Abstract
We report the establishment and characterization of immortalized human fetal liver progenitor cells by expression of the Simian virus 40 large T (SV40 LT) antigen. Well-characterized cells at various passages were transplanted into nude mice with acute liver injury and tested for functional capacity. The SV40LT antigen-immortalized fetal liver cells showed a morphology similar to primary cells. Cultured cells demonstrated stable phenotypic expression in various passages, of hepatic markers such as albumin, CK 8, CK18, transcription factors HNF-4α and HNF-1α and CYP3A/7. The cells did not stain for any of the tested cancer-associated markers. Albumin, HNF-4α and CYP3A7 expression was confirmed by reverse transcription polymerase chain reaction (RT-PCR). Flow cytometry showed expression of some progenitor cell markers. In vivo study showed that the cells expressed both fetal and differentiated hepatocytes markers. Our study suggests new approaches to expand hepatic progenitor cells, analyze their fate in animal models aiming at cell therapy of hepatic diseases.
Introduction
Cultured human fetal liver cells (hFLCs) are unique in their potential utility in understanding molecular events of cell engraftment and differentiation in cell therapy. Even though they have an extended life-span compared to adult primary cells, they lose their ability to divide and enter senescence after a finite number of population doublings. To study the in vivo fate of cells, for example, proliferation, engraftment and differentiation require large numbers of cells with reproducible quality. Thus, a renewable source of cells that is constant and can be expanded into large number is necessary. Primary cultures from explanted animal or human tissue do not fulfill such needs [Citation1–3].
In order to obtain cells with an extended replicating capacity, immortalized cells are needed. Such cells can be created by induction of oncogenes or down-regulation of tumor suppressor genes. One way to break senescence and induce immortality is through overexpression of the SV40 LT antigen [Citation4]. SV40 LT has been shown to be the simplest and most reliable agent for the transformation of many different cell types in culture, and its mechanisms of action are well studied. For the most part, viral genes achieve immortalization by inactivating tumor suppressor genes such as p53, Rb and others, which can induce a replicative senescent state in cells [Citation5].
Under standard culture condition, it is observed that human fetal hepatocytes can proliferate up to 12–14 passages before entering a growth arrest phase [Citation6] during which the cells exhibit protruded elongations with a large, more flattened and irregular shape [Citation7]. This phenotype is referred to as a marker of senescence [Citation8,9]. It has proven difficult to establish conditions to support long-term primary cultures of adult human liver. Kobayashi et al. established several immortalized hepatocyte lines derived from human fetal or nonhuman adult hepatocytes [Citation3,10]. Immortalized hepatocytes retain some of the differentiated features of normal primary hepatocytes in culture, including the expression of albumin (ALB), transferrin, hemopexin and glucose-6-phosphatase (G-6-P). Further, these cells do not produce detectable α-fetoprotein or show characteristics of fetal or abnormal liver cells [Citation3,10,11]. Similar results were obtained by the Andres research group [Citation12]. They established two immortalized hepatocyte lines from normal human liver cells following transformation with the SV40 LT antigen. These cell lines, which lacked tumorigenic properties, expressed many mature hepatocyte markers and possessed enzymatic pathways responsible for xenobiotic metabolism.
Early fetal hepatoblasts, found in the developing liver, are good candidates for generation of liver progenitor cell lines by means of conditional immortalization. Such cells will be of great interest to study the molecular events involved in their proliferation and differentiation in vitro as well as their fate in vivo after transplantation in the livers of recipient mice. Therefore, in this study, we immortalized human fetal hepatocytes and succeeded in establishing a reliable cell line, in which all the hepatic markers and hepatic transcription factors remained unaltered over several passages.
Materials and methods
hFLCs preparation and culture
Principles of Laboratory Animal Care (http://www.jordbruksverket.se/) were followed, as well as specific national laws (e.g., the current version of the Swedish Law on the Protection of Animals) where applicable. Primary hFLCs were collected from a legally aborted human fetus 6.5 weeks of gestational age. A single cell suspension was prepared as described earlier [Citation7]. Also see supplement S1.
