Abstract
Purpose: Hyperthermic isolated limb perfusion (HILP) with TNF-α and melphalan has high response rates in patients with soft tissue sarcomas (STS) or melanomas of the limbs. Its effectiveness is based on the destructive effect of TNF-α on the blood supply of the tumours. Shedding of soluble TNF-receptor (sTNF-R) negatively modulates the effects of TNF-α, whereas hyperthermia (HT) induces shedding. Here, we investigated whether sTNF-R shedding in response to HT occurs during HILP.
Material and methods: The serum levels of sTNFR-1 were measured in 23 patients with HILP by obtaining serum from the extracorporeal and central circuits. The samples were taken from the patients under normothermic (37°C) and hyperthermic (39°C) conditions. Additionally, cell cultures of HUVEC, human fibrosarcoma cells and peripheral blood cells were used to confirm the effects of HT on sTNF-R1 shedding by ELISA and western blot.
Results: Under HT, levels of sTNF-R1 increased 23.5% in the extracorporeal circuit, but this increase was not observed in the systemic circuit. However, we could not confirm this effect using the cell culture model, where cellular TNF-R1 and sTNF-R1 of culture supernatants, respectively, were not significantly different between NT and HT conditions.
Conclusions: HT is associated with an increase of sTNF-R1 in the extracorporeal circuit of perfused limbs. Interestingly, HT does not exhibit the same effect on cells cultured in vitro. Additional studies will be aimed at determining whether our findings have an impact in the clinic by analysing the relationship between TNF-R1 shedding and tumour response to HILP.
Introduction
In regionally advanced soft tissue sarcomas (STS) and malignant melanomas of the limbs, primary limb-saving surgery is not always an option. Because amputation does not improve the overall survival of patients with STS, salvage of the limb is one of the major goals of surgery Citation[1]. Currently, one of the most promising limb-saving neoadjuvant treatments involves the use of hyperthermic isolated limb perfusion (HILP), which shows high response and limb salvage rates in patients with STS Citation[2]. During HILP, hyperthermia (HT) in combination with high-dose melphalan (L-phenylalanine mustard) and recombinant human tumour necrosis factor alpha (rhTNF-α) is administered to the affected limb via the oxygenated extracorporeal circuit Citation[3].
TNF-α is a cytokine that modulates a variety of immune processes, including inflammatory reactions, cytokine production, activation and expression of adhesion molecules, growth stimulation, apoptosis and tumour necrosis Citation[4]. Because the dose of TNF-α required to induce antitumoural effects is approximately 10-fold higher than the dose found to induce major systemic effects, HILP allows the application of excess levels of rhTNF-α Citation[5], Citation[6]. The tumour-destroying properties of TNF-α can be ascribed to the biphasic effects it has on tumour vessels. First, TNF-α immediately enhances the permeability of intratumoural microvessels Citation[5], Citation[7], which increases the uptake of alkylating agents that are simultaneously administered Citation[8], Citation[9]. Second, TNF-α promotes the delayed disintegration of tumour vessels, which most likely occurs via apoptosis of the endothelial cell layer Citation[10].
TNF-α exerts its biological functions by binding to TNF receptor type 1 (TNF-R1; also known as CD120a and p55TNF-R) and/or TNF-R2 (also known as CD120b and p75TNF-R). Whereas TNF-R1 is constitutively expressed in nearly all tissues, the expression of TNF-R2 is restricted to lymphoid cells. In the majority of cells, TNF-R1 seems to be the initiator of TNF-α triggered activity Citation[11], Citation[12]. Both TNF-Rs can be released from the cell surface as soluble receptor fragments (sTNF-R) by proteolytic cleavage triggered by various stimuli Citation[13], such as the administration of TNF-α during HILP Citation[14], Citation[15]. Shedding of sTNF-Rs modulates the functions of TNF-α in two ways: (1) through the transient desensitisation of cells due to a decrease in the density of the membrane-bound receptor; and (2) through the neutralisation of TNF-α by sTNF-R (decoy function).
sTNF-R shedding has been studied by several groups and has been found to increase during many clinical situations, such as in infection Citation[16], Citation[17], sepsis Citation[15], Citation[17] and cancer Citation[18], Citation[19]. However, no data exists regarding the influence of HT on the release of sTNF-Rs during TNF-α-based HILP Citation[20–22]. The aim of this study was to determine whether HT can contribute to sTNF-R shedding during HILP. For this purpose, we first examined the serum levels of sTNF-R1 in patients with HILP-treated limbs before and after undergoing HT. In addition, we also used a cell culture-based model of HT-induced TNF-R1 shedding.
