Abstract
Hyperthermia (HT) is a potent sensitiser for radiotherapy (RT) and chemotherapy (CT) and has been proven to modulate directly or indirectly cells of the innate and adaptive immune system. We will focus in this article on how anti-tumour immunity can be induced by HT. In contrast to some in vitro assays, in vivo examinations showed that natural killer cells and phagocytes like granulocytes are directly activated against the tumour by HT. Since heat also activates dendritic cells (DCs), HT should be combined with further death stimuli (RT, CT or immune therapy) to allocate tumour antigen, derived from, for example, necrotic tumour cells, for uptake by DCs. We will outline that induction of immunogenic tumour cells and direct tumour cell killing by HT in combination with other therapies contributes to immune activation against the tumour. Studies will be presented showing that non-beneficial effects of HT on immune cells are mostly timely restricted. A special focus is set on immune activation mediated by extracellular present heat shock proteins (HSPs) carrying tumour antigens and further danger signals released by dying tumour cells. Local HT treatment in addition to further stress stimuli exerts abscopal effects and might be considered as in situ tumour vaccination. An increased natural killer (NK) cell activity, lymphocyte infiltration and HSP-mediated induction of immunogenic tumour cells have been observed in patients. Treatments with the addition of HT therefore can be considered as a personalised cancer treatment approach by specifically activating the immune system against the individual unique tumour.
Introduction
Hyperthermia (HT) is a clinical treatment for malignant diseases, in which tumour tissue should be heated to minimum temperatures of 40–41°C for a sufficiently long period of time. HT has been proven to modify blood circulation thereby delivering oxygen into the tumour tissue, to result in increased metabolism leading to reduced ATP levels and increased anaerobic metabolites, to foster protein aggregation aggravating DNA repair, to sensitise tumour cells for radio- and chemotherapy, and to modulate the immune system (summarised in Schildkopf et al. Citation[1]). However, talking as radiation immune biologists about HT nearly always evokes divided reactions. This is mainly due to contradictory published results ranging from immune activation to immune suppression induced by HT. The inconsistent outcomes after HT are often due to different temperatures and exposure times used for the experiments. We will outline in this review old and new facts about how HT modulates the immune system and will carve out factors responsible for immune activatory modes of action of HT treatment.
As hour of birth of immune therapy is often regarded the treatment of cancer patients with Coley's toxin, developed by Sir William Bradley Coley at the end of the 19th century. Killed bacterial cultures were directly injected into the tumour or the blood stream evoking strong fever with resultant spontaneous tumour regressions observed in some patients. Those findings merit a place in history, but also in the present and the future Citation[2]. We will outline that ‘historic results’ have many implications for the future of HT as an additional element for anti-cancer therapy by inducing, besides many other effects, anti-tumour immunity, most pronounced when combined with radiotherapy (RT) and/or chemotherapy (CT) or further immune therapies (IT).
In the beginning of the 20th century many studies on the pathogenesis of fever were carried out and the linkage to cells of the innate immune system was made Citation[3]. It was discovered that fever in response to exogenous agents is mediated by a host phagocyte product called endogenous pyrogen (EP). Pyrogenes are released by phagocytosing granulocytes and stimulate immune responses. EP was later found to be identical to interleukin (IL)-1, being a powerful immune stimulator Citation[4]. Such findings suggest a strong relationship between fever, heat and immune responses Citation[5]. HT was further found to affect metabolism and membrane potential of innate immune cells (granulocytes) Citation[6]. Rosen suggested that increased body temperatures after HT lead to modifications of cellular membranes; the tumour cell loses its ‘random properties’. He concluded that HT increases the efficacy of the immune system Citation[7]. The latter might be the main mediator of the abscopal effects observed after distinct settings of HT treatment Citation[8]. Before summarising the literature on immune modulations induced by whole body HT (WBHT) and local HT (LHT) application, we will briefly introduce the immune system.
Basics about innate and adaptive immunity
An immune response can be divided into two main phases, namely an initial non-specific phase (innate immunity) mediated by granulocytes, monocytes, immature dendritic cells (DCs), natural killer (NK) cells, and soluble factors such as cytokines and a later specific phase (adaptive immunity) mediated by cellular (T lymphocytes) and humoral (B lymphocytes) immune reactions. The initial innate immune response uses germline genes to recognise foreign substances or damaged tissue. The adaptive one utilises somatically rearranged genes to generate multiple structural specificities that allow the induction of responses specific to individual invading organisms and damaged cells (modified from Colaco Citation[9]). It has become clear that these two responses are integrated in the response to an infectious organism and to ‘danger’ in the body Citation[10]. DCs were discovered by the Nobel Prize in Physiology or Medicine 2011 laureate Ralph Steinman, being the antigen presenting cells (APCs) as the site of this integration Citation[11].
