Abstract
Purpose: To investigate simultaneous and sequential injection thermochemical ablation in a porcine model, and compare them to sham and acid-only ablation.
Materials and methods: This IACUC-approved study involved 11 pigs in an acute setting. Ultrasound was used to guide placement of a thermocouple probe and coaxial device designed for thermochemical ablation. Solutions of 10 M acetic acid and NaOH were used in the study. Four injections per pig were performed in identical order at a total rate of 4 mL/min: saline sham, simultaneous, sequential, and acid only. Volume and sphericity of zones of coagulation were measured. Fixed specimens were examined by H&E stain.
Results: Average coagulation volumes were 11.2 mL (simultaneous), 19.0 mL (sequential) and 4.4 mL (acid). The highest temperature, 81.3°C, was obtained with simultaneous injection. Average temperatures were 61.1°C (simultaneous), 47.7°C (sequential) and 39.5°C (acid only). Sphericity coefficients (0.83–0.89) had no statistically significant difference among conditions.
Conclusions: Thermochemical ablation produced substantial volumes of coagulated tissues relative to the amounts of reagents injected, considerably greater than acid alone in either technique employed. The largest volumes were obtained with sequential injection, yet this came at a price in one case of cardiac arrest. Simultaneous injection yielded the highest recorded temperatures and may be tolerated as well as or better than acid injection alone. Although this pilot study did not show a clear advantage for either sequential or simultaneous methods, the results indicate that thermochemical ablation is attractive for further investigation with regard to both safety and efficacy.
Introduction
Treatment of solid tumours such as hepatocellular carcinoma (HCC) that respond poorly to intravenous (i.v.) chemotherapy or newer targeted agents is an ongoing challenge Citation[1–3]. Surgery is often held to be the gold standard, but the majority of patients are disqualified due to tumour location, degree of underlying liver disease, multiple or infiltrative tumours, or comorbidities. In response, alternative therapies have been developed for ablation or embolisation. While interest in these methods continues to grow, chemical ablation would seem to have been relegated to historical interest. Nevertheless, it does offer benefits worthy of further consideration and study Citation[4–6].
The two chemical ablation agents receiving the most attention in the literature are ethanol Citation[7] and 50% acetic acid Citation[8–10]. Delivery is usually via instillation through a multiple side-hole needle under ultrasound or computerised tomography (CT) guidance. Systemic toxicity Citation[11–14] limits the amount that can be safely delivered. This results in smaller doses and multiple treatment sessions and is one of the major criticisms of this technique. The other major criticism is that hydrostatic pressure from intraparenchymal injection forces the agent to follow the path of least resistance. This can cause unpredictable distribution of the agent and may result in inadequate treatment, damage to non-target tissues Citation[15], and/or systemic exposure. With the advent of multi-tined needle devices and premedication protocols Citation[16–18], these criticisms can be addressed to some extent. This approach, however, does not appear to have been widely adopted.
An alternative to conventional chemical ablation would be to address the dose-limiting toxicity problem through a strategy designed to create local conditions that preserve local toxicity but decrease the systemic toxicity. If hyperthermia and hyperosmolarity were intrinsic components of a chemical method, thermal conductive effects and concentration effects in tissue might lead to a more predictable zone of coagulation. If successful, such an approach could potentially allow safe, predictable treatment of large tumour volumes in a single session, thereby addressing both of the major criticisms of chemical ablation.
Thermochemical ablation is a concept in which exothermic reactions are used as a heat source. One such reaction is neutralisation of an acid by a base, which could be done with either simultaneous or sequential administration of the reagents. The purpose of this study is to investigate these two injection methods in a porcine model, and compare them to sham as a negative control, and acid-only ablation as a positive control. The porcine model was chosen to allow for adequate size for prototype device placement and monitoring. No porcine tumour models are readily available Citation[19] but there is an extensive body of ablation literature in pigs. Thus healthy swine were utilised for these experiments. Temperatures, coagulation volumes, sphericities, and histopathology were analysed. Our hypothesis is that combined injection would improve on acid ablation by increasing coagulum volume and be as well or better tolerated than acid alone.
