Abstract
1. When the immune system encounters incoming infectious agents, this generally leads to immunity. The evoked immune response is usually robust, but can be severely perturbed by potentially harmful environmental agents such as chemicals, pharmaceuticals and allergens.
2. Immunosuppression, hypersensitivity and autoimmunity may occur due to changed immune activity. Evaluation of the immunotoxic potency of agents as part of risk assessment is currently established in vivo with animal models and in vitro with cell lines or primary cells.
3. Although in vivo testing is usually the most relevant situation for many agents, more and more in vitro models are being developed for assessment of immunotoxicity. In this context, hypersensitivity and immunosuppression are considered to be a primary focus for developing in vitro methods. Three-dimensional organotypic tissue models are also part of current research in immunotoxicology.
4. In recent years, there has been a revival of interest in organotypic tissue models. In the context of immunotoxicity testing, precision-cut lung slices in particular have been intensively studied. Therefore, this review is very much focused on pulmonary immunotoxicology. Respiratory hypersensitivity and inflammation are further highlighted aspects of this review. Immunotoxicity assessment currently is of limited use in other tissue models, which are therefore described only briefly within this review.
Role of the innate and adaptive immune systems and the nervous system in the respiratory tract
The respiratory tract has a large fragile epithelial surface with an area of about 75 m2 in adults (CitationPavelka & Roth 2010). Every day, it is continuously exposed to 10,000 L of inhaled air containing a multitude of potentially harmful agents (CitationHolt et al. 2008). These agents can be detected by two very efficient major control systems – the nervous system and the immune system – playing fundamental roles in the maintenance of the local physiological homeostasis. The anatomical organization of both systems is specialized to deal with unique problems encountered in the microenvironment of the respiratory tract (CitationHolt et al. 2008; CitationVeres 2008).
The nervous system detects a wide variety of hazards that are of physical and chemical nature such as cold air and irritant gases, respectively. Immediate response of the autonomic nervous system is very important to prevent tissue damage due to unusual short-term inhalation of large amounts of irritating or noxious chemicals or physical hazards. Sensory nerves of the airways are activated via receptors on sensory nerve terminals, quickly followed by protective reflex bronchoconstriction, mucus production and cough (CitationBarnes 2001; CitationVeres 2008). These physiological reactions immediately prevent acute tissue injury (CitationBarnes 2001).
The immune system is a sensor for incoming agents that are of biological origin. They can be either infectious (bacteria, viruses and fungi) or non-infectious (allergens, derived from plants and animals) (CitationHammad & Lambrecht 2008). The network of tissues and cells of the immune system in the respiratory tract is tightly regulated and has developed a range of specific mechanisms to discriminate between innocuous and harmful antigens (CitationHolt et al. 2008). This is mainly done by local elements belonging to either innate immunity such as macrophages or adaptive immunity such as memory T-cells. Other cells such as airway dendritic cells (DC) are located at the interface between innate and adaptive immunity, playing also a crucial role in the regulation of immune responses to inhaled particles (CitationVeres 2008). They all communicate via soluble mediators contributing to local immunoregulation, controlling wound healing and tissue repair in response to antigens.
Obviously, both control systems serve the same purpose – protection of the organism from tissue injury after exposure to hazards. There are several mechanisms of interaction and the organism benefits from their close contact (CitationJames & Nijkamp 1999). In summary, the immune system and the nervous system mutually amplify one another, which is advantageous in health but can be deleterious in disease conditions such as allergic asthma (CitationVeres 2008).
Immunotoxicity testing – a tendency to include in vitro assays in the assessment of agents
When the immune system encounters incoming infectious agents such as bacteria, viruses or fungi, this generally leads to immunity. The evoked immune response is robust and usually less severe when recalled to the same agent later in life. Of course, there are many intrinsic factors that influence susceptibility of the immune response. Over the past 30 years, however, concerns have been raised that there are additionally many potentially harmful environmental agents such as chemicals, pharmaceuticals and allergens, which may perturb immunological responses and exacerbate certain diseases. In particular, onset and maintenance of an immunological response can be severely influenced by agents interacting with cells and functions of the immune system.
In general, immunotoxicity is defined as adverse health effect of xenobiotics on components and functions of the immune system (CitationLuster & Kimber 1996; CitationDescotes 2006). Due to decreased or increased immune activity, diseases and outcomes can be as follows: (i) Suppression of immune responses may lead to increased infection rates and higher incidence of cancer. Common infectious diseases can occur more frequently, for example, in immunocompromised patients and are often more severe due to reduced resistance and limited ability to respond to bacterial, viral, or parasitic infectious agents (CitationDescotes 2006). Cancer, for example virus-induced, may occur due to decreased tumour resistance. (ii) Enhancement of immune responses can induce hypersensitivity and exaggerate autoimmune diseases, leading to chronic inflammation as observed for example in allergic asthma, which will be presented in detail in the next section (CitationSnodin 2004; CitationDescotes 2006). These alterations of normal immune functions have to be detected by testing for adverse immunotoxic effects of xenobiotics.
Evaluation of the immunotoxic potency of agents as part of risk assessment is currently established in vivo with animal models and in vitro with cell lines or primary cells. Many testing methods and approaches that can be used for investigations are recommended in relevant guidelines. Selection of the best testing approach for xenobiotics such as chemicals, however, is still under discussion. There is substantial variation between all current immunotoxicity guidelines depending on product (e.g. chemicals, drugs, biopharmaceuticals, or pesticides) and geography (e.g. guidelines of CitationEMA 2000, EPA, CitationFDA 2002 and CitationOECD 2008 and guideline ICH/EMEA/FDA S8). Most guidelines allow immunotoxicity testing based on tiered approaches. For chemicals, for example, first information on potential adverse immunomodulatory effects may be obtained from standard toxicity studies, for example from haematology, lymphoid organ weights, histopathology, and bone marrow cellularity. Nevertheless, these endpoints alone are not sufficient to predict immunotoxic effects (CitationLuster et al. 1992). Additional follow-up investigations have to be initiated by alerts from basic toxicological studies (CitationTaylor & Pauels 2006). However, it is at the discretion of the investigator to choose appropriate models and test methods (Taylor & Pauels 2006). Currently, many investigators tend to include in vitro models with high biological relevance early in testing strategies to increase safety for further product developments. In particular hypersensitivity and immunosuppression are considered to be a primary focus for developing in vitro methods in immunotoxicology (CitationGalbiati et al. 2010). Examples are application of proliferation assays using, for example, progenitor cells of cord blood or lymphocytes of peripheral blood, and cytokine release assays using, for example, peripheral blood of human donors (CitationCarfi’ et al. 2007).
With this increasing tendency to use in vitro models with high biological relevance for immunotoxicity testing of chemicals and pharmaceuticals, in particular precision-cut lung slices – although this is not a newly developed model and has been used for many years – represent a promising technology for respiratory research. Other precision-cut tissue slices are currently of limited use in immunotoxicity assessment. Therefore, this review is primarily focused on pulmonary immunotoxicology.
Pathogenesis of allergic asthma and molecular mechanisms of respiratory sensitization
Allergic asthma is one of the most common chronic inflammatory lung diseases. It must be defined as adverse environmental health effect that results from stimulation of specific adaptive immune responses after inhalation, for example, of proteins or chemicals. It is characterized by intermittent airway obstruction, persistent airway inflammation and airway hyperreactivity, which is a state above normal responsiveness to bronchoconstrictors (CitationWills-Karp 1999). The incidence of asthma has globally increased over the past years (CitationHolgate 1999; CitationMasoli et al. 2004). In particular in industrialized countries rates are very high. On the cellular level the disease is basically associated with infiltration of the airway mucosa and surrounding parenchyma with eosinophils, mast cells, and allergen-specific T-lymphocytes. This event is coordinated by activity of cytokines such as interleukin (IL)-4, IL-5, and IL-13, which further perpetuate the inflammatory process. Permanent allergic inhalation exposure and induced chronic inflammation eventually lead to “airway remodelling”. These are changes observed on the histological level, including thickening of the basement membrane, hyperplasia, and hypertrophy of the smooth muscle layer. Nevertheless, mechanisms that cause asthma are complex and vary among population groups and individuals (CitationHolgate 1999).
Occupational asthma as a form of asthma is defined as a hypersensitivity reaction of the respiratory tract to an agent occurring in exposure scenarios at workplaces (CitationMapp et al. 2005). It can be life-threatening in severe cases, too (CitationBoverhof et al. 2008). Hypersensitivity reactions are based on the same immediate immunological mechanisms as mentioned above and described in more detail below. Nevertheless, the nature of immunological processes and molecular events through which occupational agents may cause respiratory hypersensitivity is not yet well understood and remains controversial.
