Abstract
Purpose: To compare the effectiveness of microwave ablation (MWA) and multipolar radiofrequency ablation (RFA) in ex vivo porcine livers, in both cases using a pair of internally cooled interstitial applicators.
Materials and methods: MWA was performed on ex vivo porcine livers (n = 60) using a pair of simultaneously powered, internally cooled shaft interstitial antennae. Four power settings were used: 50 W, 60 W, 70 W and 80 W (n = 15 per setting). Multipolar RFA was also performed on ex vivo porcine livers (n = 30), also using a pair of simultaneously powered, internally cooled shaft interstitial bipolar applicators. This was performed for two applicator types T30 (3 cm length) and T40 (4 cm length) at a manufacturer prescribed power of 60 W and 80 W, respectively (n = 15 per applicator). Spacing between the two probes was 2 cm in all cases. Each power setting was applied for 15 ablations for 10 min each. The long-axis diameter (Dl), short-axis diameter (Ds) and the ratio Ds/Dl for each ablation were measured. Temperature data were recorded at 10 positions. Temperature curves were recorded at 3 locations, as well as the time required for the temperature to rise to 50°C.
Results: Dl and Ds for all the power settings of MWA were significantly larger than that of both kinds of multipolar RFA (P < 0.05). The rates of temperature to rise to 50°C in all the MW ablations power settings were significantly faster than those in both multipolar RF ablations.
Conclusion: MWA by the simultaneous application of double antennae may be more advantageous for treating larger liver tumour than multipolar RFA.
Introduction
Hepatocellular carcinoma is one of the most common malignant tumours worldwide, and its incidence is increasing Citation[1]. Surgical resection is regarded as the standard curative treatment of HCC Citation[2]. But only 10% to 37% of the patients are suitable for surgical resection because of multifocal disease, due to proximity of the tumour to major vascular and biliary structures precluding margin-negative surgical resection, extra hepatic metastasis, and poor hepatic functional reserve from the underlying chronic liver diseases or medical comorbidities in many patients Citation[3–6]. In recent years imaging-guided extra thermal ablation using different energy sources such as radiofrequency (RF), microwave (MW) and laser, has become increasingly attractive in treating hepatic malignant tumours and has improved the prognosis in HCC patients Citation[7], Citation[8]. For small HCC (diameter ≤ 3 cm), MWA and RFA had achieved therapeutic effects equivalent to those obtained by curative hepatectomy, meanwhile showing significantly lower complications Citation[9–12]. But for lesions with a diameter larger than 5 cm, complete ablation could be obtained for less than 30% of the lesions, even if the procedure is repeated Citation[13], Citation[14]. In order to reduce the relapse rate or residual tumour, an appropriate safety margin to ensure that the thermally induced coagulation zone covers the entire tumour volume is a challenge Citation[15]. Recent technical advances have facilitated remarkable progress in obtaining a larger ablation zone in RF, such as internally cooled probe, cluster electrodes Citation[16], larger multi-toned expandable electrodes Citation[17], perfusion electrodes Citation[18], or bipolar arrays Citation[15], Citation[19], Citation[20]. Similarly, MWs can create larger zones of ablation with cooled shaft antennae Citation[21], double or multiple antennae Citation[22], Citation[23]. However, to our knowledge, no comparison between simultaneous application of double microwave antennae and multipolar RF ablation has been reported. The purpose of this study was to compare the effectiveness of microwave (MW) ablation and multipolar radiofrequency (RF) ablation both simultaneously using two internally cooled applicators in ex vivo porcine livers.
Materials and methods
Institutional review board approval was not necessary and animal committee approval was unnecessary because an ex vivo porcine liver model was used and the animals were not specifically killed for this study.
Microwave system
A microwave delivery system (FORSEA; Qinghai Microwave Electronic Institute, Nanjing, China) was used in the studies. This system consisted of an MTC-3 microwave generator (FORSEA) with a frequency of 2450 MHz, a power output of 10–150 W, a flexible low-loss cable, and a 14-gauge cooled-shaft antenna. The generator was equipped with two output sources, which allowed simultaneous application of microwave energy through two antennas. The cooled-shaft antenna, which consisted of a 10-cm-long cable connection portion, a 16.5-cm-long shaft coated with Teflon, and a 1.5-cm-long active tip coated with polytetrafluoroethylene, was used to deliver energy to the liver tissue (). The antenna shaft contained two lumina that enabled the delivery of 4°C saline solution to the tip of the shaft and the return of the warmed solution to a 500 mL plastic bag outside the body. A steady-flow pump (BT01–100 LanGe-Pump, Baoding, China) was used to push the chilled saline solution circulating within the lumina of the antenna shaft at 50–60 mL/min. The amount of circulating chilled solution could be adjusted to maintain a mean shaft temperature of 10°C ± 2° (standard deviation).
