Abstract
Background. Whole blood viscoelastic tests such as the fibrin-based thromboelastometry (ROTEM®) test FIBTEM are increasingly used in the perioperative setting to quickly identify deficits in fibrin quality, and to guide hemostatic therapy. The recently developed FibScreen2 test of the ReoRox® method, based on free oscillation rheometry, also provides an evaluation of fibrin clot quality. To date, little information is available on the performance of this test in hemodiluted blood, by comparison to FIBTEM. Methods. Whole blood samples from eight healthy volunteers were analyzed using FIBTEM and Fibscreen2. Native and diluted (to 33% and 50% using saline, gelatin or hydroxyethyl starch [HES]) samples were analyzed. Clot strength parameters, including FIBTEM maximum clot firmness (MCF), FIBTEM maximum clot elasticity (MCE) and Fibscreen2 maximum elasticity (G'max), were measured. Results. In repeatedly measured samples from two volunteers, FIBTEM MCF and Fibscreen2 G'max revealed a coefficient of variation (CV) of 5.3 vs. 16.3% and 5.6 vs. 31.7% for each volunteer, respectively. Hemodilution decreased clot strength. Both Fibscreen2 G'max and FIBTEM parameters decreased proportionally to the dilution ratio when saline was used. The observed reductions in FIBTEM and Fibscreen2 parameters were more severe in samples diluted with gelatin and HES, compared to saline. Finally, a regression analysis between FIBTEM MCE and Fibscreen2 G'max revealed a poor goodness of fit (r2 = 0.37, p < 0.0001). Conclusions. ReoRox® Fibscreen2 test has a high coefficient of variation, and its application in various hemodilution conditions showed limited comparability with the ROTEM® FIBTEM test.
Introduction
Thromboelastometry (ROTEM®, Tem International GmbH, Germany) is a viscoelastic test (VET) [Citation1] which measures the viscoelastic properties of clot formation in whole blood under low shear conditions. With this technique, a pin is immersed in a blood sample; the pin oscillates slowly and when coagulation is initiated (either naturally or by the addition of an activating reagent), fibrin strands form between the pin and the wall of the cup. The strands exert a force on the pin, and the amplitude of the pin's movement is recorded through a specific measurement system. The output, visualized as a curve over time, provides real-time information on the rapidity of coagulation initiation, kinetics of clot growth, clot strength and breakdown [Citation2].
Whole blood clot strength is dependent on both platelets and polymerized fibrin [Citation3–5]. While extrinsically activated VET (such as ROTEM® EXTEM) measure whole blood clotting, other tests contain inhibitors of specific components contributing to the formation, maintenance and lysis of the clot. For example, fibrinogen polymerization tests (such as ROTEM® FIBTEM) eliminate the contribution of platelets to the whole blood clot, enabling the assessment of the properties of the fibrin-based clot and effectively distinguishing between various types of coagulopathies, such as dilutional coagulopathy or thrombocytopenia [Citation6]. FIBTEM has been shown to reliably assess the quality of the fibrin-based clot in various clinical settings [Citation7–10], and is often used in theragnostic algorithms to guide fibrinogen supplementation in bleeding patients [Citation11–15].
A novel VET (ReoRox®, Medirox AB, Sweden) based on free oscillation rheometry has been developed and made commercially available. Here, the cup containing the blood sample is set into free oscillation by a torsion wire device and the damping and frequency of the change in the oscillating movement is recorded and translated by the system into viscous and elastic parameters, respectively () [Citation16–19]. Compared to ROTEM®, free oscillation rheometry allows for the detection of a wider range of elasticity changes [Citation20]. By using a platelet inhibitor in addition to the coagulation activator thromboplastin, it is possible to measure the quality of the fibrin-based clot with the ReoRox® method. The ReoRox® Fibscreen2 test contains the platelet inhibitor abciximab, and provides an evaluation of the fibrin-based clot, comparable to the thromboelastometry FIBTEM test, which contains cytochalasin D as platelet inhibiting agent () [Citation20].
Figure 1. ReoRox® and ROTEM® output traces. The ReoRox® device displays information on viscosity (dashed curve) and elasticity (plain curve) during the coagulation process (A). Viscosity parameters include the time of the initial formation of fibrin strands (COT1) and the time to complete clot formation (COT2) before the clot starts to strengthen. Clot strength is represented by the maximum elasticity parameter G'max obtained either in the absence (Fibscreen1 G'max) or presence (Fibscreen2 G'max) of platelet inhibitor. Similar coagulation parameters are obtained with the ROTEM® system (B), the equivalent of COT2 being the clotting time (CT, time from start to 2 mm amplitude). Maximum clot firmness (MCF) parameters, obtained either in the absence (EXTEM test) or presence (FIBTEM test) of platelet inhibitor, can be converted into maximum clot elasticity (MCE) parameters, using the following formula: MCE = (MCF × 100)/(100 − MCF).
