Abstract
Photodynamic therapy (PDT) is an effective treatment for superficial epithelial skin cancers and is also being utilised for skin rejuvenation. PDT with porphyrin-derived photosensitisers may be capable of inducing rapid cytochrome c release initiating apoptotic cascade via an activation of different caspases. Hsp70 has been demonstrated to directly bind to the caspase recruitment domain (CARD) of apoptotic-protease activating factor 1 (Apaf-1), thereby preventing the recruitment of oligomerisation of Apaf-1 and association of Apaf-1 with procaspase 9. Further, cytoplasmic Hsp70 rapidly translocates to the cell surface where it stabilises damaged membranes and preserves their integrity depending on the PDT dose. The induced cell surface expression and release of Hsp70 and its relevance for tumor response or skin rejuvenation is not fully understood, but seems to be of interest for monitoring, predicting or optimising treatment regimens. This review aims to summarise the current knowledge on PDT mediated cell signalling.
Introduction
Photodynamic therapy (PDT) is a promising treatment for certain types of cancer and other non-malignant conditions, which involves the administration of a photosensitising agent (one of the porphyrin-based compounds is 5-aminolevulinic acid (ALA) which is a precursor of protoporphyrin IX (PpIX)). The photosensitiser accumulates in more rapidly proliferating cells causing injury to various cutaneous targets (). Subsequent exposure to photoactivating light induces the generation of reactive oxygen species, particularly singlet oxygen, with following apoptotic or necrotic cell death Citation[1], Citation[2]. Furthermore, photosensitisers are often taken up with some selectivity by malignant or dysplastic tissues and light can be targeted to the appropriate tissue Citation[1] limiting the damage to the surrounding normal tissue.
Figure 1. Porphyrin metabolism under physiologic conditions (A) and in tumour cells during ALA-based PDT (B).
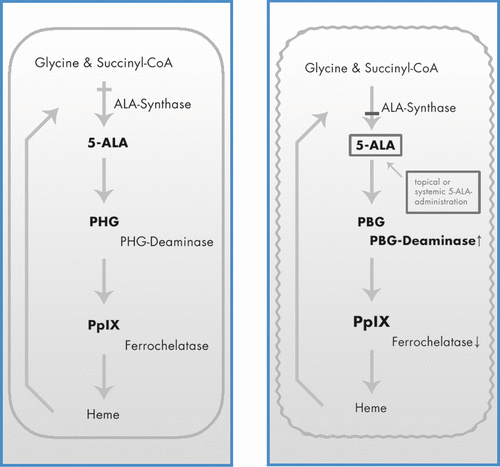
The absorption spectrum of porphyrins are in the Soret band ranging from 360 nm to 400 nm as well as in Q-bands between 500 nm and 635 nm. The usually used red light with a wavelength of 630 nm has a maximal tissue penetration of approximately 2–3 mm Citation[3–5] (). Non-coherent light sources, emitting light in a broader arrangement of wavelengths, can improve the efficacy of the treatment due to the absorption in several wavelengths. Wavelengths of 700 nm to 800 nm reach cells as deep as 1 to 2 cm under the epidermal surface Citation[6–8]. Light at wavelengths longer than 850 nm does not lead to sufficient photochemical response in deeper tissue sections due to the weak absorption of photosensitisers of these wavelengths.
PDT has been used as an effective organ preserving treatment for actinic keratoses, Bowen's disease and superficial basal cell carcinomas (BCCs) Citation[4], Citation[9]. PDT is used because of its minimal invasiveness, easy repeatability and favourable aesthetic results. Other advantages of PDT in dermatology include the simultaneous treatment of multiple tumours and the relatively short healing time. One of the most important side effects is considerable pain. Apart from the therapy of cancers, PDT is also being utilised for skin rejuvenation Citation[3], Citation[4].
Numerous studies have been attempted to identify subcellular PDT targets. These efforts have successfully identified mitochondria, lysosomes and various cytoplasmic membranes among the primary targets of PDT-mediated damage, depending on the particular photosensitiser and dose. Furthermore, the sensitivity of cells to PDT varies between cell types such as kerantinocytes or fibroblasts. PDT also activates several signal transduction pathways such as protein kinase cascades, cytokine expression, expression of early response genes, induction of glucose-regulated proteins and heat shock proteins leading to apoptosis Citation[9], Citation[10].
In PDT-induced apoptosis, cytoplasmic Hsp70 rapidly translocates to the cell surface after treatment, depending on the PDT dose. Moreover, the translocation of Hsp70 is related to the changes in mitochondrial transmembrane potential. With non-lethal PDT-induced surface stress, Hsp70 also translocates to the cell surface, but with a slower rate and a lower final surface-concentration Citation[9], Citation[10].
The induced cell surface expression and release of Hsp70 including its relevance for tumour response and skin rejuvenation is not fully understood Citation[9–11] but may be of interest for monitoring, predicting or optimising treatment regimes. For this reason we were interested in giving an overview of the working mechanism of PDT, the role of HSPs during PDT as a non-thermal therapy with the foci of tumour eradication and skin rejuvenation.
Molecular mechanism of PDT
In the case of PDT-induced apoptosis, translocated cytoplasmic Hsp70 to the cell surface has been shown to have a crucial role in tumour treatment response Citation[9], Citation[10]. Additionally, it initiates cell reparation and wound healing leading ultimately to collagen remodelling Citation[12], Citation[13].