Materials and methods
We examined the kinetics of TNF-R shedding in the systemic and extracorporeal (limb) circuits during HILP. To minimise the influence of the factors of the clinical procedure, we also examined the shedding of sTNF-R1 in an experimental setting using a cell culture-based model.
Clinical investigation of TNF-R1 shedding in response to hyperthermia
Hyperthermic isolated limb perfusion (HILP)
All the patients provided informed consent, and the study was performed following the rules and regulations of the Declaration of Helsinki. The evaluation of the patients and the HILP procedure were performed exclusively by the same specialised team. Briefly, HILP was performed under mild conditions of HT (39°C) with TNF-α leakage monitoring. The patients were administered rhTNF-α at a dose of 0.25 mg/L of perfused tissue volume and 11 mg/L of melphalan (L-phenylalanine mustard) in the legs and 13 mg/L in the arms.
Analysis of the patients’ serum during HILP
Blood samples from the systemic and extracorporeal circuits were taken from 23 patients who underwent HILP (). Blood from the extracorporeal circuit was obtained under conditions of normothermia (NT) (35°–36°C) and mild HT (39°C) prior to administration of TNF-α or melphalan. The blood samples of the systemic and extracorporeal circuits of each patient were drawn at the same time point (). Each sample was collected in blood sample tubes (Sarstedt S-Monovette Neutral, Nümbrecht, Germany), and the serum was isolated by centrifugation (15 min at 7500 rpm) and subsequently frozen at −70°C. Quantitative analysis of sTNF-R1 in the serum was performed by ELISA, as described below.
Figure 1. Overview of the clinical procedure. The schematic shows the procedure used for blood sampling during HILP. HLM, heart lung machine.
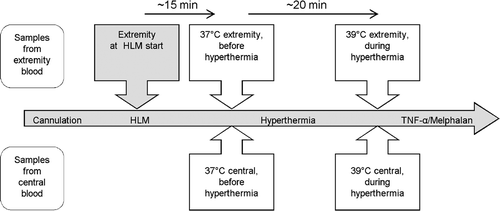
Table I. Clinical characteristics of the patients.
To exclude the possible influence of the heart/lung machine (HLM) on sTNF-R1 shedding, we collected an additional blood sample from the limbs of five patients at the start of the extracorporeal circuit ().
Cell culture model of TNF-R1 shedding in response to hyperthermia
For our cell culture experiments three different cell types were used: human umbilical vein endothelial cells (HUVECs, passage numbers 5, 6), human fibrosarcoma cells (HT-1080) and peripheral blood mononuclear cells (PBMNCs). HUVECs (PromoCell, Heidelberg, Germany) were cultured in cell culture flasks (Falcon, Heidelberg, Germany) at 37°C in an atmosphere of 5% CO2 in modified MCDB-131 cell culture medium (Gibco/Invitrogen, Karlsruhe, Germany) supplemented with 20% fetal calf serum (FCS) (Biowest, Nuaillé, France), 50 µg/mL heparin, 2 mM L-glutamine and 50 µg/mL penicillin/streptomycin (Sigma-Aldrich, Munich, Germany). HT-1080 cells (ATCC, Manassas, VA) were cultured in cell culture flasks in modified Eagle's minimum essential medium (Sigma-Aldrich) supplemented with 10% FCS, 1 mM sodium pyruvate (Sigma-Aldrich), 1 mM L-glutamine and 50 µg/mL penicillin/streptomycin. PBMNCs were isolated from healthy human donors using a modified method from Boyum et al. Citation[23]. Once isolated, the PBMNCs were resuspended in RPMI-1640 (Gibco/Invitrogen, Karlsruhe, Germany) supplemented with 2% FCS and 50 µg/mL penicillin/streptomycin and seeded into 6-mm Petri dishes (Falcon, Heidelberg, Germany) at a density of 3 × 106/3 mL. HUVECs and HT-1080 cells were seeded into Petri dishes at a density of 7 × 105 cells/3 mL in fresh medium. One day later, the cells were incubated at 37°C (NT conditions) and at 39°C (HT conditions) in a HERAcell incubator (Heraeus Instruments, Hanau, Germany). Samples of cells and media from each group were taken at six different time points after incubation (0, 3, 6, 12, 24 and 48 h). The cells were lysed in 40 µL of cell lysis buffer (50 mM Tris/HCl pH 7.4, 250 mM NaCl, 5 mM EDTA, 50 mM NaF, 0.5 mM Na3V04, 10 β-glycerophosphate, 0.5% NP-40, 10% glycerol, 5 mg/mL leupeptin and aprotinin), snap frozen and stored at −70°C.