Materials and methods
Animal handling
Animals were treated in accordance with protocols reviewed and approved by the institutional animal care and use committee and cared for as recommended in the Guide for the Care and Use of Laboratory Animals Citation[20]. Eleven swine 60–75 kg were acclimated as per institutional guidelines. After induction of anaesthesia (tiletamine/zolazepam/xylazine 0.4 mL/kg intramuscularly (i.m.) then 2–5% isoflurane) and surgical exposure intraoperative ultrasound allowed device placement into the liver at least 10 mm away from major vessels (10 MHz z.one ultra system, ZONARE, Mountain View, CA). Euthanasia was accomplished with pentobarbital/phenytoin 0.2 mg/kg i.v. Blood pressure, heart rate, and oxygenation were recorded at 30-s intervals.
Reagents and device preparation
Acetic acid and sodium hydroxide (Sigma-Aldrich, St Louis, MO, USA) were diluted to 10 mol/L. Iodinated contrast (Iodixanol, GE Healthcare) was used at 5% v/v in acid for all injections and saline sham to aid in visualisation at CT. A 17G device was assembled as reported by Farnam et al. Citation[21] with slight modifications: the trocar tip was cut free then soldered to the outer cannula and side holes were created near the tip of the cannula.
Temperature measurements
A 23G thermocouple (Physitemp type T MT-23/3, Clifton, NJ) was inserted parallel at 5 mm to the same depth. Temperature data were monitored continuously and recorded every 5 s with a T-type thermocouple (Digi-Sense, Cole-Palmer, Vernon Hills, IL).
Ablation (injection) experiments
Reagent concentrations were 10 mol/L, prewarmed to 40°C. Four different injections were carried out in each liver in the same sequence for all 11 animals: sham, simultaneous, sequential, and acid-only for a total of 44 injections. The device was assembled, connected to the syringes by extension tubing, and primed prior to insertion into the liver. Separate lobes were used for each injection to avoid cross-contamination issues. Injections were performed in the right medial (acid and sham), left medial (simultaneous), and left lateral (sequential) lobes. Location assignments were kept constant. Due to challenges with surgical exposure the right lateral lobe was not used. A syringe pump (Standard Infusion Only Harvard Pump 11 Plus Dual Syringe Pump, Harvard Apparatus, Holliston, MA) delivered a combined 4 mL/min from two syringes with 2 mL volumes of reagent in each. In the saline sham and acid-only injections where a single syringe was used, the rate was also 4 mL/min. Volume in these cases was kept constant at 2 mL. Acid was injected first in all sequential injections. Haemostasis at the conclusion of each injection was obtained by inserting a piece of spaghetti into the tract Citation[22]. Temperature was recorded for 5–10 min after each injection. Livers were fixed in 10% neutral buffered formalin for sectioning.
Gross pathology
Individual lobes were sectioned perpendicular to the axis of the probe insertion tract. Gross images of 2-mm thick specimens were obtained and analysed in ImageJ (National Institutes of Health, Bethesda, MD). Two independent, unblinded observers (M.G. and M.S.) obtained tracings of the coagulated regions to allow measurement of the perimeter and area of each slice. Gross areas were determined by the colour change in the specimens compared to untreated adjacent tissue. Outer margins were used for measurement, but demarcations were generally very narrow (<2 mm) and clearly demarcated between affected and unaffected tissues. Area and perimeter were then used with slice thickness to calculate slice volumes, total volume, 3D surface area, and sphericity for each treatment. Data are reported in accordance with Society of Interventional Radiology Reporting Standards Citation[23], Citation[24].
Sphericity calculations
The sphericity corresponds to mathematical convention in which the surface area/volume ratio of a sphere is represented by a value of 1. Sphericity was calculated using the following formula:where Vp = Volume and Ap = surface area. Values less than 1 correlate with the degree of deviation away from an ideal sphere.
Histology
After gross pathology analysis, the formalin-fixed specimens 2 mm thick were processed and embedded into paraffin, sectioned at 5 µm and stained with haematoxylin and eosin (H&E). Approximately 1.5 cm × 1 cm sections were taken from central and margin zones of each sample.
