclear factor-kappa B (NF-κB), stimulated by factors such as interleukin-1 (IL-1) or NOD2 and its ligand muramyl dipeptide (MDP). Intracrine metabolism of vitamin D also promotes bacterial killing via enhanced autophagy which promotes formation of an autophagosome. Induction of an intracrine vitamin D system in monocytes via TLR activation appears to require interleukin-15 (IL-15) as an intermediary and can also be stimulated by interferon γ (IFNγ), with enhanced antibacterial activity. Conversely, interleukin-4 (IL-4) suppresses intracrine antibacterial activity of vitamin D by enhancing the vitamin D catabolic enzyme 24-hydroxylase (CYP24A1).
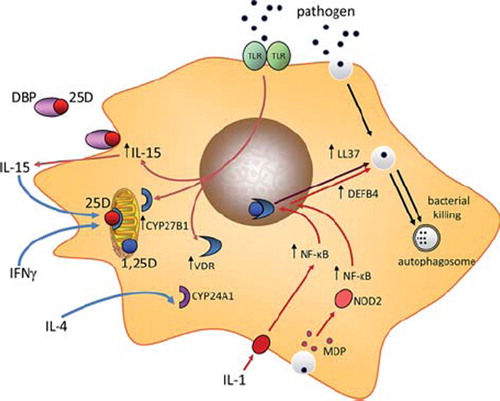
The precise mechanism by which pathogens such as M. tb enhance transcription of VDR and CYP27B1 via TLR2/1 has yet to be clarified. Monocytes stimulated with the TLR4 ligand LPS in combination with IFNγ have shown that the JAK-STAT, p38 MAP kinase, and NF-κB pathways are involved in stimulating CYP27B1 expression [Citation41]. However, other studies suggest that induction of CYP27B1 via TLR2 involves a cytokine intermediary in the form of interleukin-15 (IL-15) [Citation42] (see ). In this setting IL-15 appears to act in an autocrine rather than intracrine fashion in that the cytokine is secreted by the TLR-activated monocyte to then act on the same cell. However, to date, the precise mechanism by which this occurs has yet to be defined.
Since the original study by Lui et al. [Citation34], the intracrine model for vitamin D-mediated antibacterial function in monocytes has been greatly expanded to include other mechanisms that facilitate the metabolism of 25(OH)D and enhance innate immune responses to 1,25(OH)2D (see ). For example, 1,25(OH)2D has been shown to induce expression of other antibacterial proteins such as β-defensin 2. Like LL37, the β-defensin 2 gene (DEFB4) promoter contains a VDRE [Citation37], but this did not appear to be stimulated by 1,25(OH)2D alone [Citation34]. Instead induction of DEFB4 by 1,25(OH)2D-VDR appears to involve cooperation with another transcription factor, nuclear factor kappa N (NF-κB). This can be achieved by treatment of monocytes with cytokines such as interleukin-1β (IL-1β) [Citation43], or as a consequence of signaling via the intracellular pathogen recognition receptor NOD2 [Citation44], which is potently induced by 1,25(OH)2D in a variety of cell types, and enhances sensitivity to the NOD2 ligand muramyl dipeptide (MDP), a product of Gram-positive and Gram-negative bacteria. Both IL-1 and liganded NOD2 can therefore act as co-stimuli to promote 1,25(OH)2D-VDR-mediated induction of DEFB4. In this way, the intracrine function of vitamin D in monocytes or macrophages may not simply be dependent on vitamin D status and the availability of substrate 25(OH)D for conversion to 1,25(OH)2D. Rather, it seems more likely that effective handling of vitamin D will require additional intracrine or paracrine stimuli as part of the broader response immune response to a particular pathogen.
The intracrine activity of vitamin D in monocytes is not restricted to induction of antibacterial proteins such as LL37 and DEFB4. Recent studies suggest that vitamin D can also act to enhance the environment in which monocytes carry out bacterial killing. In particular, studies have focused on autophagy, an intracellular mechanism known to be essential for the general cytoplasmic homeostasis in eukaryotes [Citation45], but which is also important as a mechanism for intracellular isolation and eradication of pathogens [Citation46]. The induction of autophagy in monocytes following treatment with 1,25(OH)2D is associated with enhanced intracellular killing of M. tb [Citation47], and TLR2/1-mediated induction of autophagy appears to involve intracrine 25(OH)D metabolism via CYP27B1 [Citation48]. Intriguingly, it also appears that activation of autophagy in monocytes by 1,25(OH)2D is dependent on expression of LL37 [Citation47]. To date no direct autophagy effects have been described for monocytes treated with 1,25(OH)2D, but it is interesting to note recent studies showing that 1,25(OH)2D induces expression of the mTOR inhibitor DDIT4 [Citation49]. Conventional inhibition of mTOR with the chemical agent rapamycin is known to promote autophagy [Citation50]. Thus, it is tempting to speculate that similar inhibition of mTOR via 1,25(OH)2 D-mediated induction of DDIT4 will also promote direct effects in suppressing mTOR and enhancing autophagy [Citation51].
Paracrine factors that modulate intracrine vitamin D metabolism
In considering the regulation of antibacterial responses via an intracrine vitamin D pathway, it is important to recognize that successful immune response to pathogens such as M. tb cannot be achieved by the innate immune system alone. Instead, T cells from the adaptive immune system also play a pivotal role in directing the eradication of pathogens. This is illustrated by recent studies showing that T cell cytokines such as IFNγ further enhance TLR2/1-induced CYP27B1 and associated antibacterial activity [Citation52]. Indeed more recent studies have shown that IFNγ-mediated antibacterial responses in macrophages are dependent on vitamin D [Citation53], underlining the link between IFNγ and intracrine metabolism of vitamin D. IFNγ is a marker of the T helper (Th)1 subset of T cell immune responses, whilst Th2 T-cells are characterized by cytokines such as IL-4. It is therefore interesting to note studies showing that IL-4 and IFNγ exert opposing effects on monocyte vitamin D metabolism, with IL-4 targeting an alternative intracrine pathway for vitamin D metabolism involving the catabolic enzyme 24-hydroxylase (CYP24A1), which generates less active 24-hydroxylated metabolites from 25(OH)D or 1,25(OH)2D [Citation54]. Expression of CYP24A1 is induced by its primary substrate, 1,25(OH)2D, and in monocytes expression of CYP24A1 parallels antibacterial LL37 [Citation34]. Similar to 1,25(OH)2D, treatment of monocytes with IL-4 stimulated 24-hydroxylase activity in monocytes, but in this case the effect was associated with attenuated TLR2/1-mediated intracrine induction of LL37 [Citation52]. This effect was dependent on the CYP24A1 enzyme but treatment with the IL-4 did not increase monocyte expression of mRNA for CYP24A1. Instead the cytokine suppressed expression of CYP24A1 and several other cytokines with putative capacity for 24-hydroxylation [Citation52]. Thus, it is possible that IL-4 enhanced 24-hydroxylase activity in monocytes is due to indirect suppression of a competitor enzyme to CYP24A1. The data suggest that the Th1 and Th2 adaptive immune responses have opposing effects on vitamin D metabolism [Citation52]. By utilizing these cytokine-specific mechanisms for regulation of its intracrine metabolism vitamin D may therefore act as a coordinator of the interface between the innate and adaptive immune function.