To quantify the proliferation of the cells used in the experiments, particularly to detect asymmetrical proliferation in the NT and HT groups, the cells were stained with trypan blue and counted using an improved Neubauer haemocytometer at the indicated time points.
ELISA
Quantitative analysis of sTNF-R1 in the cell cultures and in the patients’ serum was performed in Nunc MaxiSorp 96-well plates (Nunc, Roskilde, DK) in duplicate (100 µL sample/well) using an ELISA kit for the detection of human sTNF-R1 (ELISA DuoSet, R&D-Systems, MN) according to the manufacturer's instructions. The samples were analysed spectrophotometrically using an ELX-808 ELISA reader (Bio-Tek Instruments, VT).
Western blot
To determine the levels of membrane-bound TNF-R1 in the cultured cells, we quantified the total protein concentration of the cell lysates by performing a bicinchoninic acid assay (BCA) using the BCA Protein Assay Reagent Kit (Pierce, Rockford, IL). For this purpose, the cell lysates were first denatured in reducing sample buffer for 5 min and then separated by polyacrylamide gel (10%) electrophoresis. The separated proteins were then transferred onto nitrocellulose membranes, incubated with monoclonal anti-human TNF-R1 antibody at a dilution of 1:5000 (R&D-Systems, Rockford, IL), detected by chemiluminescence using the ECL western blotting substrate (Pierce) according to the manufacturer's instructions, and developed onto films, which were analysed densitometrically using Image Master Total Lab software (Version 2.01, Amersham Pharmacia Biotech, Piscataway, NJ).
Statistical analysis
The data were analysed and compared using the Student's t-test with SPSS 15.0 for Windows (Chicago, IL). P-values of <0.05 were considered to be statistically significant.
Results
Increase of patients’ serum sTNF-R1 in the extracorporeal circuit in response to hyperthermia during HILP
The temperature of the systemic circuit remained within a normothermic range throughout the entire procedure (NT: 35.5–36.5°C; HT: 36–37°C). No significant differences of sTNF-R1 in the serum could be detected between the serum samples obtained from the systemic circuit (NT mean: 828.5 pg/mL; HT mean: 805.5 pg/mL) despite the influences associated with the administration of systemic fluid and drugs, the application of general anaesthesia and the surgical intervention ().
Figure 2. Concentration of sTNF-R1 in the serum of HILP-treated patients (n = 23). The levels of sTNF-R1 in the serum increase in the extracorporeal circuit during HT (extremity). There were no changes of serum sTNF-R1 observed in the systemic circuit despite the influence of the anaesthesia and the ongoing surgery (central). Error bars: 95% confidence interval. n.s. = not significant.
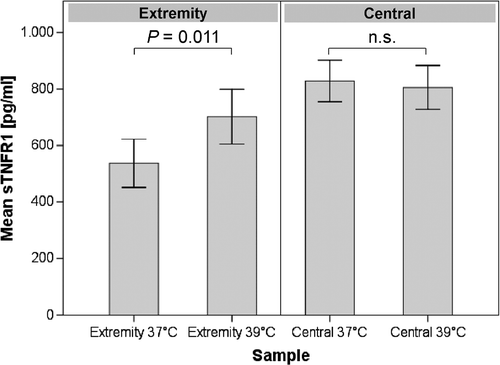
Analysis of the blood samples obtained from the extracorporeal circuit revealed a significant difference in the concentration of sTNF-R1 in the serum between conditions of NT and HT (P = 0.011). The median sTNF-R1 concentration for the samples taken under conditions of NT was 536.9 pg/mL compared to 701.9 pg/mL in the samples taken under conditions of HT, reflecting an increase of 23.5% during hyperthermic conditions ().
The difference in the concentration of sTNF-R1 observed in the extracorporeal and systemic circuits (536.9 pg/mL versus 828.5 pg/mL), which were under normothermic conditions (baseline), is related to the dilution of the extracorporeal circuit with hydroxyethyl starch (HAES). Approximately 500 mL of 10% HAES are required to initiate the extracorporeal circuit prior to cannulation and connection of the circuit to the affected limb.
The matched pairs of blood samples taken from the extracorporeal circuit of 5 different patients when (1) the HLM was started and (2) before undergoing HT (after the HLM had been working for approximately 15 min, ) did not exhibit differences in the serum levels of sTNF-R1 (479.8 pg/mL versus 445 pg/mL, respectively, P = 0.822), which excludes the influence of artificial surfaces/altered blood flow conditions from the HLM or of HAES on sTNF-R1 shedding ().