Figure 1. Photographs of microwave and radiofrequency applicators. (A) Prototype microwave applicators. Microwave antennae are embedded in slit-radiating segments (thick arrows). (B) Two internally cooled bipolar probes T30 (top) and T40 (bottom) with two electrodes (thin arrows) on a single shaft separated by an insulator (arrowhead).
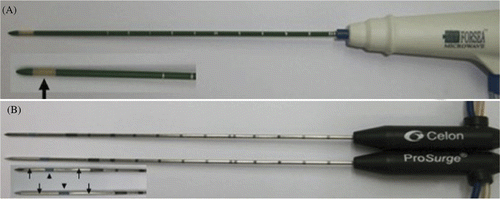
Radiofrequency system
All RF procedures in this study were performed using a 470-kHz (± 10) multipolar RF generator (CelonLab POWER, Teltow, Germany) that provided a maximum power output of 250 W. RF energy was deposited using an internally cooled applicator with two electrodes (CelonPro Surge). When one bipolar applicator is connected to the RF generator, the energy is applied in bipolar mode. When two or three bipolar applicators are connected, the RF system is operating in multipolar mode (). The electrode shaft (diameter 1.8 mm; length, 15 cm) contains two lumina that enable internal fluid circulation. Saline solution was delivered at a rate of 30 mL/min at room temperature by using a peristaltic pump (CelonAquaflowIII). The exposure tip of the bipolar applicator had a length of 3 cm (T30) and 4 cm (T40), which consisted of two uninsulated electrodes that were separated by 3 mm and 4 mm of insulations respectively (), at a manufacturer-prescribed power of 60 W and 80 W, respectively. The parameters of tissue resistance, ablation time and power output were transferred to a personal computer connected to the RF generator and were recorded using a software program, CelonLab PowerTerm, version 2.5.
Temperature measuring system
For each power setting, temperature measurements were performed in 10 of the 15 ablations in the experiment. Three 20-gauge thermistor probes (FORSEA) (response time <1 second, accuracy 0.1°C) were respectively placed at t1, t2 and t3, locations 0 cm, 2 cm and 3 cm from the parallel central line between the two probes, respectively (), to investigate the temperature change in the tissue. Temperatures were recorded at 1-min intervals in 10 of the 15 for each parameter setting. A thin insulation of epoxy resin was used to insulate the metallic shaft of the thermistor probes (the tip of thermocouple for temperature monitoring was exposed) to avoid any interference from the metal in the electric field.
Ex vivo study and protocol
A total of 30 fresh porcine liver blocks (adequate tissue for three ablations per liver) were obtained from a slaughterhouse, and were immersed in a 50 × 20 × 25 cm saline-filled bath at room temperature(20–25°C) before use. The bath container was made of acrylic plate. Microwave antennas and radiofrequency electrodes were inserted at least 8 cm into the liver to ensure that the entire ablation zone would be within the liver parenchyma, and the two applicators had the same implantation depth and power output. Placement of the probes too close to large vessels or biliary ducts was avoided. Every power setting was applied for 15 ablations and 10 min heating duration. After ablation, the liver blocks containing lesions were dissected along the two parallel needle tracks. All ablation procedures were performed by three authors (X.L., L.Z. and H.J.) with three, eight and two years of experience with thermal ablation procedures, respectively.
Microwave ablation and protocol
Under direct visualization, two microwave antennae were simultaneously inserted into the liver tissue and a rigid template was used to maintain antenna spacing and parallel insertion. Microwave ablations (n = 60) were induced by using different power outputs (50 W, 60 W, 70 W, 80 W). The spacing between the two antennae was 2 cm.