HSPs belong to a large family of protein chaperones involved in assisting protein folding and unfolding in cells. In addition to the induction by hyperthermia, HSPs are activated by a wide range of physical, chemical and biological stresses including various laser therapies or PDT Citation[14–23].
Increasing levels of HSPs enhance the ability of cells to deal with the resultant accumulation of abnormally folded proteins, either facilitating the refolding of damaged proteins or participating in the synthesis of new proteins to replace those irreparably damaged. The most important HSPs are the family members of Hsp70 (72 kDa = Hsp72 and 73 kDa = Hsp73) and Hsp47. Both, Hsp72 and Hsp73 are present in the cytoplasm and nucleus of keratinocytes, fibroblasts and adipocytes. Hsp73 is synthesised constitutively in all mammalian cells and therefore is often referred to as the ‘constitutive Hsp70’. The synthesis of Hsp72 is usually restricted to the cell experiencing stress and therefore often is referred to as the ‘inducible Hsp70’. The two proteins exhibit extremely high sequence homology (95%) and similar biochemical properties Citation[19]. The inducible response involves transcriptional activation mediated by heat shock factor (HSF) which binds to a specific heat shock element in the heat shock gene leading to the up-regulation of HSP after PDT Citation[21–23].
Hsp47 is up-regulated at 42°C and higher temperatures Citation[17] and is the only known stress molecule able to bind collagen Citation[24]. It is found in the endoplasmic reticulum of cells producing collagen type I Citation[25] and is actively involved in collagen type I biosynthesis Citation[26]. Expression of Hsp47 is age related and its up-regulation by heat in older organisms is reduced Citation[27]. Various conditions lead to the involvement of Hsp47 in the collagen metabolic pathway resulting in the modulation of collagen I synthesis rate Citation[28–30]. Hsp47 was not up-regulated in in vitro fibroblasts after conventional PDT compared with a PDT that caused collagen damage consistent with the hypothesis that this collagen chaperone is usually up-regulated by modalities known to damage collagen such as laser hyperthermia Citation[31], Citation[32].
The molecular machinery that drives the apoptotic programme consists of a family of cysteine proteases, caspases, which cleave their substrates at specific aspartic acid residues Citation[33]. In normal cells, caspases are constitutively expressed as inactive single polypeptide chains, procaspases, and their activation requires specific proteolytic cleavage Citation[34], Citation[35]. Active caspases can amplify apoptotic events by their ability to cleave their own precursor forms as well as those of other caspases Citation[36], Citation[37]. Mitochondria have a crucial function in initiating the cascade of caspase activation in response to different apoptotic signals Citation[38]. Disruption of the outer mitochondrial membrane by apoptotic stimuli results in the release of cytochrome c into the cytoplasm Citation[39]. Cytochrome c binds to the cytosolic apoptotic-protease activating factor 1 (Apaf-1), promoting Apaf-1-mediated activation of caspase 9 in an adenosine triphosphate/deoxyadenosine diphosphate (ATP/dADP)-dependent manner Citation[40], Citation[41]. Active caspase 9 can subsequently amplify the caspase cascade by its ability to process its own proenzyme as well as the effector caspase 3 Citation[42]. Association of procaspase 9 with Apaf-1 is an essential step in its initial activation and is mediated by the CARD sequence located at the amino termini of both proteins Citation[43] ().
Hsp70 as well as Hsp90 have been demonstrated to directly bind to the caspase recruitment domain (CARD) of Apaf-1, thereby preventing the oligomerisation of Apaf-1 and association of Apaf-1 with procaspase 9 Citation[44–46]. Treatment of cells with diverse DNA-damaging agents dissociates the Hsp90–Apaf-1 complex relieving the inhibition of procaspase-9 activation. Release of cytochrome c from mitochondria by apoptotic signals induces ATP/dADP-dependent formation of the oligomeric Apaf-1-caspase-9 apoptosome, thereby activating caspase 3 Citation[4], Citation[47–49]. Furthermore, Hsp90 binding to Apaf-1 induces a potential conformational change in Apaf-1 and inhibits cytochrome c-ATP/dADP-mediated oligomerisation of Apaf-1, thereby exerting a dominant-negative effect on the function of Apaf-1 to activate procaspase-9 Citation[44]. In receptor-mediated apoptosis, caspase 8 is mobilised before cytochrome c release in both normal and transformed cell types during PDT-induced apotosis.
