Abstract
Purpose: It is widely accepted that chronic stress can induce anxiety; however, the cellular and molecular mechanisms of stress-induced anxiety are far from being elucidated. Hyperthermia has been shown to induce expression of heat shock proteins (HSPs) to provide protection against a variety of stresses. To our knowledge, the effect of hyperthermia on the development of chronic unpredictable stress (CUS)-induced anxiety has not been studied. This study was to determine the relationship between hyperthermia induced Hsp72 and CUS related anxiety.
Materials and methods: Heat shock factor 1 knockout (hsf1−/−) and wild-type (hsf1+/+) mice were subjected to CUS with or without hyperthermia treatment. Anxiety-like behaviours were evaluated by elevated plus maze and open field tests. Apoptosis in the hippocampal CA3 area was detected by TUNEL staining. Hsp72 protein level in the hippocampus was measured by Western blot.
Results: CUS caused significant apoptosis in hippocampal CA3 cells in both hsf1−/− and hsf1+/+ mice, which significantly correlated with anxiety-like behaviours. Hyperthermia induced Hsp72 expression in hsf1+/+ mice, but not in hsf1−/− mice. Importantly, hyperthermia protected hsf1+/+ mice against developing CUS-related anxiety-like behaviours and reduced CUS-induced apoptosis in hippocampal CA3 cells. In contrast, hyperthermia exhibited no protective role in hsf1−/− mice.
Conclusions: Apoptosis of hippocampal CA3 cells is involved in the development of anxiety-like behaviours underlying CUS. Hsp72 protein is a crucial player in the protective effect of hyperthermia against CUS-induced apoptosis and development of anxiety-like behaviours. Our study suggests hyperthermia is an effective treatment for CUS-induced mood disorders.
Introduction
Stress is associated with a greater risk for the development of mental illnesses and contributes both to the etiology and the severity of mental diseases Citation[1–3]. For example, early life events, such as sexual and physical abuse, can increase several-fold the risk of developing clinical depression during adulthood Citation[4], Citation[5]. Chronic stress is widely recognised as a risk factor for the development of major depression and anxiety disorders Citation[6]. Moreover, unpredictable stressors exhibit greater negative impact on people than predictable stressors Citation[7]. Chronic stress is proposed to induce CA3 dendritic retraction and CA3 or dentate gyrus (DG) cell apoptosis, which then disrupts hypothalamic-pituitary-adrenal axis activity leading to changes in mood and memory Citation[8], Citation[9].
Animal studies have also demonstrated that exposure to stress increases behaviours associated with anxiety or depression. For example, following exposure to chronic unpredictable stress (CUS) for 14 consecutive days, rats showed a significant increase in anxiety-like behaviour in an elevated plus maze Citation[7]. Exposure to CUS also leads to depression-like behaviour in rats Citation[10]. Our previous study demonstrated that exposure to CUS for 8 weeks significantly increased anxiety-like behaviours in mice Citation[11]. Importantly, chronic stress-induced behavioural profiles in rodents may be reflective of human psychopathology like anxiety, depression and post-traumatic stress disorders Citation[12–14]. A CUS animal model has been suggested as an appropriate animal model for anxiety disorder Citation[12]. However, the key molecules and cells involved in the development of chronic unpredictable stress-induced anxiety have not yet been elucidated.
Early views on the development of stress-induced anxiety suggested that the disturbance of neurotransmitters was an important factor for mood disorders. As such, clinical therapies to treat anxiety were primarily focused on the modulation of neurotransmitters. Recently, the stress-induced impairment of structural plasticity and cellular resilience was proposed as a critical mechanism for stress-induced mood disorders Citation[15]. Prolonged psychosocial stresses are known to induce apoptosis or synaptic plasticity in the hippocampus, which results in impairment in hippocampus-dependent functions and induces anxiety-like behaviours Citation[16], Citation[17]. Moreover, multiple signalling pathways that regulate cell survival and cell death are considered important targets of anti-anxiety treatment Citation[18]. Our recent study revealed that CUS induced more anxiety-like behaviours in hsf1-null mice compared to wild-type mice, which was associated with apoptosis of hippocampal CA3 cells Citation[11]. However, the molecular mechanisms underlying stress induced apoptosis and anxiety have not been identified.
Hsf1-null mice are unable to synthesize heat shock proteins (HSP) due to the deletion of hsf1 gene. In wild-type mice, HSP synthesis is rapidly increased in response to stressors and this response is facilitated by the activation of HSFs, which bind to the hsp promoter element Citation[11]. HSPs are a set of highly conserved proteins and were originally identified as responsible proteins to hyperthermia. Subsequently, HSP synthesis was found to be induced by a variety of physiological and environmental stressors. Newly synthesised HSPs provide protective effects against stress. For example, exposure of primary cultured neuronal cells to mild hyperthermia induces overexpression of HSPs Citation[19] to protect cells from glutamate toxicity Citation[20], Citation[21] or severe heat exposure Citation[19]. In animal models hyperthermia has been shown to protect the brain from damage caused by ischaemia Citation[22]. Recently, Hsp72 was identified as a main HSP involved in the regulation of mood disorders during thermal stress Citation[23], Citation[24]. However, whether treatment with hyperthermia, and more specifically the induction of Hsp72, can protect hippocampus cells against CUS-induced apoptosis remains unknown.