Radiofrequency ablation and protocol
Similar to microwave ablation, two cool-tip bipolar electrodes were simultaneously inserted into the liver tissue under direct visualisation. Precise spacing and parallelism of the bipolar applicators was assured using an acrylic puncture aid. One group had two cool-tip bipolar radiofrequency applicators with a 3-cm (T30) active tip, and another group had a 4-cm (T40) active tip. Multipolar radiofrequency ablations (n = 30) were performed under pulsed-energy mode with a rated power of 60 W and 80 W to facilitate the most ideal ablation zone according to the manufacturer's recommendations, respectively. In both cases the spacing of the two electrodes was 2 cm.
Evaluation of lesion diameters, shape and temperature data
The ablated zones were sectioned along the two parallel needles. We assessed whether coagulation was confluent, partially confluent, or non-confluent. The long-axis diameters (Dl, along the needle insertion axis) and short-axis diameters (Ds, perpendicular to longitudinal plane) of the coagulation zone were assessed macroscopically with calipers. The sphericity index may be simplified to the short/long axis (Ds/Dl). A perfect sphere has an index of 1.0, and ellipsoids have indexes of <1.0. Each ablated area consisted of three visually distinctive zones, which were the centre zone (charring zone), white zone (coagulation zone),and red zone (congestion zone), respectively. The diameters were measured from the zone of complete necrosis (the centre charring zone and white coagulation zone), not from the surrounding red congestion zone of partial necrosis Citation[24]. Temperature curves at location t1, t2, t3 were recorded. The time to rise to 50°C was recorded at location t2. We did not record the time to rise to 50°C at location t1, t3 because the temperature at location t1 reaches 60°C very fast, inducing irreversible cellular destruction almost instantaneously, whereas the temperature at location t3 did not reach 50°C within 10 min in some of the settings. The results of all diameter measurements were made in consensus of the two investigators (W.J.F. and M.Z.) with nine and seven years of experience with thermal ablation procedures, respectively.
Statistical analysis
Results of lesion diameters and temperature data were reported as means ± standard deviations (SDs). Differences in the Dl, Ds and Ds/Dl ratio between groups were evaluated by independent samples t-test. Differences in the time of temperature to rise to 50°C at location t2 were also evaluated by independent samples t-test. All statistical analyses were performed using SPSS 16.0 statistical software (Chicago, IL). P values less than 0.05 were considered to indicate a statistically significant difference.
Results
Evaluation of lesion size and shape
All coagulation zones were ellipsoidal (when the applicators were placed too close to large large vessels or biliary ducts, inconsistent lesion size was generated; we excluded those from the analysis and another ablation was performed to replace these). MW ablation achieved larger coagulation zone than that of multipolar RF ablation. A wider and deeper colour of arrow-shaped charring around the shaft in MW mode than that in RF mode was apparent (). In all ablations of a duration of 10 min (), the Dl and Ds for all the power settings of microwave ablation were significantly larger than those of both kinds of multipolar radiofrequency ablations (P < 0.05). As the power increased, the microwave ablation zone showed an increasing tendency. The ratios Ds/Dl were almost all >0.85, so both in microwave ablation and multipolar radiofrequency, using two applicators simultaneously tended to produce more spherical-shaped ablation zone. The ratio of Ds/Dl of T30 multipolar radiofrequency ablation was significantly larger than that of all power settings of microwave ablation, but the T40 multipolar radiofrequency ablation had no significant difference from all power settings of microwave ablation. Compared with multipolar radiofrequency, microwave ablations tended to produce larger coagulation zones, but T30 multipolar radiofrequency ablation tended to produce more spherical ablation zones than all microwave ablations.
Figure 4. Photograph shows gross specimen of porcine liver tissue treated with MW and RF ablation in ex vivo study, which was produced by using two internally cooled probes with intervals of 2 cm for a duration of 10 min. The charring is arrow-shaped. (A) MW ablation with two probes at 50 W. (B) MW ablation with two probes at 60 W. (C) MW ablation with two probes at 70 W. (D) MW ablation with two probes at 80 W. (E) multipolar RF ablation with two T30 probes and rated power 60 W. (F) multipolar RF ablation with two T40 probes and rated power 80 W.