The induction of LL37 by 1,25(OH)2D has been reported for a variety of cell types including bronchial epithelial cells [Citation55], myeloid cell lines [Citation38], and decidual [Citation56], and trophoblastic cells of the placenta [Citation57], but this response is not universal [Citation58]. Moreover, in some cells that show induction of LL37 following treatment with 1,25(OH)2D, it is unclear whether these cells are also able to exhibit intracrine synthesis of 1,25(OH)2D capable of supporting induction of antibacterial activity. Human keratinocytes have relatively low expression of TLR2 and are therefore less able to induce CYP27B1 in response to a pathogenic challenge [Citation59]. In this setting other tissue-specific factors such as transforming growth factor-beta (TGFβ) can act as co-stimulatory factors to enhance keratinocyte CYP27B1. The resulting increased production of 1,25(OH)2D acts to stimulate keratinocyte TLR expression, thereby enhancing sensitivity to TLR2 ligands. This, in turn, leads to further stimulation of epidermal CYP27B1, and intracrine 1,25(OH)2 D-mediated production of antimicrobial LL37 [Citation59]. Because TGFβ is released in the skin following epidermal wounding, it has been suggested that vitamin D-induced LL37 may provide a mechanism for the prevention of infection following wounding.
Studies in vitro suggest that paracrine factors play an important role in determining the efficacy of intracrine antibacterial responses to vitamin D [Citation52,Citation53]. However, similar paracrine factors may also promote dysregulation of vitamin D function under disease conditions. Recent analysis of tissue from different forms of the mycobacterial disease Leprosy has shown that different forms of the disease have completely different patterns of CYP27B1 and VDR expression [Citation60]. DNA array analysis of gene expression profiles associated with tuberculoid (T-lep) and lepromatous (L-lep) forms of leprosy showed elevated expression of the vitamin D-activating enzyme CYP27B1, the catabolic enzyme CYP24A1, and the VDR in T-lep vs L-lep lesions [Citation60] (see ). Activation of CYP27B1 and VDR in one form of leprosy (T-lep), but not in another form (L-lep), despite similar exposure to the leprosy pathogen (Mycobacterium leprae or Mycobacterium lepromatosis) suggests that additional stimuli are involved in controlling the vitamin D system in this disease. This appears to involve differential patterns of cytokine expression, with T-lep being characterized by production of IFNγ and IL-15, and L-lep by interferon IFNα and/or IFNβ, IL-10 and IL-4 [Citation61, Citation62]. Given that IFNγ and IL-15 are potent inducers of CYP27B1 and vitamin D-mediated antibacterial activity, whilst IL-4 suppresses vitamin D function via enhanced 24-hydroxylases activity, it is possible to speculate that the T-lep form of leprosy benefits from optimal vitamin D intracrine function, whilst the opposite is true of L-lep. This model is further endorsed by recent studies showing that the L-lep form of leprosy is associated with expression of the microRNA (miR) species miR-21 which has the potential to target CYP27B1 and suppress expression of 1α-hydroxylase [Citation63] (). The over-arching conclusion form these observations is that the efficacy of intracrine vitamin D metabolism as a modulator of immune function is not simply defined by available 25(OH)D substrate for CYP27B1, but it may also be influenced by disease-specific factors, including microRNAs. In other settings, disease-associated factors may cause inappropriate activity of the vitamin D system. For example, elevated expression of IL-15 is frequently associated with inflammatory diseases, notably the granulomatous disease sarcoidosis [Citation64]. In view of the fact that over-production of 1,25(OH)2D is frequently observed in patients with granulomatous diseases [Citation65], it is possible that IL-15-mediated induction of 1α-hydroxylase contributes to the dysregulation of 1,25(OH)2D production observed in some inflammatory diseases.
Figure 2. Leprosy, a model for disease-associated dysregulation of intracrine vitamin D. The disease leprosy is associated with infection by Mycobacterium leprae or Mycobacterium lepromatosis (mLep), with mLep signaling via TLR2/1. Two forms of leprosy, Tuberculoid leprosy (T-lep) and Lepromatous leprosy (M-lep) are associated with distinct cytokine profiles: IFNγ and IL-15 in T-lep and interferon α (IFNα), IL-4 and interleukin-10 (IL-10) in L-lep. L-lep monocytes also express the microRNA, miR-21 that targets expression of the CYP27B1 gene product. DNA array analysis of T-lep and L-lep lesions, as well as reversal reaction (rr) L-lep lesion reveals differential patterns of gene expression for CYP27B1, VDR and CYP24A1, with expression of all three gene being decreased in L-lep. Expression of CYP27B1, VDR and CYP24A1 is retsored in L-lep lessions that undergo spontaneous conversion to T-lep (rr). The enhanced intracrine vitamin D system in T-lep monocytes enables synthesis of 1,25(OH)2D (1,25D) from 25(OH)D (25D) and associated antimycobacterial activity in these cells. By contrast, in L-lep IL-10 promotes phagocytosis but without an effective intracrine vitamin D system to kill phagocytosed mycobacteria.
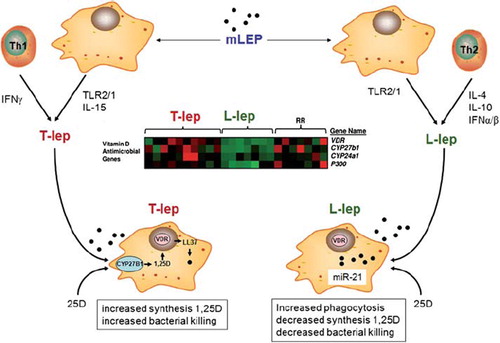
Paracrine vitamin D – a role for vitamin D in antigen presentation and T-cell function
Optimal response to infection involves both innate and adaptive immunity. At the interface between these two arms of the immune system are antigen-presenting cells (APCs), which deliver bacterial antigens to cells from the adaptive immune system such as T cells. APCs include macrophages but antigen presentation is more effectively carried out by dendritic cells (DCs). As with monocytes and macrophages, DCs isolated from lymphoid tissue express VDR [Citation66], and show immunomodulatory responses to 1,25(OH)2D, which suppresses DC maturation, and promotes a more immunosuppressive APC phenotype [Citation67,Citation68]. This effect appears to be more pronounced in myeloid DCs (mDCs) relative to plasmacytoid DCs (pDCs), despite the fact that both subsets exhibit similar concentrations of VDR [Citation69]. Under steady state conditions mDCs actively prime naïve T cell responses, whereas pDCs are more tolerogenic or immunosuppressive. In this setting, 1,25(OH)2D fulfills a more tolerogenic function by suppressing mDC activity but without any major effect on the already tolerogenic pDCs.