Construction of the CMV/SV40LT/PAC plasmid
The SV40 LT cDNA was amplified by PCR from a plasmid containing its full length sequence using 5′-cgc ggg ctc gag acc atg gat aaa gtt tta aac-3′ and 5′-cgc ggg gcg gcc gct tta tgt ttc agg ttc agg-3′ as forward and reverse primers, respectively. The vector used to generate stable transfectants were bidirectional having the Spleen focus-forming virus (Sffv) long terminal repeat (Ltr) upstream of a polylinker, a splice donor and acceptor site, and the bidirectional poly(A) addition signal of SV40; opposite in orientation to this transcription unit, and utilizing the poly(A) signals from the opposite direction was a second transcription unit consisting of the HSV TK promoter followed by the coding sequences for puromycin acetyltransferase (Sffv/PAC; N. Chiu, J. Holgersson and B. Seed, unpublished). The SV40LT cDNAs was swapped into the Sffv/PAC vector using Xho I and Not I. Thereafter, the Sffv Ltr was removed and the IE CMV promoter from CDM8 cloned into the vector using Spe I and Xho I, thus creating CMV/SV40LT/PAC. Please see Supplement S1 for details of the transfection assay.
Growth assay
Stable transfected SV40 LT-HFL cells cultured for 2.5 months in passage 9 were seeded in collagen-coated 6-well plates (BD Biosciences, NJ, USA) at a density of 80,000 cells/well and the growth was followed for 7 days. Culture medium was changed every third day. At 24-h intervals, cells were detached, spun at 200 g for 5 min and cell numbers in triplicates were determined by a manual hemocytometer. Population doubling time (PDT) was calculated at the time of exponential growth (log phase), that is, between 48 h and 96 h after initial plating.
Detection of hepatic markers
Hepatic markers expressed by the transfected SV40 LT-HFL cells were determined in early and late passages using three different assays: a) immunocytochemical analysis, b) RT-PCR and c) flow cytometry (see supplement S1 for details). Liver-specific markers such as G-6-P and glycogen were demonstrated in transfected cells as described earlier [Citation13,14].
Transplantation studies
The animal care and ethics committee at Sahlgrenska University Hospital in Gothenburg, Sweden approved of the animal protocols. Liver injury was induced in 8-weeks-old nude male Balb/C mice (n = 10) by administration of D-galactosamine (GalN) (Sigma Chemicals Co., Gothenburg, Sweden) intraperitonially at 0.7 g/kg body weight [Citation15]. GalN was dissolved in phosphate-buffered saline, pH 7.4 (PBS) at 100 mg/ml. Under general anesthesia all animals underwent 30% partial hepatectomy (removal of left lobe) 36 h after GalN treatment. SV40 LT HFL cells with a viability of 95% in passage 22 were transplanted into the spleens of these animals. Animals were anesthetized under isofluorane and 2 × 106 cells in 200 µl of Dulbecco's modified eagle medium (DMEM) were injected into the spleen of Tx group (n = 5) over approximately 10–15s. However, sham group (n = 5) received only DMEM medium with no cells. After securing hemostasis, the abdominal incision was closed and the animals were monitored closely until recovery.
Post transplantation organ retrieval and analysis
Mice were sacrificed 4 weeks after transplantation and their livers, spleen and lungs were excised. The liver tissue was shock frozen in liquid nitrogen for fluorescence and immunohistochemical analyses. Cryosections, 5 µm in thickness, were air dried and fixed with cold 30% acetone in methanol for 10 min and further analyzed by immunohistochemistry.
Immunohistochemistry
The tissue slides were incubated overnight at 4°C with antibodies specific for human nuclear antigen (1:100, Millipore, Stockholm, Sweden), human c-Met (1:100, RDI, Concord, MA, USA), human α-fetoprotein (1:200), human CK8 (1:200), human CK18 (1:200), human CK19 (1:200), human hepatocyte-specific antigen (1:100, all antibodies from AH diagnostics) and anti-p53 (1:100, antibodies-online GmbH) and anti-BER EP4 (Dako). The rest of the procedure is described by us in detail elsewhere [Citation7]. For details, please see supplement S1.