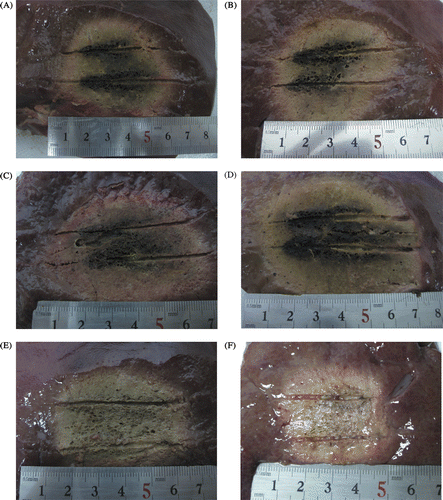
Table I. Technical parameters of microwave ablation and multipolar RF.
Evaluation of temperature effect
All the temperature at location t1, t2, t3 correlated with the ablation duration in a sigmoidal curve fashion. Temperature for approximately heating 5 or 6 min reached its relative plateau (). Larger power in microwave ablation and longer active tip in multipolar radiofrequency ablation tended to produce the highest temperatures. All power settings in microwave ablation produced higher temperature than multipolar radiofrequency ablation (P < 0.05), except that comparison highest temperature (°C) 0 cm from the parallel central line between the two applicators of the power settings (50 W) of microwave ablation and both the T30 and T40 multipolar radiofrequency ablation (P > 0.05). The maximum temperatures in all microwave ablations for all power settings were higher than those in multipolar radiofrequency ablations (P < 0.05). Besides, the comparison of maximum temperature (°C) at location t1 in 50 W microwave ablation and the T30 or T40 multipolar radiofrequency ablation did not reach statistical significance (P > 0.05) (). The rates of temperature at location t2 to rise to 50°C in all the MW ablations power settings were significantly faster than those in both multipolar RF ablations.
Figure 5. Graphs show the different temperature rising curves in all ablation settings. (A) The temperature rising curve t1 of all ablations. (B) The temperature rising curve t2 of all ablations. (C) The temperature rising curve t3 of all ablations.
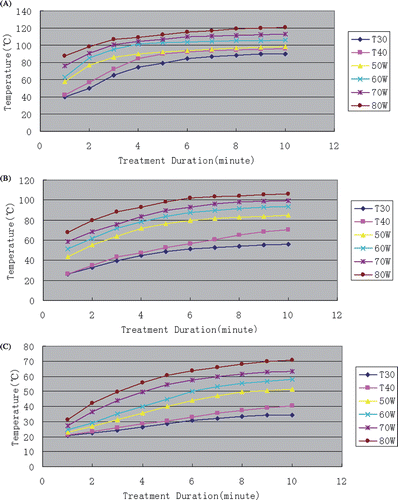
Table II. Coagulation necrosis parameters of the MWA and multipolar RFA.
Discussion
Image-guided thermal ablation is a reliable option for the treatment of primary and metastatic hepatic malignant tumours because of its effectiveness, safety in percutaneous, laparoscopic or open surgical access, and relative ease of use. Regardless of the primary energy source (microwave, radiofrequency or laser) of the thermal ablation, all of these modalities induce cellular destruction by means of the direct effects of heat, with irreversible cellular damage occurring at temperatures above 50°C, when applied for 4–6 min and almost instantaneously at temperatures above 60°C Citation[25].
However, MWA has several theoretical advantages over RFA in consistently higher intratumoural temperatures, larger ablation volumes, faster ablation times, better penetration depth, less reliant on conduction of electricity into tissue and energy delivery less limited by the electrical impedances of tumour tissue and less affected by the heat-sink effect that is thought to contribute to local recurrence after RF ablation Citation[26–29]. Moreover, MWA offers the ability to simultaneously place multiple antennae without interference and had a synergistic effect when compared with sequential placement of three antennae, and also appeared to preferentially ablate perivascular tissue Citation[28], Citation[30]. But the bipolar RF system used in our study also has its advantages. The combination of multiple applicators in multipolar mode encourages larger coagulations with a single RF ablation Citation[15]. And the electrical current is closed between the electrodes so the grounding pad is not required, and not contraindicated by the metallic materials like surgical clips or pacemaker. So we could place the thermistor probes in a short distance from the radiofrequency probes in our study, but not without influencing the electric field.