DCs belong to the same hematopoietic lineage as monocytes, and both types of cell express a potent intracrine vitamin D system [Citation70,Citation71]. However, whereas in monocytes and macrophages intracrine metabolism of 25(OH)D promotes bacterial killing, in DCs intracrine synthesis of 1,25(OH)2D acts to suppress the maturation and function of these cells [Citation70]. Differentiation of DCs towards a mature, APC phenotype, is associated with increased expression of CYP27B1 but with a reciprocal decrease in VDR expression [Citation70]. It therefore unlikely that any 1,25(OH)2D produced by mature DCs will act in an intracrine fashion due to low VDR concentrations in CYP27B1-expressing DCs. In this setting it seems more likely that DCs will utilize a paracrine vitamin D system, with VDR-rich immature DCs responding to 1,25(OH)2D produced by VDR-depleted mature DCs. A mechanism such as this has significant advantages in that it allows some DCs to mature and promote activation of normal adaptive immune responses, whilst preventing an over-elaboration of this response that could lead to pathological, autoimmune consequences (). The importance of 1,25(OH)2D as a modulator of DC function is supported by studies of VDR and CYP27B1 gene knockout mice, in which both types of mouse model presented with lymphatic abnormalities consistent with increased numbers of mature DCs [Citation72,Citation73] and aberrant DC trafficking [Citation74].
Figure 3. Paracrine metabolism of 25(OH)D and the regulation of adaptive immunity. Synthesis of 1,25(OH)2D (1,25D) from 25(OH)D (25D) in mature dendritic cells (mDC) expressing CYP27B1 can exert effects on: 1) immature DCs (iDC) expressing VDR; 2) activated T cells (Th0) expressing VDR. Resulting effects on Th0 cells include: 1) activation of regulatory T cells (Treg); 2) activation of Th2 cells; 3) suppression of interleukin-17 (IL-17) expressing Th17 cells; suppression of Th1 cells.
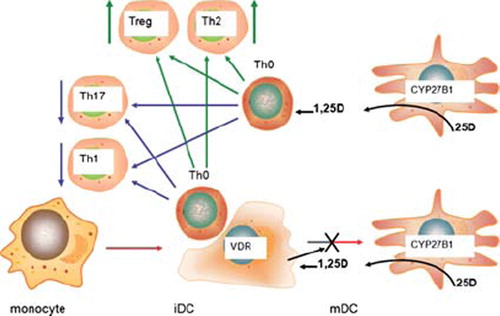
DC generation of 1,25(OH)2D may influence antigen presentation in a paracrine fashion, but a similar paracrine mechanism may also induce direct effects on T and B cells independent of DC responses. T cells and B cells express VDR [Citation75,Citation76], and because these concentrations increase with cell proliferation [Citation77] it was initially assumed that 1,25(OH)2D acts to suppress T cell/B cell proliferation [Citation77–79]. Subsequently it was shown that 1,25(OH)2D could also modulate T cell phenotype. In vitro exposure to 1,25(OH)2D inhibited Th1 cells, a subset of CD4 + effector T cells associated with cellular immune responses [Citation80], whilst enhancing cytokines associated with humoral immunity Th2 cells [Citation81,Citation82]. In this way it was proposed vitamin D could protect against inflammatory tissue damage associated with Th1 cellular immune responses. However, more recent studies suggest that the effects of 1,25(OH)2D on T cells are more complex and involve a third effector T cell population termed Th17 cells [Citation83,Citation84]. Th17 cells produce the cytokine interleukin-17 (IL-17) and they are important mediators of immune responses to some pathogens. Nevertheless they have also been linked to inflammatory tissue damage and consequently represent a potentially important disease marker [Citation85,Citation86]. In vitro, expression of IL-17 [Citation87], and development of Th17 cells is suppressed by 1,25(OH)2D [Citation88,Citation89]. Similar suppression of IL-17 expression by 1,25(OH)2D is also observed in mouse models of inflammatory bowel disease [Citation90]. By contrast, loss of 1,25(OH)2D in CYP27B1 gene knockout mice is associated with elevated concentrations of IL-17 [Citation91].
In addition to effector T cells, 1,25(OH)2D also exerts effects on suppressor or regulatory cells (Treg), a group of CD4+ T cells that inhibit the proliferation of other CD4+ T cells. Treatment of naive CD4+ T cells with 1,25(OH)2D potently induces Treg populations [Citation92], with potential benefits for prevention of autoimmune disease or host-graft rejection [Citation93–95]. Studies in vitro have shown that 1,25(OH)2D can act directly on VDR-expressing CD4+ T cells to promote Treg development [Citation96,Citation97]. However, it is also possible that 1,25(OH)2D stimulates Tregs indirectly by promoting immature DCs [Citation98–100]. As outlined above and in , both of these mechanisms may be influenced by variations in DC intracrine synthesis of 1,25(OH)2D, providing a possible link between low serum 25(OH)D status and impaired Treg activity [Citation29]. The overall conclusion from studies of T cell phenotype is that 1,25(OH)2D acts to maintain a balance between inflammatory Th1/Th17 cells and immunosuppressive Th2/Treg.
Other populations of lymphocytes may also be influenced by vitamin D. CD8+ cytotoxic T cells express VDR but they appear to be relatively insensitive to antiproliferative responses compared to CD4+ T cells [Citation101,Citation102]. The effects of vitamin D on CD8+ T cells may be sub-set-specific. The CD8 molecule can be expressed as either an alpha-beta heterodimer or as an alpha-alpha homodimer, with the latter being influenced by 1,25(OH)2D. Studies using the VDR gene knockout mouse have shown aberrant gut migration of CD8+ alpha alpha cells, with this being linked to increased risk of gastrointestinal inflammation [Citation103]. This is similar to the positive effect of 1,25(OH)2D on epidermal T cell homing [Citation104], but contrasts its negative effects on T cell homing to lymph nodes [Citation105]. CD8 + cells have been implicated in the pathophysiology of MS and the mouse model of this disease, Experimental Autoimmune Encephalomyelitis (EAE) [Citation106]. However, although 1,25(OH)2D can protect against EAE [Citation107], this does not appear to require the presence of CD8 + cells. VDR-expressing B cells show antiproliferative [Citation108] and immunoglobulin inhibitory [Citation109] responses to 1,25(OH)2D. In vitro treatment of B cells with 1,25(OH)2D has also been shown to inhibit the differentiation of plasma cells and class switched memory cells [Citation110], highlighting a potential role for vitamin D in B cell-related disorders such as systemic lupus erythamtosus.