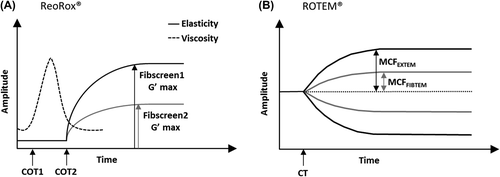
Currently, only one study has compared ROTEM® and ReoRox® in a clinical setting [Citation16]. In this prospective study, involving 40 trauma patients, clot property parameters were found to correlate between the two systems, including clot strength parameters in the presence of platelet inhibitor (ROTEM® FIBTEM maximum clot firmness [MCF] and ReoRox® Fibscreen2 maximum elasticity [G'max]). In addition, the maximum elasticity data was shown to correlate with the patients’ plasma fibrinogen levels. The intra-assay variability in the results, however, was not assessed. Other studies have compared the ROTEM® and ReoRox® systems using blood samples of healthy volunteers to demonstrate that both viscoelastic devices can be used to monitor fibrinolysis and investigate the effects of hemodilution on clot formation [Citation20,Citation21] and knowledge to date suggests higher coefficients of variation (CV) than predicted by the manufacturer for the ReoRox® G'max parameter [Citation22,Citation23].
With TEG® and ROTEM® already included in guidelines for bleeding management and the increasing number of studies now employing free oscillation rheometry, it is critical to evaluate this new viscoelastic method and establish its comparability with those used more widely in clinical practice. For this purpose, we aimed to compare the ReoRox® and ROTEM® systems in their ability to assess the quality of the fibrin-based clot in whole blood samples. ROTEM® FIBTEM and ReoRox® Fibscreen2 were used to evaluate the contribution of fibrin to clot elasticity both in native and hemodiluted (with saline, gelatin or hydroxyethyl starch [HES]) whole blood samples.
Methods
Design
A total of eight volunteers, considered healthy according to the American Society of Anesthesiology (ASA) physical status classification system, gave their written informed consent to participate in this study. All individuals had a normal coagulation history and had not been administered coagulation-influencing drugs within seven days before blood sampling. A single blood sample per volunteer was drawn from the cubital vein, without stasis, by the investigators at the AUVA trauma center (Salzburg, Austria). To avoid excess of tissue factor, the first 2–3 mL of each draw was discarded and blood samples were collected into citrate tubes. This study received approval from the local Ethics Committee (E-1231 Votum).
Viscoelastic tests
Thromboelastometry (ROTEM®). The ROTEM® delta device (Tem International GmbH, Munich, Germany) was used to perform the FIBTEM test following the manufacturer's recommendations. Briefly, after preparation of the cup, pin, reagents and tips and setting of the appropriate channels, 20 μL of the r ex-tem® reagent (recombinant tissue factor, phospholipids and the heparin inhibitor, hexadimethrine) and 20 μL of the fib-tem® reagent (containing 200 mM CaCl2 and cytochalasin D, a platelet inhibitor) was added to the cup, followed by 300 μL of citrated blood. The test was then initiated by automatic pipetting (out and back into the cup) of 340 μL of the reaction mixture, followed by positioning of the cup onto the pin. Clot amplitude at 5, 10 and 20 min (A5, A10 and A20, respectively) and maximum clot firmness (MCF) were directly measured, in mm, by the device. The maximum clot elasticity (MCE; derived from the MCF) provides a better representation of the clot strength. MCE values were automatically calculated by the device, according to the following formula: MCE = (100 × MCF)/(100 − MCF).
Free oscillation rheometry. The Fibscreen2 test (MRX1917; Medirox AB, Nyköping, Sweden) was run on the ReoRox G2® apparatus (Medirox AB) according to the manufacturer's instructions. The reaction chambers were first positioned in the rheometer then 100 μL of reagent 2® (containing the platelet inhibitor abciximab) and 25 μL of 500 mM CaCl2 were gently mixed with 1.0 mL citrated blood. Finally, 1.0 mL of the final reaction mix was slowly injected in the cup using a 1.0 mL disposable syringe. The maximum elasticity of the clot (G'max) was directly measured in Pa by the device. The normal range for Fibscreen2 G'max has been described to be 35–175 Pa [Citation19]. Values above 175 Pa can be obtained when the reaction chamber has not been positioned properly or pre-heated; consequently, values above 175 Pa were not considered in our analyses.