We choose 50 W, 60 W, 70 W and 80 W as ideal power settings in MWA for clinical application for our MWA system Citation[21]. The RFA unit in our study has a maximum power of 250 W, but that does not mean higher power will generate larger ablation zone. In our RFA system, continuous application of RF energy leads to an inverse relationship between volume of coagulation and power output Citation[31] and faster RF ablations at higher power outputs, which produce smaller volumes of coagulation Citation[15]. If we apply the 100 W, 120 W, 140 W and 160 W(effectively 50 W, 60 W, 70 W and 80 W) in multipolar RFA to be identical to the power applied in MWA, we will get smaller volumes of coagulation in comparison with applying the manufacturer prescribed power of 60 W and 80 W, and the difference with MWA will be even more significant. So we choose low power settings of 60 W, 80 W for multipolar RFA to facilitate most ideal ablation zones according to the commercial recommendations, but not 100 W, 120 W, 140 W, 160 W, applied power being the same for the two methods.
Jie Yu et al. Citation[32] had compared 2450 MHz and 915 MHz MWA with bipolar RFA in ex vivo and in vivo porcine livers and concluded that MWA may have higher potential for complete destruction of liver tumours than RF ablation. We designed this study to compare the simultaneous application of double microwave antennae with multipolar RFA. As Andrew et al. Citation[12] and Wang et al. Citation[33] reported that prolonged application could not result in significant enlargement of the coagulation diameter for in vivo tissue, 10 min was suitable for observing the effectiveness of MWA. Thus, an application time of 10 min was chosen for MWA in this study. To compare in the same unit-time limit, an application time of 10 min was also used in multipolar RFA. In all ablations of 10 min, all MWA generated significantly larger ablation zones than that both kinds of multipolar RFA (P < 0.05). The results showed MWA provides faster ablation times, larger ablation volumes and stronger thermal efficiency within the same 10 min than multipolar RF ablation. To get a larger volume of coagulation for multipolar RFA may need prolonged RF ablation at lower power outputs Citation[15].
Because most tumours’ morphological characteristics are spherical, the shape of ablation should also approximate a sphere in order to generate a sufficient ablative margin while minimising the destruction of normal surrounding tissue. One limitation of thermal ablation is its inability to produce a large enough short axis diameter of coagulation necrosis and global ablation zones to encompass hepatic tumours with an appropriate ablative margin at a single thermal ablation application Citation[21]. In our study, the ratios of short to long axis, Ds/Dl, were almost all >0.85, the results showed that using two probes simultaneously tended to generate longer short axis diameter and more spherical ablation zone both in MWA and multipolar RFA. That may be advantageous for treating large liver tumour in one ablation session.
The power settings (60 W, 70 W, 80W) in MWA produced higher highest temperatures in every temperature measuring spot. In the MWA for all power settings, the time for the temperature at location t2 to rise to 50°C took less time than those in both multipolar RFA, which indicated that MWA had a higher temperature rising rate. Although there is no statistical difference between the highest temperature at the centre of two applicators both in 50 W MWA and T40 multipolar RFA, 50 W MWA had a faster temperature rising rate than multipolar RFA, which applied to other power settings.
Since microwave ablation does not rely on conduction of electricity into tissue, it is not limited by charring. Therefore, temperatures greater than 100°C are readily achieved in MWA, which potentially results in a larger zone of ablation, shorter treatment time, and more complete tumour kill. On the other hand the RFA lower temperature resulted in an inefficient transformation of electrical energy into heat, especially at tissue-vessel interfaces where flowing blood thermally protects perivascular tissue and tumour, known as the heat-sink effect. Especially given the highly vascular nature of the liver, most large tumours will be in close proximity to at least one large blood vessel. We deduce that higher temperature and faster temperature rising rate may result in MWA being less affected by the heat-sink effect and cut down local recurrence after multipolar RFA. But further experimental in vivo corroboration is needed.
In a recent publication by Huang and colleagues [34], the authors reported that adoption of multiple-antenna MWA may generate inconsistent results and the unpredictable performance may be related to interactions between concurrently powered antennas. This phenomenon also occurred in our study, but that is usually because there are large vessels or biliary ducts in the ablation zones. Placement of the probes too close to large vessels or biliary ducts was avoided in our study and we only chose the consistent results. So the phenomenon would not influence the results from our study.
This study has some limitations. Firstly, similar to other experimental studies, this study used normal porcine liver as a proxy for human liver tumours. The size and morphological characteristics might not be extrapolated to clinical practice, as liver tumours, as well as cirrhotic liver have different tissue compositions and vascularity. Secondly, farther inter-antenna distance for the merging of a large enough zone of ablation without clefts was not compared. In addition, the simultaneous application of two antennae also increases probability of complications or at least complicates the application.