In this study, we investigated the following: (1) whether hyperthermia can protect mice against CUS-induced anxiety; (2) whether hippocampal CA3 cell apoptosis correlates with anxiety-like behaviour in mice exposed to CUS; and (3) whether Hsp72 was an important HSP molecule in this event.
Materials and methods
Animals
hsf1-null (hsf1−/−) and wild-type (hsf1+/+) male mice were kindly provided by the University of Texas, Southwestern Medical Center (Dallas, TX). Animals were housed in rooms under standard lighting conditions (12:12 h light-dark cycle) and temperature (22° ± 3°C). Water and food were provided ad libitum. All animal experiments were conducted under an approved protocol from the Central South University and performed in accordance with the animal care guidelines of the Chinese Council.
Fifty-six hsf1−/− and 56 hsf1+/+ mice were randomly divided into four groups: non-stressed group (NS, n = 14), hyperthermia group (HS, n = 14), chronic unpredictable stress group (CUS, n = 14), and hyperthermia plus chronic unpredictable stress (HS + CUS, n = 14). Animal experiments were performed in a designated test room next to the housing room in the animal facility and animals were allowed to acclimate to the environment for 1 h before testing. The HS group was subjected to thermal therapy by placing mice in an incubator Citation[25] for 15 min (39° ± 2°C) on Monday and Thursday. The CUS group was exposed to unpredictable stressors, including restraint stress, elevated open platform, and communication box paradigms. Animals were stressed from Monday to Saturday Citation[26] for 8 weeks. The HS plus CUS group received hyperthermia treatment 2 h after the stress episode. Anxiety-like behaviour was evaluated 24 h after the last stress episode. Behavioural tests were carried out in a fixed order with 30 min interval between tests: elevated plus maze and open field tests. Data was collected by a digital camera linked to a computerised tracking system that recorded all the motion trace and data was then read by two independent “blinded” investigators. After the behaviour tests, mice were deeply anaesthetised and decapitated. For TUNEL staining, brain tissue was harvested, paraffin-embedded, and cut in 5-µm-thick serial coronal sections starting at Bregma – 1.7 mm. For western blot, hippocampus was isolated, frozen in liquid nitrogen, and stored at −80°C.
Chronic unpredictable stress
Chronic unpredictable stress was used as a psychological stress model. This included restraint stress, elevated open platform and communication box paradigms. Mice received one stressor randomly every day except Sunday and each stressor was given twice per week randomly for 8 weeks.
Restraint stress
In restraint stress, the mouse was placed into a semitransparent plastic restraining tube (4 cm in diameter, 6 cm in length) in a quiet room for 1 h/trial Citation[27].
Elevated open platform
The open platform was elevated to a height of 50 cm above floor level. The elevated open platform paradigm was performed by exposing the mouse on an open platform (10 cm × 10 cm) for 1 h/trial Citation[28].
Communication box
The communication box paradigm was used as previously described Citation[11]. The box (64 cm × 64 cm × 40 cm) consisted of one A-compartment surrounded by eight B-compartments in a 3 × 3 array (16 cm × 16 cm each). These compartments are separated by transparent plastic walls. All compartments have stainless steel electric grid floors, but the floors of B-compartments are covered with plastic plates. The animals in the A-compartment received scrambled, uncontrollable and inescapable electric foot shock through the grid floor (0.6 mA, 5 s duration, 60 s inter-shock interval for 1 h), while the animals in the B-compartment were only exposed as witness by seeing and hearing the struggle, jumping, and/or vocalisation of the shocked mouse in A-compartment Citation[11]. The unstressed control mice were placed in the communication box without electric foot shock. This stress was given 1 h/trial.
Measurement of anxiety-like behaviours
Elevated plus maze (EPM)
The test was used to measure anxiety-like behaviours and was conducted as previously described. The elevated plus maze was comprised of two open arms (50 cm × 10 cm) and two closed arms (50 cm × 10 cm) that extended from a common central platform (10 cm × 10 cm) Citation[29]. The apparatus was elevated to a height of 50 cm above floor level. At the time of testing, the animal was gently placed on the central platform facing a closed arm and allowed to freely explore the maze for 5 min. A number of spatio-temporal parameters were collected during the session, including the total number of arm entries (four-paw criterion) and the time spent in the open arms.
Open field test
This test is commonly used to measure anxiety-like behaviour. An open field arena (100 cm × 100 cm × 30 cm) was made of an open rectangular wood box with 25 squares (20 cm × 20 cm) painted on the floor Citation[30]. The 25 squares were divided into 16 side squares and 9 centre squares. An animal was placed in the centre of the field in standard room-lighting conditions and allowed to explore the area freely for 5 min. The open field was cleaned each time after each test.