Study groups
Stability analysis. Previous studies investigating within-run CV in free oscillation rheometry described the use of 10 replicates of two human plasma samples and one quality control sample [Citation22]. Here, we used blood samples of two healthy volunteers (volunteer 1: male, age 54 years, height 1.87 m, bodyweight 90 kg; volunteer 2: female, age 31 years, height 1.70 m, bodyweight 59 kg). Each sample was processed at least 12 times with the ROTEM® FIBTEM and ReoRox® Fibscreen2 tests.
Effect of in vitro hemodilution. At the time of its design, as this was the first study to describe the effect of in vitro hemodilution on clotting parameters obtained with the ReoRox® device, we based our sample size on studies undertaken with other viscoelastic methods that used blood samples from 5–12 volunteers, processed once each [Citation24–28]. We used blood samples of six healthy volunteers (three males and three females). Their age, height and bodyweight range were as follows: 31–55 years, 1.65–1.87 m, and 59–90 kg. Samples were used native and diluted (33% and 50% dilution) with saline (sodium chloride 0.9% solution for injection; Fresenius Kabi AG, Bad Homburg, Germany), gelatin (4% succinylated gelatin solution; Gelofusin, Braun Melsungen, Melsungen, Germany) or HES (6% HES 130/0.4; Voluven, Fresenius Kabi, Graz, Austria). Each sample was processed in duplicate with both the ROTEM® FIBTEM and ReoRox® Fibscreen2 tests (n = 12).
Statistical analyses
Data distribution normality was assessed by the Kolmogorov-Smirnov test. Differences between parameters obtained from volunteers 1 and 2 were assessed using the Mann-Whitney test. The CV was calculated for intra-individual measurement system stability of each test as well as for overall comparison of accuracy between the two tests in assessing hemodiluted samples. To show the decrease in fibrin-based clot properties after several dilutions, the Kruskal-Wallis one-way ANOVA was used, and a Dunn's post hoc correction for multiple comparisons was applied. Finally, the Spearman correlation coefficient between the two tests was calculated, as well as the goodness of fit of linear regression analysis, assessed between elasticity parameters. All statistical analyses were performed using GraphPad Prism 5 (GraphPad Software, La Jolla, CA, USA). The results are expressed as median and interquartile range (IQR, 25th percentile – 75th percentile) and range (minimum to maximum). A two-tailed p-value < 0.05 was considered significant.
Results
Measurement system stability analyses
As shown in , multiple measurements of native blood samples from two volunteers, revealed that the intra-assay variation was lower with the FIBTEM measurements (A5 CV, 4.7% and 5.6% for each volunteer's blood samples; A10 CV, 4.6% and 5.3%; A20 CV, 4.8% and 5.7%; MCF CV, 5.3% and 5.6%; MCE CV, 5.5% and 6.3%) compared with the Fibscreen2 G'max (CV, 16.3% and 31.7% for each volunteer's blood samples).
Table I. Stability analysis in re-calcified citrated blood samples. Stability of fibrin-based clot strength parameters of blood samples from two healthy volunteers was assessed (with a minimum of 12 measurements each) with the ROTEM® FIBTEM and ReoRox® Fibscreen2 tests.
Moreover, the FIBTEM test showed small, but significant, differences between the two volunteers’ MCE (p < 0.0001, ) and similar findings were observed with the FIBTEM A5 (p = 0.0024), A10, A20 and MCF parameters (p < 0.0001). This difference between volunteers however, was not observed between the G'max values (p = 0.66, ) obtained with the Fibscreen2 test.
Effect of in vitro hemodilution with saline, gelatin or HES
Using blood samples from six volunteers, FIBTEM and Fibscreen2 data showed a decrease in fibrin-based clot strength as a consequence of hemodilution, in samples diluted with saline, gelatin or HES (). The decrease in the median FIBTEM MCF was proportional to the dilution when saline was used, with a 50% decrease when samples were diluted by 50% with saline (). A comparable behavior was observed with most other FIBTEM parameters: A10, A20, and MCE parameters decreased by 57.1%, 53.3% and 54.3%, respectively, and the A5 was decreased by 64.3% in samples diluted by 50% with saline. Similarly, the median Fibscreen2 G'max decreased by 57.1% in samples diluted by 50% with saline (). In contrast, FIBTEM and Fibscreen2 parameters were more affected by hemodilution when gelatin or HES was used, with decreases ranging from 71.0% (Fibscreen2 G'max) to 78.6% (FIBTEM A5 and A10) in samples diluted by 50% with gelatin and from 79.1% (Fibscreen2 G'max) to 88.6% (FIBTEM MCE) in samples diluted by 50% with HES (; ).