A particularly pronounced surface Hsp70 expression was found on apoptotic cells and even more striking on detached dying cells 18 h post-PDT Citation[10]. The cell surface expression of Hsp70 on keratinocytes and fibroblasts seems be to dose-dependent, persisting at a similar level between 15 and 120 min Citation[50] up to 6 h post-PDT. Hsp70 expression was not detectable in control samples exposed to the photosensitiser or light source alone Citation[10]. Under non-lethal PDT-induced surface stress, Hsp70 also translocated to the cell surface, but with a slower rate and a lower final surface concentration Citation[47]. The reason for this rapid emergence of surface-expressed Hsp70 remains to be elucidated. It is possible that photo-oxidative lesions in the membrane of PDT-treated cells cause the inner membrane sections to invert to the outer surface Citation[51] and expose HSP molecules associated with proteins or lipids lining the inner leaflet of the cell membrane. On the other hand, surface localised HSPs may have a role in the efforts of cells to stabilise damaged membranes and preserve their integrity Citation[52]. When Hsp70 is expressed at the cell surface, the COOH-terminal domain is exposed whereas the ATP-binding site and NH2-terminal domain remain unexposed within the membrane Citation[10], Citation[53]. The COOH-terminal domain is involved in the interaction of HSP with its chaperoned client peptides that may serve as a source of tumour rejection antigens Citation[54], Citation[55]. Hence, altered or damaged cell proteins are more likely to be found attached to the substrate-binding domain of HSP than normal non-damaged polypeptides Citation[56] and could become abundantly exposed to immune surveillance elements following PDT. The level of Hsp70 expression in cancer cells was found to correlate with their immunogenicity Citation[57] whereas specifically membrane expressed Hsp70 on such cells was shown to elicit tumour immunity and also correlate with sensitivity to allogeneic natural killer cells Citation[58–61]. Further, the opsonisation of PDT-treated cells is reduced in the presence of Hsp70-specific antibodies Citation[62]. Hsp70 directly binds complement proteins Citation[63]. Hence, the opsonisation of PDT-treated cells by complement proteins may occur by direct binding to surface-expressed Hsp70 that were recognised as altered self targets by the complement. Moreover, various types of immune cells including macrophages, neutrophils, dendritic, natural killer and B cells have specific receptors for complement proteins as well as for HSPs Citation[64] and are prone to use them to attack cells that are surface-expressing HSPs and/or are opsonised by complement proteins. In addition to the elevated expression of Hsp70 by cancer cells themselves Citation[60], Citation[61], Citation[65], Citation[66]. Tumours like squamous cell carcinomas contain significant numbers of tumour-associated leukocytes or are newly infiltrated because PDT induces a massive infiltration of these inflammatory cells Citation[67]. Hsp70 released from PDT-treated tumour cells is captured by macrophages triggering in these cells toll-like receptor-based signal transduction (TLR2 and TLR4) activity with downstream activation of NF-κB, resulting in the production of inflammatory cytokine tumour necrosis factor α Citation[1]. PDT inhibits Hsp90 from stabilising client proteins modulating tumour responsiveness following PDT Citation[68].
Furthermore, PDT induces matrix metalloproteinases directly but also by an indirect paracrine loop mediated by soluble factors released by epidermal keratinocytes Citation[69]. MMP1 initiates collagen breakdown and its gene expression was elevated 1 day post-PDT while returning to baseline levels within the following 24 h Citation[70]. Prolyl-4-hydroxylase α, an enzyme involved in post-translational modification of collagen in fibroblasts, was significantly elevated 1 month after PDT Citation[71]. It catalyses the hydroxylation of proline residues. This is required for collagen synthesis Citation[72],Citation[73] and correlates with Hsp47 Citation[71].
PDT and the treatment of epithelial cancers
PDT is effective in the treatment of actinic keratoses, Bowen's disease and superficial BCCs, and has been approved in the USA for actinic keratoses since December 1999 Citation[11], Citation[74–76].
PDT is a non-thermal photochemical process and therefore does not cause deep tissue heating. This fact in combination with no or superficial eschar formation leads to excellent cosmetic outcomes. PDT is also considered a cost-effective therapy and may be an option in patients for whom surgery is not feasible Citation[77].
However, in the therapy of BCCs, PDT is only recommended to patients with histological proven superficial and non-pigmented BCCs because two basic factors limit its application within cutaneous oncology Citation[2]. First, the complete destruction of a tumour by PDT depends on a sufficiently high concentration and uniform distribution of PpIX in the malignant tissue. ALA is a hydrophilic molecule with a stratum corneum/water partition coefficient of 0.1 Citation[78] and permeates poorly across intact skin Citation[3]. Tumour cells have an increased metabolic rate and their ‘low ferrochelatase activity’ results in a reduced depletion of PPIX into haeme leading to a higher ALA content than in the surrounding normal cells.
Deep penetrating tumours such as nodular BCCs can be reached theoretically by systemically administered ALA; however, prolonged cutaneous photosensitivity is the major drawback Citation[76], Citation[79]. The second limiting factor is the penetration depth reached by the different light sources (see indroduction) Citation[1]. To resolve these problems, the tumour mass can be reduced before PDT, photosensitisers with an increased tissue penetration potential have to be developed, they have to be applied deeper in the tissue using special techniques or have to be used in combination with deep penetrating wavelengths.
For this reason, PDT has been combined with different laser modalities. Different lasers (Er:YAG-, CO2-, flashlamp-pumped dye laser) are themselves effective in the ablation of non-malignant, pre-malignant, and malignant tumours Citation[80–83] and can reduce the tumour mass before PDT as seen in nodular BCCs Citation[84]. Combining Er:YAG laser ablation (600–1000 mJ at a frequency of 7 Hz) reducing tumour size below 2 mm with subsequent ALA methyl ester PDT was significantly more effective than the two treatments alone, defined at 3, 6 and 12 months with a final efficacy of 98.97% versus 94.85% (PDT only) and 91.75% (Er:YAG laser only) Citation[85]. The combined method also provided the best aesthetic results compared to PDT only and Er:YAG laser only, although 67% of patients preferred solitary Er:YAG laser treatment over the PDT method (20%) and the combined treatment (13%) because of its simplicity Citation[85].