Agents that are known to cause occupational asthma by inhalation at work include industrial high molecular weight compounds such as proteins, detergents, or polysaccharides (CitationWild & Lopez 2003), low molecular weight chemicals such as isocyanates, reactive dyes, and acid anhydrides, or metals, all of which are known or suspected to cause respiratory allergy in humans (CitationBernstein 2003; CitationIsola et al. 2008). Low molecular weight chemicals in their native state, unlike large compounds, are unable to induce respiratory sensitization by themselves. Upon respiratory exposure they become allergenic only after conjugation with larger native self molecules (e.g. human serum albumin). These hapten/protein carrier complexes can be recognized by antigen-presenting cells (APCs) as antigens and are able to provoke an immune response. Inhaled high molecular molecules such as proteins can often be recognized as such by cells of the immune system. They are either directly sampled by APCs which are distributed throughout the lungs and located beneath the epithelial layer or gain access to these cells by enzymatic cleavage of cell-cell contacts. The latter is possible because many potent allergens have intrinsic protease activity (CitationSokol et al. 2008). Irritating chemicals entering the respiratory tract are able to disrupt membranes of epithelial cells of the respiratory tract (CitationMapp et al. 2005). Tissue injury leads to release of pro-inflammatory cytokines such as IL-1α and tumour necrosis factor (TNF)-α which perpetuate the inflammation process. Antigens taken up by professional APCs are processed into peptides and presented in secondary lymphoid organs via major histocompatibility complex (MHC) class II to specific T-lymphocytes. Activity of released cytokines such as IL-4, IL-5 and IL-13 produced by allergen-specific CD4+ T-lymphocytes with a type-2 (Th2) profile induces antibody class switching in B-lymphocytes to immunoglobulin (Ig)E production, recruits primary effector cells, eosinophils, and mast cells into the airway mucosa (CitationDearman et al. 2003; CitationMapp et al. 2005). Allergen-specific IgE antibodies are bound to high-affinity Fc receptors (FcϵR) I on the surface of mast cells. Upon re-exposure, allergen binds to surface-bound IgE and thereby cross-links FcϵRI on mast cells. Subsequently, mast cells degranulate and release mediators such as histamine, causing acute bronchospasm and inflammation. This early asthmatic reaction (EAR) occurring within minutes after exposure to an inhaled allergen can be followed by a more prolonged late asthmatic reaction (LAR). Symptoms of LAR such as mucus hypersecretion, bronchoconstriction and airway inflammation are developed hours after allergen exposure and might persist for several days. Furthermore, airway hyperresponsiveness to bronchoconstrictors such as methacholine and histamine develops, which is characteristic for manifested allergic asthma.
Most of our knowledge of immunologic mechanisms of asthma has been derived from animal models in which important features in the histological picture and inflammation of human diseases can be reproduced. In recent years, there have also been attempts to implement precision-cut lung slices as a human-based model in asthma research, which will be presented in detail in the next sections.
Short review of the history of precision-cut lung slices
In recent years, there has been a revival of interest in organotypic tissue models. The reasons for this are diverse. First of all, there have been significant improvements in culturing and slicing technologies providing the opportunity to apply in vitro tissue models for the first time (CitationKrumdieck et al. 1980; CitationBrendel et al. 1987; CitationSmith et al. 1988). Nowadays, it is feasible to prepare very thin slices of precise sectioning, which is important for the generation of reproducible results (CitationMartin et al. 1996). Even if slicing itself means stress for the tissue, after resting it does not influence induction of immediate early genes and cytokines (CitationMartin et al. 2001; CitationHenjakovic et al. 2008a). Due to these improvements the model shows good reproducibility of findings (CitationSwitalla et al. 2010). Secondly, there is a need for complex but in comparison to in vivo reduced models with high biological relevance. And thirdly, there is a public demand to limit the number of animals and reduce distress of laboratory animals according to the 3R concept. It is a great advantage of precision-cut lung slices that copious numbers of slices can be obtained from one lung lobe. In order to get similar results in animals, for example with regard to dose response curves and kinetics of released mediators, large numbers of animals have to be used. Each lung lobe of laboratory animals and humans gives sufficient material to perform diverse exposure scenarios in parallel, and experiments can be performed such that each specimen is its own internal control. All these different aspects supported development of precision-cut lung slices as an ex vivo model.
Within the past 25 years, lung slices from different laboratory animals (e.g. mouse, rats, guinea pigs) and humans have been applied in many different studies (CitationFisher & Placke 1987; CitationFisher et al. 1994; CitationMartin et al. 1996; CitationMartin et al. 2001; CitationKott et al. 2002; CitationWohlsen et al. 2003; CitationVietmeier et al. 2007; CitationCooper et al. 2009). The majority of these studies were focused on investigation of functional responses of the airways and, for example, their contribution to outcomes of sensitization. Airway contraction in response to certain stimuli is usually assessed in lung slices of sensitized animals or passively sensitized lung explants (CitationWohlsen et al. 2001; CitationWohlsen et al. 2003; CitationCooper & Panettieri 2008). The biological relevance of those models is remarkable as demonstrated in comparison studies evaluating allergen-induced bronchoconstriction measurements in vivo and in vitro (CitationHenjakovic et al. 2008a). Furthermore, the technique also led to identification of molecular mediators of allergic airway constriction and elucidation of molecular mechanisms of bronchoconstriction.
Besides measurement of bronchial contractility many other aspects have been studied. Precision-cut lung slices have been used, for example, to study vascular responses and mucociliary functions (CitationKurosawa et al. 1995; CitationHeld et al. 1999; CitationMoreno et al. 2006; CitationPaddenberg et al. 2006). They have been applied in pharmacological and toxicological studies (CitationFisher & Placke 1987; CitationFisher et al. 1994; CitationParrish et al. 1995; CitationBach et al. 1996; CitationMonteil et al. 1999; CitationCatania et al. 2007; CitationSturton et al. 2008; CitationNassimi et al. 2009). Xenobiotically induced metabolism, bioactivation and detoxification have also been studied (CitationParrish et al. 1995; CitationPrice et al. 1995; CitationPrice et al. 1996; CitationLake et al. 2003; CitationDe Kanter et al. 2004; CitationPrice et al. 2004; CitationHarrigan et al. 2006; CitationUmachandran & Ioannides 2006; CitationPushparajah et al. 2008; CitationHanlon et al. 2009). Another interesting aspect of precision-cut lung slices is the use of tissue sections for infection studies. To this end, infections, for example, with viruses (CitationEbsen et al. 2002; CitationGoris et al. 2009; CitationWu et al. 2010), fungi (A. fumigatus in CitationErpenbeck et al. 2006) or bacterial spores (Bacillus anthracis in CitationChakrabarty et al. 2007 and CitationLanger et al. 2008) have been performed. The use of lung tissue for immunological investigations is a newly developed application of precision-cut lung slices, although the use of organotypic tissue for these purposes in general has been published, for example, for spleen tissue (CitationSkibinski & James 1997).
Recent developments for use of precision-cut lung slices as in vitro model for prediction of immunological responses
In the past years, it has also been principally demonstrated that precision-cut lung slices can be applied for immunomodulatory testing of inhalable chemicals, drugs and biopharmaceuticals. The model has been shown to be adequately sensitive and specific (CitationHenjakovic et al. 2008a, CitationSwitalla et al. 2010). The use of precision-cut lung slices for these purposes is mainly based on the ability to induce cytokines and cell surface markers on diverse cell populations in lung tissue sections. The normal architectural anatomy of precision-cut lung slices gives the possibility to very closely reflect immune responses in the lungs and to reproduce certain key steps of immune activation in lung tissue. This can be, for example, allergen uptake and presentation, defence reactions, cytokine signalling, and induction of cell surface markers on special cell populations. Nevertheless, other features of immune activation are partly or completely missing. Migration of cells, T-cell activation and proliferation in draining lymph nodes, for example, can hardly be mimicked. Because of the lack of circulatory systems and missing connection to draining lymph nodes it is not possible to study trafficking of cells from blood into lungs and from lungs into blood and lymphatic fluid. Nevertheless, these reactions are orchestrated by production of pro-inflammatory cytokines. Induction and suppression of these cytokines as a characteristic hallmark of inflammation processes in the airways can be used for evaluation of immune-modulating effects of agents.
Changes in the cytokine release pattern of lung tissue are now frequently used for testing of the immunomodulatory potency of xenobiotics. Recent studies have demonstrated the initiation of inflammation responses of the innate immune system in precision-cut lung slices of healthy mice, primates, and humans (CitationHenjakovic et al. 2008a, CitationSwitalla et al. 2010; CitationWu et al. 2010; CitationSeehase et al. 2012). The local immune responses in lung tissue sections have been assessed using a broad variety of immunological endpoints, and induced cytokine release was shown to be inhibited by added immunosuppressive drugs. These aspects of the use of precision-cut lung slices will be intensively described in the next sections. Inflammatory responses of the acquired immune system in precision-cut lung slices of sensitized animals have also been characterized (CitationHenjakovic et al. 2008b). For all approaches mentioned, precision-cut lung slices offer a novel and unique way to assess either lung function or local immune responses of lung tissue in vitro. The immune response of lung tissue has been investigated on different levels (e.g. protein production, gene expression, and mediator release) and results have been extrapolated to in vivo immune responses (CitationSewald & Braun 2011). The high degree of histological complexity provides a physiologically relevant model and circumvents drawbacks of simple single-cell cultures (e.g. lack of polarized phenotype) (CitationRoggen et al. 2006). The preserved cellular structure of precision-cut lung slices offers the possibility to study cellular dynamics and responses of immune cells located within tissue. Their role in immune surveillance and in controlling local processes can be studied. This is of interest in particular for cells that are not a homogeneous cell population.
Cells and cell populations of the immune system in precision-cut lung slices
Lung tissue sections maintain the complex and native three-dimensional histological organization of the intact organ with all relevant cell-cell interactions. Morphology, orientation, and structural integrity of local cells within tissue are preserved for many different cell types. The multicellular composition of parenchyma with intercellular connections and accessory cells can be studied intensively in live sections of lung tissue. Thin sections of precision-cut lung slices confirm a normal histological appearance showing tissue – depending on the preparation of the specimen – mainly composed of respiratory airways, bronchioles and alveoli (CitationMartin et al. 1996). Alveolar epithelium is formed by alveolar type I and type II cells. Interalveolar septa have layers of connective tissue with fibroblasts, fibres and capillaries (CitationChakrabarty et al. 2007; CitationHenjakovic et al. 2008a; CitationSwitalla et al. 2010).