Figure 2. Comparison of functional fibrinogen in undiluted and diluted re-calcified citrated blood samples obtained with the ROTEM® and ReoRox® devices. Blood samples were diluted at 33% and 50% with saline (NaCl), gelatin (GEL) and hydroxyethyl starch (HES). Duplicates of each dilution of blood samples from six different volunteers were evaluated with (A) the derived FIBTEM maximum clot elasticity (MCE) and (B) Fibscreen2 maximum elasticity (G'max). For each group, the median is represented by a bar within a box (extending from the 25th to 75th percentiles). Whiskers extend from the smallest to the largest values.
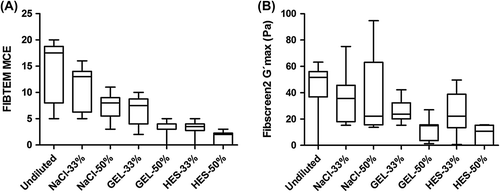
Table II. Fibrin-based clot strength parameters of citrated undiluted and hemodiluted blood samples. Undiluted and hemodiluted (33% and 50% with saline, gelatin or hydroxyethyl starch [HES]) blood of samples of healthy volunteers (n = 6) were assessed with the ROTEM® FIBTEM (A5, A10, A20, MCF, MCE) and ReoRox® Fibscreen2 (G'max) tests. Values are presented as median [25th percentile – 75th percentile].
Finally, shows a significant correlation between FIBTEM MCE and Fibscreen2 G'max (Spearman's rho = 0.62, p < 0.0001); however, the goodness of fit of linear regression was found to be low (Pearson's r2 = 0.37, p < 0.0001).
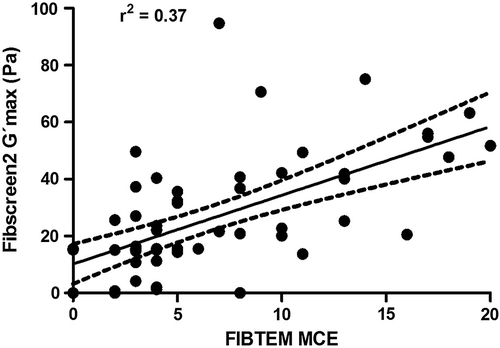
Discussion
This study showed that the measurement of clot elasticity under platelet inhibition in whole blood samples appears to be more precise with the classical thromboelastometric FIBTEM test than with the recently introduced ReoRox® FibScreen2 test. This was shown by our intra-individual stability data with low CVs, in the range of 5.3–5.6%, for the FIBTEM test, in line with a previous publication describing a CV < 6% in the measurement of FIBTEM MCF [Citation29]. The ReoRox® Fibscreen2 test was found to have higher CVs ranging from 16.3–31.7%, above the previously described value of 13.3% for samples assessed in the presence of abciximab [Citation23]. Fibrin-based tests have previously been shown to exhibit higher within-run CVs than tests without platelet inhibitors with the ROTEM® device [Citation30]; a similar conclusion can be drawn for the ReoRox® device as work from Pederson and colleagues revealed within-run CVs below 3% in samples without abciximab measured on ReoRox® [Citation22].
In a recent study investigating the correlation between ReoRox® Fibscreen2 G'max and ROTEM® FIBTEM MCF, the authors showed that ReoRox® is able to isolate and depict the contribution of fibrin to clot formation in whole blood from severely injured trauma patients [Citation16]. The authors demonstrated that the MCF significantly correlated with G'max (Spearman's correlation coefficient rho = 0.69, p < 0.001). We chose to use MCE as both MCE and G'max are a measure of elasticity and we found a very similar correlation in the present study (rho = 0.62, p < 0.0001) between MCE and G'max. Free oscillation rheometry has not currently been integrated in hemostatic therapy algorithms; however, based on our results, it may be possible to calculate ReoRox® G'max trigger values equivalent to published FIBTEM MCF triggers. For example, a recent ROTEM®-based algorithm suggests a FIBTEM MCF < 8 mm (or MCE < 8.7) for fibrinogen concentrate administration in children undergoing elective major craniofacial surgery [Citation11]. Using our correlation data (), this trigger would be the equivalent of a G'max < 31. Similarly, a FIBTEM A10 lower than 7 mm, suggested as a trigger for fibrinogen supplementation in a trauma-induced coagulopathy setting [Citation12], would be the equivalent of a G'max < 28. However, a prospective evaluation appears mandatory before ReoRox®-based algorithms become integrated in clinical practice. Indeed, our data show a low goodness of fit (r2 = 0.37, p < 0.0001) between MCE and G'max, raising concerns about the potential use of free oscillation rheometry for the guidance of fibrinogen supplementation in bleeding patients as compared to currently established VETs for such settings and algorithms.