The innate immune system
Cells of the innate immune system act as first line defence against invading pathogens. Via pattern recognition receptors (PPR) on the surface of phagocytes (monocytes, macrophages, granulocytes, immature DCs), pathogen-associated molecular patterns (PAMP) such as lipopolysaccharide (LPS) are recognised. After binding to receptors, such as Toll-like receptors (TLR), the pathogens are engulfed by phagocytes. The latter secrete cytokines initiating inflammation and the induction of adaptive immune responses. Like PAMP, damage-associated molecular patterns (DAMP) are capable of active immune responses in a similar way Citation[12] (). DAMP result from damaged cells, for example, after exposure of tumour cells to RT and HT Citation[13]. Besides phagocytes, NK cells belong to the cellular innate immune defence system. They possess activating and inhibitory receptors and recognise virus infected or tumour cells often displaying a reduced MHC class I surface expression Citation[14].
Figure 1. Hyperthermia modulates innate and adaptive immune responses. A general scheme of the main cells of the innate and adaptive immune systems that are involved in anti-tumour responses and can be modulated by hyperthermia is displayed. Heat damaged tumour cells expose damage-associated molecular patterns (DAMP) and can be recognised by innate immune cells via pattern recognition receptors (PRR). Natural killer (NK) cells are activated against the tumour via triggering activating receptors (AR) and inhibiting inhibitory receptors (IR) via MHC loss of the tumour cells. A beneficial cytokine milieu is created in the tumour microenvironment when innate immune cells are exposed to heat. Dendritic cells (DC) link the innate and adaptive immune response and take up heat shock protein/tumour antigen (Ag) complexes by way of heat shock protein receptors (HSPR). DCs present the tumour Ag to T cells and heat induces maturation and migration of DCs to lymph nodes (LN). There, T cells are activated in a MHC-dependent manner. They expand and traffick to the tumour cells by passing through high endothelial venules (HEV). Finally, the tumour cells are attacked and killed by the activated CD8+ T cells (cytotoxic T lymphocytes, CTL). As outlined in detail in the text, hyperthermia fosters in vivo all of the presented immune mechanisms against the tumour cells.
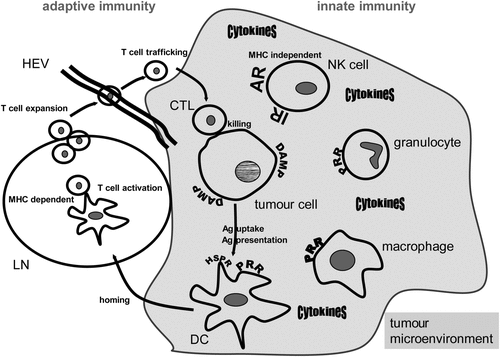
The adaptive immune system
In contrast to non-MHC restricted tumour cell recognition by NK cells, the triggering of adaptive immune responses requires uptake and presentation of antigen via MHC molecules by APCs. DCs are immune cells connecting innate and adaptive immunity and belong to the group of APCs. After recognition, uptake and processing of tumour antigen (Ag) resulting, for example, from damaged tumour cells, DCs mature and migrate into the lymph nodes (LN) to present peptides of tumour-associated Ag on their MHC complexes, express co-stimulatory receptors and secrete cytokines to prime naïve CD4+ and CD8+ T-lymphocytes against the presented Ag ().
Antigens derived from intracellular sources such as viruses are presented on MHC class I to CD8+ T cells whereas extracellular-derived phagocytosed material such as tumour peptides/Ag are usually presented on MHC class II to CD4+ T cells. However, the latter mentioned material can also be cross-presented on MHC class I molecules, a prerequisite for priming of CD8+ cytotoxic T cells (CTL). CTL expand and traffic from the LN to the tumour where they exert their killing function. Importantly, efficient cross-presentation of tumour Ag requires both Ag uptake and a maturation signal for DCs resulting from, for example, necrotic tumour cells Citation[15]. Heat shock proteins (HSPs) comprise both functions by delivering tumour Ag and fostering maturation of DCs Citation[16], Citation[17]. DCs pulsed with heat shocked tumour cells mediated a significant enhanced CD4+/8+ cellular cytotoxicity response against the tumour cells compared to pulsed DCs with lysates of non-heat shocked cells Citation[18]. It has to be stressed that adaptive and innate immune responses complement each other. When tumour cells shed MHC class I molecules to escape killing by CTL, NK cells are activated against the tumour since inhibitory receptors are no longer triggered by MHC I molecules. Furthermore, a cross-talk between NK cells and DCs exists. As example, NK cells were shown to foster the maturation of DCs Citation[19] and DC-NK cell contact promotes NK cell proliferation Citation[20].