Imaging
CT imaging of explanted livers to interrogate the relationship of contrast distribution from the injections and the zones of coagulation was performed on a Siemens Sensation 64 scanner (Erlangen, Germany) with the following parameters: mAs 400, 120 kVp, 1 mm slice width, 0.6 mm collimation, pitch 0.7, and rotation time of 0.5 s. Multiplanar reconstructions were created and archived for further review (unblinded).
Statistical analysis
Outcomes including ablation volume, sphericity, and temperature, (except sham as no detectable changes were noted) were measured. General linear models for random block design were used to determine the effects of injection types (simultaneous, sequential, and acid alone) on ablation volume and sphericity, temperature, and change in heart rate. The variance-covariance structure was compound symmetry. P values were adjusted by Tukey's method. Inter-observer agreement on ablation volume and sphericity by two observers was evaluated using intraclass correlation coefficients (ICC) from general linear models for split-plot design, with lesions from the same animal as the whole-plot experiment units and observer as the split-plot factor. All analyses were conducted in SAS 9.2 (SAS Institute, Cary, NC). A p value of less than 0.05 indicated significance.
Results
Temperatures in vivo
Average peak temperatures for each injection method are shown in . There were significant differences in mean temperature among the three treatment groups (p < 0.0001). No temperature change was detected in the sham injections. The sequential injection mean temperature was intermediate (47.7°C), and simultaneous injection produced the highest mean temperature (61.1°C). Multiple pairwise comparisons were all significant: acid versus sequential (adjusted p = 0.0397), acid versus simultaneous (adjusted p < 0.0001), and sequential versus simultaneous (adjusted p = 0.0009). Maximum peak temperature was also considerably lower in the sequential versus simultaneous injection (57°C versus 81°C). It is noteworthy that the highest temperature measured in sequential injection was lower than the average temperature obtained with simultaneous injection. In addition, heart rate changes were minimal in controls and simultaneous instillation but one pig arrested after sequential injection and had to be revived with epinephrine and a fluid bolus.
Figure 1 Bar graph depiction of peak temperatures measured at thermocouple for (L to R) saline sham, simultaneous injection, sequential injection, and acid-only injection. All solutions had been warmed to 39–40°C (pig body temperature) prior to placing into a syringe pump with extension tubing to the device. SIM, simultaneous injection; SEQ, sequential injection.
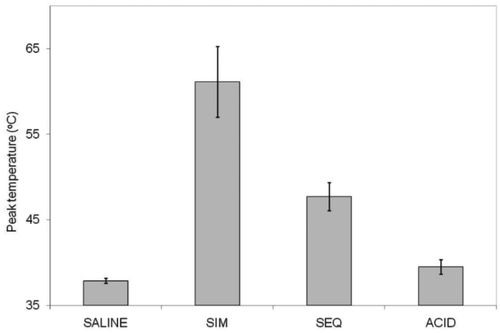
Histopathology and imaging
Ablated regions exhibited coagulative to lytic necrosis (). Cells in these regions were shrunken with nuclear pyknosis to lysis and loss of cytoplasmic and membrane definition. The demarcation zone between ablated and unablated tissue was most clearly delineated for sequential and simultaneous injection, largely due to congestion of sinusoids and haemorrhage at the margins of the lesion.
Figure 2 Histology: Gross and corresponding histologic sections after acid (A, B, C), simultaneous (D, E, F) or sequential (G, H, I) injection. Cells in ablated regions exhibit coagulative necrosis and are shrunken with pale cytoplasm and indistinct nuclei compared to unablated tissue (inset, C). Haemorrhage is obvious in simultaneous and sequential sections (E, F). Imaging marker (spaghetti) is visible in histological sections (B, C, stars). A, D, G: Bar equals 1 cm. B, E, H: H&E, 40× magnification, bar equals 500 µm. C, F, I: H&E, 400× magnification, bar equals 50 µm.