Hemodilution is well known to affect clotting parameters, and clot strength was shown to be more severely affected by colloids compared with crystalloids [Citation25–28,Citation31]. As previously described, dilution with saline led to proportional decreases of both Fibscreen2 G'max and FIBTEM parameters [Citation19,Citation25]. With colloids being particularly detrimental to fibrin polymerization, as demonstrated by both in vitro and clinical studies [Citation32–37], our results showed that dilution with HES led to further decreased MCF read-outs (− 76.7% and − 86.7% in 33% and 50% diluted samples, respectively) (). Similarly, as previously shown, Fibscreen2 G'max values also reflected the severe effects of HES on fibrin polymerization with a 79.1% decrease in samples diluted by 50% with HES [Citation19]. The decrease of the Fibscreen2 G'max was lower than for the FIBTEM MCF, corroborating the results of Tynngård et al. who found that a 10% HES in vitro dilution led to a lower decrease of the Fibscreen1 G'max compared to ROTEM® EXTEM MCF [Citation20].
Numerous studies have described the use of TEG® or ROTEM® in both research and clinical/perioperative settings and these devices have proven to be useful for the rapid assessment of hemostasis in patients with coagulopathy, in settings such as cardiovascular surgery, trauma and liver transplantation [Citation15,Citation38–42]. The ReoRox® system provides separate information on both elastic and viscous parameters of the whole blood clot, in the absence (Fibscreen1 test) and presence (Fibscreen2 test) of platelet inhibition [Citation19,Citation20]. The Fibscreen1 test is usually used to report the viscosity parameter COT1 as well as COT2 and the elasticity parameter G'max (the latter two parameters are equivalent to CT and MCE with the ROTEM® device). Both the FIBTEM and Fibscreen2 tests are used to investigate the fibrin-based clot properties and only the FIBTEM MCF/MCE and Fibscreen2 G'max elasticity parameters are generally reported for these tests. We therefore focused our analyses on these elasticity parameters in both tests and found that the ReoRox® Fibscreen2 test does not seem to reach the precision obtained with the ROTEM® FIBTEM test. Due to the different mechanical principles and measurement units, a direct comparison of ROTEM® MCF/MCE with ReoRox® G'max is not possible and therefore, it is not feasible to calculate the limits of agreement according to Bland and Altman [Citation43].
Previous studies comparing ReoRox® and ROTEM® have indicated that Fibscreen1 G'max was more influenced by platelets than by fibrinogen or FXIII, compared to its equivalent EXTEM MCF [Citation19]. Compared to ROTEM® which provides clotting information under minimal shear rate (0.1/sec) [Citation44], the ReoRox® system runs at a fixed shear rate of 69/sec, similar to the level of shear produced by the venous flow [Citation45]. Because platelets have been described as activated by increased shear conditions [Citation46], the difference in shear rate between the devices might suggest higher platelet activation in samples assessed with ReoRox®, compared to ROTEM®. However, this technical difference might not be considered an issue when comparing the results obtained with both systems as previous studies demonstrated that the concentration of abciximab used in the Fibscreen2 test is at saturating level, higher than the level above which no further effect on elasticity parameters can be observed [Citation23].
Previous studies showed that neither abciximab nor cytochalasin D has an effect on clot firmness in platelet-free plasma samples [Citation47]. It is currently unclear whether the potency of abciximab versus cytochalasin D to inhibit platelet function could contribute to the differences in CV observed between the Fibscreen2 and FIBTEM tests, because both inhibitors have different mechanisms of action [Citation37]. Investigations by Lang et al. revealed that neither abciximab nor cytochalasin D could achieve a full blockage of platelet function and that combining both compounds would provide a more effective way of eliminating platelet contribution to the formation of the clot [Citation4]. A similar conclusion was recently reached by Schlimp and collaborators who also observed that, compared to abciximab based-functional fibrinogen tests, those using cytochalasin D are more effective at inhibiting platelet contribution to clot strength in whole blood samples [Citation5].
In our study, 10 Fibscreen2 G’ max measurements had to be discarded post-hoc because they were above the appropriate reference range (35–175 Pa), while no FIBTEM measurements were excluded from the analysis. This might be considered as a limitation of the ReoRox® system as in a clinical situation, one may assume that some Fibscreen2 measurements might need to be run again, which would be less likely to be the case with FIBTEM measurements.