ELISA
Human albumin concentration in serum samples of Tx, sham and normal animal groups was measured using ELISA (enzyme-linked immunosorbent assay) Quantitation Kit (Bethyl Laboratories Inc, Montgomery, TX) according to the manufacturer's protocol. Absorbance was read at 450 nm using an ELISA plate reader (Synergy H4, Hybrid Reader, Biotek). Also see supplement 1.
Results
Generation and growth of SV40 LT antigen-immortalized hFLCs
Colonies of cells grew in the selection marker puromycin and these cells were passaged onto new culture flasks and handled as separated bulk clones. So far, we have investigated cells in one of the bulk clones. To investigate whether immortalized cells retain the morphologic characteristics of primary liver cells, the cells were examined by phase contrast microscopy (). Immortalized hepatocytes grew in clusters of closely apposed cells of typical morphology including large size, poly- or hexagonal shape and with more than one nucleus ().
Figure 1. Phase contrast microscopy of SV40LT-HFL cells. (A) Derived from one colony after 13 days of culture in medium with puromycin, (B) from a single colony after 2 months with puromycin selection, (C) showing cells with two or three nuclei, typical of differentiating hepatocytes and (D) control adult hepatocytes. Scale bar A, B & D = 10 µm, C = 40 µm.
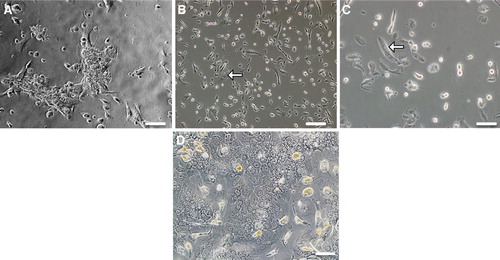
Following plating of SV40 LT-HLF cells, there was a lag phase of 0–48 h before the cells grew exponentially. The population doubling time was 30.8 for cells cultured for 2.5 months. The cultures were confluent after 3 days and the cell numbers dropped following another 3 days in culture. There was a relative quick decrease in cell numbers when the culture reached confluence indicating that the stable transfected cells in the SV40 LT line inhibit growth via contact inhibition as normal cells do.
Phenotypic characterization of SV40 LT antigen-immortalized hFLCs
Expression of hepatic markers
Detection by immunocytochemistry of human hepatocyte-specific proteins in transfected fetal hepatocytes revealed expression of albumin, both in early (p12) as well as late (p22) passage cells. The cells also expressed hepatocyte marker proteins, CK8 and CK18, as clear cytoplasmic filamentous structures (). In general, the staining was distributed equally in almost all the cells both in early and late passages.
Figure 2. Characterization of SV40LT-HFL cells. Immunofluorescent staining of SV40LT-HFL cells in passages 12 (left) and 22 (right) showing in A, from left to right, the detection of cytoplasmic staining of hepatic markers CK8, 18 and albumin. The level of expression as judged from the intensity of staining was similar in both passages. In B, the same cells are stained with the nuclear stain, DAPI. In A, from left to right, positive cytoplasmic staining of CYP3A4/7 and nuclear staining of transcription factors HNF-1α and HNF-4α is seen in all cells. In B, the same cells are stained with the nuclear stain, DAPI. In C, a small number of cells staining positive for the hepato-biliary marker CK19 was detected in both early and late passages. (Magnifications 40×)
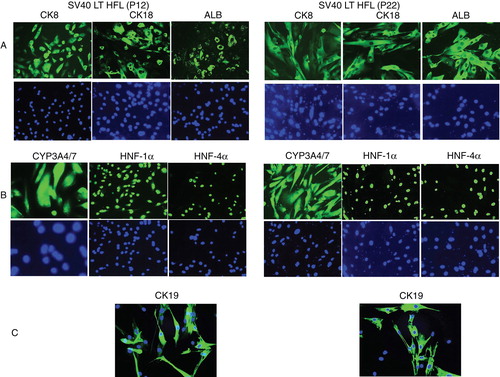
Cytochrome P450 3A4/7 isoenzymes are primarily membrane-associated proteins believed to be the predominant cytochrome P450 isoforms, and were found to be expressed in the cytoplasm of the transfected cells (). Expression was seen both in early as well as late passages. Furthermore, the transcription factors HNF-1α and HNF-4α () were expressed in these SV40 LT-HFL cells. They were detected in the nuclei of nearly all the cells. Also in this case, these markers were expressed in early and late passages. The biliary marker CK19 was also found to be expressed in P12, albeit less intensely in P22 ().