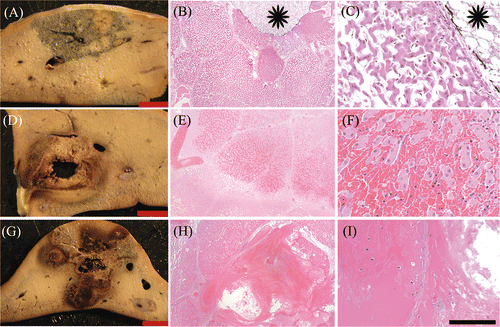
Haemorrhage was visible in gross sections as red to black areas (, ). Mild haemorrhage was rarely noted in sections treated with acid only. Both sequential and simultaneous treatments exhibited increased haemorrhage compared to acid only (, , ). In both of these treatment groups, necrosis of large vessels occurred, and vessel damage, as indicated by margination of neutrophils and fibrin deposition to frank vascular necrosis, was evident in some vessels outside the central ablation zone ().
Figure 3 Vascular damage adjacent to ablated region. Fibrin and neutrophils (arrow) adhere to vascular endothelium in vessel adjacent to viable hepatocytes. H&E, 200× magnification. Bar equals 50 µm.
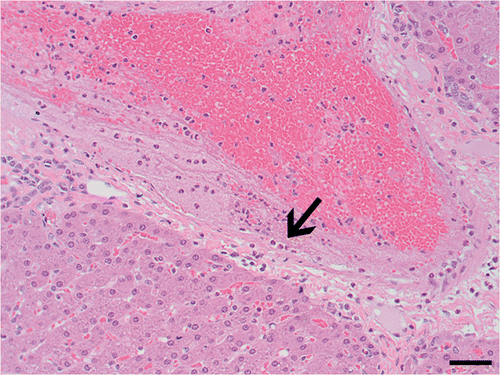
Correlation of pathology with CT imaging was uneven. The most consistent results were observed with acid-only injection (, first series).
Figure 4 Gross pathology correlations with adjacent CT rendering corresponding in each case. Acetic acid alone (A, B), simultaneous injection (C, D), sequential injection (E, F). Note that although acid alone correlates fairly well, with either of the two other methods there is inconsistent density and/or shape compared to CT.
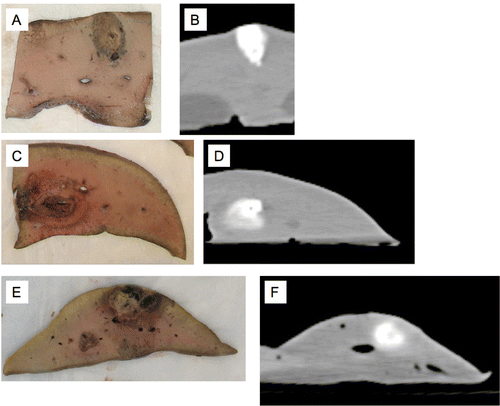
Volumes and lesion shapes
Volumes of the coagulated tissues for each method of injection are shown as bar graphs in . There were significant differences in mean ablation volumes for both observers among the three injection methods (p = 0.0006 for observer 1, p = 0.0002 for observer 2). Acid alone had the lowest least squares mean ablation volume (4.3 mL by observer 1 and 4.5 mL by observer 2). Simultaneous injection was intermediate (11.9 mL by observer 1 and 9.8 mL by observer 2), and sequential injection produced the highest least squares mean ablation volume (18.9 mL by observer 1 and 19.3 mL by observer 2).
Figure 5 Bar graph illustrating average summation volumes of zones of coagulation for simultaneous injection (SIM), sequential injection (SEQ), and acid alone. Saline sham is not listed as no detectable lesion was identified in that case.
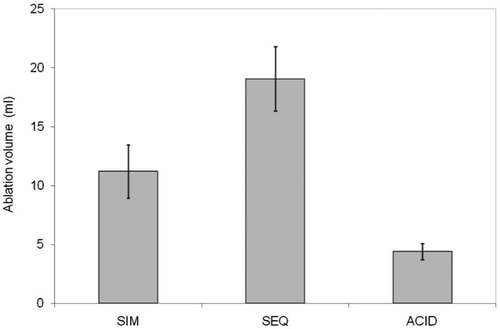
Sphericity for each injection type is plotted in . The least squares means of sphericity in acid injection, simultaneous and sequential methods were 0.87, 0.89 and 0.84, respectively for observer 1 and 0.80, 0.82 and 0.87 for observer 2. There were no significant differences in mean sphericity by either observer among three injection methods (p = 0.3920 for observer 1, p = 0.1223 for observer 2).