Protein extraction and Western blots
Western blots were performed as previously described Citation[31]. Briefly, hippocampal tissue was homogenised at 4°C. Protein concentration was determined by Bradford assay (Bio-Rad, Hercules, CA). Equal amounts of proteins (10 µg) were loaded in each lane and separated on SDS-PAGE gel. After proteins were transferred to nitrocellulose membranes, the membranes were blocked with 2% albumin overnight at 4°C, and then incubated with anti-Hsp72 antibody (1:1000, Stressgen, Plymouth Meeting, PA) for 2 h at room temperature. After washing with TBST buffer, the membranes were incubated with horseradish peroxidase-conjugated secondary antibody for 2 h and finally developed with chemiluminescent substrate (Pierce, Rockford, IL). To control for loading efficiency, the blots were stripped and reprobed with β-actin. The images were scanned with Adobe photoshop and quantified with NIH Image J Citation[31]. Expression level of Hsp72 protein was evaluated relative to that of β-actin protein (ie, relative density = protein/ β-actin level). Background values were subtracted from each lane to minimise the variability across membranes.
TUNEL staining of hippocampal CA3 cells
The terminal deoxynucleotidyl transferase-mediated dUTP nick-end labelling (TUNEL) was performed on formalin-fixed and paraffin-embedded sections. Sections were treated with 20 µg/mL proteinase K (Promega, USA) in PBS for 15 min at room temperature and then rinsed in 3% hydrogen peroxide to deactivate endogenous peroxidase. Subsequently, sections were incubated in TUNEL reaction solution for 60 min at room temperature. After washing, streptavidin-HRP was applied to the slices for 30 min at 37°C. Then, slices were stained with DAB and counterstained lightly with hematoxylin. The apoptotic cells in the hippocampal CA3 area were counted under an Olympus light microscope by two investigators who were unaware of the experiment design. The apoptotic cells were identified according to the following criteria: condensed chromatin, smaller, and dark brown nucleus. For each animal, apoptotic nuclei were counted in ten serial hippocampus sections and the total apoptotic cell numbers from ten sections were assessed and plotted.
Statistical analysis
Statistical analysis was performed using SPSS 11.0. Data is expressed as mean ± SEM and analysed using three-way ANOVA. p < 0.05 was considered statistically significant.
Results
Effects of CUS, hyperthermia, and hsf1 gene on anxiety-like behaviours
presents the effect of CUS, hyperthermia, and hsf1 gene on the percentage time that mice spent in open arms (PTOA) and the ratio of time in open versus closed arms (ROVCA) in the EPM test.
Table 1. Behaviour of HSF−/− and HSF1+/+ in the elevated plus maze.
In the analysis of PTOA, three-way ANOVA revealed significant effect of CUS (F(3, 76) = 127.63, p < 0.01) and significant interactions between CUS × gene (F(3, 76) = 19.13, p < 0.01) and CUS × hyperthermia × gene (F(3, 76) = 7.63, p < 0.01). CUS significantly decreased the PTOA in both the hsf1−/− mice (F(3, 76) = 114.53, p < 0.01) and hsf1+/+ mice (F(3, 76) = 28.04, p < 0.01), while hyperthermia significantly increased PTOA in CUS-treated hsf1+/+ mice (F(3, 76) = 7.28, p < 0.01), whereas it had a tendency to further decrease the PTOA in hsf1−/− mice (F(3, 76) = 3.69, p = 0.06).
In the analysis of ROVCA, three-way ANOVA revealed significant effects of CUS (F(3, 76) = 118.35, p < 0.01), gene (F(3, 76) = 8.59, p < 0.01), and hyperthermia (F(3, 76) = 7.98, p < 0.01), significant interaction between CUS × gene (F(3, 76) = 25.96, p < 0.01), and CUS × hyperthermia × gene (F(3, 76) = 9.39, p < 0.01). CUS significantly decreased the ROVCA in hsf1−/− mice with or without treatment of hyperthermia (F(3, 76) = 83.65, p < 0.01; F(3, 76) = 33.00, p < 0.01, respectively). In hsf1+/+ mice, with the presence of hyperthermia, CUS did not decrease the ROVCA (F(3, 76) = 2.53, p > 0.05).
represents the effect of CUS, hyperthermia, and hsf1 gene on the mean number of squares that mice crossed in 5 min in open field test. Three-way ANOVA revealed significant effects of gene (F(3, 76) = 145.34, p < 0.05) and CUS (F(3, 76) = 70.48, p < 0.05) in decreasing the number of squares crossed in 5 min, while significant interaction between hyperthermia × gene (F(3, 76) = 7.52, p < 0.05) were observed. Hyperthermia significantly increased the number of squares crossed in 5 min in the open field test in hsf1+/+ mice exposed to CUS (p < 0.05), but not in hsf1−/− mice (p > 0.05). In contrast, in hsf1−/− mice, hyperthermia further decreased the number of squares crossed (p < 0.05).