Furthermore, the ALA permeation can be increased by different chemical enhancers like dimethylsulphoxide Citation[79], Citation[86], iontophoresis Citation[87–91] or destruction of the cornified layer. The formation of PpIX was also found to be strongly temperature dependent Citation[38] with more formation at higher temperatures. Another approach to the problem is the pre-treatment of the tumour with a fractionated laser before application of ALA to enhance its tissue penetration depth. ALA applied to Er:YAG laser-treated skin produced a significantly higher accumulation of PpIX within superficial skin and subcutaneous tumours as compared to the non-treated group measured by confocal laser scanning microscopy. The pre-treatment with the Er:YAG laser at 1.4–2.0 J/cm2 enhanced the PpIX concentration within the BCCs at 2 h post-application. On the other hand, there was no significant difference in PpIX accumulation at 4 and 6 h between laser and non-laser treated BCCs Citation[92].
PDT utilises the destructive power of reactive oxygen species generated via visible light irradiation of a photosensitive dye accumulated in the cancerous tissue/cells and up-regulates HSPs including Hsp70, Hsp47 and Hsp90. Simultaneously, PDT leads to the infiltration of various immune cells (e.g. neutrophils) into the treated site as well as to the activation of different immune phenomena, e.g. acute-phase response, complement cascade and production of cytokines/chemokines Citation[93–95].
PDT and skin rejuvenation
Based on the good healing and anecdotal reports of the reversal of some of the signs of photodamage post-PDT, PDT is being used for skin rejuvenation although the exact mechanism of the improvements of the appearance of sun-damaged skin has not been fully elucidated. There are a few studies which could show an improvement of skin texture and tone as well as improvement in skin elasticity Citation[4–6], Citation[96]. The results are similar to the changes induced by topical retinoids occuring more rapidly Citation[97–99]. PDT stimulated epidermal proliferation, resulting in an increase in epidermal thickness. This was parallelled by an up-regulation of Ki 67 and cytokeratin 16 2 to 7 days post-PDT. Also, procollagen I and III mRNA was up-regulated followed by the up-regulation of the corresponding proteins. As a marker of photodamage, elevated p53 might imply greater loss of normal barrier function resulting in a greater conversion of ALA to PpIX in the skin Citation[71]. Because measurements of epidermal thickness and collagen I production returned to baseline values within a few months, maintenance therapy would be required to maintain clinical benefits. An increased amount of collagen could be due to the induction of Hsp70 and subsequent increase of Hsp47 such as after thermal ablative and thermal, non-ablative laser therapies Citation[100–102], but has not been investigated until now.
Outlook
PDT is an effective treatment alternative for actinic keratoses, Bowen's disease and superficial BCCs as well as skin rejuvenation besides other more invasive therapy options.
In the case of PDT-induced apoptosis, translocated cytoplasmic Hsp70 to the cell surface has been shown to have a crucial role in treatment response Citation[9], Citation[10] due to its inhibitiory effect of both apoptotic and necrotic pathways Citation[103]. Monitoring of Hsp70 expressions and other influencing factors such as skin cooling in clinical trials seem to be of interest to optimise treatment regimens for both indications.
In the future, combinations of PDT with fractionated laser regimen or other skin rejuvenation therapies could possibly improve clinical outcome in skin rejuvenation indications. Further, clinical studies comparing different light sources and photosensitisers seem to be important to optimise treatment outcome.
Declaration of interest: The authors report no conflicts of interest. The authors alone are responsible for the content and writing of the paper.
References
- Dougherty TJ, Gomer CJ, Henderson BW, Jori G, Kessel D, Korbelik M, Moan J, Peng Q. Photodynamic therapy. J Natl Cancer Inst 1998; 90: 889–905
- Peng Q, Warloe T, Berg K, Moan J, Kongshaug M, Giercksky KE, Nesland JM. 5-Aminolevulinic acid-based photodynamic therapy. Clinical research and future challenges. Cancer 1997; 79: 2282–2308
- Dover JS, Bhatia AC, Stewart B, Arndt KA. Topical 5-aminolevulinic acid combined with intense pulsed light in the treatment of photoaging. Arch Dermatol 2005; 141: 1247–1252