These features show that nearly all naturally occurring cell types of the respiratory tract are situated in their physiological environment in precision-cut lung slices. Immune-activating and -responding cells are distributed within tissue interacting with each other and with other resident/accessory cells relevant for immune responses (CitationSewald & Braun 2011). Defence cells of innate immunity such as macrophages are located freely in the alveolar space (). Populations of DC are distributed throughout the tissue also forming an extensive network in the mucosa of conducting airways (CitationVeres et al. 2007; ). They play a decisive role at the onset of immunological processes and are essential for controlling effector T-cells in established inflammation (CitationLambrecht & Hammad 2003). As the primary task of APCs involves uptake, processing and transport of digested antigens to draining lymph nodes for presentation to antigen-specific T-cells, it becomes obvious how important it is to study molecular mechanisms in fine tuning of local respiratory processes.
Figure 1. Macrophages stained for CD68 in human precision-cut lung slices (CitationSwitalla et al. 2010). Untreated slices were stained with anti-human CD68 antibodies and subsequently incubated with Cy5-conjugated F(ab′)2 fragments. The appropriate isotypes were used as controls for staining specificity. Precision-cut lung slices were mounted with ProLong Gold anti-fade to avoid bleaching, and fluorescence was detected by confocal laser scanning microscopy (40× water immersion objective, 633 nm, emission filter LP680, thickness 20 µm, major grid spacing = 50 µm).
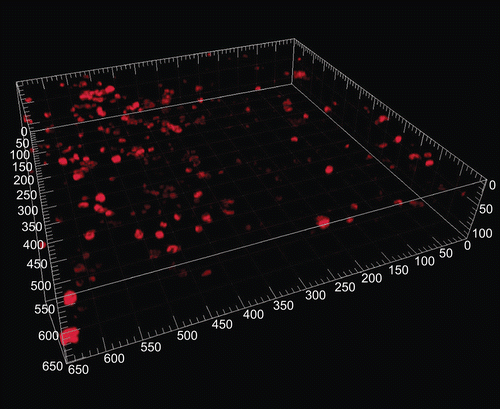
Figure 2. Airway mucosal dendritic cells forming a three-dimensional network within the airway mucosa. They display a typical dendritic morphology, with long, branched arms interdigitating between surrounding epithelial cells. The image shows a three-dimensional reconstruction of a confocal image showing CD11c+ DCs in a murine lung section. The image was taken at the proximal 1/4 of the main axial pathway of the left lung. The smooth muscle was visualized by phalloidin-staining of F-actin filaments (CitationVeres et al. 2007, by courtesy of T. Veres). Picture was reproduced with permission from CitationSewald and Braun (2011).
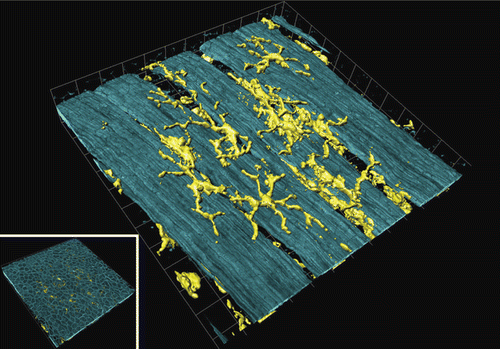
Frequently used protocols for cytokine release assay and bronchoconstriction measurements in precision-cut lung slices
The technique of preparation of lung tissue sections has been well described and reported in many publications (e.g. CitationHeld et al. 1999; CitationRessmeyer et al. 2006). Briefly, laboratory animals or animals of livestock breeding are sacrificed painlessly. Lung is extracted directly post mortem in order to maintain and conserve viability of tissue. For preparation of human precision-cut lung slices tissue is usually obtained from patients who, for example, undergo lobectomy because of different live-threatening diseases such as lung cancer or from lungs rejected after transplantation. Studies performed with human tissue have to be approved by an ethics committee. Furthermore, human tissue has to be processed immediately on the day of resection. Limitations of using human tissue are obvious: patients are normally elder than 50 years and 80% of patients suffering from lung cancer are smokers. Medication of patients can also influence the outcome in experiments using human tissue.
In any case, only tissue that has not been damaged is further used for in vitro experiments. Airways and surrounding parenchyma are filled up inside with a medium/agarose solution and cooled on ice. This step is necessary to solidify the very soft tissue for the further preparation procedures. It even seems to enhance nutrient exchange in tissue and improves lifetime of tissue by increasing the surface area (CitationFisher & Placke 1987). Lungs are cut into 250-µm-thick lung sections, which was reported to be optimal for lung slices by CitationMartin et al. 1996 (for human lung tissue see also CitationWohlsen et al. 2003; CitationRessmeyer et al. 2006). This can be done by using, for example, a specialized microtome (e.g. Krumdieck microtome) or vibratome (e.g. OTS-5000, Electron Microscopy Sciences). After very intensive washing steps, which are necessary to remove cell debris and released enzymes, slices can either be taken into culture and maintained under normal cell culture conditions for several days, or used immediately. Viability restricts the use of precision-cut lung slices to few days after preparation (). Within this time, tissue sections can be used for exposure experiments. For immunomodulatory assessments viable tissue sections are exposed to stimulatory and suppressive chemicals, substances, and drugs. Endpoints that are assessed include (i) cytotoxicity measurements using different assays such as the detection of enzyme activities (lactate dehydrogenase LDH, water-soluble tetrazolium Wst-1) or live/dead staining for confocal microscopy; (ii) cytokine release assays by measurement of cytokines and chemokines in supernatants; (iii) changes in expression profile, for example, of activated cytokines (CitationChakrabarty et al. 2007); (iv) measurement of formation of reactive oxygen species (CitationSpringer et al. 2007); (v) changes in ciliary beating; (vi) bronchoconstriction measurements; and (vii) characterization of changes in cellular populations by immunohistopathological methods (CitationMartin et al. 1996, 2001; CitationHenjakovic et al. 2008a).
Figure 3. Image analysis of time-dependent cell death in murine precision-cut lung slices. Tissue slices were stained with 4 µM calcein AM and 4 µM EthD-1 after 4 h (A), 24 h (B), 48 h (C), and 72 h (D) of cultivation and after cell lysis (E). The images were examined by two-colour immunofluorescence confocal microscopy (40× water immersion objective, excitation wavelengths 488 nm and 543 nm, emission filters BP 505–550 nm and LP 560 nm, thickness 20 µm, grid spacing = 20 µm) and analysed with IMARIS 4.5.2. Red colour shows cell nuclei (Ø 5 µm) of dead cells and green colour the cytoplasm of viable cells. Picture was reproduced with permission from CitationHenjakovic et al. 2008a.
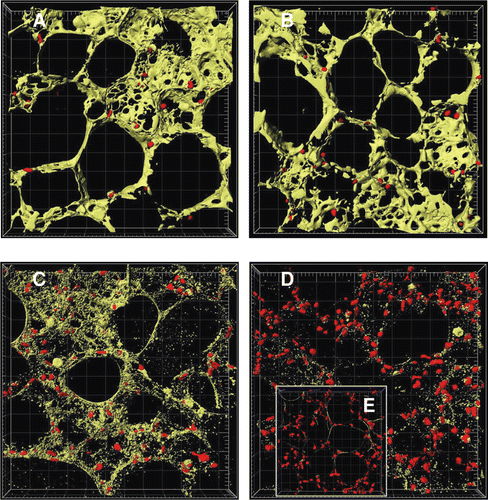
Lung tissue slices reflect the natural immunological response of the intact organ
Precision-cut lung slices were implemented to test their ability to respond to lipopolysaccharide (LPS), which is an activator of the innate immune system. LPS is a macromolecule integrated in the outer membrane of most Gram-negative bacteria. It is ubiquitous in the environment. LPS typically consists of a hydrophobic domain known as lipid A part, a non-repeating carbohydrate core, and a distal polysaccharide domain (O-antigen). In particular its highly conserved lipid A moiety is considered to be very effective in inducing inflammation responses (CitationRaetz & Whitfield 2002). LPS is identified by the host as a non-self molecule by binding to receptors of the innate immune system such as Toll-like receptors (TLRs) 4/7, which are present on macrophages and endothelial cells (CitationRaetz & Whitfield 2002). These receptors are called pattern recognition receptors (PRRs) and are specialized to be activated after binding of pathogen-associated molecular patterns (PAMP) such as LPS. Multiple pathways are involved in activation of the innate immune response. The TLR/IL-1 receptor pathway is one of the most prominent. Activation of these pathways generally results in immune responses that are regulated by T helper type 1 (Th1) cells and IL-17-producing T helper cells (CitationSokol et al. 2008).