Expression of the SV40 LT antigen and tumor-associated markers
As determined by immunocytochemistry, virtually all SV40 LT-HFL cells that survived continuous puromycin selection expressed SV40 LT antigen (). The nuclear staining appeared to be of similar intensity (). The SV40 large T antigen expression in SV40 LT-HFL cells was consistent during cell culture suggesting stable integration into the host genome of the plasmid encoding SV40 LT antigen. In addition, the transfected cells did not stain positively for the cancer-associated markers p53, MOC-31 or Ber EP4, while the cancer cell line HepG2 cell line strongly expressed MOC-31 and Ber EP4, but not p53 ().
Figure 3. Staining of SV40 LT HFL cells for cancer-associated markers. (A) Immunofluorescent staining with an antibody to SV40 LT antigen demonstrated strong expression of the SV40 LT antigen in nuclei of all the cells at passage 22, as revealed after staining of the same cells with the nuclear stain, DAPI (B). (C) Immunofluorescent staining of SV40 LT-HFLcells in passage 11 and the liver cancer cell line, HepG2, for cancer-associated markers Ber EP4, MOC-31 and p53. The SV40 LT-HFL cells were negative for all the three markers, while Hep G2 cells were positive for Ber EP4 and MOC-31, but not p53. (D) Enzymatic staining of the transfected SV40 LT-HFL cells and liver cancer cell line HepG2 cells showing the expression of glucose -6-phosphatase and glycogen in the transfected cells but not the cancer cell line Hep G2. (Magnifications 20×).
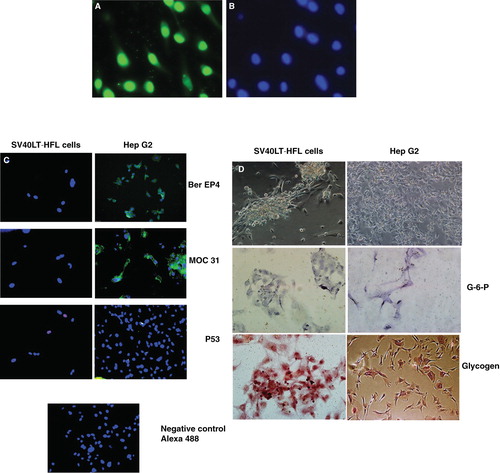
Further, using immunocytochemistry we found that the transfected cells expressed the liver specific marker G-6-P and stored glycogen ().
Expression of progenitor cell markers using flow cytometry
Hepatic progenitor cell markers such as EPCAM, CD90 and CD133 [Citation16] were detected in early passages of SV40 LT-HFL cells (p11, p22), using flow cytometry. However, later passages showed a decreased expression of these markers (). The cells however, did not express DLK-1 at any time point. We also confirmed expressions of CK 8 and albumin in the cells, which were strongly expressed in both early and late passages. Interestingly, the cells did not express the mesenchymal stem cell marker, CD271. Further, the cells did not express the hematopoietic stem cell marker, CD34, or the leukocyte marker, CD45.