In conclusion, MWA by the simultaneous application of double antennae could generate a larger ablation zone in ex vivo porcine liver without clefts between the two antennae than multipolar radiofrequency ablation. MWA by the simultaneous application of double antennae may be more advantageous for treating larger liver tumour in one ablation session than multipolar RFA.
Declaration of interest: The authors report no conflicts of interest. The authors alone are responsible for the content and writing of the paper.
References
- Peto J. Cancer epidemiology in the last century and the next decades. Nature 2001; 411: 390–395
- El-Serag HB, Mason AC. Rising incidence of hepatocellular carcinoma in the United States. N Engl J Med 1999; 340: 745–750
- Bruix J, Sherman M. Management of hepatocellular carcinoma. Hepatology 2005; 42: 1208–1236
- Fan ST, Lo CM, Liu CL, Lam CM, Yuen WK, Yeung C, Wong J. Hepatectomy for hepatocellular carcinoma: Toward zero hospital deaths. Ann Surg 1999; 229: 322–330
- Colella G, Bottelli R, De Carlis L, Sansalone CV, Rondinara GF, Alberti A, Belli LS, Gelosa F, Iamoni GM, Rampoldi A, et al. Hepatocellular carcinoma: Comparison between liver transplantation, resective surgery, ethanol injection, and chemoembolization. Transplant Int 1998; 11(Suppl 1)S193-6
- Fong Y, Sun RL, Jarnagin W, Blumgart LH. An analysis of 412 cases of hepatocellular carcinoma at a western center. Ann Surg 1999; 229: 790–799, discussion 799–800
- Wright AS, Sampson LA, Warner TF, Mahvi DM, Lee FT Jr. Radiofrequency versus microwave ablation in a hepatic porcine model. Radiology 2005; 236: 132–139
- Kuriyama H, Okada S, Okusaka T, Ueno H, Ikeda M. Prognostic factors in patients with small hepatocellular carcinoma treated by percutaneous ethanol injection. J Gastroenterol Hepatol 2002; 17: 1205–1210
- Liu FY, Yu XL, Liang P, Wang Y, Zhou P, Yu J. Comparison of percutaneous 915 MHz microwave ablation and 2450 MHz microwave ablation in large hepatocellular carcinoma. Int J Hyperthermia 2010; 26(5)448–455
- Yu Z, Liu W, Fan L, Shao J, Huang Y, Si X. The efficacy and safety of percutaneous microwave coagulation by a new microwave delivery system in large hepatocellular carcinomas: Four case studies. Int J Hyperthermia 2009; 25(5)392–398
- Shibata T, Iimuro Y, Yamamoto Y, Maetani Y, Ametani F, Itoh K, Konishi J. Small hepatocellular carcinoma: Comparison of radiofrequency ablation and percutaneous microwave coagulation therapy. Radiology 2002; 223: 331–337
- Hines-Peralta AU, Pirani N, Clegg P, Cronin N, Ryan TP, Liu Z, Goldberg SN. Microwave ablation: Results with a 2.45-GHz applicator in ex vivo bovine and in vivo porcine liver. Radiology 2006; 239: 94–102
- Livraghi T, Goldberg SN, Lazzaroni S, Meloni F, Ierace T, Solbiati L, Gazelle GS. Hepatocellular carcinoma: Radiofrequency ablation of medium and large lesions. Radiology 2000; 214: 761–768
- Curley SA. Radiofrequency ablation of malignant liver tumor. Ann Surg Oncol 2003; 10(4)338–347
- Clasen S, Schmidt D, Boss A, Dietz K, Kröber SM, Claussen CD, Pereira PL. Multipolar radiofrequency ablation with internally cooled electrodes: Experimental study in ex vivo bovine liver with mathematic modeling. Radiology 2006; 238: 881–890
- Goldberg SN, Solbiati L, Hahn PF, Cosman E, Conrad JE, Fogle R, Gazelle GS. Large-volume tissue ablation with radiofrequency by using a clustered, internally cooled electrode technique: Laboratory and clinical experience in liver metastases. Radiology 1998; 209: 371–379