- Touma DJ, Gilchrest BA. Topical photodynamic therapy: A new tool in cosmetic dermatology. Semin Cutan Med Surg 2003; 22: 124–130
- Dougherty TJ. Photodynamic therapy – new approaches. Semin Surg Oncol 1989; 5: 6–16
- Driver I, Lowdell CP, Ash DV. In vivo measurement of the optical interaction coefficients of human tumours at 630 nm. Phys Med Biol 1991; 36: 805–813
- Wilson BC, Patterson MS. The physics of photodynamic therapy. Phys Med Biol 1986; 31: 327–360
- Frazier CC. Photodynamic therapy in dermatology. Int J Dermatol 1996; 35: 312–316
- Nonaka M, Ikeda H, Inokuchi T. Inhibitory effect of heat shock protein 70 on apoptosis induced by photodynamic therapy in vitro. Photochem Photobiol 2004; 79: 94–98
- Korbelik M, Sun J, Cecic I. Photodynamic therapy-induced cell surface expression and release of heat shock proteins: Relevance for tumor response. Cancer Res 2005; 65: 1018–1026
- Calzavara-Pinton PG. Repetitive photodynamic therapy with topical delta-aminolaevulinic acid as an appropriate approach to the routine treatment of superficial non-melanoma skin tumours. J Photochem Photobiol B 1995; 29: 53–57
- Laplante AF, Moulin V, Auger FA, Landry J, Li H, Morrow G, Tanguay RM, Germain L. Expression of heat shock proteins in mouse skin during wound healing. J Histochem Cytochem 1998; 46: 1291–1301
- Shi Y, Massague J. Mechanisms of TGF-beta signaling from cell membrane to the nucleus. Cell 2003; 113: 685–700
- Blake MJ, Gershon D, Fargnoli J, Holbrook NJ. Discordant expression of heat shock protein mRNAs in tissues of heat-stressed rats. J Biol Chem 1990; 265: 15275–15279
- Maytin EV, Wimberly JM, Anderson RR. Thermotolerance and the heat shock response in normal human keratinocytes in culture. J Invest Dermatol 1990; 95: 635–642
- Morimoto RI. Cells in stress: Transcriptional activation of heat shock genes. Science 1993; 259: 1409–1410
- Nagata K, Saga S, Yamada KM. A major collagen-binding protein of chick embryo fibroblasts is a novel heat shock protein. J Cell Biol 1986; 103: 223–229
- O'Connell-Rodwell CE, Shriver D, Simanovskii DM, McClure C, Cao YA, Zhang W, Bachmann MH, Beckham JT, Jansen ED, Palanker D, et al. A genetic reporter of thermal stress defines physiologic zones over a defined temperature range. FASEB J 2004; 18: 264–271
- Welch WJ. Mammalian stress response: Cell physiology, structure/function of stress proteins, and implications for medicine and disease. Physiol Rev 1992; 72: 1063–1081
- Wilmink GJ, Opalenik SR, Beckham JT, Abraham AA, Nanney LB, Mahadevan-Jansen A, Davidson JM, Jansen ED. Molecular imaging-assisted optimization of hsp70 expression during laser-induced thermal preconditioning for wound repair enhancement. J Invest Dermatol 2009; 129: 205–216
- Gomer CJ, Ryter SW, Ferrario A, Rucker N, Wong S, Fisher AM. Photodynamic therapy-mediated oxidative stress can induce expression of heat shock proteins. Cancer Res 1996; 56: 2355–2360
- Curry PM, Levy JG. Stress protein expression in murine tumor cells following photodynamic therapy with benzoporphyrin derivative. Photochem Photobiol 1993; 58: 374–379
- Gomer CJ, Ferrario A, Hayashi N, Rucker N, Szirth BC, Murphree AL. Molecular, cellular, and tissue responses following photodynamic therapy. Lasers Surg Med 1988; 8: 450–463
- Nagata K, Hirayoshi K, Obara M, Saga S, Yamada KM. Biosynthesis of a novel transformation-sensitive heat-shock protein that binds to collagen. Regulation by mRNA levels and in vitro synthesis of a functional precursor. J Biol Chem 1988; 263: 8344–8349
- Shroff B, Smith T, Norris K, Pileggi R, Sauk JJ. Hsp 47 is localized to regions of type I collagen production in developing murine femurs and molars. Connect Tissue Res 1993; 29: 273–286
- Natsume T, Koide T, Yokota S, Hirayoshi K, Nagata K. Interactions between collagen-binding stress protein Hsp47 and collagen. Analysis of kinetic parameters by surface plasmon resonance biosensor. J Biol Chem 1994; 269: 31224–31228
- Miyaishi O, Ito Y, Kozaki K, Sato T, Takechi H, Nagata K, Saga S. Age-related attenuation of Hsp47 heat response in fibroblasts. Mech Ageing Dev 1995; 77: 213–226
- Takechi H, Hirayoshi K, Nakai A, Kudo H, Saga S, Nagata K. Molecular cloning of a mouse 47-kDa heat-shock protein (Hsp47), a collagen-binding stress protein, and its expression during the differentiation of F9 teratocarcinoma cells. Eur J Biochem 1992; 206: 323–329
- Masuda H, Fukumoto M, Hirayoshi K, Nagata K. Coexpression of the collagen-binding stress protein Hsp47 gene and the alpha 1(I) and alpha 1(III) collagen genes in carbon tetrachloride-induced rat liver fibrosis. J Clin Invest 1994; 94: 2481–2488