To conclude, this study shows that fibrin-based clot elasticity can be assessed with both the ROTEM® FIBTEM and ReoRox® Fibscreen2 tests in healthy volunteers; however, the Fibscreen2 test shows higher CVs than expected. Nevertheless, both tests reflected elasticity impairment induced by hemodilution with colloids beyond the hemodilution level.
Acknowledgements
Editorial assistance with manuscript preparation was provided by medical writers at Meridian HealthComms (Plumley, UK), funded by CSL Behring.
Ethical approval
This study received approval of the local Ethics Committee (E-1231 Votum), Sebastian-Stief-Gasse 2, 5020, Salzburg, Austria.
Contributions of authors
CS and HS designed the study and performed the experiments. CS and CJS performed the statistical analysis. All authors analyzed and interpreted the results. CS and CJS drafted the manuscript. HS, MR, and US critically revised the manuscript for important intellectual content. All authors attest to the integrity of the original data and the analysis reported in this manuscript. The final manuscript was approved by all authors, who all qualify for authorship.
Declaration of interest: Cristina Solomon is an employee of CSL Behring and previously received speaker honoraria and research support from Tem International and CSL Behring, and travel support from Haemoscope Ltd (former manufacturer of TEG®). Herbert Schöchl has received study grants and speaker fees from CSL Behring and Tem International. Marco Ranucci received speaker honoraria and research support from CSL Behring and Grifols, speaker honoraria from Medtronic and Haemoscope, research support from Tem International, and was on the steering committee of a FXIII study (until 2010) for Novo Nordisk. Ulf Schött has received grants from CSL Behring. Christoph J. Schlimp has received research support and speaker fees from CSL Behring, and research support from Tem International. The authors report no other conflicts of interest. The authors alone are responsible for the content and writing of the paper.
References
- Schöchl H, Voelckel W, Grassetto A, Schlimp CJ. Practical application of point-of-care coagulation testing to guide treatment decisions in trauma. J Trauma Acute Care Surg 2013;74:1587–98.
- Ganter MT, Hofer CK. Coagulation monitoring: current techniques and clinical use of viscoelastic point-of-care coagulation devices. Anesth Analg 2008;106:1366–75.
- Kalina U, Stohr HA, Bickhard H, Knaub S, Siboni SM, Mannucci PM, Peyvandi F. Rotational thromboelastography for monitoring of fibrinogen concentrate therapy in fibrinogen deficiency. Blood Coagul Fibrinolysis 2008;19:777–83.
- Lang T, Toller W, Gutl M, Mahla E, Metzler H, Rehak P, Marz W, Halwachs-Baumann G. Different effects of abciximab and cytochalasin D on clot strength in thrombelastography. J Thromb Haemost 2004;2:147–53.
- Schlimp CJ, Solomon C, Ranucci M, Hochleitner G, Redl H, Schöchl H. The effectiveness of different functional fibrinogen polymerization assays in eliminating platelet contribution to clot strength in thromboelastometry. Anesth Analg 2014;118:269–76.
- Larsen OH, Fenger-Eriksen C, Christiansen K, Ingerslev J, Sorensen B. Diagnostic performance and therapeutic consequence of thromboelastometry activated by kaolin versus a panel of specific reagents. Anesthesiology 2011;115:294–302.
- Solomon C, Cadamuro J, Ziegler B, Schöchl H, Varvenne M, Sorensen B, Hochleitner G, Rahe-Meyer N. A comparison of fibrinogen measurement methods with fibrin clot elasticity assessed by thromboelastometry, before and after administration of fibrinogen concentrate in cardiac surgery patients. Transfusion 2011;51:1695–706.
- Rugeri L, Levrat A, David JS, Delecroix E, Floccard B, Gros A, Allaouchiche B, Negrier C. Diagnosis of early coagulation abnormalities in trauma patients by rotation thrombelastography. J Thromb Haemost 2007;5:289–95.
- Roullet S, Pillot J, Freyburger G, Biais M, Quinart A, Rault A, Revel P, Sztark F. Rotation thromboelastometry detects thrombocytopenia and hypofibrinogenaemia during orthotopic liver transplantation. Br J Anaesth 2010;104:422–8.
- Huissoud C, Carrabin N, Benchaib M, Fontaine O, Levrat A, Massignon D, Touzet S, Rudigoz RC, Berland M. Coagulation assessment by rotation thrombelastometry in normal pregnancy. Thromb Haemost 2009;101:755–61.
- Haas T, Goobie S, Spielmann N, Weiss M, Schmugge M. Improvements in patient blood management for pediatric craniosynostosis surgery using a ROTEM-assisted strategy – feasibility and costs. Paediatr Anaesth 2014;24:774–80.