LPS is widely used in basic and applied research for activation of components of the innate immune system and thereby for induction of inflammatory processes. Due to its highly conserved structure and species cross-reactivity it can be used to induce comparable inflammatory responses in both in vivo and in vitro models of different species. It is a very potent activator of monocytes and macrophages triggering production and release of pro-inflammatory cytokines including TNF-α (CitationMorelli et al. 2001; CitationTogbe et al. 2007). Alveolar macrophages are known to respond to LPS in vivo and in vitro (CitationMartin et al. 1992). Moreover, monocytes and macrophages are not the only cell populations that are sensitive to presence of LPS. Other cells such as respiratory epithelial cells or endothelial cells, which cannot easily be studied in in vitro models in the presence of other cells, are also involved in production of mediators and adhesion molecules in response to LPS. This is a great advantage of precision-cut lung slices: the opportunity to study immune responses of different cell types simultaneously in culture and to assess the entire immune response of activated lungs (CitationSewald & Braun 2011).
Precision-cut lung slices of different species (e.g. human, primate, rat, murine) have been implemented to test for their ability to respond to LPS as a well-defined modulator of the innate immune system (Henjakovic et al. 2008; CitationSwitalla et al. 2010; CitationSeehase et al. 2012). Precision-cut lung slices have been prepared and exposed to LPS at increasing concentrations. The effect of LPS on cytokine levels was determined. LPS-induced cytokine release was initiated by significant and rapid accumulation, for example, of TNF-α, followed by other important pro-inflammatory cytokines such as IL-1α, macrophage inflammatory protein-1β (MIP-1β), Regulated upon Activation, Normal T-cell Expressed and Secreted (RANTES), macrophage chemoattractant protein (MCP-1), IFN-γ, granulocyte-macrophage colony-stimulating factor (GM-CSF), and anti-inflammatory IL-10 (). Release of TNF-α, IL-1α, and IL-6 after in vitro stimulation of monocytes and macrophages with LPS is also known from literature (CitationBailly et al. 1990). Since TNF-α was used as a marker of macrophage function, the increased TNF-α production indicates production of these mediators by macrophages in lung tissue upon activation by LPS (CitationSewald & Braun 2011). The cytokine response starting at picomolar concentrations of LPS shows that lung tissue can not only be activated for an inflammatory response, but is also a very sensitive sensor of environmental immunotoxic agents. The highest amount of, for example, TNF-α was detected at an early time point, whereas the release of IL-1α increased progressively over 24 h. Remarkably, the observed cytokines not only play a decisive role in respiratory inflammatory diseases, but also the sequence and amount of their appearance followed cascades of inflammatory events known from in vivo scenarios. This was shown by comparison of LPS-induced acute cytokine responses of human lung tissue to clinical data. Studies published by CitationSchaumann et al. (2008) demonstrated that segmental provocation of volunteers with mild asthma resulted in an inflammatory response in the lungs comparable to studies described by CitationO’Grady et al. (2001). Comparison of induced local pulmonary effects on cytokine levels with LPS-provoked cytokines in ex vivo lung tissue revealed a high and significant correlation (CitationSwitalla et al. 2010). Similar results have been shown for LPS-induced TNF-α in marmoset whole-blood cultures and marmoset lung tissue (CitationSeehase et al. 2012). Hence, the LPS-induced inflammatory response in precision-cut lung slices resembles the in vivo situation very closely.
Figure 4. IL-1α and TNF-α in human precision-cut lung slices (A, C) and bronchoalveolar lavage (BAL) fluid from asthmatic patients after provocation with LPS (B, D). TNF-α levels in culture supernatants and IL-1α levels in lysates were determined by ELISA in human precision-cut lung slices after 24 h of culture. Cytokine amounts in BAL fluid of 17 non-smoking subjects were determined using Luminex technology (CitationSchaumann et al. 2008). Data are presented as mean ± SEM for precision-cut lung slices from 12 donors, *p < 0.05; **p < 0.01; ***p < 0.005 (one-way ANOVA Dunnett’s test for precision-cut lung slices, t-test for BAL).
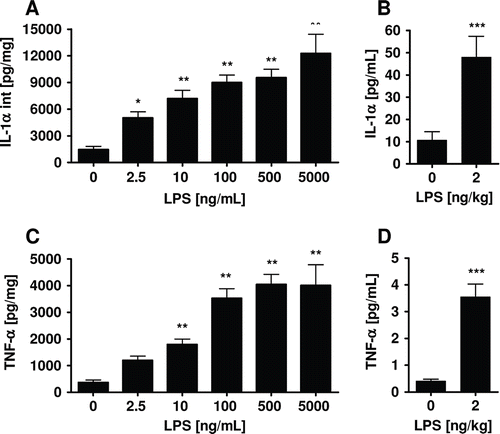
On the cellular level, LPS induces maturation by increased expression of cell surface markers in respiratory APCs (CitationWeigt et al. 2003). APCs are known to be distributed throughout the lungs, forming a dense network within airways and parenchyma. They play a decisive role at the onset of immune responses (CitationVeres et al. 2007). Phenotype and localization of LPS-responding cells in lung parenchyma of precision-cut lung slices were characterized by fluorescence imaging of MHC class II (CitationSewald & Braun 2011; ). In the absence of inflammation and infection it was observed that a low number of cells located in alveolar regions and showing DC morphology were MHC class II+ in the tissue. Most pulmonary DCs showed an immature phenotype and expressed MHC class at low level (CitationCella et al. 1997; CitationInaba et al. 2000). After activation by inflammatory LPS, expression of MHC class II was increased in tissue, which is in line with publication showing that APCs, for example, strongly enhance expression of MHC class II (CitationCella et al. 1997; CitationInaba et al. 2000). Lung slices of CD11c-EYFP-transgenic mice were used for further characterization. Within the tissue CD11c+ cells morphologically were heterogeneously distributed. Co-staining of MHC class II revealed a cell population that was highly positive for both markers. Fewer cells were found to be positive for CD86 and CD40. From literature it is well-known that mature APCs express co-stimulatory molecules for effective stimulation of T-cell proliferation after migration to lymph nodes (CitationBanchereau & Steinman 1998; CitationLambrecht 2001). Incubation of precision-cut lung slices with LPS did not induce increased expression of CD86. Expression of CD86 occurs after migration to lymph nodes (CitationBanchereau & Steinman 1998; CitationLambrecht 2001). Migration of DCs from the lungs into regional lymph nodes is a critical part of their function. However, this part of the induction of immune response during induced immune responses cannot be evaluated using ex vivo precision-cut lung slices because of their lacking connection to local lymph nodes. Nevertheless, it is believed that DCs collect and integrate all relevant information in the periphery which is accompanied with phenotypical and functional changes and transfer this information to lymph nodes. Upregulation of MHC class II in precision-cut lung slices demonstrated that parts of this information can be detected in lung tissue (CitationHenjakovic et al. 2008a).
Figure 5. Ex vivo staining of MHC class II in live murine precision-cut lung slices after 24 h. The images were taken by confocal microscopy. A limited number of MHC class II+ cells were found in alveolar regions displaying DC morphology. Inset in the bottom left corner shows the respective isotype antibody control.
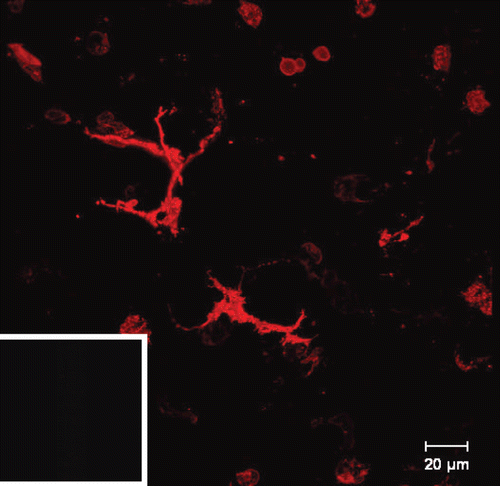
In summary, barrier epithelial cells, airway DCs, and macrophages make up one of the first line of defence against inhaled agents such as allergens and endotoxins (CitationHammad et al. 2009). Agents that enter the lung modify not only functional and phenotypic behaviour of APCs, but also of structural cells such as epithelial cells, fibroblasts, endothelial cells, smooth muscle cells, and other stromal cells. These cells are an important source of activating cytokines and play an active role in modulating the immune response, which suggests that they also might be involved in the outcome of the lung immune response, for example in maintaining chronic inflammation (CitationSewald & Braun 2011). In this context, a solid understanding of processes occurring in vivo when tissue is challenged by a potential immunomodulator is needed.
Effect of immunomodulatory agents on immune responses in precision-cut lung slices
LPS-induced activation of respiratory inflammation in lung sections can be inhibited by immunosuppressive drugs. The anti-inflammatory drug dexamethasone was used to demonstrate inhibition of induced cytokine release in precision-cut lung slices of different species. Dexamethasone is a corticosteroid which is widely administered in clinical use as an effective therapy for patients with chronic inflammatory diseases such as chronic obstructive pulmonary disease (COPD) or asthma (CitationBarnes 1995; CitationWagelie-Steffen et al. 2006). Corticosteroids inhibit expression of key inflammatory proteins such as cytokines and, partly as a consequence thereof, influx of inflammatory cells such as neutrophils and eosinophils. Precision-cut lung slices have been incubated with LPS with or without dexamethasone. The effect of dexamethasone on the activated immune response in precision-cut lung slices was determined (CitationHenjakovic et al. 2008a, CitationSwitalla et al. 2010; CitationSeehase et al. 2012). Indeed, dexamethasone prevented LPS-induced release of nearly all measured proteins, including TNF-α, IL-1α, and IL-12 (). Dexamethasone is known to influence several transcription factors such as NF-κB, which is a key factor in downregulation of many pro-inflammatory gene transcripts such as IL-1, MCP-1 and RANTES (CitationHart et al. 2000). Altogether, employing LPS and dexamethasone it was possible to demonstrate the application of immunomodulatory agents in precision-cut lung slices. But also other immunomodulatory agents such as roflumilast – which is a phosphodiesterase-4 inhibitor – suppressed cytokine response in precision-cut lung slices, as recently shown by CitationSeehase et al. 2012.