Endocrine vitamin D – normal neutrophil function and inflammatory disease
Immune cell-specific expression of CYP27B1 and associated intracrine synthesis of 1,25(OH)2D is cornerstone of the interaction between vitamin D and the immune system. Nevertheless, it is possible that some immune functions of vitamin D are mediated at the level of systemic, endocrine responses. For example, granulocytic cells such as neutrophils are a crucial component of innate immune activity by providing a rapid response to infection. Neutrophils express abundant VDR [Citation111]. and show 1,25(OH)2D-induced expression of LL37 similar to that observed in monocytes [Citation37]. The physiological significance of this is not yet clear as there is no evidence for functional 1α-hydroxylase activity in neutrophils. As such, it is possible that these cells act as systemic responders to active 1,25(OH)2D, rather than precursor 25(OH)D this may be functionally important as the abundance of neutrophils means that they are the major systemic source of the LL37 protein, also known as human cathelicidin antibacterial protein (hCAP) [Citation112]. Recent studies have described a link between serum hCAP and disease mortality in patients with chronic kidney disease [Citation12], with low hCAP concentrations being correlated with circulating concentrations of 1,25(OH)2D rather than 25(OH)D. In the setting of VDR-positive, CYP27B1-negative neutrophils it is possible to speculate that some immune responses to infection are due to 1,25(OH)2D acting as endocrine factor. However, this does not appear to be a universal mechanism for regulation of systemic hCAP. In patients with sepsis, a systemic inflammatory response to infection characterized by high concentrations of neutrophils, low circulating concentrations of hCAP in more critically ill patients were associated with low serum concentrations of 25(OH)D [Citation11].
Conclusions
In the last five years there have been remarkable advances in our understanding of how vitamin D influences normal human immune function. This new perspective on vitamin D and immunity has arisen in part from studies that have clarified the mechanisms by which precursor 25(OH)D is able to direct immunomodulatory actions in a wide array of immune cells types. As detailed in this review, intracrine, paracrine or endocrine pathways may be involved in synthesizing 1,25(OH)2D, with concomitant innate and adaptive immune effects on diverse cells expressing VDR. The efficacy of these actions will be determine in large part by the availability of substrate 25(OH)D for the enzyme 1α-hydroxylase and thus this will be strongly influenced by patient vitamin D status. However, as outlined in this review, disease- or immune response-specific factors can act to fine-tune the systems for vitamin D metabolism and function. Thus future therapeutic use of vitamin D may be improved by co-therapies that optimize the metabolic potential of vitamin D. Many questions still remain. For example, are intracrine responses to vitamin D restricted to innate antibacterial or antigen presentation responses? Both T cells [Citation104], and B cells [Citation110] have been reported to express CYP27B1, suggesting that these cells may be influenced by intracrine synthesis of 1,25(OH)2D. Another key question is whether or not the innate antibacterial activity of vitamin D is restricted to primates? Lower mammals such as mice do not express the promoter VDREs required for vitamin D-mediated transcriptional regulation of antibacterial proteins such as LL37 and DEFB4, but are there other mechanisms for immune regulation by vitamin D in mice? Perhaps the most important challenge facing vitamin D-immunity research is the initiation of new prospective clinical trials that will complement the existing observational association analyses. For many diseases, particularly autoimmune diseases, this is a huge logistical undertaking complicated by uncertainty over whether vitamin D can be used as therapy for some diseases or whether it simply acts to protect against the onset of disease. Further investigation of the basic molecular mechanisms underpinning the immune function of vitamin D will greatly help to address these issues.
Questions and answers
JC Souberbielle, France
I understood that FGF23 modulates CYP27B1 and maybe CYP24 in the monocytes. However, FGF23 acts through the binding of FGF receptors which leads to association with the Klotho protein. So did you study the presence of the Klotho protein in these cells, since I am not aware that it is expressed there?
M Hewison
We looked at all FGF receptor isoforms. There is a strong expression of FGFR1 but not much at all of the other three isoforms. The Klotho is very weak. The Klotho expression was induced when we treated the cells with FGF234 so I don't know whether this is an example of FGF23 acting independently of Klotho. We do get a kinase response to the interaction of FGF23 to the receptor, so something is going on, it just may not be as strong as you see in the kidney. I'm not sure that it matters because in the monocyte there is this strong regulation of the CYP27, so it is being induced in response to an immune challenge. I'm wondering whether FGF23 is there in large amounts or will just block. I don't think it needs Klotho.
G Jones, Canada
In your studies of the 24 hydroxylase, I'm interested to know whether it is active. I thought it had been shown that the form of CYP24 found in the immune system is defective, so is that a CYP24 that works to catabolise vitamin D or not?
M Hewison
If a monocyte is treated with 1,25(OH)2D3, it will induce a lot of CYP24A1 and much of that will be a splice variant form which is catabolically inactive, so you don't get as much 24,25(OH)2D3 production in the monocytes as you would expect to see, perhaps, in the kidney cell. However, with the IL4 effect we experienced, that was absolutely induction of 24 hydroxylase activity. This is interesting, because in the study in which we reported this, we looked at CYP24A1, with which we did not get an IL4 effect. IL4 does not up-regulate CYP24A1; it's actually suppressing it and a whole lot of other cytochrome P450s, so there is some other mechanism, not transcriptional, by which IL4 is able to really strongly boost 24 hydroxylation and we don't know what this is.
G Jones
We have already discussed how we would like to know the intracellular concentration of 1,25(OH)2D3 in all the different target cells. Given that there is a slice variant and there is a normal form of CYP24, what would you speculate the 1,25(OH)2D3 concentration is? Is it higher or lower that the circulating blood concentration or does it change, dependent on status?
M Hewison
I always think it is going to be higher. We did a study where we tried to compare efficiency pathways, using 25(OH)D3 and converting it locally within a monocyte or a dendritic cell. The amount of 1,25(OH)2D3 we produced, disrupting the cells and looking at the supernatant in 25(OH)D3 treated cells and got a biological response and we needed much less 1,25(OH)2D3 to get the biological response than we were adding exogenously. So, it is possible we don't actually need as much 25(OH)D3 to produce an intracellular reaction as we have in the circulation. I always think it is inefficient to be taking something which has to be converted locally to be effective. However, in this case, it does seem to be more effective to take 25(OH)D3 than to have endogenous 1,25(OH)2D3.