The cell culture model of HILP does not show changes of sTNF-R1or TNF-R1 levels in response to hyperthermia
As shown in , we observed a clear increase in the levels of sTNF-R1 in the supernatants of HUVECs, HT-1080 cells and PBMNCs. Between the beginning of the experiment (0 h) and 48 h later, the mean levels of sTNF-R1 in the culture supernatants increased from 150 pg/mL to 400 pg/mL in HUVECs, 80 pg/mL to 360 pg/mL in HT-1080 cells and 70 pg/mL to 85 pg/mL in PBMNCs. We suggest that this increase is associated with the accumulation of sTNF-R1 in the culture supernatants due to (1) ongoing cell proliferation (for the HT-1080 cells) and (2) the lack of clearance of sTNF-R1 (for all the cell types), which is normally done by the kidneys and other organs. However, there were no differences of sTNF-R1 in the culture supernatants between the cells whether they were incubated under normothermic or hyperthermic conditions.
Figure 4. Analysis of the concentration of sTNFR-1 in the cell culture supernatants by ELISA. The graphs show the increase in the concentration of sTNF-R1 throughout the duration of the experiment. No detectable differences in the concentration of sTNF-R1 are observed between cells that underwent NT (black symbols) and those that underwent HT (grey symbols).
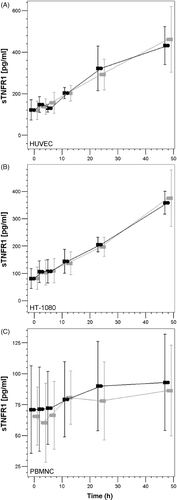
Quantification of the number of HUVECs and PBMNCs revealed that the cells remained in constant numbers during the experiment. In addition, the number of cells did not differ during NT and HT. At 0 h, the density of HUVECs incubated under normothermic and hyperthermic conditions remained at 2.9 × 105/mL. After 48 h, NT-incubated HUVECs had a density of 2.8 × 105/mL, whereas HT-incubated cells had a density of 2.6 × 105/mL. Similarly, NT-incubated PBMNCs at 0 h had a density of 4.7 × 105/mL, and those incubated under HT conditions had a density of 4.4 × 105/mL; at 48 h NT-incubated PBMNCs had a density of 4.7 × 105/mL, and those incubated under HT conditions had a density of 3.8 × 105/mL.
Only HT-1080 cells had a slightly higher proliferation rate after 48 h of exposure to normothermic and hyperthermic conditions (0 h: NT 4.6 × 105/mL and HT 4.0 × 105/mL; 48 h: NT 7.7 × 105/mL and HT 6.6 × 105/mL) but exhibited no temperature-dependent differences in proliferation. Western blot analysis revealed that all the cells expressed measurable levels of membrane-bound TNF-R1 protein. Although the expression levels differed slightly throughout the duration of the experiment, there were no differences in the levels of membrane-bound TNF-R1 protein in any of the cells when they were incubated under normothermic or hyperthermic conditions ().
Figure 5. (A–C) Graphical representation of the densitometric analysis of the protein levels of cellular (membrane-bound) TNF-R1, as determined by western blot. (Bottom panel) Representative western blot of HUVECs. The western blot analysis shows that there are no notable changes in the levels of membrane-bound TNF-R1 when the cells are exposed to HT (NT, black symbols; HT, grey symbols).
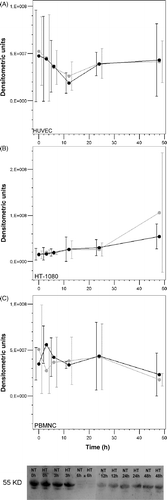
Discussion
In this study we report a significant increase (mean increase: 23.5%) in the levels of sTNF-R1 in the serum of 23 patients who underwent HILP of the limb. We suggest that this increase is most likely associated with the HT that the patients underwent as part of the HILP procedure. However, we failed to confirm our results using a cell culture-based model of HILP.
HILP in combination with the administration of rhTNF-α and melphalan has been reported to result in remarkably high response rates in patients with advanced sarcomas/melanomas of the limbs Citation[3], Citation[5], Citation[24], Citation[25]. Whereas HT and melphalan administration have proven to be indispensable prerequisites for HILP Citation[8], Citation[22], the use of rhTNF-α offers the advantages of helping to deteriorate the tumour vasculature and enhance the uptake of melphalan into the tumour tissue Citation[8], Citation[22], Citation[26–28]. However, little is known about the effect of HT on the function of TNF-α during HILP. Carrol et al. recently reported that HT significantly reduces the procoagulant effects that TNF-α exerts on endothelial tissues Citation[29]. As occurs in diseases involving pyrexia, shedding Citation[15–17], Citation[29] of sTNF-Rs might occur during HT in the extracorporeal circuit, resulting in the subsequent desensitisation of TNF-α target cells (e.g. endothelial cells) and neutralisation of circulating TNF-α.