Figure 6 Bar graph depiction of sphericity coefficients for simultaneous (SIM), sequential (SEQ), and acid-only injection. Saline sham is not listed as no detectable lesion was identified in that case.
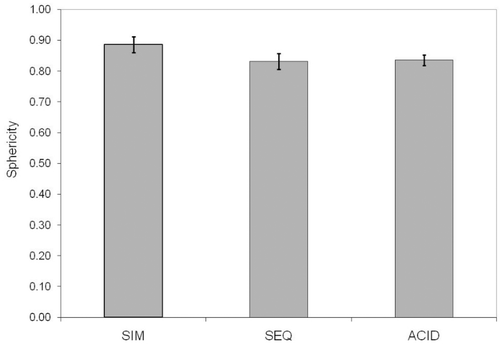
The ICC for volume was 0.8597 and that for sphericity was 0.7562, indicating strong agreement between the two observers for both outcomes.
Discussion
Recently, exothermic acid-base neutralisation chemistry has been shown in phantom Citation[25], Citation[26] and ex vivo Citation[21], Citation[27] studies to have potential for tissue ablation based on the amount of energy released as water is formed. Oxidation-reduction chemistry has also been studied and can be more energetic but may have greater limitations regarding toxicity Citation[27]. With respect to acids and bases, salts are formed as a result of neutralisation and in tissues can be present at concentrations many times higher than the physiological range. With simultaneous injection the acid and base would be consumed in the formation of the salts. Therefore the deviation from neutral pH should be minimal if reaction occurred to completion. Under these circumstances, exposure to either an acid or base load that would alter the systemic pH should be effectively eliminated. These salts may also have local hyperosmolar effects leading to cell destruction as described in more detail below. Sequential injection on the other hand would still allow for some degree of mixing directly in the tissues but by its nature would entail direct injection of unreacted, highly concentrated substances that could lead to systemic exposure and its consequences.
Reagent selection and conditions
Reagent choice for acid was based on existing clinical experience with acetic acid. Most clinical use of acetic acid is with 50% concentration which is approximately 8.5 molar. The use of 10 molar acid was based on anticipation of future studies using various concentrations and a desire to maintain a consistent body of data. Use of NaOH was based on the requirements for a strong base that would produce a salt, sodium acetate (NaOAc) composed of ions already present in the body. Furthermore, acetate is quickly converted to bicarbonate and thus whatever portion reached the systemic circulation should be readily metabolised Citation[29]. Both the volume and the concentration of acid were kept constant in all injections because our purpose was to compare the other methods against it. Order of injections was likewise kept constant to minimise the number of variables.
Although the acid alone seemed to correlate fairly well with contrast distribution Citation[30], the results from CT correlated poorly with the gross pathology as shown in . We speculate that the solutions behave much as they would in column chromatography. That is to say that the different components would interact with and partition differently into the medium and thus migrate relative to the solvent front at different rates.
Temperatures in vivo
Volume data suggest that temperature may not be the dominant factor in determining the outcome of the ablation. A modest exotherm was observed with sequential injection but peak temperatures were recorded with simultaneous injection. One possible reason is that less mixing occurred with sequential injection.
Histopathology
Regions identified on gross examination as ablated exhibited complete necrosis, although we cannot rule out the presence of small foci of viable tissue within ablated regions in this small, acute study. Haemorrhage was most common after sequential and simultaneous treatment, suggesting a greater degree of vascular damage and possible intravascular administration or leakage of reagents or products (i.e. hyperosmolar sodium acetate).