Declaration of interest: The author report no conflicts of interest. The author alone is responsible for the content and writing of the paper.
References
- Moller KI, Kongshoj B, Philipsen PA, Thomsen VO, Wulf HC. How Finsen's light cured lupus vulgaris. Photodermatol Photoimmunol Photomed 2005;21:118–124.
- Grad R. Cod and the consumptive: a brief history of cod-liver oil in the treatment of pulmonary tuberculosis. Pharmacy in history 2004;46:106–120.
- Herrera G. Vitamin D in massive doses as an adjuvant to the sulfones in the treatment of tuberculoid leprosy. Int J Lepr 1949;17:35–42.
- Wilkinson RJ, Llewelyn M, Toossi Z, Patel P, Pasvol G, Lalvani A, . Influence of vitamin D deficiency and vitamin D receptor polymorphisms on tuberculosis among Gujarati Asians in west London: a case-control study. Lancet 2000;355:618–621.
- Ustianowski A, Shaffer R, Collin S, Wilkinson RJ, Davidson RN. Prevalence and associations of vitamin D deficiency in foreign-born persons with tuberculosis in London. J Infect 2005;50:432–437.
- Williams B, Williams AJ, Anderson ST. Vitamin D deficiency and insufficiency in children with tuberculosis. Pediatr Infect Dis J 2008;27:941–942.
- Wejse C, Gomes VF, Rabna P, Gustafson P, Aaby P, Lisse IM, . Vitamin D as supplementary treatment for tuberculosis: a double-blind, randomized, placebo-controlled trial. Am J Respir Crit Care Med 2009;179: 843–850.
- Martineau AR, Wilkinson RJ, Wilkinson KA, Newton SM, Kampmann B, Hall BM, . A single dose of vitamin d enhances immunity to mycobacteria. Am J Respir Crit Care Med 2007;176:208–213.
- Nursyam EW, Amin Z, Rumende CM. The effect of vitamin D as supplementary treatment in patients with moderately advanced pulmonary tuberculous lesion. Acta Med Indones 2006;38:3–5.
- Martineau AR, Timms PM, Bothamley GH, Hanifa Y, Islam K, Claxton AP, . High-dose vitamin D(3) during intensive-phase antimicrobial treatment of pulmonary tuberculosis: a double-blind randomised controlled trial. Lancet 2011;377:242–250.
- Jeng L, Yamshchikov AV, Judd SE, Blumberg HM, Martin GS, Ziegler TR, . Alterations in vitamin D status and anti-microbial peptide levels in patients in the intensive care unit with sepsis. J Transl Med 2009;7:28.
- Gombart AF, Bhan I, Borregaard N, Tamez H, Camargo CA, Jr., Koeffler HP, . Low plasma level of cathelicidin antimicrobial peptide (hCAP18) predicts increased infectious disease mortality in patients undergoing hemodialysis. Clin Infect Dis 2009;48:418–424.
- Cannell JJ, Vieth R, Umhau JC, Holick MF, Grant WB, Madronich S, . Epidemic influenza and vitamin D. Epidemiol Infect 2006;134:1129–1140.
- Holick MF, Chen TC. Vitamin D deficiency: a worldwide problem with health consequences. Am J Clin Nutr 2008;87:1080S–1086S.
- Adorini L, Penna G. Control of autoimmune diseases by the vitamin D endocrine system. Nat Clin Pract Rheumatol 2008;4:404–412.
- Mathieu C, Gysemans C, Giulietti A, Bouillon R. Vitamin D and diabetes. Diabetologia 2005;48:1247–1257.
- Littorin B, Blom P, Scholin A, Arnqvist HJ, Blohme G, Bolinder J, . Lower levels of plasma 25-hydroxyvitamin D among young adults at diagnosis of autoimmune type 1 diabetes compared with control subjects: results from the nationwide Diabetes Incidence Study in Sweden (DISS). Diabetologia 2006;49:2847–2852.
- Harris SS. Vitamin D in type 1 diabetes prevention. J Nutr 2005;135:323–325.
- Ramos-Lopez E, Jansen T, Ivaskevicius V, Kahles H, Klepzig C, Oldenburg J, . Protection from type 1 diabetes by vitamin D receptor haplotypes. Ann N Y Acad Sci 2006;1079:327–334.
- Bailey R, Cooper JD, Zeitels L, Smyth DJ, Yang JH, Walker NM, . Association of the vitamin D metabolism gene CYP27B1 with type 1 diabetes. Diabetes 2007.
- Raghuwanshi A, Joshi SS, Christakos S. Vitamin D and multiple sclerosis. J Cell Biochem 2008;105:338–343.
- Vagianos K, Bector S, McConnell J, Bernstein CN. Nutrition assessment of patients with inflammatory bowel disease. JPEN J Parenter Enteral Nutr 2007;31:311–319.
- Pappa HM, Gordon CM, Saslowsky TM, Zholudev A, Horr B, Shih MC, . Vitamin D status in children and young adults with inflammatory bowel disease. Pediatrics 2006;118:1950–1961.
- Pappa HM, Grand RJ, Gordon CM. Report on the vitamin D status of adult and pediatric patients with inflammatory bowel disease and its significance for bone health and disease. Inflamm Bowel Dis 2006;12:1162–1174.
- Packey CD, Sartor RB. Commensal bacteria, traditional and opportunistic pathogens, dysbiosis and bacterial killing in inflammatory bowel diseases. Curr Opin Infect Dis 2009;22:292–301.
- Rook GA, Steele J, Fraher L, Barker S, Karmali R, O'Riordan J, . Vitamin D3, gamma interferon, and control of proliferation of Mycobacterium tuberculosis by human monocytes. Immunology 1986;57:159–163.
- Adams JS, Gacad MA. Characterization of 1 alpha-hydroxylation of vitamin D3 sterols by cultured alveolar macrophages from patients with sarcoidosis. J Exp Med 1985;161:755–765.
- Papapoulos SE, Clemens TL, Fraher LJ, Lewin IG, Sandler LM, O'Riordan JL. 1, 25-dihydroxycholecalciferol in the pathogenesis of the hypercalcaemia of sarcoidosis. Lancet 1979;1:627–630.
- Hewison M, Burke F, Evans KN, Lammas DA, Sansom DM, Liu P, . Extra-renal 25-hydroxyvitamin D3 - 1alpha-hydroxylase in human health and disease. J Steroid Biochem Mol Biol 2007;103:316–321.
- Koeffler HP, Reichel H, Bishop JE, Norman AW. Gamma-Interferon stimulates production of 1,25-dihydroxyvitamin D3 by normal human macrophages. Biochem Biophys Res Commun 1985;127:596–603.