- Sauk JJ, Van Kampen CL, Norris K, Foster R, Somerman MJ. Expression of constitutive and inducible Hsp70 and Hsp47 is enhanced in cells persistently spread on OPN1 or collagen. Biochem Biophys Res Commun 1990; 172: 135–142
- Verrico AK, Moore JV. Expression of the collagen-related heat shock protein Hsp47 in fibroblasts treated with hyperthermia or photodynamic therapy. Br J Cancer 1997; 76: 719–724
- Verrico AK, Haylett AK, Moore JV. In vivo expression of the collagen-related heat shock protein Hsp47, following hyperthermia or photodynamic therapy. Lasers Med Sci 2001; 16: 192–198
- Alnemri ES. Mammalian cell death proteases: A family of highly conserved aspartate specific cysteine proteases. J Cell Biochem 1997; 64: 33–42
- Cryns V, Yuan J. Proteases to die for. Genes Dev 1998; 12: 1551–1570
- Thornberry NA, Lazebnik Y. Caspases: Enemies within. Science 1998; 281: 1312–1316
- Fernandes-Alnemri T, Armstrong RC, Krebs J, Srinivasula SM, Wang L, Bullrich F, Fritz LC, Trapani JA, Tomaselli KJ, Litwack G, et al. In vitro activation of CPP32 and Mch3 by Mch4, a novel human apoptotic cysteine protease containing two FADD-like domains. Proc Natl Acad Sci USA 1996; 93: 7464–7469
- Stennicke HR, Jurgensmeier JM, Shin H, Deveraux Q, Wolf BB, Yang X, Zhou Q, Ellerby HM, Ellerby LM, Bredesen D, et al. Pro-caspase-3 is a major physiologic target of caspase-8. J Biol Chem 1998; 273: 27084–27090
- Green DR, Reed JC. Mitochondria and apoptosis. Science 1998; 281: 1309–1312
- Goldstein JC, Waterhouse NJ, Juin P, Evan GI, Green DR. The coordinate release of cytochrome c during apoptosis is rapid, complete and kinetically invariant. Nat Cell Biol 2000; 2: 156–162
- Saleh A, Srinivasula SM, Acharya S, Fishel R, Alnemri ES. Cytochrome c and dATP-mediated oligomerization of Apaf-1 is a prerequisite for procaspase-9 activation. J Biol Chem 1999; 274: 17941–17945
- Zou H, Li Y, Liu X, Wang X. An Apaf-1.cytochrome c multimeric complex is a functional apoptosome that activates procaspase-9. J Biol Chem 1999; 274: 11549–11556
- Srinivasula SM, Ahmad M, Fernandes-Alnemri T, Litwack G, Alnemri ES. Molecular ordering of the Fas-apoptotic pathway: The Fas/APO-1 protease Mch5 is a CrmA-inhibitable protease that activates multiple Ced-3/ICE- like cysteine proteases. Proc Natl Acad Sci USA 1996; 93: 14486–14491
- Cain K, Brown DG, Langlais C, Cohen GM. Caspase activation involves the formation of the aposome, a large (approximately 700 kDa) caspase-activating complex. J Biol Chem 1999; 274: 22686–22692
- Pandey P, Saleh A, Nakazawa A, Kumar S, Srinivasula SM, Kumar V, Weichselbaum R, Nalin C, Alnemri ES, Kufe D, et al. Negative regulation of cytochrome c-mediated oligomerization of Apaf-1 and activation of procaspase-9 by heat shock protein 90. EMBO J 2000; 19: 4310–4322
- Saleh A, Srinivasula SM, Balkir L, Robbins PD, Alnemri ES. Negative regulation of the Apaf-1 apoptosome by Hsp70. Nat Cell Biol 2000; 2: 476–483
- Beere HM, Wolf BB, Cain K, Mosser DD, Mahboubi A, Kuwana T, Tailor P, Morimoto RI, Cohen GM, Green DR. Heat-shock protein 70 inhibits apoptosis by preventing recruitment of procaspase-9 to the Apaf-1 apoptosome. Nat Cell Biol 2000; 2: 469–475
- Avram DK, Goldman MP. Effectiveness and safety of ALA-IPL in treating actinic keratoses and photodamage. J Drugs Dermatol 2004; 3: S36–S39
- Alster TS, Tanzi EL, Welsh EC. Photorejuvenation of facial skin with topical 20% 5-aminolevulinic acid and intense pulsed light treatment: A split-face comparison study. J Drugs Dermatol 2005; 4: 35–38
- Ochsner M. Photodynamic therapy: The clinical perspective. Review on applications for control of diverse tumorous and non-tumorous diseases. Arzneimittelforschung 1997; 47: 1185–1194
- Zhou F, Xing D, Chen WR. Dynamics and mechanism of Hsp70 translocation induced by photodynamic therapy treatment. Cancer Lett 2008; 264: 135–144
- John K, Schreiber S, Kubelt J, Herrmann A, Muller P. Transbilayer movement of phospholipids at the main phase transition of lipid membranes: Implications for rapid flip-flop in biological membranes. Biophys J 2002; 83: 3315–3323
- Tsvetkova NM, Horvath I, Torok Z, Wolkers WF, Balogi Z, Shigapova N, Crowe LM, Tablin F, Vierling E, Crowe JH, et al. Small heat-shock proteins regulate membrane lipid polymorphism. Proc Natl Acad Sci USA 2002; 99: 13504–13509
- Botzler C, Li G, Issels RD, Multhoff G. Definition of extracellular localized epitopes of Hsp70 involved in an NK immune response. Cell Stress Chaperones 1998; 3: 6–11