Since hyperthermia in febrile (38–41°C) or tumour therapeutic (≥41°C) ranges is known to modulate interactions between the host immune and the tumour cells (summarised in Tomasovic and Klostergaard Citation[21]), we focus in the following sections on how WBHT or LHT modulate innate and adaptive immune responses.
General effects of HT on innate and adaptive immune responses
Many studies have been carried out analysing the effect of WBHT on immune cell subsets. Of note is that local tumour treatments might also directly affect immune cells, since mononuclear leukocytes are commonly present in a heated tumour or in the surrounding tissues. For this reason, researchers started to examine the effects of HT on macrophages. The response of macrophages to phytohaemagglutinin (PHA) measured by tritiated thymidine incorporation was enhanced when fever-range heat (38.5°C) was added while influenza virus depressed the macrophages` responsiveness Citation[22]. An enhanced production of virus-induced interferon by HT might be the cause of the beneficial effect of HT on responsiveness of influenza virus-exposed monocytes Citation[23]. Also lymphocyte transformation, mitogenesis, in response to PHA is temperature dependently influenced Citation[24]. Furthermore, IL-1 induced T cell proliferation was found to be increased by HT Citation[4]. This systemic activation of the immune system might contribute to target metastatic tumour cells by HT (summarised in von Ardenne Citation[25]). Another hint for the induction of anti-tumour immunity by heat is given by the fact that migration of Langerhans cells (DCs of the skin) is influenced by WBHT Citation[26] and that DCs primed with Toll-like receptor-agonists respond to HT (39.5°C) with significant increased IL-12p70 secretion. This is the main cytokine that drives TH1 polarisation Citation[27]. In general, heat induces an up-regulation of TLR4 on DCs and macrophages Citation[28]. In addition, HT in the fever range (38-41°C) resulted in in vitro experiments in a highly significant increase in L-selectin-dependent adhesion of these cells to LN high endothelial venules (HEV) Citation[29] and stimulates lymphocyte homing to secondary lymphoid tissues. This is mediated by an increased expression of L-selectin and alpha4beta7 integrin on circulating lymphocytes. They then interact specifically with HEV Citation[30].
Modulation of NK cells by HT
The modulation of NK cells by WBHT is a double-edged sword. NK cell activity was found to be decreased at higher temperatures in several in vitro assays Citation[31]. However, NK cell activity was observed to be enhanced by HT in vivo indicating that additional factors such as interferons resulting from latent infections contribute to the immune stimulating activity of heat Citation[32]. WBHT with 40.5°C resulted in decreased burden of lung metastases in mice, and NK cells were found to be involved in those anti-tumour effects induced by HT Citation[33]. In xenogeneic tumour models with SCID mice, host NK cells and injected human NK cells were enriched at the tumour site after HT treatment. NK cell depletion led to highly reduced amounts of dead tumour cells after HT Citation[34]. However, WBHT also suppresses the granzyme B/perforin NK cell mediated killing of tumour cells. Nevertheless, combination of WBHT with stimulation of NK cells by alpha-galactosylceramide ligand significantly retarded tumour progression and enhanced survival of the mice Citation[35]. A summary of how HT modulates NK cell activities was published some years ago by Repasky and colleagues Citation[36].
Modulation of granulocytes by HT
Several studies prove that even locally applied HT activates the innate immune system. HT increases the amount of neutrophilic granulocytes. Giving granulocyte colony stimulating factor (GCSF) in addition, a significant enhanced anti-tumour activity mediated via active oxygen species generation by neutrophils was observed in preclinical mouse models Citation[37]. Granulocytes displaying anti-tumour activity were enhanced in tumours after HT. This recruitment into the tumour could be also enhanced by addition of GCSF Citation[38]. Furthermore, an increase of the bactericidal capacity of granulocytes at 40°C Citation[39] and 42°C Citation[40] relative to 37°C was observed for many, but not all bacteria. However, higher temperature did not influence, at least in the applied in vitro systems, bactericidal capacity of macrophages. The authors concluded that HT enhances certain host defence mechanisms. Furthermore, peritoneal macrophages were not functionally suppressed or injured by microwave hyperthermia in vivo Citation[41].