- Reichel H, Koeffler HP, Bishop JE, Norman AW. 25-Hydroxyvitamin D3 metabolism by lipopolysaccharide-stimulated normal human macrophages. J Clin Endocrinol Metab 1987;64:1–9.
- Zehnder D, Bland R, Williams MC, McNinch RW, Howie AJ, Stewart PM, . Extrarenal expression of 25-hydroxyvitamin d(3)-1 alpha-hydroxylase. J Clin Endocrinol Metab 2001;86:888–894.
- Fu GK, Lin D, Zhang MY, Bikle DD, Shackleton CH, Miller WL, . Cloning of human 25-hydroxyvitamin D-1 alpha-hydroxylase and mutations causing vitamin D-dependent rickets type 1. Mol Endocrinol 1997;11: 1961–1970.
- Liu PT, Stenger S, Li H, Wenzel L, Tan BH, Krutzik SR, . Toll-like receptor triggering of a vitamin D-mediated human antimicrobial response. Science 2006;311:1770–1773.
- Zaiou M, Gallo RL. Cathelicidins, essential gene-encoded mammalian antibiotics. J Mol Med 2002;80:549–561.
- Zanetti M. Cathelicidins, multifunctional peptides of the innate immunity. J Leukoc Biol 2004;75:39–48.
- Wang TT, Nestel FP, Bourdeau V, Nagai Y, Wang Q, Liao J, . Cutting edge: 1,25-dihydroxyvitamin D3 is a direct inducer of antimicrobial peptide gene expression. J Immunol 2004;173:2909–2912.
- Gombart AF, Borregaard N, Koeffler HP. Human cathelicidin antimicrobial peptide (CAMP) gene is a direct target of the vitamin D receptor and is strongly up-regulated in myeloid cells by 1,25-dihydroxyvitamin D3. Faseb J 2005;19:1067–1077.
- Gombart AF, Saito T, Koeffler HP. Exaptation of an ancient Alu short interspersed element provides a highly conserved vitamin D-mediated innate immune response in humans and primates. BMC Genomics 2009;10:321.
- Adams JS, Ren S, Liu PT, Chun RF, Lagishetty V, Gombart AF, . Vitamin d-directed rheostatic regulation of monocyte antibacterial responses. J Immunol 2009;182: 4289–4295.
- Stoffels K, Overbergh L, Giulietti A, Verlinden L, Bouillon R, Mathieu C. Immune regulation of 25-hydroxyvitamin-D3 - 1alpha-hydroxylase in human monocytes. J Bone Miner Res 2006;21:37–47.
- Krutzik SR, Hewison M, Liu PT, Robles JA, Stenger S, Adams JS, . IL-15 links TLR2/1-induced macrophage differentiation to the vitamin D-dependent antimicrobial pathway. J Immunol 2008;181:7115–7120.
- Liu PT, Schenk M, Walker VP, Dempsey PW, Kanchanapoomi M, Wheelwright M, . Convergence of IL-1beta and VDR activation pathways in human TLR2/1-induced antimicrobial responses. PLoS One 2009;4:e5810.
- Wang TT, Dabbas B, Laperriere D, Bitton AJ, Soualhine H, Tavera-Mendoza LE, . Direct and indirect induction by 1,25-dihydroxyvitamin D3 of the NOD2/CARD15-defensin beta2 innate immune pathway defective in Crohn disease. J Biol Chem 285:2227–2231.
- Klionsky DJ, Emr SD. Autophagy as a regulated pathway of cellular degradation. Science 2000;290:1717–1721.
- Levine B, Deretic V. Unveiling the roles of autophagy in innate and adaptive immunity. Nat Rev Immunol 2007;7: 767–777.
- Yuk JM, Shin DM, Lee HM, Yang CS, Jin HS, Kim KK, . Vitamin D3 induces autophagy in human monocytes/macrophages via cathelicidin. Cell Host Microbe 2009;6:231–243.
- Shin DM, Yuk JM, Lee HM, Lee SH, Son JW, Harding CV, . Mycobacterial lipoprotein activates autophagy via TLR2/1/CD14 and a functional vitamin D receptor signalling. Cell Microbiol 2011;12:1648–1665.
- Lisse TS, Liu T, Irmler M, Beckers J, Chen H, Adams JS, . Gene targeting by the vitamin D response element binding protein reveals a role for vitamin D in osteoblast mTOR signaling. Faseb J 2011;25:937–947.
- Fabri M, Realegeno SE, Jo EK, Modlin RL. Role of autophagy in the host response to microbial infection and potential for therapy. Curr Opin Immunol 2011;23:65–70.
- Lisse TS, Hewison M. Vitamin D: a new player in the world of mTOR signaling. Cell Cycle 2011;10:1888–1889.
- Edfeldt K, Liu PT, Chun R, Fabri M, Schenk M, Wheelwright M, . T-cell cytokines differentially control human monocyte antimicrobial responses by regulating vitamin D metabolism. Proc Natl Acad Sci U S A 2010;107:22593–22598.
- Fabri M, Stenger S, Shin DM, Yuk JM, Liu PT, Realegeno S, . Vitamin D is required for IFN-gamma-mediated antimicrobial activity of human macrophages. Sci Transl Med 2011;3:104ra102.
- Sakaki T, Kagawa N, Yamamoto K, Inouye K. Metabolism of vitamin D3 by cytochromes P450. Front Biosci 2005;10:119–134.
- Yim S, Dhawan P, Ragunath C, Christakos S, Diamond G. Induction of cathelicidin in normal and CF bronchial epithelial cells by 1,25-dihydroxyvitamin D(3). J Cyst Fibros 2007;6:403–410.
- Evans KN, Nguyen L, Chan J, Innes BA, Bulmer JN, Kilby MD, . Effects of 25-hydroxyvitamin D3 and 1, 25-dihydroxyvitamin D3 on cytokine production by human decidual cells. Biol Reprod 2006;75:816–822.
- Liu N, Kaplan AT, Low J, Nguyen L, Liu GY, Equils O, . Vitamin D Induces Innate Antibacterial Responses in Human Trophoblasts via an Intracrine Pathway. Biol Reprod 2009;80:398–406.
- Schauber J, Dorschner RA, Yamasaki K, Brouha B, Gallo RL. Control of the innate epithelial antimicrobial response is cell-type specific and dependent on relevant microenvironmental stimuli. Immunology 2006;118:509–519.
- Schauber J, Dorschner RA, Coda AB, Buchau AS, Liu PT, Kiken D, . Injury enhances TLR2 function and antimicrobial peptide expression through a vitamin D-dependent mechanism. J Clin Invest 2007;117:803–811.