- Hartl FU, Hayer-Hartl M. Molecular chaperones in the cytosol: From nascent chain to folded protein. Science 2002; 295: 1852–1858
- Linderoth NA, Popowicz A, Sastry S. Identification of the peptide-binding site in the heat shock chaperone/tumor rejection antigen gp96 (Grp94). J Biol Chem 2000; 275: 5472–5477
- Menoret A, Chaillot D, Callahan M, Jacquin C. Hsp70, an immunological actor playing with the intracellular self under oxidative stress. Int J Hyperthermia 2002; 18: 490–505
- Clark PR, Menoret A. The inducible Hsp70 as a marker of tumor immunogenicity. Cell Stress Chaperones 2001; 6: 121–125
- Chen X, Tao Q, Yu H, Zhang L, Cao X. Tumor cell membrane-bound heat shock protein 70 elicits antitumor immunity. Immunol Lett 2002; 84: 81–87
- Multhoff G, Botzler C, Wiesnet M, Muller E, Meier T, Wilmanns W, Issels RD. A stress-inducible 72-kDa heat-shock protein (Hsp72) is expressed on the surface of human tumor cells, but not on normal cells. Int J Cancer 1995; 61: 272–279
- Ciocca DR, Clark GM, Tandon AK, Fuqua SA, Welch WJ, McGuire WL. Heat shock protein hsp70 in patients with axillary lymph node-negative breast cancer: Prognostic implications. J Natl Cancer Inst 1993; 85: 570–574
- Jaattela M. Escaping cell death: Survival proteins in cancer. Exp Cell Res 1999; 248: 30–43
- Cecic I, Korbelik M. Deposition of complement proteins on cells treated by photodynamic therapy in vitro. J Environ Pathol Toxicol Oncol 2006; 25: 189–203
- Prohaszka Z, Singh M, Nagy K, Kiss E, Lakos G, Duba J, Fust G. Heat shock protein 70 is a potent activator of the human complement system. Cell Stress Chaperones 2002; 7: 17–22
- Asea A. Chaperokine-induced signal transduction pathways. Exerc Immunol Rev 2003; 9: 25–33
- Dunning AM, Healey CS, Pharoah PD, Teare MD, Ponder BA, Easton DF. A systematic review of genetic polymorphisms and breast cancer risk. Cancer Epidemiol Biomarkers Prev 1999; 8: 843–854
- Wei YQ, Zhao X, Kariya Y, Teshigawara K, Uchida A. Inhibition of proliferation and induction of apoptosis by abrogation of heat-shock protein (Hsp) 70 expression in tumor cells. Cancer Immunol Immunother 1995; 40: 73–78
- Krosl G, Korbelik M, Dougherty GJ. Induction of immune cell infiltration into murine SCCVII tumour by photofrin-based photodynamic therapy. Br J Cancer 1995; 71: 549–555
- Ferrario A, Gomer CJ. Targeting the 90 kDa heat shock protein improves photodynamic therapy. Cancer Lett 2010; 289: 188–194
- Karrer S, Bosserhoff AK, Weiderer P, Landthaler M, Szeimies RM. Keratinocyte-derived cytokines after photodynamic therapy and their paracrine induction of matrix metalloproteinases in fibroblasts. Br J Dermatol 2004; 151: 776–783
- Karrer S, Bosserhoff AK, Weiderer P, Landthaler M, Szeimies RM. Influence of 5-aminolevulinic acid and red light on collagen metabolism of human dermal fibroblasts. J Invest Dermatol 2003; 120: 325–331
- Orringer JS, Hammerberg C, Hamilton T, Johnson TM, Kang S, Sachs DL, Fisher G, Voorhees JJ. Molecular effects of photodynamic therapy for photoaging. Arch Dermatol 2008; 144: 1296–1302
- Pinnel SR, Murad S, Darr D. Induction of collagen synthesis by ascorbic acid. A possible mechanism. Arch Dermatol 1987; 123: 1684–1686
- Riekki R, Harvima IT, Jukkola A, Risteli J, Oikarinen A. The production of collagen and the activity of mast-cell chymase increase in human skin after irradiation therapy. Exp Dermatol 2004; 13: 364–371
- Nestor MS, Gold MH, Kauvar AN, Taub AF, Geronemus RG, Ritvo EC, Goldman M P, Gilbert DJ, Richey DF, Alster TS, et al. The use of photodynamic therapy in dermatology: Results of a consensus conference. J Drugs Dermatol 2006; 5: 140–154
- Szeimies RM, Sassy T, Landthaler M. Penetration potency of topical applied delta-aminolevulinic acid for photodynamic therapy of basal cell carcinoma. Photochem Photobiol 1994; 59: 73–76
- Kennedy JC, Pottier RH. Endogenous protoporphyrin IX, a clinically useful photosensitizer for photodynamic therapy. J Photochem Photobiol B 1992; 14: 275–292
- Kalka K, Merk H, Mukhtar H. Photodynamic therapy in dermatology. J Am Acad Dermatol 2000; 42: 389–416
- Tsai JC, Chen IH, Wong TW, Lo YL. In vitro/in vivo correlations between transdermal delivery of 5-aminolaevulinic acid and cutaneous protoporphyrin IX accumulation and effect of formulation. Br J Dermatol 2002; 146: 853–862
- Peng Q, Warloe T, Moan J, Heyerdahl H, Steen HB, Nesland JM, Giercksky KE. Distribution of 5-aminolevulinic acid-induced porphyrins in noduloulcerative basal cell carcinoma. Photochem Photobiol 1995; 62: 906–913