Modulation of T and B cells by HT
In a similar manner to NK cells, beneficial and non-beneficial effects of heat on T cells were observed. HT resulted in a reduced in vitro cytolytic activity of CTL Citation[42]. Besides T cells, B cells are modulated as cells of the adaptive immune system by HT. In vivo, B lymphocytes are more susceptible to heat damage compared to T cells Citation[43]. However, this is only observed during the treatment, and many in vitro experiments were carried out just giving heat directly to the immune cells Citation[44]. In vivo, immune cells can recover Citation[45] and may interact with the immunogenic cells induced by HT Citation[46]. Heat induced transient lymphopenia in serum can even result in elevated levels of T and B cells in the spleen Citation[47].
T cells might migrate from the blood into tissue and come in contact with Ag Citation[39]. Experiments with immune deficient and silica (known to suppress macrophage function) injected mice and rats revealed that an activated macrophage–antigen–T cell processing is necessary for complete tumour cell destruction and systemic tumour control by local HT application Citation[48–50]. HT further enhances Fas-ligand mediated T cell cytotoxicity via over-expression of heat shock factor-1 (HSF-1) Citation[51]. Fas-L is a type II transmembrane protein able to trigger cell death by binding to its Fas receptor (CD95). The expression of CD95 by tumour cells may enable their killing by T cells via CD95/Fas-L-dependent mechanisms Citation[52]. schematically summarises the plethora of cells of the innate and adaptive immune system that are directly and/or indirectly activated by HT.
In general, starting from fever-like conditions (39°C), the immune cell activity of cancer patients is increased during heat application Citation[53]. High temperature short duration WBHT (41.8°C) enhanced the amount of lymphocytes, monocytes and granulocytes significantly shortly after treatment compared to low temperature, long duration WBHT (40°C) Citation[54]. In addition to the duration of heat application, timing of WBHT application is crucial. Since heat fosters migration of Langerhans cells out of the skin, it should be applied after another stimulus. In this scenario, migrating DCs are capable of delivering Ag to the LN Citation[55]. The beneficial effects of WBHT accentuate the additional mechanism of killing of cancer cells directly by HT, as outlined below, and contribute to anti-tumour efficacy Citation[56].
Direct tumour cell killing by HT contributes to immune activation
Overgaard's summary of the literature until 1972 indicated that tumour cells can be directly killed by HT with higher temperatures Citation[57]. Cavaliere and colleagues previously used temperatures of 42°C in their studies Citation[58]. Nowadays it has become clear that heat-mediated cell killing may result in immune stimulation Citation[59]. Early studies already revealed that the immunogenicity of the tumour cells can be increased by HT Citation[60]. Repeated local HT treatment of tumours resulted in significant reduction in the weight of retroperitoneal metastases in a mamma carcinoma model in rats. On the day of the HT treatment the tumours displayed necrotic areas and cellular injury Citation[61]. Some in vitro models have shown that Ag shedding of the tumour cells was fostered by HT Citation[62]. This again highlights that multiple tumour Ag should be accessible after treatment of tumours with HT, a scenario that can be achieved by induction of necrotic tumour cells with, for example, RT plus HT Citation[13], Citation[63]. HT further resulted in increased transcription of several tumour-associated Ag Citation[64].
Non-beneficial effects of HT on immune cells are timely restricted
HT may also impair functions and counts of immune cells such as NK cells and monocytes Citation[65]. This effect is mostly time restricted (recovery usually after 48 h) Citation[66], Citation[67] and can be avoided by targeted application of HT Citation[68]. Analyses of mRNA level on the effect of HT on immune cells within the tumour showed that the suppression in gene expression of a range of immune cells is only a transient phenomenon Citation[69]. Localised application of HT in a preclinical model with sheep resulted in an increased lymph flow and most importantly lymphocyte trafficking Citation[70]. Local HT application on legs of mice resulted primarily in the suppression of NK cell activity. However, after 2 days the activity was partially recovered and even enhanced after another 5 days Citation[71]. Furthermore, the amount and density of Langerhans cells is increased after local application of HT on tongues of rats Citation[72]. A plethora of differences in beneficial and non-beneficial effects of HT are mostly due to varying heating times and stress conditions. It will be of great importance to focus in the future on distinct temperatures and heating times as well as efficient temperature controls Citation[73]. The thermal dose is mandatory (summarised in Milani and Noessner Citation[74]) and more complete time and temperature analyses in vitro and in vivo for various types of immune cells and end points are needed Citation[75].