- Montoya D, Cruz D, Teles RM, Lee DJ, Ochoa MT, Krutzik SR, . Divergence of macrophage phagocytic and antimicrobial programs in leprosy. Cell Host Microbe 2009;6:343–353.
- Yamamura M, Uyemura K, Deans RJ, Weinberg K, Rea TH, Bloom BR, . Defining protective responses to pathogens: cytokine profiles in leprosy lesions. Science 1991;254:277–279.
- Jullien D, Sieling PA, Uyemura K, Mar ND, Rea TH, Modlin RL. IL-15, an immunomodulator of T cell responses in intracellular infection. J Immunol 1997;158:800–806.
- Liu PT, Wheelwright M, Teles R, Komisopoulou E, Edfeldt K, Ferguson B, . MicroRNA-21 targets the vitamin D-dependent antimicrobial pathway in leprosy. Nat Med 2012;18:267–273.
- Agostini C, Semenzato G. Cytokines in sarcoidosis. Semin Respir Infect 1998;13:184–196.
- Kallas M, Green F, Hewison M, White C, Kline G. Rare causes of calcitriol-mediated hypercalcemia: a case report and literature review. J Clin Endocrinol Metab 2010;95: 3111–3117.
- Brennan A, Katz DR, Nunn JD, Barker S, Hewison M, Fraher LJ, . Dendritic cells from human tissues express receptors for the immunoregulatory vitamin D3 metabolite, dihydroxycholecalciferol. Immunology 1987;61:457–461.
- Penna G, Adorini L. 1 Alpha,25-dihydroxyvitamin D3 inhibits differentiation, maturation, activation, and survival of dendritic cells leading to impaired alloreactive T cell activation. J Immunol 2000;164:2405–2411.
- Adorini L, Penna G, Giarratana N, Uskokovic M. Tolerogenic dendritic cells induced by vitamin D receptor ligands enhance regulatory T cells inhibiting allograft rejection and autoimmune diseases. J Cell Biochem 2003;88:227–233.
- Penna G, Amuchastegui S, Giarratana N, Daniel KC, Vulcano M, Sozzani S, . 1,25-Dihydroxyvitamin D3 selectively modulates tolerogenic properties in myeloid but not plasmacytoid dendritic cells. J Immunol 2007;178: 145–153.
- Hewison M, Freeman L, Hughes SV, Evans KN, Bland R, Eliopoulos AG, . Differential regulation of vitamin D receptor and its ligand in human monocyte-derived dendritic cells. J Immunol 2003;170:5382–5390.
- Fritsche J, Mondal K, Ehrnsperger A, Andreesen R, Kreutz M. Regulation of 25-hydroxyvitamin D3 - 1 alpha-hydroxylase and production of 1 alpha,25-dihydroxyvitamin D3 by human dendritic cells. Blood 2003;102:3314–3316.
- Griffin MD, Lutz W, Phan VA, Bachman LA, McKean DJ, Kumar R. Dendritic cell modulation by 1alpha,25 dihydroxyvitamin D3 and its analogs: a vitamin D receptor-dependent pathway that promotes a persistent state of immaturity in vitro and in vivo. Proc Natl Acad Sci USA 2001;98:6800–6805.
- Panda DK, Miao D, Tremblay ML, Sirois J, Farookhi R, Hendy GN, . Targeted ablation of the 25-hydroxyvitamin D 1alpha -hydroxylase enzyme: evidence for skeletal, reproductive, and immune dysfunction. Proc Natl Acad Sci U S A 2001;98:7498–7503.
- Enioutina EY, Bareyan D, Daynes RA. TLR-induced local metabolism of vitamin D3 plays an important role in the diversification of adaptive immune responses. J Immunol 2009;182:4296–4305.
- Bhalla AK, Amento EP, Clemens TL, Holick MF, Krane SM. Specific high-affinity receptors for 1,25-dihydroxyvitamin D3 in human peripheral blood mononuclear cells: presence in monocytes and induction in T lymphocytes following activation. J Clin Endocrinol Metab 1983;57:1308–1310.
- Provvedini DM, Tsoukas CD, Deftos LJ, Manolagas SC. 1,25-dihydroxyvitamin D3 receptors in human leukocytes. Science 1983;221:1181–1183.
- Nunn JD, Katz DR, Barker S, Fraher LJ, Hewison M, Hendy GN, . Regulation of human tonsillar T-cell proliferation by the active metabolite of vitamin D3. Immunology 1986;59:479–484.
- Provvedini DM, Manolagas SC. 1 Alpha,25-dihydroxyvitamin D3 receptor distribution and effects in subpopulations of normal human T lymphocytes. J Clin Endocrinol Metab 1989;68: 774–779.
- Karmali R, Hewison M, Rayment N, Farrow SM, Brennan A, Katz DR, . 1,25(OH)2D3 regulates c-myc mRNA levels in tonsillar T lymphocytes. Immunology 1991;74: 589–593.
- Lemire JM, Archer DC, Beck L, Spiegelberg HL. Immunosuppressive actions of 1,25-dihydroxyvitamin D3: preferential inhibition of Th1 functions. J Nutr 1995;125: 1704S–1708S.
- Overbergh L, Decallonne B, Waer M, Rutgeerts O, Valckx D, Casteels KM, . 1alpha,25-dihydroxyvitamin D3 induces an autoantigen-specific T-helper 1/T-helper 2 immune shift in NOD mice immunized with GAD65 (p524 - 543). Diabetes 2000;49:1301–1307.
- Boonstra A, Barrat FJ, Crain C, Heath VL, Savelkoul HF, O'Garra A. 1alpha,25-Dihydroxyvitamin D3 has a direct effect on naive CD4(+) T cells to enhance the development of Th2 cells. J Immunol 2001;167:4974–4980.
- Harrington LE, Mangan PR, Weaver CT. Expanding the effector CD4 T-cell repertoire: the Th17 lineage. Curr Opin Immunol 2006;18:349–356.
- Weaver CT, Hatton RD, Mangan PR, Harrington LE. IL-17 family cytokines and the expanding diversity of effector T cell lineages. Annu Rev Immunol 2007;25:821–852.
- Bettelli E, Korn T, Kuchroo VK. Th17: the third member of the effector T cell trilogy. Curr Opin Immunol 2007;19: 652–657.
- Korn T, Oukka M, Kuchroo V, Bettelli E. Th17 cells: effector T cells with inflammatory properties. Semin Immunol 2007;19:362–371.
- Chang SH, Chung Y, Dong C. Vitamin D suppresses Th17 cytokine production by inducing C/EBP homologous protein (CHOP) expression. J Biol Chem 2010;285: 38751–38755.