Some studies have reported that patients who undergo TNF-α-based HILP exhibit sTNF-R shedding, but these studies focused on TNF-α-induced shedding only in the systemic circuit Citation[5], Citation[20], Citation[21]. Gérain and colleagues reported that the baseline levels of sTNF-Rs, prior to the application of TNF-α, were 6.2 ng/mL for sTNF-R1 and 11.3 ng/mL for sTNF-R2 Citation[21]. These results differed from those published by Aderka et al. who found that cancer patients had sTNF-R1 and sTNF-R2 levels of 1.9 ng/mL and 6.4 ng/mL, respectively, in the plasma, whereas healthy patients had 0.79 ng/mL of sTNF-R1 and 3.2 n/mL of sTNF-R2 Citation[18], Citation[19], Citation[21], Citation[30]. In our study, the levels of sTNF-R1 (0.72 ng/mL) taken from the systemic circuit were comparable to those of the healthy controls reported by Aderka et al. Citation[18] but differed quite significantly from their group of cancer patients and those of Gérain et al. Citation[18], Citation[20], Citation[21]. These discrepancies are likely due to the administration of TNF-α prior to measuring the levels of sTNF-Rs in the patients examined by Gérain et al. and to the presence of systemic disease in the patients examined by Aderka et al., conditions that lead to elevated levels of sTNF-R1. Our study revealed that, throughout the HILP procedure, our patients exhibited relatively stable levels of sTNF-R1 in the systemic circuit, indicating that the general anesthesia, surgical trauma and administration of heparin did not have an effect on the shedding of sTNF-R1.
In our study, we found that the levels of sTNF-R1 were significantly lower in the extracorporeal than in the systemic circuit. This result can be due to the dilution of the blood that occurs during the priming procedure in the extracorporeal circuit, which requires the use of approximately 500 mL of 10% HAES Citation[25], Citation[26]. Our results also indicate that shedding of sTNF-R1 does not play a major role in an established extracorporeal circuit under normothermic conditions. In addition, the contact of blood with artificial surfaces or the priming solution did not have an influence on our clin-HILP/sTNF-R1 experiments, which does occur in cardiopulmonary bypasses Citation[30]. Our results indicate that HT is associated with an increase in sTNF-R1 shedding when compared to conditions of NT. However, shedding of sTNF-Rs may have a variable impact on the desensitisation of target cells because no clear correlation exists between receptor density and the response of cells to TNF-α. In addition, the density of the receptor at the cell membrane of the target cells can vary between 200–10,000 receptors per cell Citation[31].
Another possible mechanism of sTNF-Rs involves their ability to neutralise TNF-α, which can disrupt the effects that TNF-α has on the perfused limb (decoy function). Complete neutralisation of circulating TNF-α requires an approximately 100-fold molar excess of sTNF-Rs Citation[5], Citation[21]. Thus, using a sufficient dose of TNF-α is important for TNF-α-based isolated limb perfusion. Based on the dose of TNF-α that was previously deemed to have antitumoural effects (50 mg/kg in experimental mouse models, as published by Lejeune et al.) Citation[22], Citation[26], Citation[28], Citation[32], administration of a high dose of TNF-α in humans (0.25 mg/L of perfused volume) should rapidly exhaust the possible decoy function of sTNF-Rs. Interestingly, Lejeune et al. previously reported that such a high dose of TNF-α would very likely have a saturation effect within the extracorporeal circuit and the tissue of the limb Citation[5], Citation[21]. This finding is in line with our results showing that HT causes an increase in the levels of sTNF-R1 and that some of the TNF-α that is administered into the limb is neutralised by sTNF-Rs. Reflecting on these results and those published by Carroll et al. in which they show that HT decreases the procoagulant activity of TNF-α, we suggest that the use of low-dose TNF-α during HILP Citation[33] should be carefully reconsidered.