Volume and shape relationships
Agreement between observers on volume measurements was reasonably strong. Comparison of sphericity revealed no statistically significant differences among techniques or observers. Conventional practice for acid ablation is to use an amount of acid that is one third of the volume of the desired treatment volume. Therefore, given 2 mL of acid used, the predicted final coagulation volume would be in the range of 6 mL (i.e. a factor of 3). In this model however, we observed a slightly lower value, just over twice the injected volume for coagulation. This could be due to the acute setting and in normal liver.
Comparison of the volumes from the different methods results in some interesting observations. There is a nearly 2.5-fold increase in coagulation volume over that seen with acid alone with simultaneous injection that was well tolerated. Sequential injection showed even larger volumes but this came at a price of less control and higher risk. In fact, one animal required resuscitation after cardiovascular collapse (tachycardia/hypotension progressing to asystole) following a sequential injection, a situation not encountered with simultaneous injection. We believe this is due to the neutralisation that occurred with simultaneous injection that may not have occurred with the sequential method but direct proof is lacking. The total injection time was only 1 min and the amount of tissue ablated per min would appear, at least initially, to be competitive with radio frequency (RF) energy-based devices.
The sphericity coefficient ranged from 0.80–0.89, which was reasonably high.
The injection rate in this model (4 mL/min) was an order of magnitude faster than recommended with ethanol by Tapani et al. Citation[31] for maintaining some control over shape, and the injection volumes were considerably larger. Despite these differences, sphericity remained fairly high and within a narrow range. We propose that this may be due to three factors: the effect of the thermal component, differences in viscosity compared to ethanol, and the in vivo nature of the experiment.
Mechanism of action
How thermochemical ablation (i.e. the simultaneous injection method) coagulates tissue is not yet known, but at least two mechanisms, heat and hyperosmolarity, seem likely. We hypothesise that heat and hyperosmolarity act together additively or possibly synergistically, recognising that the duration of temperature excursion is actually quite brief in comparison to conventional methods. Since the exotherm is so brief but the salt exposure is much longer in duration, the relative contributions of heat and hyperosmolarity must be studied in more detail to understand and assign their relative importance using this method. Presuming that the reaction occurred to completion in the simultaneous injection experiments, the salt was produced at a concentration of 5 mol/L. Due to the binary nature of the salt (two particles in solution) this was effectively 10 osmolar (Osm/L). Most physiological settings are in the range of 270–300 mOsm. This means at the device tip the salt concentration is 1 to 2 orders of magnitude higher than physiological conditions. Furthermore the change in osmotic pressure occurred abruptly. Centrally where the temperature and osmotic pressure would already be extremely high this should not be an issue. However, at the margins, this still allowed essentially no time for cellular adaptation. The lytic necrosis observed histologically supports this idea.
The study had several important limitations. First, only one thermocouple was used. Multiple thermocouples could be incorporated or magnetic resonance thermography (MRT) could be considered to address this and evaluate the volume and time distribution of the temperature excursion. No direct comparison was made to a thermal therapy such as RF ablation. Lack of either tumour or cirrhosis as noted earlier, and the acute nature of the experiments could affect the results with regard to distribution of the reagents in tissues and long-term effects. Also, the injections were done in the same sequence in the same lobe each time. Although thought to be unlikely, this is a potential source of variation in the results and was based on preliminary, unpublished prior experiments. It will be of interest to further explore the relationship of volume injected and concentration to the coagulum volume. More detailed temperature studies such as MRT would further define the effects in real time, and subacute studies would be useful for understanding the evolution of lesions. As the area is developed, transition to a tumour model will allow more rigorous evaluation of the method.
Conclusion
Thermochemical ablation produced substantial volumes of coagulated tissues relative to the amounts of reagents injected, considerably greater than acid alone in either technique employed. The largest volumes were obtained with sequential injection, yet this came at a price in one case of cardiac arrest. Simultaneous injection yielded the highest recorded temperatures and may be tolerated as well as or better than acid injection alone. Although this pilot study did not show a clear advantage for either sequential or simultaneous methods, the results indicate that thermochemical ablation is attractive for further investigation with regard to both safety and efficacy.
Acknowledgements
We thank the University of Minnesota Department of Radiology and the Society of Interventional Radiology Foundation Dr Ernest J. Ring Academic Development Award.