Figure 1. Behavioural analysis in open field test. Mean number of squares crossed in 5 min. Significant anxiolytic action of hyperthermia was seen in hsf1+/+ mice, but not in hsf1−/− mice. NS group: non-experimental stress group; HS group: hyperthermia group; CUS group: chronic unpredictable stress group; HS + CUS group: hyperthermia + chronic unpredictable stress group. *p < 0.05, #p < 0.05 versus NS and HS groups; **p < 0.05, ##p < 0.05 versus CUS group; OF: open field, N = 14.
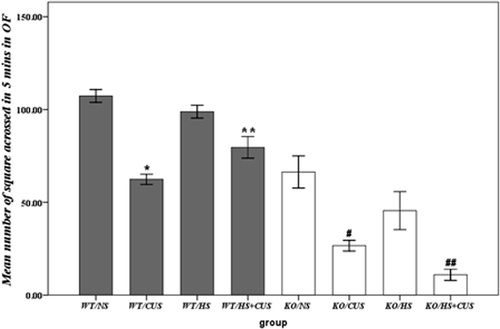
Hyperthermia stimulated Hsp72 expression in hippocampus in hsf1+/+ mice, but not in hsf1−/− mice
Hsp72 level in hippocampal tissues was detected by Western blot assay. As shown in , Hsp72 expression was not detected in hsf1−/− mice underlying all of the treatments. In contrast, in hsf1+/+ mice, Hsp72 expression in control mice (unstimulated) was detectable. Stimulations of CUS, HS, and CUS + HS significantly increased Hsp72 expression compared to the control group (p < 0.05).
Figure 2. Western blot analysis of Hsp72 expression in mice hippocampus. Upper panel: semi-quantitative analysis of Hsp72 expression. In hsf1+/+ mice, mice in HS, and HS + CUS groups were treated with hyperthermia (39° ± 2°C) for 15 min. The Hsp72 expression was significantly higher in HS and HS + CUS groups than NS and CUS groups after 8 weeks (*p < 0.05). In hsf1−/− mice, Hsp72 expression was abolished in all groups. Lower panel: representative Western blot of HSP and β-actin expression. Animals were grouped as described in .
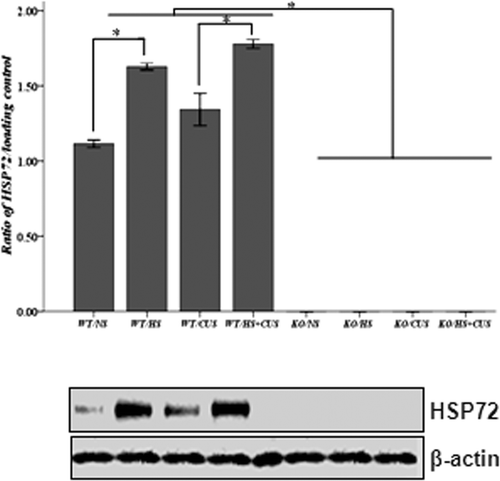
Chronic unpredictable stress induced apoptosis in hippocampal CA3 cells
TUNEL-positive cells displayed obvious chromatin margination or apoptotic bodies ( and ). shows quantitative results of TUNEL staining in wild-type mice (hsf1+/+) and shows quantitative results of TUNEL staining in hif1 knockout mice (hsf1−/−). Apoptotic cells were counted in all groups and the number was used for statistic analysis. Three-way ANOVA showed significant effects of gene (F(3, 76) = 13.54, p < 0.05) and CUS (F(3, 76) = 26.93, p < 0.05), and significant interaction of hyperthermia × gene (F(3, 76) = 4.68, p < 0.05). Hyperthermia significantly decreased the amount of apoptotic hippocampal CA3 cells in CUS-stressed hsf1+/+ mice (F(2, 40) = 5.12, p < 0.05) (), but slightly increased apoptosis in the CUS-stressed hsf1−/− mice (F(2,36) = 1.32, p > 0.05) (). CUS induced more apoptosis in the KO mice compared to the WT mice without statistical significance.
Figure 3. Apoptosis in hippocampal CA3 area in the hsf1+/+ mice. (A) TUNEL staining of hippocampal CA3 area, original magnification ×400. The apoptotic cells exhibited condensed chromatin and smaller, dark brown nucleus. (B) The amount of apoptotic cells in WT mice. In hsf1+/+ mice, chronic unpredictable stress increase apoptosis in the CA3, but hyperthermia decrease chronic unpredictable stress induced apoptosis (n = 8–12). *p < 0.05 versus NS. Animals were grouped as described in .
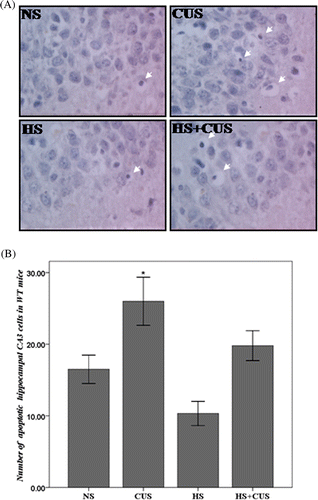
Figure 4. Apoptosis in hippocampal CA3 area in the hsf1−/− mice. (A) TUNEL staining of hippocampal CA3 area, original magnification ×400. The apoptotic cells exhibited condensed chromatin and smaller, dark brown nucleus. (B) The amount of apoptotic cell in hsf1−/− mice. Chronic unpredictable stress increased apoptosis in the CA3 cells, but hyperthermia further increase CUS-induced apoptosis (n = 8–12). *p < 0.01 versus NS. Animals were grouped as described in .