Figure 4. Flow cytometric and RT-PCR analysis of SV40LT-HFL cells. (A) Flow cytometry of SV40LT-HFL cells showed expression of the progenitor cell markers EPCAM, CD133 and CD90 in early passages with decreased expression in the later passages. Dlk-1 was not detected in early or late passages (p11 and 22 respectively). In addition, the cells also expressed the hepatic markers albumin, CK 8, CK18, confirming our immunocytochemical results. No expression of CD271 a mesenchymal stem cell marker was observed. The cells did not express the hematopoietic marker CD34 or the leukocyte marker CD45. (B) RT-PCR analysis of SV40LT-HFL cells in passage 10 showing expression of detoxifying factor CYP3A7, albumin and transcription factor HNF-4α. The cells however, did not express CYP3A4 found in adult hepatocytes. β-actin was used as housekeeping gene. (C) HepG2 cells served as positive control, and expressed CYP3A4, CYP3A7, HNF-4α, HNF-1β and albumin transcripts as revealed by RT-PCR.
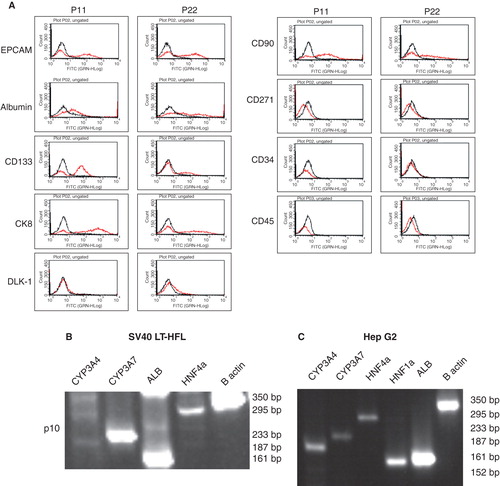
mRNA detection of liver markers by RT-PCR
To determine whether the immortalized cells retained liver-specific protein expression, the cells were also analyzed by RT-PCR. Results indicated that the immortalized HFL cells were positive for some of these markers, including albumin, HNF-4α (). There was a pronounced difference in the level of gene expression of CYP3A7 as compared to that of CYP3A4, suggesting that the positive staining of the CYP3A isoenzymes can be attributed to CYP3A7. Housekeeping gene was β-actin. Hep G2 cells were used as control cells ().
SV40 HFL cells differentiate into mature hepatocytes in vivo
To test whether SV40 LT-HFL cells have the potential to differentiate and be functional in vivo, we transplanted these cells into mice that were first treated with d-galactosamine to induce liver injury followed by partial hepatectomy. The transplanted cells differentiated into hepatocytes (), and formed bile ducts () at four weeks after transplantation. We also observed clear areas of bile ducts repopulated by human progenitors. These cells stained positively with an antibody specific for human CK19 (). We found several clusters of cells expressing human CK8, human c-Met and cells expressing the hepatocyte-specific antigen () in all of the five transplanted mice. A few AFP positive cells were also found in the transplanted animals (). Furthermore, the transplanted cells did not express the tumor suppressor marker p53 or the cancer marker Ber-EP4 (Supplement S2, ). As a note of interest no tumor formations were observed grossly or histologically using hematoxylin and eosin staining in any of the mice analyzed and the architecture of the liver was found to be normal (Supplement S2, ).
Figure 5. Expression of human liver-specific markers in the livers of nude mice transplanted with SV40 LT hFLCs. Two million SV40 LT HFL were transplanted into the spleen of D-galactosamine treated nude mice that underwent 30% partial hepatectomy at the time of transplantation. Immunohistochemistry was performed on fresh frozen liver sections of transplanted animals, and small clusters of human CK8-, CK19-, hepatocyte specific antigen-, and c-Met-expressing, engrafted cells (dark red-brown) with hepatocyte morphology were detected throughout the liver. Some cells expressing alphafeto protein were also detected. Biopsy sections from patients with liver cancer served as positive control and staining of liver sections from sham transplanted animals served as negative control. HE was used as counter-stain. (Magnification 40×.) CK, cytokeratin; Hep Ag, hepatocyte-specific antigen; AFP, alphafeto protein.