Declaration of interest: This work was supported in part by the National Institutes of Health, grant number P30 CA77598, utilising the Masonic Cancer Center, University of Minnesota shared resource: Biostatistics and Bioinformatics Core. The authors alone are responsible for the content and writing of the paper.
References
- Kuo YH, Lu SN, Chen CL, Cheng YF, Lin CY, Hung CH, et al. Hepatocellular carcinoma surveillance and appropriate treatment options improve survival for patients with liver cirrhosis. Eur J Cancer 2010; 46: 744–751
- Schumacher PA, Powell JJ, MacNeill AJ, Buczkowski AK, Erb SR, Ho SG, et al. Multimodal therapy for hepatocellular carcinoma: A complementary approach to liver transplantation. Ann Hepatol 2010; 9: 23–32
- Cabibbo G, Enea M, Attanasio M, Bruix J, Craxi A, Camma C. A meta-analysis of survival rates of untreated patients in randomized clinical trials of hepatocellular carcinoma. Hepatology 2010; 51: 1274–1283
- Germani G, Pleguezuelo M, Gurusamy K, Meyer T, Isgro G, Burroughs AK. Clinical outcomes of radiofrequency ablation, percutaneous alcohol and acetic acid injection for hepatocelullar carcinoma: A meta-analysis. J Hepatol 2010; 52: 380–388
- Clark TW. Chemical ablation of liver cancer. Tech Vasc Interv Radiol 2007; 10: 58–63
- Clark TW, Soulen MC. Chemical ablation of hepatocellular carcinoma. J Vasc Interv Radiol 2002; 13: S245–252
- Cho YB, Lee KU, Suh KS, Kim YJ, Yoon JH, Lee HS, et al. Hepatic resection compared to percutaneous ethanol injection for small hepatocellular carcinoma using propensity score matching. J Gastroenterol Hepatol 2007; 22: 1643–1649
- Fartoux L, Arrive L, Andreani T, Serfaty L, Chazouilleres O, Tubiana JM, et al. Treatment of small hepatocellular carcinoma with acetic acid percutaneous injection. Gastroenterol Clin Biol 2005; 29: 1213–1219
- Tsai WL, Cheng JS, Lai KH, Lin CP, Lo GH, Hsu PI, et al. Clinical trial: Percutaneous acetic acid injection vs. percutaneous ethanol injection for small hepatocellular carcinoma – A long-term follow-up study. Aliment Pharmacol Ther 2008; 28: 304–311
- Schoppmeyer K, Weis S, Mossner J, Fleig WE. Percutaneous ethanol injection or percutaneous acetic acid injection for early hepatocellular carcinoma. Cochrane Database Syst Rev 2009; 3: CD006745
- Arnulf F, Monika S, Herwig S, Monika H, Johannes PD, Gregor U, et al. Atropine for prevention of cardiac dysrhythmias in patients with hepatocellular carcinoma undergoing percutaneous ethanol instillation: A randomized, placebo-controlled, double-blind trial. Liver Int 2009; 29: 715–720
- Burton KR, O'Dwyer H, Scudamore C. Percutaneous ethanol ablation of hepatocellular carcinoma: Periprocedural onset alcohol toxicity and pancreatitis following conventional percutaneous ethanol ablation treatment. Can J Gastroenterol 2009; 23: 554–556
- Sidi A, Naik B, Urdaneta F, Muehlschlegel JD, Kirby DS, Lobato EB. Treatment of ethanol-induced acute pulmonary hypertension and right ventricular dysfunction in pigs, by sildenafil analogue (UK343-664) or nitroglycerin. Ann Card Anaesth 2008; 11: 97–104
- Sidi A, Naik B, Muehlschlegel JD, Kirby DS, Lobato EB. Ethanol-induced acute pulmonary hypertension and right ventricular dysfunction in pigs. Br J Anaesth 2008; 100: 568–569
- Da Ines D, Buc E, Petitcolin V, Flamein R, Lannareix V, Achim A, et al. Massive hepatic necrosis with gastric, splenic, and pancreatic infarctions after ethanol ablation for hepatocellular carcinoma. J Vasc Interv Radiol 2010; 21: 1301–1305