Figure 6. Total production of GM-CSF, IL-12, IL-1β, IL-10, MIP-1β, and IFN-γ in human precision-cut lung slices after 24 h of incubation with lipopolysaccharide and dexamethasone (CitationSwitalla et al. 2010). Cytokine levels in culture supernatants and after lyses were determined by MSD technology. Data are presented as mean ± SEM, *p < 0.05; **p < 0.01; ***p < 0.005 (GM-CSF, IL-12, IFN-γ, and MIP-1β: n = 3, IL-1β and IL-10: n = 6). dxm, dexamethasone, GM-CSF, granulocyte-macrophage colony-stimulating factor, IFN, interferon, IL, interleukin, int, intracellular, MIP, macrophage inflammatory protein.
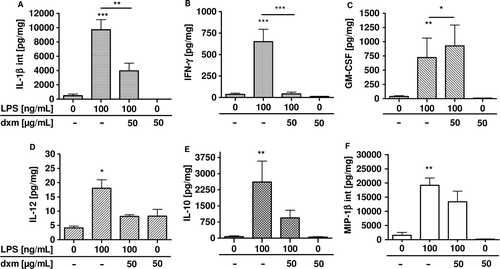
Chemical-induced activation of local inflammatory responses has also been studied intensively over the past years (CitationSewald & Braun 2011). In particular for identification of respiratory sensitizers a strategy was pursued that was thought to allow prediction of whether a chemical is a sensitizer or not. Human and murine precision-cut lung slices were therefore exposed to respiratory sensitizers (e.g. trimellitic anhydride, ammonium hexachloroplatinate) and contact sensitizers (e.g. cinnamaldehyde, 2,4-dinitrochlorobenzene), and the toxicity effect of chemicals on lung tissue was determined. Differences in cytokine release patterns, for example for IL-1α, TNF-α, IL-8, MCP-1, MIP-1, RANTES, IL-6, eotaxin etc. following exposure to chemicals have been intensively assessed (Sewald & Braun 2011). The cytokine response comprised proteins produced by alveolar macrophages and structural cells including fibroblasts, epithelial and endothelial cells. Most cytokines were shown to be induced by chemical-induced loss of cell viability (irritation). This effect is currently being further studied in a ring trial according to ECVAM criteria. For some immunotoxic chemicals, however, induction of cytokines was also found at subtoxic concentrations. For example, respiratory allergens increased TNF-α and IL-1α at non-toxic concentrations, while contact allergens failed to induce release of these cytokines to the same extent (CitationSewald & Braun 2011). This suggests that combination of cytokine patterns with cytotoxic data may represent a promising in vitro model for screening of allergens.
In summary, the reviewed studies show that models using primate or human lung tissue might be highly reliable regarding induced and suppressed immune responses. In particular for studies with immunological background classic rodent models may fail to predict reliable safety and efficacy data. Here, application of human or primate-based organotypic models may help to predict immunological responses after exposure to xenobiotics.
Use of other precision-cut tissue culture systems for evaluation of immunotoxicity
Precision-cut tissue slices have been described for many other organs such as liver (references see below), spleen (references see below), kidney (e.g. CitationDe Kanter et al. 2002; CitationVittorelli et al. 2004; CitationVickers & Fisher 2005), brain (e.g. CitationNoraberg et al. 2005; CitationMontero et al. 2009), heart (CitationBull et al. 2000), intestine (e.g. CitationDe Kanter et al. 2002; Citationvan de Kerkhof et al. 2005), and prostate (CitationParrish et al. 2002). They have been intensively used, for example, for toxicology, pharmacology, and metabolism research. Currently, the use of precision-cut tissue slices of other organs than lung for immunotoxicity assessments is limited. Nevertheless, precision-cut tissue slices from other organs have also the potential to be involved in the assessment of adverse effects of agents.
Lymphoid tissue (spleen and lymph nodes)
Important parts of the adaptive immune response to foreign antigens occur largely in lymphatic tissue of spleen and lymph nodes. Lymphatic tissue has a highly complex architecture consisting of several cell types. In spleen it is arranged around blood vessels and mainly composed of B and T lymphocytes, macrophages, and DC. These cells are able to react to blood-borne antigens by producing large amounts of Ig and to remove filtered defective blood cells.
Precision-cut spleen and lymph node slices have been shown to be useful for investigation of primary and secondary immune responses in lymphoid tissue (CitationSkibinski & James 1997). Several aspects of immune activation in lymphatic tissue, such as proliferation, spontaneous Ig production, expression of cell surface markers such as CD23 and CD25 on B-cells and T-cells, respectively, have been investigated (CitationHoffmann et al. 1995; CitationJames et al. 1996). Pro-inflammatory cytokines such as IL-1, IL-6 and IL-8 have been measured in mitogen-stimulated slice cultures. The immunosuppressive effect of added agents such as cyclosporine initially demonstrated flexibility of the organ tissue model. The model has also been shown to react upon polyclonal stimulation or to the presence of mitogens like phytohemagglutinin (PHA) and pokeweed mitogen (PWM) with increased expression of cell activation markers. The provoked response was highly comparable to single-cell cultures (CitationHoffmann et al. 1995). Others used spleen slices primarily for determination of colony-forming cells (Citationvon Melchner et al. 1980). Neural modulation of cytokine release in lymphoid tissue was studied by Straub et al. (1996, 1998). Neuronal controlling of lympoid organs might be important as feedback for cytokine secretion during inflammation (CitationStraub et al. 1998).
Very interesting molecular aspects of T-cell activation, migration, and polarization have been studied on excised lymph node and thymic preparations, for example by CitationAsperti-Boursin et al. 2007. Here, the identity of chemokines controlling T-cell motility and signalling events has been investigated in a physiological environment. A mixture of labelled T and B lymphocytes was overlaid on a slice surface and allowed to migrate into tissue. Cells were found to be positioned in their respective T-cell and B-cell zones. T-cells were very motile within tissue. Chemokines involved in T-cell trafficking within lymph node slices were identified by using lymph node slices, for example, of C-C chemokine receptor (CCR)7-deficient mice. Positioning and migration of T-cells within living tissue clearly demonstrated dependence of T-cell migration on chemoattractants (CitationAsperti-Boursin et al. 2007). These are interesting examples of the application of viable lymphoid tissue for the investigation of molecular aspects in adaptive immune responses.
Up to now, precision-cut tissue slices of spleen, lymph nodes, and thymus have not yet found frequent application as an in vitro tool in immunology research. Contrary to this, lymphoid cell suspensions are being widely used to analyse cytokine production, quantify cell subtypes, and investigate immune cell proliferation. Suspensions are prepared, for example, by mechanical disintegration of lymphoid organs, and the isolated lymphocytes can be cultured for analysis, for example, of cytokine production and proliferation. Immune responses of primary lymphocytes can be stimulated, for example, by mitogens such as LPS, PHA, and concanavalin A (ConA). Concurrently, adverse effects of agents on mitogen-induced cytokine release and proliferation can be evaluated. Furthermore, flow cytometric cell subtyping of lymphocytes can be carried out for subsetting using a large variety of cell surface markers. The manifold use of lymphocytes as single-cell suspension has constrained the development of ex vivo models based on lymphoid tissue in immunotoxicology.
Liver
Metabolism of xenobiotics occurs mainly in the liver. The liver has a complex organ histology mainly consisting of hepatocytes, Kupffer cells, endothelial and stellate cells. The interaction of hepatocytes which express most of the enzymes involved in drug metabolism with other cells is very important for the physiology of the liver. For many years, precision-cut liver slices have been successfully used to study predominately liver-specific mechanisms and functions such as metabolism and toxicity of applied xenobiotics (CitationDe Kanter et al. 2002; de CitationGraaf et al. 2007 and references therein, Citationde Graaf et al. 2010). Results obtained from ex vivo precision-cut liver slices have been correlated with in vivo animal experiments (CitationDe Kanter et al. 2002; CitationElferink et al. 2008). Other studies focused on inflammation processes occurring in liver using precision-cut liver slices as models of liver injury and fibrosis (Citationvan de Bovenkamp et al. 2007; Citationvan de Bovenkamp et al. 2008; CitationSchaffert et al. 2010). The use of precision-cut liver slices to study inflammatory responses includes very interesting aspects that will be highlighted in detail in the following.
Local inflammation processes in the liver result from interaction between different parenchymal and non-parenchymal cell types. Cells such as resident macrophages – also known as Kupffer cells – can be stimulated by LPS to produce pro-inflammatory cytokines such as TNF-α, IL-10, IL-1β, and other cytokines (CitationOlinga et al. 2001; CitationSzalowska et al. 2009). Thus, hepatic macrophages contribute to the control of early phases of inflammation processes in the liver. It was clearly shown that LPS induces a concentration-dependent increase in TNF-α, IL-1β, and IL-10 in culture medium of precision-cut liver slices (CitationOlinga et al. 2001). The molecular process is mediated by CD14 expressed on Kupffer cells. The concentration of cytokines in medium was probably underestimated with respect to the amount released, because cytokines produced in liver tissue were specifically bound to receptors on hepatocytes, degraded, and only partly released into medium (CitationOlinga et al. 2001). Immunomodulatory agents such as dexamethasone and pentoxifylline – a phosphodiesterase inhibitor – significantly decreased production of TNFα and IL-1β. LPS stimulation also caused release of NO by inducible nitric oxide synthase (iNOS), which was measured as nitrate/nitrite (NOx) concentration in medium. Production of NO was also significantly decreased by inhibitors. These data show that immune-responding cells such as Kupffer cells, endothelial cells, and hepatocytes are viable for at least 24 h in precision-cut lung slices and can be activated by activators of the innate immune response such as LPS. Moreover, liver slices also produce acute-phase proteins during liver inflammation (CitationNijsten et al. 2000).