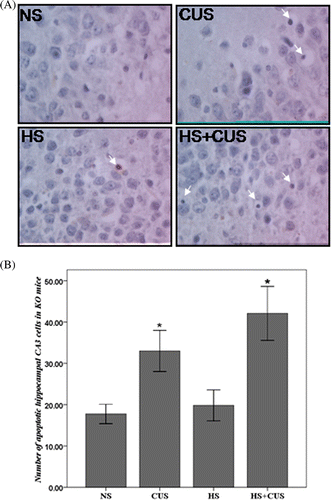
Relationship between cell apoptosis and anxiety-like behaviour
Correlations were analysed in all stressed mice. There were significantly negative correlations between the number of apoptotic hippocampal CA3 cells and PTOA (r = −0.33, p < 0.05) as well as ROVCA (r = −0.28, p < 0.05) in the EPM test (). Negative correlations were also found between the number of apoptotic hippocampal CA3 cells and the mean number of squares crossed in 5 min in the open field test (r = −0.54, p < 0.01) (). These correlations suggested a positive correlation between apoptotic hippocampal CA3 cells and anxiety-like behaviours.
Figure 5. Correlation between apoptotic hippocampal CA3 cells and anxiety-like behaviours. (A) Correlation between the number of apoptotic hippocampal CA3 cells and the percentage of time spent in the open arm in EPM test (r = −0.33, p < 0.05). (B) Correlation between the number of apoptotic hippocampal CA3 cells and the ratio of time in open versus closed arms in EPM test (r = −0.28, p < 0.05). (C) Correlation between the number of apoptotic hippocampal CA3 cells and the mean number of square crossed in 5 min in the open field test (r = −0.54, p < 0.05).
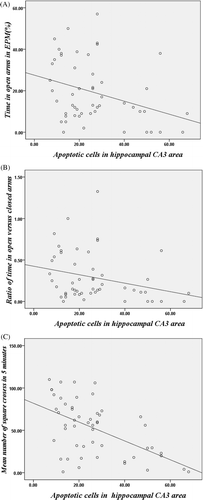
Discussion
In this study, we found that CUS significantly induced anxiety-like behaviours in both the hsf1-null and wild-type mice in the EPM and open field tests. Hyperthermia given during CUS significantly decreased CUS-induced anxiety-like behaviours in wild-type mice, but not in hsf1-null mice. Moreover, anxiety-like behaviours were associated with CUS-induced cell apoptosis in the hippocampal CA3 area, whereas the protective role of hyperthermia in wild-type mice was associated with up-regulation of hippocampal Hsp72 expression and subsequent decrease in apoptosis. However, in hsf1-null mice, hyperthermia increased CUS-induced apoptosis and anxiety-like behaviours due to the lack of Hsp72 synthesis. Therefore, our study provided evidence that: (1) hippocampal CA3 cell apoptosis correlated with anxiety-like behaviours in mice that experienced CUS; (2) Hsp72 is a crucial HSP molecule that protected cells against stresses-induced apoptosis; and (3) hyperthermia given during CUS exposure reduced the extent of anxiety-like behaviours that developed.
The EPM is a programme that is widely used in the assessment of anxiety-like behaviours in rodents. The reluctance in exploring the open arms reflects a fear of open spaces. Therefore, anxiogenic compounds decrease the percentage of time spent in open arms and the ratio of time in open arms versus closed arms Citation[11]. The open field test measures anxiety based on the spontaneous exploration of the environment. Anxiogenic compounds decrease exploratory behaviour in the open field test Citation[11]. In this study, CUS significantly decreased the percentage of open arm time and the ratio of time in open arms versus closed arms in the elevated plus maze test in both hsf1+/+ mice and hsf1−/− mice (). Also, CUS significantly decreased the number of squares crossed in 5 min during the open field test in both hsf1+/+ and hsf1−/− mice (). These results indicate that CUS increased anxiety-like behaviours. Interestingly, hyperthermia inhibited the CUS-induced anxiety-like behaviours in hsf1+/+ mice, whereas it tended to further increase the anxiety-like behaviours in hsf1−/− mice. These observations suggested that hsf1 gene expression is closely related to CUS-induced anxiety-like behaviours and the inhibitory effect of hyperthermia.