- Lencioni R, Crocetti L, Cioni D, Pina CD, Oliveri F, De Simone P, et al. Single-session percutaneous ethanol ablation of early-stage hepatocellular carcinoma with a multipronged injection needle: Results of a pilot clinical study. J Vasc Interv Radiol 2010; 21: 1533–1538
- Kuang M, Lu MD, Xie XY, Xu HX, Xu ZF, Liu GJ, et al. Ethanol ablation of hepatocellular carcinoma up to 5.0 cm by using a multipronged injection needle with high-dose strategy. Radiology 2009
- Ho CS, Kachura JR, Gallinger S, Grant D, Greig P, McGilvray I, et al. Percutaneous ethanol injection of unresectable medium-to-large-sized hepatomas using a multipronged needle: Efficacy and safety. Cardiovasc Intervent Radiol 2007; 30: 241–247
- Aravalli RN, Golzarian J, Cressman EN. Animal models of cancer in interventional radiology. Eur Radiol 2009; 19: 1049–1053
- National Research Council, National Academy of Sciences. Guide for the Care and Use of Laboratory Animals, 8th edition. Bethesda, MD: National Institutes of Health, 2011. Available at: http://grants.nih.gov/grants/olaw/Guide-for-the-care-and-use-of-Laboratory-animals.pdf (accessed 25 October 2011).
- Farnam JL, Smith BC, Johnson BR, Estrada R, Edelman TL, Farah R, et al. Thermochemical ablation in an ex-vivo porcine liver model using acetic acid and sodium hydroxide: Proof of concept. J Vasc Interv Radiol 2010; 21: 1573–1578
- Okuda S, Kuroda K, Oshio K, Mulkern RV, Colucci V, Morrison PR, et al. MR-based temperature monitoring for hot saline injection therapy. J Magn Reson Imaging 2000; 12: 330–338
- Goldberg SN, Grassi CJ, Cardella JF, Charboneau JW, Dodd GD, III, Dupuy DE, et al. Image-guided tumor ablation: Standardization of terminology and reporting criteria. J Vasc Interv Radiol 2009; 20: S377–390
- Mulier S, Ni Y, Frich L, Burdio F, Denys AL, De Wispelaere JF, et al. Experimental and clinical radiofrequency ablation: Proposal for standardized description of coagulation size and geometry. Ann Surg Oncol 2007; 14: 1381–1396
- Freeman LA, Anwer B, Brady RP, Smith BC, Edelman TL, Misselt AJ, et al. In vitro thermal profile suitability assessment of acids and bases for thermochemical ablation: Underlying principles. J Vasc Interv Radiol 2010; 21: 381–385
- Misselt AJ, Edelman TL, Choi JH, Bischof JC, Cressman EN. A hydrophobic gel phantom for study of thermochemical ablation: Initial results using a weak acid and weak base. J Vasc Interv Radiol 2009; 20: 1352–1358
- Deng ZS, Liu J. Minimally invasive thermotherapy method for tumor treatment based on an exothermic chemical reaction. Minim Invasive Ther Allied Technol 2007; 16: 341–346
- Cressman EN, Tseng HJ, Talaie R, Henderson BM. A new heat source for thermochemical ablation based on redox chemistry: Initial studies using permanganate. Int J Hyperthermia 2010; 26(4)327–337
- Tsai IC, Huang JW, Chu TS, Wu KD, Tsai TJ. Factors associated with metabolic acidosis in patients receiving parenteral nutrition. Nephrology 2007; 12: 3–7
- Arrive L, Rosmorduc O, Dahan H, Fartoux L, Monnier-Cholley L, Lewin M, et al. Percutaneous acetic acid injection for hepatocellular carcinoma: Using CT fluoroscopy to evaluate distribution of acetic acid mixed with an iodinated contrast agent. Am J Roentgenol 2003; 180: 159–162
- Tapani E, Vehmas T, Voutilainen P. Effect of injection speed on the spread of ethanol during experimental liver ethanol injections. Acad Radiol 1996; 3: 1025–1029