In order to better understand the mechanism of HT-induced sTNF-R1 shedding, we conducted in vitro experiments using three different types of cells: HUVECs, HT-1080 cells and PBMNCs. We used HUVECs because endothelial cells are a major target of TNF-α-based antitumour therapy and HT-1080 cells because they have been used by other groups in various models of sarcoma Citation[7], Citation[29], Citation[34–36]. Although we found similar concentrations of cellular TNF-R1 protein on HUVECs, HT-1080 cells and PBMNCs under normothermic and hypothermic conditions, we were unable to confirm the kinetics of TNF-R1 release from cells that we observed in our in vivo analysis. In our opinion, these negative results are of importance because the desensitisation of endothelial cells due to the eradication of membrane-bound TNF-Rs, which can hardly be circumvented by high dose TNF-α, may present a more serious problem. Other groups have demonstrated that shedding of TNF-R1 is induced by TNF-α and interleukin-1β and downstream by MAP kinase signalling pathways and metalloproteinases Citation[37], Citation[38], processes that are also influenced by HT. Our experimental finding that sTNF-R1 accumulates in culture supernatants is likely due to baseline shedding of the receptor and lack of its physiological clearance Citation[31].
The differences between our in vivo and in vitro results are surprising. It seems that our in vivo system does not fully recapitulate the biology of HILP. The simplest explanation for this is that other cells, different from the ones we analysed in vitro, are the source of the elevated sTNF-R1 levels that we observed in the clinical experiments. In particular, other activated leukocytes, such as neutrophils, are possible candidates Citation[39], Citation[40]. However, the reason for the observed differences may be more complex, especially because cell culture experiments cannot fully reproduce the complexity of tumours, where different cell types interact. Thus, HT may require additional factor(s) to effectively induce sTNF-Rs shedding, or it may represent only one link in a cascade of factors that may be involved in sTNF-R1 shedding. For example, the intratumoural microenvironment, which affects tumour response to treatment can be strongly modulated by HT. Major microenvironmental factors are: perfusion, permeability of tumour vessels, pO2, pH, or intratumoural pressure Citation[41–43]. Of these HT induced changes, tumour perfusion and blood flow are probably the most important factors. During mild hyperthermia (39°C) tumour blood flow and oxygenation generally increase, most likely due to vasodilatation of incorporated non-neoplastic arterioles and consecutive opening of the tumour vascular bed Citation[41–45]. Changes in vascular flow may influence endothelial shear stress or the interactions between leukocytes and the endothelial cell layer of tumour vessels. The microenvironment of tumours and HT-induced changes of these factors cannot be recapitulated adequately in simple cell cultures.
We conclude that conditions of HT in the extracorporeal circuit of HILP patients contribute to shedding of sTNF-R1. The large dose of administered and circulating TNF-α may compensate for the neutralisation of a relevant amount of TNF-α. Despite the benefit of in vitro experiments for oncological research, our cell culture-based model of HILP failed to recapitulate the mechanisms of HT-induced sTNF-R shedding that we clearly identified using clinical procedures.
Acknowledgements
The authors wish to thank Heike Uhlenkott for excellent technical assistance.
Declaration of interest: The authors report no conflicts of interest. The authors alone are responsible for the content and writing of the paper.
References
- Williard WC, Collin C, Casper ES, Hajdu SI, Brennan MF. The changing role of amputation for soft tissue sarcoma of the extremity in adults. Surg Gynecol Obstet 1992; 175: 389–396
- Grunhagen DJ, de Wilt JH, Graveland WJ, Verhoef C, van Geel AN, Eggermont AM. Outcome and prognostic factor analysis of 217 consecutive isolated limb perfusions with tumour necrosis factor-alpha and melphalan for limb-threatening soft tissue sarcoma. Cancer 2006; 106: 1776–1784