The hsf1 gene produces stress-induced heat shock factor 1, the main HSF. The synthesis of heat shock protein is rapidly increased in response to stress. This process is regulated by HSFs which bind to the hsp promoter element and enhance HSP synthesis Citation[32]. In this study we demonstrated that both CUS and hyperthermia did not stimulate Hsp72 expression in hsf1−/− mice, but induced Hsp72 synthesis in hsf1+/+ mice (). This suggested that Hsp72 was mainly regulated by HSF1. Recently, Hsp72 has been shown to be an important factor in mood disorders. For instance, antidepressant drugs and mood stabilisers increase Hsp72 expression Citation[23], while major depression patients detected with Hsp72 gene variants showed lower expression of Hsp72 in response to antidepressants and poor anti-depression treatment outcome Citation[24]. Therefore, we propose that hippocampal Hsp72 is an important inhibitory factor in the development of CUS-induced anxiety-like behaviours.
There is growing evidence that anxiety disorders are associated with impairment of cellular plasticity in the hippocampus Citation[33] and cellular apoptosis might be a key factor involved in the pathophysiology of anxiety disorders Citation[34]. For example, mice with mutation of the anti-apoptotic Bcl-2 gene showed more anxiety-like behaviours than wild-type mice Citation[18]. Tianeptine, an antidepressant, could prevent or reverse stress-associated structural and cellular changes in the hippocampus through preventing stress-induced dendritic atrophy and reducing stress-induced apoptosis, thereby conferring significant anxiolytic effects Citation[35]. Nicotinamide was demonstrated to prevent the long-term effects of perinatal asphyxia on both apoptosis and anxiety in rats Citation[36]. In our present study, chronic unpredictable stress induced a significant increase in anxiety-like behaviours and apoptotic cells in both hsf1+/+ mice and hsf1−/− mice, whereas hsf1 gene exhibited a significant protective effect on the hippocampal apoptosis during hyperthermia. A significantly positive correlation between apoptotic hippocampal CA3 cells and anxiety-like behaviours was observed, indicating that CA3 cell apoptosis plays a crucial role in CUS-induced anxiety-like behaviours. Furthermore, we demonstrated that heat shock reaction is effective in preventing CUS-induced anxiety-like behaviour through protection of hippocampal CA3 cells against apoptosis.
Although the role of hyperthermia in protecting CUS-induced apoptosis and anxiety was shown in this study, its mechanism is far from being fully elucidated. For example, CUS induces Hsp72 expression in hsf1+/+ mice, while the Hsp72 level is undetectable in hsf1−/− mice underlying CUS. However, CUS induced similar levels of anxiety-like behaviours in the EPM test in both the hsf1−/− and hsf1+/+ mice. In contrast, CUS induced more apoptosis in hsf1−/− mice compared to hsf1+/+ mice. This may suggest that: (1) apoptosis in hippocampus only plays a partial role in the development of anxiety-like behaviours underlying CUS; (2) hippocampal Hsp72 is not the only factor involved in the protective role of hyperthermia and other HSPs might also be involved; (3) the protective role of hyperthermia might be mediated by multiple factors, including the up-regulation of Hsp72 and decrease in apoptosis; (4) other brain regions may also be involved in CUS-induced development of anxiety-like behaviour, such as prefrontal cortex and amygdala Citation[37]. Therefore, the accurate mechanism that mediates CUS-induced development of anxiety-like behaviours requires further study.
Stressful life events are unavoidable. Besides psychological stress induced by trauma such as bereavement, war, disaster and other major events, many other factors in daily life can also induce psychological stress, such as the unsafe living or working environment, work stress, etc. Generally, prolonged stressful life events have profound effects on mental health. For example, prolonged periods of work or study can induce stress. For those with children, children with chronic health and learning problems cause prolonged stress in the parents. About 4%–6% of the global population suffer from various forms of anxiety disorders Citation[38]. Pharmacological and psychological treatments are still the conventional treatments for anxiety disorders Citation[39]. However, pharmacological treatment is associated with a number of side-effects Citation[38]. The current study provides experimental evidence for the possible application of hyperthermia in treating chronic stress-induced anxiety. For example, sauna bathing features a typical thermal therapy and exhibited clear efficacy in reducing chronic pain Citation[40], treating appetite loss and mild depression Citation[41]. A study by Hayasaka et al. demonstrated that sauna bathing could improve mood and decrease anxiety Citation[42]. Thus, using sauna to treat chronic stress-induced anxiety might be a reasonable therapeutic modality for anxiety underlying chronic stress.
Conclusion
Hippocampal CA3 cell apoptosis closely correlated with chronic unpredictable stress-induced anxiety-like behaviours. Hyperthermia could inhibit the development of anxiety underlying chronic psychological stresses. The protective role of hyperthermia might be associated with up-regulation of Hsp72 expression in hippocampus. However, our study also suggested that other brain areas or other signal molecules might also be involved in chronic psychological stress-induced behaviour disorders.
Acknowledgements
The authors would like to thank Ivor Benjamin (University of Texas Southwestern Medical Center, USA) for providing hsf1-null mice and Guangwen Chen (Institute of Pathophysiology, Central South University, China) for his valuable advice on experimental design. The authors would like to thank Isabel Lauren Jackson (Department of Pathology, Duke University) for editing the language.