In summary, liver slices can be used to study effects and mechanism of action of anti-inflammatory agents (CitationOlinga et al. 2001; CitationKleemann et al. 2004). In particular the application of human precision-cut liver slices would be very useful. The application of precision-cut liver slices for immunotoxicity assessments is currently rather limited. Incidences of adverse effects on liver so far have been obtained mainly in standard toxicity studies or assays based on hepatocyte cultures.
Declaration of interest
The authors report no conflicts of interest.
References
- Asperti-Boursin F, Real E, Bismuth G, Trautmann A, Donnadieu E. (2007). CCR7 ligands control basal T cell motility within lymph node slices in a phosphoinositide 3-kinase-independent manner. J Exp Med 204:1167–1179.
- Bach PH, Vickers AEM, Fisher R, Baumann A, Brittebo E, Carlile DJ, Koster HJ, Lake BG, Salmon F, Sawyer TW, Skibinski G. (1996). The use of tissue slices for pharmacotoxicology studies. ATLA 24:893–923.
- Bailly S, Ferrua B, Fay M, Gougerot-Pocidalo MA. (1990). Differential regulation of IL 6, IL 1 A, IL 1 beta and TNF alpha production in LPS-stimulated human monocytes: role of cyclic AMP. Cytokine 2:205–210.
- Banchereau J, Steinman RM. (1998). Dendritic cells and the control of immunity. Nature 392:245–252.
- Barnes PJ. (1995). Inhaled glucocorticoids for asthma. N Engl J Med 332:868–875.
- Barnes PJ. (2001). Neurogenic inflammation in the airways. Respir Physiol 125:145–154.
- Bernstein DI. (2003). Occupational asthma caused by exposure to low-molecular-weight chemicals. Immunol Allergy Clin North Am 23:221–34, vi.
- Boverhof DR, Billington R, Gollapudi BB, Hotchkiss JA, Krieger SM, Poole A, Wiescinski CM, Woolhiser MR. (2008). Respiratory sensitization and allergy: current research approaches and needs. Toxicol Appl Pharmacol 226:1–13.
- Brendel K, McKee RL, Hruby VJ, Johnson DG, Gandolfi AJ, Krumdieck CL. (1987). Precision cut tissue slices in culture: a new tool in pharmacology. Proc West Pharmacol Soc 30:291–293.
- Bull DA, Reid BB, Connors RC, Albanil A, Stringham JC, Karwande SV. (2000). Improved biochemical preservation of heart slices during cold storage. Int J Surg Investig 2:117–123.
- Carfi’ M, Gennari A, Malerba I, Corsini E, Pallardy M, Pieters R, Van Loveren H, Vohr HW, Hartung T, Gribaldo L. (2007). In vitro tests to evaluate immunotoxicity: a preliminary study. Toxicology 229:11–22.
- Catania JM, Pershing AM, Gandolfi AJ. (2007). Precision-cut tissue chips as an in vitro toxicology system. Toxicol In Vitro 21:956–961.
- Cella M, Engering A, Pinet V, Pieters J, Lanzavecchia A. (1997). Inflammatory stimuli induce accumulation of MHC class II complexes on dendritic cells. Nature 388:782–787.
- Chakrabarty K, Wu W, Booth JL, Duggan ES, Nagle NN, Coggeshall KM, Metcalf JP. (2007). Human lung innate immune response to Bacillus anthracis spore infection. Infect Immun 75:3729–3738.
- Cooper PR, Panettieri RA Jr. (2008). Steroids completely reverse albuterol-induced beta(2)-adrenergic receptor tolerance in human small airways. J Allergy Clin Immunol 122:734–740.
- Cooper PR, McParland BE, Mitchell HW, Noble PB, Politi AZ, Ressmeyer AR, West AR. (2009). Airway mechanics and methods used to visualize smooth muscle dynamics in vitro. Pulm Pharmacol Ther 22:398–406.
- Dearman RJ, Betts CJ, Humphreys N, Flanagan BF, Gilmour NJ, Basketter DA, Kimber I. (2003). Chemical allergy: considerations for the practical application of cytokine profiling. Toxicol Sci 71:137–145.
- de Graaf IA, Olinga P, de Jager MH, Merema MT, de Kanter R, van de Kerkhof EG, Groothuis GM. (2010). Preparation and incubation of precision-cut liver and intestinal slices for application in drug metabolism and toxicity studies. Nat Protoc 5:1540–1551.
- De Kanter R, De Jager MH, Draaisma AL, Jurva JU, Olinga P, Meijer DK, Groothuis GM. (2002). Drug-metabolizing activity of human and rat liver, lung, kidney and intestine slices. Xenobiotica 32:349–362.
- De Kanter R, Monshouwer M, Draaisma AL, De Jager MH, de Graaf IA, Proost JH, Meijer DK, Groothuis GM. (2004). Prediction of whole-body metabolic clearance of drugs through the combined use of slices from rat liver, lung, kidney, small intestine and colon. Xenobiotica 34:229–241.
- Descotes J. (2006). Methods of evaluating immunotoxicity. Expert Opin Drug Metab Toxicol 2:249–259.
- Ebsen M, Mogilevski G, Anhenn O, Maiworm V, Theegarten D, Schwarze J, Morgenroth K. (2002). Infection of murine precision cut lung slices (PCLS) with respiratory syncytial virus (RSV) and chlamydophila pneumoniae using the Krumdieck technique. Pathol Res Pract 198:747–753.
- Elferink MG, Olinga P, Draaisma AL, Merema MT, Bauerschmidt S, Polman J, Schoonen WG, Groothuis GM. (2008). Microarray analysis in rat liver slices correctly predicts in vivo hepatotoxicity. Toxicol Appl Pharmacol 229:300–309.
- EMA. (2000). Note for guidance on repeated dose toxicity (CPMP/SWP/1042/99corr). The European Agency for the Evaluation of Medical Products, London, UK.
- EPA. (1998). Health Effects Guidelines OPPTS 870.7800: Immunotoxicity.
- Erpenbeck VJ, Ziegert M, Cavalet-Blanco D, Martin C, Baelder R, Glaab T, Braun A, Steinhilber W, Luettig B, Uhlig S, Hoymann HG, Krug N, Hohlfeld JM. (2006). Surfactant protein D inhibits early airway response in Aspergillus fumigatus-sensitized mice. Clin Exp Allergy 36:930–940.
- FDA. (2002). Immunotoxicology evaluation of investigational new drugs. Guidance for Industry. Centers for Drug Evaluation and Research. Food and Drug Administration. US Department of Health and Human Services, USA.
- Fisher GL, Placke ME. (1987). In vitro models of lung toxicity. Toxicology 47:71–93.
- Fisher RL, Smith MS, Hasal SJ, Hasal KS, Gandolfi AJ, Brendel K. (1994). The use of human lung slices in toxicology. Hum Exp Toxicol 13:466–471.
- Galbiati V, Mitjans M, Corsini E. (2010). Present and future of in vitro immunotoxicology in drug development. J Immunotoxicol 7:255–267.
- Goris K, Uhlenbruck S, Schwegmann-Wessels C, Köhl W, Niedorf F, Stern M, Hewicker-Trautwein M, Bals R, Taylor G, Braun A, Bicker G, Kietzmann M, Herrler G. (2009). Differential sensitivity of differentiated epithelial cells to respiratory viruses reveals different viral strategies of host infection. J Virol 83:1962–1968.
- Graaf IA, Groothuis GM, Olinga P. (2007). Precision-cut tissue slices as a tool to predict metabolism of novel drugs. Expert Opin Drug Metab Toxicol 3:879–898.
- Hammad H, Lambrecht BN. (2008). Dendritic cells and epithelial cells: linking innate and adaptive immunity in asthma. Nat Rev Immunol 8:193–204.
- Hammad H, Chieppa M, Perros F, Willart MA, Germain RN, Lambrecht BN. (2009). House dust mite allergen induces asthma via Toll-like receptor 4 triggering of airway structural cells. Nat Med 15:400–416.
- Hanlon N, Coldham N, Sauer MJ, Ioannides C. (2009). Modulation of rat pulmonary carcinogen-metabolising enzyme systems by the isothiocyanates erucin and sulforaphane. Chem Biol Interact 177:115–120.
- Harrigan JA, McGarrigle BP, Sutter TR, Olson JR. (2006). Tissue specific induction of cytochrome P450 (CYP) 1A1 and 1B1 in rat liver and lung following in vitro (tissue slice) and in vivo exposure to benzo(a)pyrene. Toxicol In Vitro 20:426–438.
- Hart L, Lim S, Adcock I, Barnes PJ, Chung KF. (2000). Effects of inhaled corticosteroid therapy on expression and DNA-binding activity of nuclear factor kappaB in asthma. Am J Respir Crit Care Med 161:224–231.