- Schöchl H, Schlimp CJ. Trauma bleeding management: the concept of goal-directed primary care. Anesth Analg 2014;119:1064–73.
- Rahe-Meyer N, Hanke A, Schmidt DS, Hagl C, Pichlmaier M. Fibrinogen concentrate reduces intraoperative bleeding when used as first-line hemostatic therapy during major aortic replacement surgery: results from a randomized, placebo-controlled trial. J Thorac Cardiovasc Surg 2013;145:S178–85.
- Solomon C, Pichlmaier U, Schoechl H, Hagl C, Raymondos K, Scheinichen D, Koppert W, Rahe-Meyer N. Recovery of fibrinogen after administration of fibrinogen concentrate to patients with severe bleeding after cardiopulmonary bypass surgery. Br J Anaesth 2010;104:555–62.
- Noval-Padillo JA, Leon-Justel A, Mellado-Miras P, Porras-Lopez F, Villegas-Duque D, Gomez-Bravo MA, Guerrero JM. Introduction of fibrinogen in the treatment of hemostatic disorders during orthotopic liver transplantation: implications in the use of allogenic blood. Transplant Proc 2010;42:2973–4.
- Sølbeck S, Windeløv NA, Baek NH, Nielsen JD, Ostrowski SR, Johansson PI. In-vitro comparison of free oscillation rheometry (ReoRox) and rotational thromboelastometry (ROTEM) in trauma patients upon hospital admission. Blood Coagul Fibrinolysis 2012;23:688–92.
- Tynngård N, Lindahl TL, Ramström S, Räf T, Rugarn O, Berlin G. Free oscillation rheometry detects changes in clot properties in pregnancy and thrombocytopenia. Platelets 2008;19:373–8.
- Ramström S, Ranby M, Lindahl TL. The role of platelets in blood coagulation – effects of platelet agonists and GPIIb/IIIa inhibitors studied by free oscillation rheometry. Thromb Res 2002;105:165–72.
- Winstedt D, Tynngård N, Olanders K, Schött U. Free oscillation rheometry monitoring of haemodilution and hypothermia and correction with fibrinogen and factor XIII concentrates. Scand J Trauma Resusc Emerg Med 2013;21:20.
- Tynngård N, Berlin G, Samuelsson A, Berg S. Low dose of hydroxyethyl starch impairs clot formation as assessed by viscoelastic devices. Scand J Clin Lab Invest 2014;74:344–50.
- Nilsson CU, Tynngård N, Reinstrup P, Engström M. Monitoring fibrinolysis in whole blood by viscoelastic instruments: a comparison of ROTEM and ReoRox. Scand J Clin Lab Invest 2013;73:457–65.
- Pedersen L, Nielsen EB, Christensen MK, Buchwald M, Nybo M. Measurement of plasma viscosity by free oscillation rheometry: imprecision, sample stability and establishment of a new reference range. Ann Clin Biochem 2013;51:495–8.
- Tynngård N, Lindahl T, Ramström S, Berlin G. Effects of different blood components on clot retraction analysed by measuring elasticity with a free oscillating rheometer. Platelets 2006;17:545–54.
- Fries D, Innerhofer P, Reif C, Streif W, Klingler A, Schobersberger W, Velik-Salchner C, Friesenecker B. The effect of fibrinogen substitution on reversal of dilutional coagulopathy: an in vitro model. Anesth Analg 2006;102:347–51.
- Schlimp CJ, Cadamuro J, Solomon C, Redl H, Schöchl H. The effect of fibrinogen concentrate and factor XIII on thromboelastometry in 33% diluted blood with albumin, gelatine, hydroxyethyl starch or saline in vitro. Blood Transfus 2013;11:510–7.
- Kind SL, Spahn-Nett GH, Emmert MY, Eismon J, Seifert B, Spahn DR, Theusinger OM. Is dilutional coagulopathy induced by different colloids reversible by replacement of fibrinogen and factor XIII concentrates?Anesth Analg 2013;117:1063–71.
- Weiss G, Lison S, Spannagl M, Heindl B. Expressiveness of global coagulation parameters in dilutional coagulopathy. Br J Anaesth 2010;105:429–36.
- Konrad CJ, Markl TJ, Schuepfer GK, Schmeck J, Gerber HR. In vitro effects of different medium molecular hydroxyethyl starch solutions and lactated Ringer's solution on coagulation using SONOCLOT. Anesth Analg 2000;90:274–9.