- Arata MA, Nisenbaum HL, Clark TW, Soulen MC. Percutaneous radiofrequency ablation of liver tumors with the LeVeen probe: Is roll-off predictive of response?. J Vasc Interv Radiol 2001; 12: 455–458
- Raman SS, Aziz D, Chang X, Ye M, Sayre J, Lassman C, Lu DS. Minimizing central bile duct injury during radiofrequency ablation: Use of intraductal chilled saline perfusion – Initial observations from a study in pigs. Radiology 2004; 232: 154–159
- Frericks BB, Ritz JP, Roggan A, Wolf KJ, Albrecht T. Multipolar radiofrequency ablation of hepatic tumors: Initial experience. Radiology 2005; 237: 1056–1062
- Lee JM, Han JK, Kim SH, Son KR, Kim HC, Kim SJ, Choi BI. In vivo efficiency of multipolar radiofrequency ablation with two bipolar electrodes: A comparative experimental study in pig kidney. J Vasc Interv Radiol 2007; 18(12)1553–1560
- Kuang M, Lu MD, Xie XY, Xu HX, Mo LQ, Liu GJ, Xu ZF, Zheng YL, Liang JY. Liver cancer: Increased microwave delivery to ablation zone with cooled-shaft antenna: Experimental and clinical studies. Radiology 2007; 242(3)914–924
- Shi W, Liang P, Zhu Q, Yu X, Shao Q, Lu T, Wang Y, Dong B. Microwave ablation: Results with double 915 MHz antennae in ex vivo bovine Livers. Eur J Radiol 2010, doi:10.1016/j.ejrad.2010.03.015
- Wright AS, Lee FT, Mahvi DM. Hepatic microwave ablation with multiple antennae results in synergistically larger zones of coagulation necrosis. Ann Surg Oncol 2003; 10: 275–283
- Simon CJ, Dupuy DE, Mayo-Smith WW. Microwave ablation: Principles and applications. Radiographics 2005; 25: S69–S83
- Goldberg SN, Gazelle GS, Mueller PR. Thermal ablation therapy for focal malignancy: A unified approach to underlying principles, techniques, and diagnostic imaging guidance. Am J Roentgenol 2000; 174: 323–331
- Shock SA, Meredith K, Warner TF, Sampson LA, Wright AS, Winter TC, 3rd, Mahvi DM, Fine JP, Lee FT Jr. Microwave ablation with loop antenna: In vivo porcine liver model. Radiology 2004; 231(1)143–149
- Wright AS, Sampson LA, Warner TF, Mahvi DM, Lee FT Jr. Radiofrequency versus microwave ablation in a hepatic porcine model. Radiology 2005; 236(1)132–139
- Emami B, Stauffer P, Dewhirst MW, Prionas S, Ryan T, Corry P, Herman T, Kapp DS, Myerson RJ, Samulski T, et al. RTOG quality assurance guidelines for interstitial hyperthermia. Int J Radiat Oncol Biol Phys 1991; 20(5)1117–1124
- Nan Q, Zheng W, Fan Z, Liu Y, Zeng Y. Analysis to a critical state of thermal field in microwave ablation of liver cancer influenced by large vessels. Int J Hyperthermia 2010; 26(1)34–38
- Clasen S, Geng A, Herberts T, Boss A, Schmidt D, Schraml C, Fritz J, Kröber SM, Claussen CD, Pereira PL. Internally cooled bipolar radiofrequency ablation: Is a lower power output more effective?. Rofo 2007; 179(3)282–288
- Yu J, Liang P, Yu X, Liu F, Chen L, Wang Y. A comparison of microwave ablation and bipolar radiofrequency ablation both with an internally cooled probe: Results in ex vivo and in vivo porcine livers. Eur J Radiol 2009, doi:10.1016/j.ejrad.2009.12.009
- Wang Y, Sun Y, Feng L, Gao Y, Ni X, Liang P. Internally cooled antenna for microwave ablation: Results in ex vivo and in vivo porcine livers. Eur J Radiol 2005; 67: 357–361
- Huang Y, Sampson L, Andreano A, Brace CL. Microwave ablation: an experimental comparison between time-interleaved and concurrent arrays. Paper presented at the World Conference on Interventional Oncology 2010 (The Loews Philadelphia Hotel, Philadelphia, Pennsylvania, USA, June 9–12, 2010). Available at http://www.wcio2011.com/2010/25.cgi (accessed Sep 24, 2010)