- Grunhagen DJ, de Wilt JH, ten Hagen TL, Eggermont AM. Technology insight: Utility of TNF-alpha-based isolated limb perfusion to avoid amputation of irresectable tumors of the extremities. Nat Clin Pract Oncol 2006; 3: 94–103
- Bradley JR. TNF-mediated inflammatory disease. J Pathol 2008; 214: 149–160
- Lejeune FJ, Lienard D, Matter M, Ruegg C. Efficiency of recombinant human TNF in human cancer therapy. Cancer Immun 2006; 6: 6
- Eggimann P, Chiolero R, Chassot PG, Lienard D, Gerain J, Lejeune F. Systemic and hemodynamic effects of recombinant tumor necrosis factor alpha in isolation perfusion of the limbs. Chest 1995; 107: 1074–1082
- Menon C, Ghartey A, Canter R, Feldman M, Fraker DL. Tumor necrosis factor-alpha damages tumor blood vessel integrity by targeting VE-cadherin. Ann Surg 2006; 244: 781–791
- de Wilt JH, ten Hagen TL, de Boeck G, van Tiel ST, de Bruijn EA, Eggermont AM. Tumour necrosis factor alpha increases melphalan concentration in tumour tissue after isolated limb perfusion. Br J Cancer 2000; 82: 1000–1003
- Grunhagen DJ, de Wilt JH, Graveland WJ, van Geel AN, Eggermont AM. The palliative value of tumor necrosis factor alpha-based isolated limb perfusion in patients with metastatic sarcoma and melanoma. Cancer 2006; 106: 156–162
- Lejeune FJ, Ruegg C. Recombinant human tumor necrosis factor: An efficient agent for cancer treatment. Bull Cancer 2006; 93: E90–100
- Hehlgans T, Mannel DN. The TNF-TNF receptor system. Biol Chem 2002; 383: 1581–1585
- Wajant H, Pfizenmaier K, Scheurich P. Tumor necrosis factor signaling. Cell Death Differ 2003; 10: 45–65
- Bemelmans MH, van Tits LJ, Buurman WA. Tumor necrosis factor: Function, release and clearance. Crit Rev Immunol 1996; 16: 1–11
- Aderka D. The potential biological and clinical significance of the soluble tumor necrosis factor receptors. Cytokine Growth Factor Rev 1996; 7: 231–240
- Spinas GA, Keller U, Brockhaus M. Release of soluble receptors for tumor necrosis factor (TNF) in relation to circulating TNF during experimental endotoxinemia. J Clin Invest 1992; 90: 533–536
- Godfried MH, van der Poll T, Weverling GJ, Mulder JW, Jansen J, van Deventer SJ, Sauerwein HP. Soluble receptors for tumor necrosis factor as predictors of progression to AIDS in asymptomatic human immunodeficiency virus type 1 infection. J Infect Dis 1994; 169: 739–745
- Kern WV, Engel A, Schieffer S, Prummer O, Kern P. Circulating tumor necrosis factor alpha (TNF), soluble TNF receptors, and interleukin-6 in human subacute bacterial endocarditis. Infect Immun 1993; 61: 5413–5416
- Aderka D, Englemann H, Hornik V, Skornick Y, Levo Y, Wallach D, Kushtai G. Increased serum levels of soluble receptors for tumor necrosis factor in cancer patients. Cancer Res 1991; 51: 5602–5607
- Aderka D, Engelmann H, Shemer-Avni Y, Hornik V, Galil A, Sarov B, Wallach D. Variation in serum levels of the soluble TNF receptors among healthy individuals. Lymphokine Cytokine Res 1992; 11: 157–159
- Aderka D, Sorkine P, Abu-Abid S, Lev D, Setton A, Cope AP, Wallach D, Klausner J. Shedding kinetics of soluble tumor necrosis factor (TNF) receptors after systemic TNF leaking during isolated limb perfusion. Relevance to the pathophysiology of septic shock. J Clin Invest 1998; 101: 650–659
- Gerain J, Lienard D, Pampallona S, Baumgartner M, Ruegg C, Buurman WA, Eggermont A, Lejeune F. Systemic release of soluble TNF receptors after high-dose TNF in isolated limb perfusion. Cytokine 1997; 9: 1034–1042
- Lejeune F, Lienard D, Eggermont A, Schraffordt KH, Rosenkaimer F, Gerain J, Klaase J, Kroon B, Vanderveken J, Schmitz P. Administration of high-dose tumor necrosis factor alpha by isolation perfusion of the limbs. Rationale and results. J Infus Chemother 1995; 5: 73–81
- Boyum A. Isolation of leucocytes from human blood. Further observations. Methylcellulose, dextran, and ficoll as erythrocyteaggregating agents. Scand J Clin Lab Invest Suppl 1968; 97: 31–50
- Taeger G, Grabellus F, Podleska LE, Muller S, Ruchholtz S. Effectiveness of regional chemotherapy with TNF-alpha/melphalan in advanced soft tissue sarcoma of the extremities. Int J Hyperthermia 2008; 24: 193–203