- Theusinger OM, Nurnberg J, Asmis LM, Seifert B, Spahn DR. Rotation thromboelastometry (ROTEM) stability and reproducibility over time. Eur J Cardiothorac Surg 2010;37:677–83.
- Lang T, Bauters A, Braun SL, Potzsch B, von Pape KW, Kolde HJ, Lakner M. Multi-centre investigation on reference ranges for ROTEM thromboelastometry. Blood Coagul Fibrinolysis 2005;16:301–10.
- Schramko A, Suojaranta-Ylinen R, Kuitunen A, Raivio P, Kukkonen S, Niemi T. Hydroxyethylstarch and gelatin solutions impair blood coagulation after cardiac surgery: a prospective randomized trial. Br J Anaesth 2010;104:691–7.
- Hiippala ST. Dextran and hydroxyethyl starch interfere with fibrinogen assays. Blood Coagul Fibrinolysis 1995;6:743–6.
- Fenger-Eriksen C, Moore GW, Rangarajan S, Ingerslev J, Sorensen B. Fibrinogen estimates are influenced by methods of measurement and hemodilution with colloid plasma expanders. Transfusion 2010;50:2571–6.
- Mittermayr M, Streif W, Haas T, Fries D, Velik-Salchner C, Klingler A, Oswald E, Bach C, Schnapka-Koepf M, Innerhofer P. Hemostatic changes after crystalloid or colloid fluid administration during major orthopedic surgery: the role of fibrinogen administration. Anesth Analg 2007;105:905–17.
- Godier A, Durand M, Smadja D, Jeandel T, Emmerich J, Samama CM. Maize- or potato-derived hydroxyethyl starches: is there any thromboelastometric difference?Acta Anaesthesiol Scand 2010;54:1241–7.
- Brummel-Ziedins K, Whelihan MF, Ziedins EG, Mann KG. The resuscitative fluid you choose may potentiate bleeding. J Trauma 2006;61:1350–8.
- Solomon C, Sorensen B, Hochleitner G, Kashuk J, Ranucci M, Schöchl H. Comparison of whole blood fibrin-based clot tests in thrombelastography and thromboelastometry. Anesth Analg 2012;114:721–30.
- Westbrook AJ, Olsen J, Bailey M, Bates J, Scully M, Salamonsen RF. Protocol based on thromboelastograph (TEG) out-performs physician preference using laboratory coagulation tests to guide blood replacement during and after cardiac surgery: a pilot study. Heart Lung Circ 2009;18:277–88.
- Rahe-Meyer N, Solomon C, Winterhalter M, Piepenbrock S, Tanaka K, Haverich A, Pichlmaier M. Thromboelastometry-guided administration of fibrinogen concentrate for the treatment of excessive intraoperative bleeding in thoracoabdominal aortic aneurysm surgery. J Thorac Cardiovasc Surg 2009;138:694–702.
- Kashuk JL, Moore EE, Wohlauer M, Johnson JL, Pezold M, Lawrence J, Biffl WL, Burlew CC, Barnett C, Sawyer M, Sauaia A. Initial experiences with point-of-care rapid thrombelastography for management of life-threatening postinjury coagulopathy. Transfusion 2012;52:23–33.
- Schöchl H, Maegele M, Solomon C, Gorlinger K, Voelckel W. Early and individualized goal-directed therapy for trauma-induced coagulopathy. Scand J Trauma Resusc Emerg Med 2012;20:15.
- Wang SC, Shieh JF, Chang KY, Chu YC, Liu CS, Loong CC, Chan KH, Mandell S, Tsou MY. Thromboelastography-guided transfusion decreases intraoperative blood transfusion during orthotopic liver transplantation: randomized clinical trial. Transplant Proc 2010;42:2590–3.
- Bland JM, Altman DG. Statistical methods for assessing agreement between two methods of clinical measurement. Lancet 1986;1:307–10.
- Schött U, Johansson PI. Bringing flow into haemostasis diagnostics. Br J Anaesth 2013;111:864–7.
- Evans PA, Hawkins K, Williams PR. Rheometry for blood coagulation studies. The British Society of Rheology 2006. Available from: http://www.bsr.org.uk/rheology-reviews/RheologyReviews/rheometry-blood-coagulation-EvansHawkinsWilliams.pdf [Accessed 15 April 2014].
- Hathcock JJ. Flow effects on coagulation and thrombosis. Arterioscler Thromb Vasc Biol 2006;26:1729–37.
- Schlimp CJ, Solomon C, Hochleitner G, Zipperle J, Redl H, Schöchl H. Thromboelastometric maximum clot firmness in platelet-free plasma is influenced by the assay used. Anesth Analg 2013;117:23–9.