- Held HD, Martin C, Uhlig S. (1999). Characterization of airway and vascular responses in murine lungs. Br J Pharmacol 126:1191–1199.
- Henjakovic M, Sewald K, Switalla S, Kaiser D, Müller M, Veres TZ, Martin C, Uhlig S, Krug N, Braun A. (2008a). Ex vivo testing of immune responses in precision-cut lung slices. Toxicol Appl Pharmacol 231:68–76.
- Henjakovic M, Martin C, Hoymann HG, Sewald K, Ressmeyer AR, Dassow C, Pohlmann G, Krug N, Uhlig S, Braun A. (2008b). Ex vivo lung function measurements in precision-cut lung slices (PCLS) from chemical allergen-sensitized mice represent a suitable alternative to in vivo studies. Toxicol Sci 106:444–453.
- Hoffmann P, Skibinski G, James K. (1995). Organ culture of human lymphoid tissue. I. Characteristics of the system. J Immunol Methods 179:37–49.
- Holgate ST. (1999). The epidemic of allergy and asthma. Nature 402:B2–B4.
- Holt PG, Strickland DH, Wikström ME, Jahnsen FL. (2008). Regulation of immunological homeostasis in the respiratory tract. Nat Rev Immunol 8:142–152.
- ICH/EMEA/FDA. (2006). S8: Immunotoxicity Studies for Human Pharmaceuticals.
- Inaba K, Turley S, Iyoda T, Yamaide F, Shimoyama S, Reis e Sousa C, Germain RN, Mellman I, Steinman RM. (2000). The formation of immunogenic major histocompatibility complex class II-peptide ligands in lysosomal compartments of dendritic cells is regulated by inflammatory stimuli. J Exp Med 191:927–936.
- Isola D, Kimber I, Sarlo K, Lalko J, Sipes IG. (2008). Chemical respiratory allergy and occupational asthma: what are the key areas of uncertainty? J Appl Toxicol 28:249–253.
- James K, Skibinski G, Hoffman P. (1996). A comparison of the performance in vitro of precision cut tissue slices and suspensions of human spleen with special reference to immunoglobulin and cytokine production. Hum Antibodies Hybridomas 7:138–150.
- James DE, Nijkamp FP. (1999). Neuro-immune interactions in the lung. Clin Exp Allergy 29:1309–1319.
- Kleemann R, Verschuren L, de Rooij BJ, Lindeman J, de Maat MM, Szalai AJ, Princen HM, Kooistra T. (2004). Evidence for anti-inflammatory activity of statins and PPARalpha activators in human C-reactive protein transgenic mice in vivo and in cultured human hepatocytes in vitro. Blood 103:4188–4194.
- Kott KS, Pinkerton KE, Bric JM, Plopper CG, Avadhanam KP, Joad JP. (2002). Methacholine responsiveness of proximal and distal airways of monkeys and rats using videomicrometry. J Appl Physiol 92:989–996.
- Krumdieck CL, dos Santos JE, Ho KJ. (1980). A new instrument for the rapid preparation of tissue slices. Anal Biochem 104:118–123.
- Kurosawa H, Wang CG, Dandurand RJ, King M, Eidelman DH. (1995). Mucociliary function in the mouse measured in explanted lung tissue. J Appl Physiol 79:41–46.
- Lake BG, Meredith C, Scott MP, Renwick AB, Price RJ. (2003). Use of cultured precision-cut rat lung slices to study the in vitro induction of pulmonary cytochrome P450 forms. Xenobiotica 33:691–702.
- Lambrecht BN. (2001). The dendritic cell in allergic airway diseases: a new player to the game. Clin Exp Allergy 31:206–218.
- Lambrecht BN, Hammad H. (2003). Taking our breath away: dendritic cells in the pathogenesis of asthma. Nat Rev Immunol 3:994–1003.
- Langer M, Malykhin A, Maeda K, Chakrabarty K, Williamson KS, Feasley CL, West CM, Metcalf JP, Coggeshall KM. (2008). Bacillus anthracis peptidoglycan stimulates an inflammatory response in monocytes through the p38 mitogen-activated protein kinase pathway. PLoS ONE 3:e3706.
- Luster MI, Portier C, Pait DG, White KL Jr, Gennings C, Munson AE, Rosenthal GJ. (1992). Risk assessment in immunotoxicology. I. Sensitivity and predictability of immune tests. Fundam Appl Toxicol 18:200–210.
- Luster MI, Kimber I. (1996). Immunotoxicity: hazard identification and risk assessment. Hum Exp Toxicol 15:947–948.
- Mapp CE, Boschetto P, Maestrelli P, Fabbri LM. (2005). Occupational asthma. Am J Respir Crit Care Med 172:280–305.
- Martin C, Uhlig S, Ullrich V. (1996). Videomicroscopy of methacholine-induced contraction of individual airways in precision-cut lung slices. Eur Respir J 9:2479–2487.
- Martin C, Uhlig S, Ullrich V. (2001). Cytokine-induced bronchoconstriction in precision-cut lung slices is dependent upon cyclooxygenase-2 and thromboxane receptor activation. Am J Respir Cell Mol Biol 24:139–145.
- Martin TR, Mathison JC, Tobias PS, Leturcq DJ, Monarty AM, Maunder RJ, Ulevitch RJ. (1992). Lipopolysaccharide binding protein enhances the responsiveness of alveolar macrophages to bacterial lipopolysaccharide. J Clin Invest 90:2209–2219.
- Masoli M, Fabian D, Holt S, Beasley R; Global Initiative for Asthma (GINA) Program. (2004). The global burden of asthma: executive summary of the GINA Dissemination Committee report. Allergy 59:469–478.
- Monteil C, Le Prieur E, Buisson S, Morin JP, Guerbet M, Jouany JM. (1999). Acrolein toxicity: comparative in vitro study with lung slices and pneumocytes type II cell line from rats. Toxicology 133:129–138.
- Montero M, González B, Zimmer J. (2009). Immunotoxic depletion of microglia in mouse hippocampal slice cultures enhances ischemia-like neurodegeneration. Brain Res 1291:140–152.
- Moreno L, Perez-Vizcaino F, Harrington L, Faro R, Sturton G, Barnes PJ, Mitchell JA. (2006). Pharmacology of airways and vessels in lung slices in situ: role of endogenous dilator hormones. Respir Res 7:111.
- Morelli AE, Zahorchak AF, Larregina AT, Colvin BL, Logar AJ, Takayama T, Falo LD, Thomson AW. (2001). Cytokine production by mouse myeloid dendritic cells in relation to differentiation and terminal maturation induced by lipopolysaccharide or CD40 ligation. Blood 98:1512–1523.
- Nassimi M, Schleh C, Lauenstein HD, Hussein R, Lübbers K, Pohlmann G, Switalla S, Sewald K, Müller M, Krug N, Müller-Goymann CC, Braun A. (2009). Low cytotoxicity of solid lipid nanoparticles in in vitro and ex vivo lung models. Inhal Toxicol 21 Suppl 1:104–109.
- Nijsten MW, Olinga P, The TH, de Vries EG, Koops HS, Groothuis GM, Limburg PC, ten Duis HJ, Moshage H, Hoekstra HJ, Bijzet J, Zwaveling JH. (2000). Procalcitonin behaves as a fast responding acute phase protein in vivo and in vitro. Crit Care Med 28:458–461.
- Noraberg J, Poulsen FR, Blaabjerg M, Kristensen BW, Bonde C, Montero M, Meyer M, Gramsbergen JB, Zimmer J. (2005). Organotypic hippocampal slice cultures for studies of brain damage, neuroprotection and neurorepair. Curr Drug Targets CNS Neurol Disord 4:435–452.
- OECD. (2008). OECD guideline for testing of chemicals 407: Repeated Dose 28-day Oral Toxicity Study in Rodents.
- O’Grady NP, Preas HL, Pugin J, Fiuza C, Tropea M, Reda D, Banks SM, Suffredini AF. (2001). Local inflammatory responses following bronchial endotoxin instillation in humans. Am J Respir Crit Care Med 163:1591–1598.
- Olinga P, Merema MT, de Jager MH, Derks F, Melgert BN, Moshage H, Slooff MJ, Meijer DK, Poelstra K, Groothuis GM. (2001). Rat liver slices as a tool to study LPS-induced inflammatory response in the liver. J Hepatol 35:187–194.
- Paddenberg R, König P, Faulhammer P, Goldenberg A, Pfeil U, Kummer W. (2006). Hypoxic vasoconstriction of partial muscular intra-acinar pulmonary arteries in murine precision cut lung slices. Respir Res 7:93.
- Parrish AR, Gandolfi AJ, Brendel K. (1995). Precision-cut tissue slices: applications in pharmacology and toxicology. Life Sci 57:1887–1901.
- Parrish AR, Sallam K, Nyman DW, Orozco J, Cress AE, Dalkin BL, Nagle RB, Gandolfi AJ. (2002). Culturing precision-cut human prostate slices as an in vitro model of prostate pathobiology. Cell Biol Toxicol 18:205–219.
- Pavelka M, Roth J. (2010). Functional ultrastructure, Altas of tissue biology and pathology. Wien: Springer-Verlag.
- Price RJ, Renwick AB, Beamand JA, Esclangon F, Wield PT, Walters DG, Lake BG. (1995). Comparison of the metabolism of 7-ethoxycoumarin and coumarin in precision-cut rat liver and lung slices. Food Chem Toxicol 33:233–237.