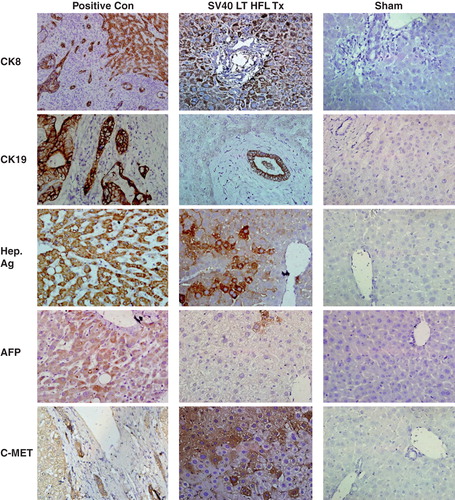
Immunofluorescence staining of transplanted mice with an antibody specific to human albumin demonstrated clusters of albumin positive human cells in all five mice (Supplement S2, ). However, variation in the expression of albumin was observed in the individual animals, with some animals showing several clusters of human albumin+ expression, while in one animal only a few clusters of positive cells was observed. Similarly, the transplanted mice (n = 5) showed detectable but varied levels of human albumin (03.39–221.30 ng/ml) compared to negative controls (0 ± 0 ng/ml) as sham and normal animal, where human serum albumin was not detected at all.
Importantly, using qPCR (core facility at Sahlgrenska, Gothenburg, Sweden), we found that the ct values for CYP3A4 in tx group animals (n = 3, 38.00 ± 0.33) varied with a log factor of 4 as compared to those found in normal human liver (n = 3, 34.52 ± 0.13) (Supplement S2, ), indicating the presence albeit lower amount of CYP3A4 in the transplanted animals. However, CYP3A7 was not detectable in the transplanted mice.
Discussion
In this study we describe the successful establishment of a hFLC line by introduction of SV40 large T antigen into normal primary HFL cells. The newly established immortalized fetal hepatocytes revealed morphologic characteristics of primary hepatocytes in standard culture systems and expressed many liver enriched markers, such as albumin, HNF-4, and CYP3A4/7. Immunohistochemistry assay demonstrated that the cells expressed liver-specific markers such as G-6-P and glycogen. Importantly, using cancer-associated markers such as these Ber-EP4, MOC-31 or p53; we demonstrated that the transfected cells in vitro and in vivo did not express any of these markers. We succeeded in maintaining these cells with stable morphology in vitro until p26 but not more. After p26 the cells went into senescence, and there were fewer cells as compared to our primary fetal liver cells (passage 12 [Citation17])
The markers, Ber-EP4 and MOC-31 have been demonstrated to be expressed on a wide range of normal and neoplastic human tissues, except hepatocytes, parietal cells, and apical cell layers in squamous epithelia [Citation18,19]. The oncogenic potential of the SV40 LT antigen resides in part in its ability to bind and inactivate many of the activities of the tumor suppressor p53 [Citation4,20,21]. However, our SV40 LT Ag transfected cells did not stain positive for p53. Furthermore, transplanted cells in vivo did not demonstrate tumor formation at 4 weeks after transplantation, or expression of p53 or Ber EP4. It is important to state that further long-term in vivo experimental studies are required to evaluate the tumorigenic potential of the present cell line.
Thus, we have succeeded in establishing in vitro expandable fetal liver progenitor cells by means of immortalization and without inducing a transformed phenotype and disrupting their differentiation potential. This cell line would facilitate studies on cell engraftment and differentiation within the hepatic parenchyma. In most cases, murine hepatic stem cell lines have been used to study immortalization and transformation in vitro [Citation22,23]. However, characteristics of murine stem cells cannot be extrapolated to their human counterparts, therefore it is important to establish human hepatic stem/progenitor cell lines to study the molecular events involved in their proliferation and differentiation in vitro as well as their fate in vivo after transplantation.
Fluorescent immunohistochemistry of frozen biopsy sections from transplanted mice and detection of serum albumin (albeit low) by ELISA revealed the functional status of the SV 40 cells. Flow cytometric analysis showed that in the early passages, these cells were positive for the hepatic progenitor cell markers [Citation24], EPCAM, CD133 and CD90 and but not DLK-1, CD34 or CD45 indicating the nonhematopoietic origin of these cells. Interestingly, unlike our primary human fetal liver precursors, the hepatoma cell line Hep G2 was found to strongly express the leukocyte marker CD45 (data not shown), confirming the hematopoietic origin of these cells.