Declaration of interest: This work was supported by the Natural Science Foundation of China (No. 81071104 to S. Yao and 81071097 to X. Zhu). The authors declare no conflict of interest. The authors alone are responsible for the content and writing of the paper.
References
- Kühl K, Schürmann W, Rief W. Mental disorders and quality of life in COPD patients and their spouses. Int J Chron Obstruct Pulmon Dis 2008; 3: 727–736
- Esch T, Stefano GB, Fricchione GL, Benson H. The role of stress in neurodegenerative diseases and mental disorders. Neuro Endocrinol Lett 2002; 23: 199–208
- Hammen C, Davila J, Brown G, Ellicott A, Gitlin M. Psychiatric history and stress: Predictors of severity of unipolar depression. J Abnorm Psychol 1992; 101: 45–52
- Gilmer WS, McKinney WT. Early experience and depressive disorders: Human and non-human primate studies. J Affect Disord 2003; 75: 97–113
- Nemeroff CB. Neurobiological consequences of childhood trauma. J Clin Psychiatry 2004; 65 S: 18–28
- Liston C, McEwen BS, Casey BJ. Psychosocial stress reversibly disrupts prefrontal processing and attentional control. Proc Natl Acad Sci USA 2009; 106: 912–917
- Bondi CO, Rodriguez G, Gould GG, Frazer A, Morilak DA. Chronic unpredictable stress induces a cognitive deficit and anxiety-like behavior in rats that is prevented by chronic antidepressant drug treatment. Neuropsychopharmacology 2008; 33: 320–331
- Conrad CD. What is the functional significance of chronic stress-induced CA3 dendritic retraction within the hippocampus?. Behav Cogn Neurosci Rev 2006; 5: 41–60
- Lucassen PJ, Heine VM, Muller MB, van der Beek EM, Wiegant VM, De Kloet ER, Joels M, Fuchs E, Swaab DF, Czeh B. Stress, depression and hippocampal apoptosis. CNS Neurol Disord Drug Targets 2006; 5: 531–546
- Pechlivanova D, Tchekalarova J, Nikolov R, Yakimova K. Dose-dependent effects of caffeine on behavior and thermoregulation in a chronic unpredictable stress model of depression in rats. Behav Brain Res 2010; 209: 205–211
- Zhu X, Cheng M, Peng M, Xiao X, Yao S, Zhang X. Basal behavioral characterization of hsf1 deficient mice and its cellular and behavioral abnormalities underlying chronic unpredictable stressors. Behav Brain Res 2008; 193: 225–229
- Matuszewich L, Karney JJ, Carter SR, Janasik PJ, O'Brien JL, Friedman RD. The delayed effects of chronic unpredictable stress on anxiety measures. Physiol Behav 2007; 90: 674–681
- Zurita A, Marinelli M, Cuadra G, Brandao ML, Molina VA. Early exposure to chronic variate stress facilitates the occurrence of anhedonia and enhanced emotional reactions to novel stressors: Reversal by naltrexone pretreatment. Behav Brain Res 2000; 117: 163–171
- Buwalda B, Kole MHP, Veenema AH, Huininga M, de Boer SF, Korte SM, Koolhass JM. Long term effects of social stress on brain and behavior: A focus on hippocampal functioning. Neurosci Biobehav Rev 2005; 29: 83–97
- Zarate CA, Jr, Du J, Quiroz J, Gray NA, Denicoff KD, Singh J, Charney DS, Manji HK. Regulation of cellular plasticity cascades in the pathophysiology and treatment of mood disorders: Role of the glutamatergic system. Ann NY Acad Sci 2003; 1003: 273–291
- Bogoch Y, Biala YN, Linial M, Weinstock M. Anxiety induced by prenatal stress is associated with suppression of hippocampal genes involved in synaptic function. J Neurochem 2007; 101: 1018–1030
- Lucassen PJ, Vollmann-Honsdorf GK, Gleisberg M, Czéh B, De Kloet ER, Fuchs E. Chronic psychosocial stress differentially affects apoptosis in hippocampal subregions and cortex of the adult tree shrew. Eur J Neurosci 2001; 14: 161–166
- Einat H, Yuan P, Manji HK. Increased anxiety-like behaviors and mitochondrial dysfunction in mice with targeted mutation of the Bcl-2 gene: Further support for the involvement of mitochondrial function in anxiety disorders. Behav Brain Res 2005; 165: 172–180
- Sato K, Saito H, Matsuki N. HSP70 is essential to the neuroprotective effect of heat-shock. Brain Res 1996; 740: 117–123
- Gursoy E, Cardounel A, Kalimi M. Heat shock preconditioning and pretreatment with glucocorticoid antagonist RU 486 protect rat myogenic cells H9c2 against glutamate-induced cell death. Mol Cell Biochem 2001; 220: 25–30
- Rossler OG, Bauer I, Chung HY, Thiel G. Glutamate-induced cell death of immortalized murine hippocampal neurons: Neuroprotective activity of heme oxygenase-1, heat shock protein 70, and sodium selenite. Neurosci Lett 2004; 362: 253–257