- Price RJ, Walters DG, Hoff C, Mistry H, Renwick AB, Wield PT, Beamand JA, Lake BG. (1996). Metabolism of [ring-U-14C] agaritine by precision-cut rat, mouse and human liver and lung slices. Food Chem Toxicol 34:603–609.
- Price RJ, Renwick AB, Walters DG, Young PJ, Lake BG. (2004). Metabolism of nicotine and induction of CYP1A forms in precision-cut rat liver and lung slices. Toxicol In Vitro 18:179–185.
- Pushparajah DS, Umachandran M, Nazir T, Plant KE, Plant N, Lewis DF, Ioannides C. (2008). Up-regulation of CYP1A/B in rat lung and liver, and human liver precision-cut slices by a series of polycyclic aromatic hydrocarbons; association with the Ah locus and importance of molecular size. Toxicol In Vitro 22:128–145.
- Raetz CR, Whitfield C. (2002). Lipopolysaccharide endotoxins. Annu Rev Biochem 71:635–700.
- Ressmeyer AR, Larsson AK, Vollmer E, Dahlèn SE, Uhlig S, Martin C. (2006). Characterisation of guinea pig precision-cut lung slices: comparison with human tissues. Eur Respir J 28:603–611.
- Roggen EL, Soni NK, Verheyen GR. (2006). Respiratory immunotoxicity: an in vitro assessment. Toxicol In Vitro 20:1249–1264.
- Schaffert CS, Duryee MJ, Bennett RG, DeVeney AL, Tuma DJ, Olinga P, Easterling KC, Thiele GM, Klassen LW. (2010). Exposure of precision-cut rat liver slices to ethanol accelerates fibrogenesis. Am J Physiol Gastrointest Liver Physiol 299:G661–G668.
- Schaumann F, Müller M, Braun A, Luettig B, Peden DB, Hohlfeld JM, Krug N. (2008). Endotoxin augments myeloid dendritic cell influx into the airways in patients with allergic asthma. Am J Respir Crit Care Med 177:1307–1313.
- Seehase S, Lauenstein HD, Schlumbohm C, Switalla S, Neuhaus V, Förster C, Fieguth HG, Pfennig O, Fuchs E, Kaup FJ, Bleyer M, Hohlfeld JM, Braun A, Sewald K, Knauf S. (2012). LPS-induced lung inflammation in marmoset monkeys - an acute model for anti-inflammatory drug testing. PLoS ONE 7:e43709.
- Sewald K, Braun A. (2011). PCLS: from learning about “in vivo” reduction and replacement. In: Roggen EL, Weltzien HU, Hermans H, eds. Progress Towards Novel Testing Strategies for in vitro Assessment of Allergens. Kerala, India: Transworld Research Network.
- Skibinski G, James K. (1997). The use of tissue slices in immunological investigations. Arch Immunol Ther Exp (Warsz) 45:411–417.
- Smith PF, Fisher R, McKee R, Gandolfi AJ, Krumdieck CL, Fisher R, Brendel K. (1988). Precision-cut liver slices: a new in vitro tool in toxicology. In: McQueen CA, ed. In vitro Toxicology: Model Systems and Methods. New Jersey: Telford Press, 93–130.
- Snodin DJ. (2004). Regulatory immunotoxicology: does the published evidence support mandatory nonclinical immune function screening in drug development? Regul Toxicol Pharmacol 40:336–355.
- Sokol CL, Barton GM, Farr AG, Medzhitov R. (2008). A mechanism for the initiation of allergen-induced T helper type 2 responses. Nat Immunol 9:310–318.
- Springer J, Groneberg DA, Dinh QT, Quarcoo D, Hamelmann E, Braun-Dullaeus RC, Geppetti P, Anker SD, Fischer A. (2007). Neurokinin-1 receptor activation induces reactive oxygen species and epithelial damage in allergic airway inflammation. Clin Exp Allergy 37:1788–1797.
- Straub RH, Herrmann M, Frauenholz T, Berkmiller G, Lang B, Schölmerich J, Falk W. (1996). Neuroimmune control of interleukin-6 secretion in the murine spleen. Differential beta-adrenergic effects of electrically released endogenous norepinephrine under various endotoxin conditions. J Neuroimmunol 71:37–43.
- Straub RH, Dorner M, Riedel J, Kubitza M, Van Rooijen N, Lang B, Schölmerich J, Falk W. (1998). Tonic neurogenic inhibition of interleukin-6 secretion from murine spleen caused by opioidergic transmission. Am J Physiol 274:R997–1003.
- Sturton RG, Trifilieff A, Nicholson AG, Barnes PJ. (2008). Pharmacological characterization of indacaterol, a novel once daily inhaled 2 adrenoceptor agonist, on small airways in human and rat precision-cut lung slices. J Pharmacol Exp Ther 324:270–275.
- Switalla S, Lauenstein L, Prenzler F, Knothe S, Förster C, Fieguth HG, Pfennig O, Schaummann F, Martin C, Guzman CA, Ebensen T, Müller M, Hohlfeld JM, Krug N, Braun A, Sewald K. (2010). Natural innate cytokine response to immunomodulators and adjuvants in human precision-cut lung slices. Toxicol Appl Pharmacol 246:107–115.
- Szalowska E, Elferink MG, Hoek A, Groothuis GM, Vonk RJ. (2009). Resistin is more abundant in liver than adipose tissue and is not up-regulated by lipopolysaccharide. J Clin Endocrinol Metab 94:3051–3057.
- Taylor J, Pauels HG. (2006). An overview of immunotoxicity guidelines – past to present. Regul Rapporteur 3:1–6.
- Togbe D, Schnyder-Candrian S, Schnyder B, Doz E, Noulin N, Janot L, Secher T, Gasse P, Lima C, Coelho FR, Vasseur V, Erard F, Ryffel B, Couillin I, Moser R. (2007). Toll-like receptor and tumour necrosis factor dependent endotoxin-induced acute lung injury. Int J Exp Pathol 88:387–391.
- Umachandran M, Ioannides C. (2006). Stability of cytochromes P450 and phase II conjugation systems in precision-cut rat lung slices cultured up to 72 h. Toxicology 224:14–21.
- van de Bovenkamp M, Groothuis GMM, Meijer DKF, Olinga P. (2007). Liver fibrosis in vitro: cell culture models and precision-cut liver slices. Toxicol In Vitro 21:545–557.
- van de Bovenkamp M, Groothuis GMM, Meijer DKF, Olinga P. (2008). Liver slices as a model to study fibrogenesis and test the effects of anti-fibrotic drugs on fibrogenic cells in human liver. Toxicol In Vitro 22:771–778.
- van de Kerkhof EG, de Graaf IA, de Jager MH, Meijer DKF, Groothuis GMM. (2005). Characterization of rat small intestinal and colon precision-cut slices as an in vitro system for drug metabolism and induction studies. Drug Metab Dispos 33:1613–1620.
- Veres TZ, Rochlitzer S, Shevchenko M, Fuchs B, Prenzler F, Nassenstein C, Fischer A, Welker L, Holz O, Müller M, Krug N, Braun A. (2007). Spatial interactions between dendritic cells and sensory nerves in allergic airway inflammation. Am J Respir Cell Mol Biol 37:553–561.
- Veres TZ. (2008). Interaction between airway dendritic cells and sensory nerves in allergic airway inflammation. Dissertation. Hannover: Hannover Medical School.
- Vickers AE, Fisher RL. (2005). Precision-cut organ slices to investigate target organ injury. Expert Opin Drug Metab Toxicol 1:687–699.
- Vietmeier J, Niedorf F, Bäumer W, Martin C, Deegen E, Ohnesorge B, Kietzmann M. (2007). Reactivity of equine airways–a study on precision-cut lung slices. Vet Res Commun 31:611–619.
- Vittorelli A, Gauthier C, Michoudet C, Baverel G. (2004). Metabolic viability and pharmaco-toxicological reactivity of cryopreserved human precision-cut renal cortical slices. Toxicol In Vitro 18:285–292.
- von Melchner H, Metcalf D, Mandel TE. (1980). Use of spleen organ cultures to monitor hemopoietic progenitor cell regeneration following irradiation and marrow transplantation. Blood 56:917–922.
- Wagelie-Steffen AL, Kavanaugh AF, Wasserman SI. (2006). Biologic therapies for the treatment of asthma. Clin Chest Med 27:133–47, vii.
- Weigt H, Mühlradt PF, Emmendörffer A, Krug N, Braun A. (2003). Synthetic mycoplasma-derived lipopeptide MALP-2 induces maturation and function of dendritic cells. Immunobiology 207:223–233.
- Wild LG, Lopez M. (2003). Occupational asthma caused by high-molecular-weight substances. Immunol Allergy Clin North Am 23:235–50, vii.
- Wills-Karp M. (1999). Immunologic basis of antigen-induced airway hyperresponsiveness. Annu Rev Immunol 17:255–281.
- Wohlsen A, Martin C, Vollmer E, Branscheid D, Magnussen H, Becker WM, Lepp U, Uhlig S. (2003). The early allergic response in small airways of human precision-cut lung slices. Eur Respir J 21:1024–1032.
- Wohlsen A, Uhlig S, Martin C. (2001). Immediate allergic response in small airways. Am J Respir Crit Care Med 163:1462–1469.
- Wu W, Booth JL, Duggan ES, Wu S, Patel KB, Coggeshall KM, Metcalf JP. (2010). Innate immune response to H3N2 and H1N1 influenza virus infection in a human lung organ culture model. Virology 396:178–188.