In the present study, we studied the expression of two important CYPs: CYP3A7 and CYP3A4 by RT-PCR and immunocytochemical assays. CYP3A7 is normally expressed in abundance in fetal livers. On the other hand, CYP3A4 is believed to be the predominant cytochrome P450s expressed in adult human liver and is involved in the oxidation of the largest range of substrates of all the CYPs. Our results demonstrated that, the transfected cells expressed the genes and proteins in vitro. However, after transplantation we found that transplanted mice expressed low amounts of CYP3A4, but no expression of CYP3A7, indicating that the in vivo conditions are conducive for differentiation of the immature fetal liver cells. Furthermore we studied the expression of two important transcription factors: HNF 4α and HNF-1α. HNF-4α is required for the PXR and CAR-mediated transcriptional activation of CYP3A4 and is a transcription factor that is involved in the regulation of the expression of several liver specific genes [Citation25]. HNF-1α (hepatocyte nuclear factor 1, homeobox B), is encoded by the transcription factor 2 gene, a liver-specific factor [Citation25]. We found that the SV40 cell line expressed both these important transcription factors. Therefore, these immortalized SV40LT-HLF cells may be useful for the development of diagnostic tools for toxicity studies.
In conclusion, we have established a SV40 LT-HFL cell line which preserved morphological and functional characteristics of normal HFL cells, differentiated in culture, had an increased growth capacity and so far have retained a stable phenotype up to p26. Further protocols to differentiate these cells into stable mature hepatocytes expressing a wide repertoire of the heptocyte markers are required and such studies are currently underway in our laboratory.
Supplementary Figure 1–4
Download MS Word (1.2 MB)Acknowledgment
This study was financed by the Gothenburg County Council (LUA-ALF), The Lars Erik Gelins foundation and the IngaBritt and Arne Lundbergs foundation to Suchitra Sumitran-Holgersson.
Author contributions
PBP: Participated in performance of the research and data analysis. No conflict of interest. SB: Participated in performance of the research and data analysis. No conflict of interest. MJ: Participated in performance of the research and data analysis. No conflict of interest. MIK: Participated in performance of the research, writing of paper and data analysis. No conflict of interest. MO: Participated in writing of paper. No conflict of interest. SSH: Participated in research design, writing of paper and data analysis.
Declaration of interest: SSH is a shareholder and board member of NovaHep AB, a company developing hepatocyte-like cell lines for diagnostic and therapeutic purposes.
References
- Barbich M, Lorenti A, Hidalgo A, Ielpi M, de Santibáñez M, de Santibáñez E, et al. Culture and characterization of human hepatocytes obtained after graft reduction for liver transplantation: a reliable source of cells for a bioartificial liver. Artif Organs 2004;28:676–82.
- Fox IJ, Roy-Chowdhury J. Hepatocyte transplantation. J Hepatol 2004;40:878–86.
- Kobayashi N, Fujiwara T, Westerman KA, Inoue Y, Sakaguchi M, Noguchi H, et al. Prevention of acute liver failure in rats with reversibly immortalized human hepatocytes. Science 2000;287:1258–62.
- May P, May E. Twenty years of p53 research: structural and functional aspects of the p53 protein. Oncogene 1999;18:7621–36.
- Ali SH, DeCaprio JA. Cellular transformation by SV40 large T antigen: interaction with host proteins. Semin Cancer Biol 2001;11:15–23.
- Shay JW, Wright WE, Werbin H. Defining the molecular mechanisms of human cell immortalization. Biochim Biophys Acta 1991;1072:1–7.
- Begum S, Joshi M, Ek M, Holgersson J, Kleman MI, Sumitran-Holgersson S. Characterization and engraftment of long-term serum-free human fetal liver cell cultures. Cytotherapy 2010;12:201–11.