- Jiang SB, Levine VJ, Nehal KS, Baldassano M, Kamino H, Ashinoff RA. Er:YAG laser for the treatment of actinic keratoses. Dermatol Surg 2000; 26: 437–440
- Iyer S, Friedli A, Bowes L, Kricorian G, Fitzpatrick RE. Full face laser resurfacing: Therapy and prophylaxis for actinic keratoses and non-melanoma skin cancer. Lasers Surg Med 2004; 34: 114–119
- Horlock N, Grobbelaar AO, Gault DT. Can the carbon dioxide laser completely ablate basal cell carcinomas? A histological study. Br J Plast Surg 2000; 53: 286–293
- Humphreys TR, Malhotra R, Scharf MJ, Marcus SM, Starkus L, Calegari K. Treatment of superficial basal cell carcinoma and squamous cell carcinoma in situ with a high-energy pulsed carbon dioxide laser. Arch Dermatol 1998; 134: 1247–1252
- Whitaker IS, Shokrollahi K, James W, Mishra A, Lohana P, Murison MC. Combined CO2 laser with photodynamic therapy for the treatment of nodular basal cell carcinomas. Ann Plast Surg 2007; 59: 484–488
- Smucler R, Vlk M. Combination of Er:YAG laser and photodynamic therapy in the treatment of nodular basal cell carcinoma. Lasers Surg Med 2008; 40: 153–158
- Peng Q, Berg K, Moan J, Kongshaug M, Nesland JM. 5-Aminolevulinic acid-based photodynamic therapy: Principles and experimental research. Photochem Photobiol 1997; 65: 235–251
- Lopez RF, Bentley MV, gado-Charro MB, Guy RH. Iontophoretic delivery of 5-aminolevulinic acid (ALA): Effect of pH. Pharm Res 2001; 18: 311–315
- Moan J, Berg K, Gadmar OB, Iani V, Ma L, Juzenas P. The temperature dependence of protoporphyrin IX production in cells and tissues. Photochem Photobiol 1999; 70: 669–673
- Rhodes LE, Tsoukas MM, Anderson RR, Kollias N. Iontophoretic delivery of ALA provides a quantitative model for ALA pharmacokinetics and PpIX phototoxicity in human skin. J Invest Dermatol 1997; 108: 87–91
- Lieb S, Szeimies RM, Lee G. Self-adhesive thin films for topical delivery of 5-aminolevulinic acid. Eur J Pharm Biopharm 2002; 53: 99–106
- Auner BG, Valenta C, Hadgraft J. Influence of lipophilic counter-ions in combination with phloretin and 6-ketocholestanol on the skin permeation of 5-aminolevulinic acid. Int J Pharm 2003; 255: 109–116
- Shen SC, Lee WR, Fang YP, Hu CH, Fang JY. In vitro percutaneous absorption and in vivo protoporphyrin IX accumulation in skin and tumors after topical 5-aminolevulinic acid application with enhancement using an erbium:YAG laser. J Pharm Sci 2006; 95: 929–938
- Garg AD, Nowis D, Golab J, Agostinis P. Photodynamic therapy: Illuminating the road from cell death towards anti-tumour immunity. Apoptosis 2010; 15: 1050–1071
- Merchant S, Korbelik M. Heat shock protein 70 is acute phase reactant: Response elicited by tumor treatment with photodynamic therapy. Cell Stress Chaperones 2011; 16: 153–162
- Uehara M, Inokuchi T, Tobita T, Ohba S, Asahina I. Expression of heat shock protein 47 in the fibrous tissue adjacent to mouse tumour subjected to photodynamic therapy. Oral Oncol 2007; 43: 804–810
- Gold MH, Bradshaw VL, Boring MM, Bridges TM, Biron JA. Split-face comparison of photodynamic therapy with 5-aminolevulinic acid and intense pulsed light versus intense pulsed light alone for photodamage. Dermatol Surg 2006; 32: 795–801
- Rittie L, Varani J, Kang S, Voorhees JJ, Fisher GJ. Retinoid-induced epidermal hyperplasia is mediated by epidermal growth factor receptor activation via specific induction of its ligands heparin-binding EGF and amphiregulin in human skin in vivo. J Invest Dermatol 2006; 126: 732–739
- Kang S, Voorhees JJ. Photoaging therapy with topical tretinoin: An evidence-based analysis. J Am Acad Dermatol 1998; 39: S55–S61
- Griffiths CE, Russman AN, Majmudar G, Singer RS, Hamilton TA, Voorhees JJ. Restoration of collagen formation in photodamaged human skin by tretinoin (retinoic acid). N Engl J Med 1993; 329: 530–535
- Helbig D, Bodendorf MO, Grunewald S, Kendler M, Simon JC, Paasch U. Immunohistochemical investigation of wound healing in response to fractional photothermolysis. J Biomed Opt 2009; 14: 064044
- Helbig D, Moebius A, Simon JC, Paasch U. Nonablative skin rejuvenation devices and the role of heat shock protein 70: Results of a human skin explant model. J Biomed Opt 2010; 15: 038002
- Helbig D, Simon JC, Paasch U. Epidermal and dermal changes in response to various skin rejuvenation methods. Int J Cosmet Sci. Jun 21, 2010, doi: 10.1111/j.1468-2494.2010.00573.x. [Epub ahead of print]
- Takayama S, Reed JC, Homma S. Heat-shock proteins as regulators of apoptosis. Oncogene 2003; 22: 9041–9047