- Eggermont AM, de Wilt JH, ten Hagen TL. Current uses of isolated limb perfusion in the clinic and a model system for new strategies. Lancet Oncol 2003; 4: 429–437
- de Wilt JH, Manusama ER, van Tiel ST, van Ijken MG, ten Hagen TL, Eggermont AM. Prerequisites for effective isolated limb perfusion using tumour necrosis factor alpha and melphalan in rats. Br J Cancer 1999; 80: 161–166
- ten Hagen TL, Eggermont AM. Changing the pathophysiology of solid tumours: The potential of TNF and other vasoactive agents. Int J Hyperthermia 2006; 22: 241–246
- van Horssen R, ten Hagen TL, Eggermont AM. TNF-alpha in cancer treatment: Molecular insights, antitumor effects, and clinical utility. Oncologist 2006; 11: 397–408
- Carroll NM, Elaraj DM, Puhlmann M, Weinreich DM, Turner EM, Xu H, Alexander HR, Jr. Alterations in tumor necrosis factor-induced endothelial cell procoagulant activity by hyperthermia. Int J Cancer 2004; 111: 457–462
- Diegeler A, Doll N, Rauch T, Haberer D, Walther T, Falk V, Gummert J, Autschbach R, Mohr FW. Humoral immune response during coronary artery bypass grafting: A comparison of limited approach, ‘off-pump’ technique, and conventional cardiopulmonary bypass. Circulation 2000; 102(Suppl. 3)III95–III100
- Bemelmans MH, Gouma DJ, Buurman WA. Tissue distribution and clearance of soluble murine TNF receptors in mice. Cytokine 1994; 6: 608–615
- Hoving S, Seynhaeve AL, van Tiel ST, aan de Wiel-Ambagtsheer G, de Bruijn EA, Eggermont AM, ten Hagen TL. Early destruction of tumor vasculature in tumor necrosis factor-alpha-based isolated limb perfusion is responsible for tumor response. Anticancer Drugs 2006; 17: 949–959
- Bonvalot S, Laplanche A, Lejeune F, Stoeckle E, Le Péchoux C, Vanel D, Terrier P, Lumbroso J, Ricard M, Antoni G, et al. Limb salvage with isolated perfusion for soft tissue sarcoma: Could less TNF-alpha be better?. Ann Oncol 2005; 16: 1061–1068
- Ferrero E, Zocchi MR, Magni E, Panzeri MC, Curnis F, Rugarli C, Ferrero ME, Corti A. Roles of tumor necrosis factor p55 and p75 receptors in TNF-alpha-induced vascular permeability. Am J Physiol Cell Physiol 2001; 281: C1173–C1179
- Friedl J, Turner E, Alexander HR, Jr. Augmentation of endothelial cell monolayer permeability by hyperthermia but not tumor necrosis factor: Evidence for disruption of vascular integrity via VE-cadherin down-regulation. Int J Oncol 2003; 23: 611–616
- Gnant MF, Turner EM, Alexander HR, Jr. Effects of hyperthermia and tumour necrosis factor on inflammatory cytokine secretion and procoagulant activity in endothelial cells. Cytokine 2000; 12: 339–347
- Islam A, Adamik B, Hawari FI, Ma G, Rouhani FN, Zhang J, Levine SJ. Extracellular TNFR1 release requires the calcium-dependent formation of a nucleobindin 2-ARTS-1 complex. J Biol Chem 2006; 281: 6860–6873
- Menschikowski M, Hagelgans A, Eisenhofer G, Siegert G. Regulation of endothelial protein C receptor shedding by cytokines is mediated through differential activation of MAP kinase signaling pathways. Exp Cell Res 2009; 315: 2673–2682
- Porteu F, Nathan C. Shedding of tumor necrosis factor receptors by activated human neutrophils. J Exp Med 1990; 172: 599–607
- Porteu F, Hieblot C. Tumor necrosis factor induces a selective shedding of its p75 receptor from human neutrophils. J Biol Chem 1994; 269: 2834–2840
- Hokland SL, Nielsen T, Busk M, Horsman MR. Imaging tumour physiology and vasculature to predict and assess response to heat. Int J Hyperthermia 2010; 26: 264–272
- Song CW, Park HJ, Lee CK, Griffin R. Implications of increased tumor blood flow and oxygenation caused by mild temperature hyperthermia in tumor treatment. Int J Hyperthermia 2005; 21: 761–767
- Vaupel PW, Kelleher DK. Pathophysiological and vascular characteristics of tumours and their importance for hyperthermia: Heterogeneity is the key issue. Int J Hyperthermia 2010; 26: 211–223
- Horsman MR. Tissue physiology and the response to heat. Int J Hyperthermia 2006; 22: 197–203
- Vujaskovic Z, Poulson JM, Gaskin AA, Thrall DE, Page RL, Charles HC, MacFall JR, Brizel DM, Meyer RE, Prescott DM, et al. Temperature‐dependent changes in physiologic parameters of spontaneous canine soft tissue sarcomas after combined radiotherapy and hyperthermia treatment. Int J Radiat Oncol Biol Phys 2000; 46: 179–185