- Colin EM, Asmawidjaja PS, van Hamburg JP, Mus AM, van Driel M, Hazes JM, van Leeuwen JP, Lubberts E. 1, 25-dihydroxyvitamin D3 modulates Th17 polarization and interleukin-22 expression by memory T cells from patients with early rheumatoid arthritis. Arthritis Rheum 2010;62: 132–142.
- Palmer MT, Lee YK, Maynard CL, Oliver JR, Bikle DD, Jetten AM, . Lineage-specific effects of 1, 25-dihydroxyvitamin D(3) on the development of effector CD4 T cells. J Biol Chem 2010;286:997–1004.
- Daniel C, Sartory NA, Zahn N, Radeke HH, Stein JM. Immune modulatory treatment of trinitrobenzene sulfonic acid colitis with calcitriol is associated with a change of a T helper (Th) 1/Th17 to a Th2 and regulatory T cell profile. J Pharmacol Exp Ther 2008;324:23–33.
- Liu N, Nguyen L, Chun RF, Lagishetty V, Ren S, Wu S, . Altered endocrine and autocrine metabolism of vitamin D in a mouse model of gastrointestinal inflammation. Endocrinology 2008;149:4799–4808.
- Gorman S, Kuritzky LA, Judge MA, Dixon KM, McGlade JP, Mason RS, . Topically applied 1,25-dihydroxyvitamin D3 enhances the suppressive activity of CD4 + CD25 + cells in the draining lymph nodes. J Immunol 2007;179: 6273–6283.
- Gregori S, Giarratana N, Smiroldo S, Uskokovic M, Adorini L. A 1alpha,25-dihydroxyvitamin D(3) analog enhances regulatory T-cells and arrests autoimmune diabetes in NOD mice. Diabetes 2002;51:1367–1374.
- Mathieu C, Badenhoop K. Vitamin D and type 1 diabetes mellitus: state of the art. Trends Endocrinol Metab 2005;16:261–266.
- Spach KM, Nashold FE, Dittel BN, Hayes CE. IL-10 signaling is essential for 1,25-dihydroxyvitamin D3-mediated inhibition of experimental autoimmune encephalomyelitis. J Immunol 2006;177:6030–6037.
- Jeffery LE, Burke F, Mura M, Zheng Y, Qureshi OS, Hewison M, . 1,25-Dihydroxyvitamin D(3) and IL-2 combine to inhibit T cell production of inflammatory cytokines and promote development of regulatory T cells expressing CTLA-4 and FoxP3. J Immunol 2009;183: 5458–5467.
- Urry Z, Xystrakis E, Richards DF, McDonald J, Sattar Z, Cousins DJ, . Ligation of TLR9 induced on human IL-10-secreting Tregs by 1alpha,25-dihydroxyvitamin D3 abrogates regulatory function. J Clin Invest 2009;119:387–398.
- Gregori S, Casorati M, Amuchastegui S, Smiroldo S, Davalli AM, Adorini L. Regulatory T cells induced by 1 alpha,25-dihydroxyvitamin D3 and mycophenolate mofetil treatment mediate transplantation tolerance. J Immunol 2001;167:1945–1953.
- Dong X, Bachman LA, Kumar R, Griffin MD. Generation of antigen-specific, interleukin-10-producing T-cells using dendritic cell stimulation and steroid hormone conditioning. Transpl Immunol 2003;11:323–333.
- Adorini L, Penna G, Giarratana N, Roncari A, Amuchastegui S, Daniel KC, . Dendritic cells as key targets for immunomodulation by Vitamin D receptor ligands. J Steroid Biochem Mol Biol 2004;89–90:437–441.
- Veldman CM, Cantorna MT, DeLuca HF. Expression of 1,25-dihydroxyvitamin D(3) receptor in the immune system. Arch Biochem Biophys 2000;374:334–338.
- Iho S, Iwamoto K, Kura F, Okuno Y, Takahashi T, Hoshino T. Mechanism in 1,25(OH)2D3-induced suppression of helper/suppressor function of CD4/CD8 cells to immunoglobulin production in B cells. Cell Immunol 1990;127:12–25.
- Yu S, Bruce D, Froicu M, Weaver V, Cantorna MT. Failure of T cell homing, reduced CD4/CD8alphaalpha intraepithelial lymphocytes, and inflammation in the gut of vitamin D receptor KO mice. Proc Natl Acad Sci USA 2008;105:20834–20839.
- Sigmundsdottir H, Pan J, Debes GF, Alt C, Habtezion A, Soler D, . DCs metabolize sunlight-induced vitamin D3 to ‘program’ T cell attraction to the epidermal chemokine CCL27. Nat Immunol 2007;8:285–293.
- Topilski I, Flaishon L, Naveh Y, Harmelin A, Levo Y, Shachar I. The anti-inflammatory effects of 1, 25-dihydroxyvitamin D3 on Th2 cells in vivo are due in part to the control of integrin-mediated T lymphocyte homing. Eur J Immunol 2004;34:1068–1076.
- Meehan TF, DeLuca HF. CD8(+) T cells are not necessary for 1 alpha,25-dihydroxyvitamin D(3) to suppress experimental autoimmune encephalomyelitis in mice. Proc Natl Acad Sci U S A 2002;99:5557–5560.
- Cantorna MT, Hayes CE, DeLuca HF. 1, 25-Dihydroxyvitamin D3 reversibly blocks the progression of relapsing encephalomyelitis, a model of multiple sclerosis. Proc Natl Acad Sci U S A 1996;93:7861–7864.
- Shiozawa K, Shiozawa S, Shimizu S, Fujita T. 1 alpha, 25-dihydroxyvitamin D3 inhibits pokeweed mitogen-stimulated human B-cell activation: an analysis using serum-free culture conditions. Immunology 1985;56:161–167.
- Provvedini DM, Tsoukas CD, Deftos LJ, Manolagas SC. 1 alpha,25-Dihydroxyvitamin D3-binding macromolecules in human B lymphocytes: effects on immunoglobulin production. J Immunol 1986;136:2734–2740.
- Chen S, Sims GP, Chen XX, Gu YY, Chen S, Lipsky PE. Modulatory effects of 1,25-dihydroxyvitamin D3 on human B cell differentiation. J Immunol 2007;179:1634–1647.
- Takahashi K, Nakayama Y, Horiuchi H, Ohta T, Komoriya K, Ohmori H, . Human neutrophils express messenger RNA of vitamin D receptor and respond to 1alpha, 25-dihydroxyvitamin D3. Immunopharmacol Immunotoxicol 2002;24:335–347.
- Sorensen O, Cowland JB, Askaa J, Borregaard N. An ELISA for hCAP-18, the cathelicidin present in human neutrophils and plasma. J Immunol Methods 1997;206:53–59.