- Wadhwa R, Kaul SC, Mitsui Y. Cellular mortality and immortalization: a complex interplay of multiple gene functions. Prog Mol Subcell Biol 2000;24:191–204.
- Toouli CD, Huschtscha LI, Neumann AA, Noble JR, Colgin LM, Hukku B, et al. Comparison of human mammary epithelial cells immortalized by simian virus 40 T-Antigen or by the telomerase catalytic subunit. Oncogene 2002;21:128–39.
- Kobayashi N, Miyazaki M, Fukaya K, Inoue Y, Sakaguchi M, Noguchi H, et al. Establishment of a highly differentiated immortalized human hepatocyte cell line as a source of hepatic function in the bioartificial liver. Transplant Proc 2000;32:237–41.
- Kobayashi N, Noguchi H, Fujiwara T, Westerman KA, Leboulch P, Tanaka N. Establishment of a highly differentiated immortalized adult human hepatocyte cell line by retroviral gene transfer. Transplant Proc 2000;32:2368–9.
- Andres D, Diez-Fernandez C, Zaragoza A, Alvarez A, Cascales M. Induction of cell proliferation by cyclosporine A in primary cultures of rat hepatocytes. Biochem Pharmacol 2001;61:427–35.
- Rutenbur AM, Kim H, Fischbei JW, Hanker JS, Wasserkr HL, Seligman AM. Histochemical and ultrastructural demonstration of gamma-glutamyl transpeptidase activity. J Histochem Cytochem 1969;17:517–26.
- Ott M, Rajvanshi P, Sokhi RP, Alpini G, Aragona E, Dabeva M, et al. Differentiation-specific regulation of transgene expression in a diploid epithelial, cell line derived from the normal F344 rat liver. J Pathol 1999;187:365–73.
- Nowak G, Ericzon BG, Nava S, Jaksch M, Westgren M, Sumitran-Holgersson S. Identification of expandable human hepatic progenitors which differentiate into mature hepatic cells in vivo. Gut 2005;54:972–9.
- Tanaka M, Miyajima A. Identification and isolation of adult liver stem/progenitor cells. Methods Mol Biol 2012;826:25–32.
- Nava S, Westgren M, Jaksch M, Tibell A, Broomé U, Ericzon BG, et al. Characterization of cells in the developing human liver. Differentiation 2005;73:249–60.
- Latza U, Niedobitek G, Schwarting R, Nekarda H, Stein H. Ber-Ep4 - new monoclonal-antibody which distinguishes epithelia from mesothelia. J Clin Pathol 1990;43:213–19.
- Woodworth CD, Kreider JW, Mengel L, Miller T, Meng YL, Isom HC. Tumorigenicity of simian-virus 40-hepatocyte cell-lines - effect of invitro and invivo passage on expression of liver-specific genes and oncogenes. Mol Cell Biol 1988;8:4492–501.
- Ali SH, DeCaprio JA. Cellular transformation by SV40 large T antigen: interaction with host proteins. Semin Cancer Biol 2001;11:15–22.
- Pipas JM, Levine AJ. Role of T antigen interactions with p53 in tumorigenesis. Semin Cancer Biol 2001;11:23–30.
- Kim BH, Sung SR, Choi EH, Kim YI, Kim KJ, Dong SH, et al. Dedifferentiation of conditionally immortalized hepatocytes with long-term in vitro passage. Exp Mol Med 2000;32:29–37.
- Zhang XY, Guckian M, Nasiri N, Lovell PA, Dalgleish AG, Barton DPJ. Normal and SV40 transfected human peritoneal mesothelial cells produce IL-6 and IL-8: implication for gynaecological disease. Clin Exp Immunol 2002;129:288–96.
- Grompe M. The role of bone marrow stem cells in liver regeneration. Semin Liver Dis 2003;23:363–71.
- Akiyama TE, Gonzalez FJ. Regulation of P450 genes by liver-enriched transcription factors and nuclear receptors. Biochim Biophys Acta 2003;1619:223–34.