- Plumier JC, David JC, Robertson HA, Currie RW. Cortical application of potassium chloride induces the low-molecular weight heat shock protein (Hsp27) in astrocytes. J Cereb Blood Flow Metab 1997; 17: 781–790
- Chuang DM. The antiapoptotic actions of mood stabilizers: Molecular mechanisms and therapeutic potentials. Ann NY Acad Sci 2005; 1053: 195–204
- Pae CU, Mandelli L, Serretti A, Patkar AA, Kim JJ, Lee CU, Lee SJ, Lee C, De Ronchi D, Paik IH. Heat-shock protein-70 genes and response to antidepressants in major depression. Prog Neuropsychopharmacol Biol Psychiatry 2007; 31: 1006–1011
- Pritchard MT, Ostberg JR, Evans SS, Burd R, Kraybill W, Bull JM, Repasky EA. Protocols for simulating the thermal component of fever: Preclinical and clinical experience. Methods 2004; 32: 54–62
- Armario A, Garcia-Marquez C, Jolin T. The effects of chronic intermittent stress on basal and acute stress levels of TSH and GH, and their response to hypothalamic regulatory factors in the rat. Psychoneuroendocrinology 1987; 12: 399–406
- Kitraki E, Kremmyda Q, Youlatos D, Alexis M, Kittas C. Spatial performance and corticosteroid receptor status in the 21-day restraint stress paradigm. Ann NY Acad Sci 2004; 1018: 323–327
- Miyata S, Shimoi T, Hirano S, Yamada N, Hata Y, Yoshikawa N, Ohsawa M, Kamei J. Effects of serotonergic anxiolytics on the freezing behavior in the elevated open-platform test in mice. J Pharmacol Sci 2007; 105: 272–278
- Pellow S, Chopin P, File SE, Briley M. Validation of open:closed arm entries in an elevated plus-maze as a measure of anxiety in the rat. J Neurosci Methods 1985; 14: 149–167
- Schmidt MV, Sterlemann V, Ganea K, Liebl C, Alam S, Harbich D, Greetfeld M, Uhr M, Holsboer F, Muller MB. Persistent neuroendocrine and behavioral effects of a novel, etiologically relevant mouse paradigm for chronic social stress during adolescence. Psychoneuroendocrinology 2007; 32: 417–429
- Zhang X, Lee TH, Davidson C, Lazarus C, Wetsel WC, Ellinwood EH. Reversal of cocaine-induced behavioral sensitization and associated phosphorylation of the NR2B and GluR1 subunits of the NMDA and AMPA receptors. Neuropsychopharmacology 2007; 32: 377–387
- Calderwood SK. Heat shock proteins in breast cancer progression – A suitable case for treatment?. Int J Hyperthermia 2010; 26: 681–685
- Duman CH, Duman RS. Neurobiology and treatment of anxiety: Signal transduction and neural plasticity. Handb Exp Pharmacol 2005; 169: 305–334
- Ren-Patterson RF, Cochran LW, Holmes A, Sherrill S, Huang SJ, Tolliver T, Lesch KP, Lu B, Murphy DL. Loss of brain-derived neurotrophic factor gene allele exacerbates brain monoamine deficiencies and increases stress abnormalities of serotonin transporter knockout mice. J Neurosci Res 2005; 79: 756–771
- Kasper S, McEwen BS. Neurobiological and clinical effects of the antidepressant tianeptine. CNS Drugs 2008; 22: 15–26
- Morales P, Simola N, Bustamante D, Lisboa F, Fiedler J, Gebicke-Haerter PJ, Morelli M, Tasker RA, Herrera-Marschitz M. Nicotinamide prevents the long-term effects of perinatal asphyxia on apoptosis, non-spatial working memory and anxiety in rats. Exp Brain Res 2010; 202: 1–14
- Zoladz PR, Muñoz C, Diamond DM. Beneficial effects of tianeptine on hippocampus-dependent long-term memory and stress-induced alterations of brain structure and function. Pharmaceuticals 2010; 3: 3143–3166
- Lee YL, Wu Y, Tsang HW, Leung AY, Cheung WM. A systematic review on the anxiolytic effects of aromatherapy in people with anxiety symptoms. J Altern Complement Med 2011; 17: 101–108
- Black DW. Efficacy of combined pharmacotherapy and psychotherapy versus monotherapy in the treatment of anxiety disorders. CNS Spectr 2006; 11: 29–33
- Masuda A, Koga Y, Hattanmaru M, Minagoe S, Tei C. The effects of repeated thermal therapy for patients with chronic pain. Psychother Psychosom 2005; 74: 288–294
- Masuda A, Nakazato M, Kihara T, Minagoe S, Tei C. Repeated thermal therapy diminishes appetite loss and subjective complaints in mildly depressed patients. Psychosom Med 2005; 67: 643–647
- Hayasaka S, Nakamura Y, Kajii E, Ide M, Shibata Y, Noda T, Murata C, Nagata K, Ojima T. Effects of charcoal kiln saunas (Jjimjilbang) on psychological states. Complement Ther Clin Pract 2008; 14: 143–148