In the following we will go into detail how a local HT treatment finally acts systemically. One way this could happen is that HT-induced denaturation of proteins results in the unfolded protein response (UPR), in release of processed Ag bound to HSP, and finally to activation of T cells by those Ag presented by DCs ().
Figure 2. Hyperthermia modified tumour cells are rendered immunogenic and should be regarded as in situ tumour vaccine. When a tumour cell is heated, protein aggregation and denaturation induces a stress response in the cell, the so called unfolded protein response (UPR). Consequently, the transcription of inducible heat shock protein 70 (Hsp70) is increased and tumour cells expose even more Hsp70 on their surface. Furthermore, hyperthermia (HT) results in enhanced levels of tumour antigens (Ag) inside the cell. A second stress stimulus for the tumour cells such as ionising irradiation (radiotherapy, RT), chemotherapy (CT) or immune therapy (IT) together with HT fosters the induction of necrotic tumour cell death forms and modifies the tumour cell surface. Since necrotic cells have lost their membrane integrity, HSPs acting as danger signals and HSP/tumour Ag complexes are released. In addition, HSPs and tumour Ag containing exosomes can be discharged from apoptotic and necrotic tumour cells. Hsp70 containing exosomes derived from heat stressed tumour cells as well as HSP/tumour Ag complexes activate and attract dendritic cells (DC). The latter take up tumour Ag, present it with co-stimulation to CD8+ T cells and thereby induce cellular anti-tumour immunity by priming cytotoxic T lymphocytes (CTL).
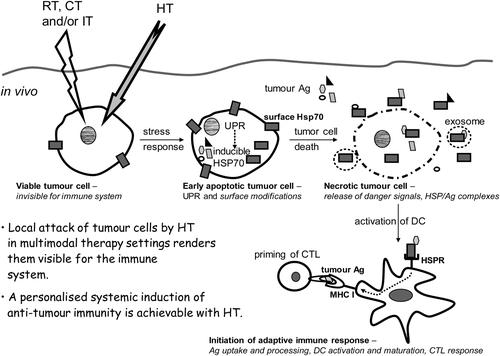
HT-induced HSP-dependent immune activation can dominate over thermo-tolerance
Before the 1980s HSPs were only known to chaperone intracellular proteins after cell stress Citation[76]. Hsp70 may act as cell survival protein by inhibiting the permeabilisation of lysosomal membranes Citation[77]. It also protects tumour cells from monocyte cytotoxicity mediated by TNF Citation[78]. HSPs are highly conserved constituents of all kinds of pro- and eukaryotic cells and appeared for a long period of time only to be connected with thermo-tolerance. In addition, a simplified view that HT may lead to immune suppression via induction of thermo-tolerance in tumour cells and RT via destroying immune cells has been in the mind of clinicians, scientists and the public for many years. However, HSPs have to be regarded as double-edged swords Citation[79], Citation[80]. Nowadays it becomes more and more evident that besides a general and timely restricted immune suppression, treatments with HT and/or RT may result in specific activation of the immune system by induction of distinct modifications of the tumour cell surface and distinct forms of cell death Citation[81].
Extracellular HSPs are immunogenic
‘New facts’ about HSP-mediated immune effects arose with the key findings in the 1980s and 1990s that intracellular and inducible HSPs may become immunogenic when complexed with tumour peptides Citation[82] and that HSPs are also found outside the cells as well as located at the tumour cell surface. Viable tumour cells of a plethora of tumour entities expose Hsp70 on their surface. This represents a unique feature to discriminate them from non-cancerous cells Citation[83]. RT has been found to further increase the amount of Hsp70 on the surface of the tumour cells Citation[84]. Heat treatment also induces exposure of Hsp72 on the tumour cell surface, thereby rendering the cell susceptible to lysis mediated by NK effector cells Citation[85]. Of note is that HSPs are not only increased at the tumour cell's surface after RT, but can also additionally be released. Increased serum levels of Hsp72 were detected in patients with prostate cancer Citation[86]. In addition, the expression of inducible Hsp70 correlates with increased tumour cell immunogenic properties Citation[87] when complexed with tumour antigens (discussed and summarised by Srivastava Citation[82]) by mediating antigen cross-presentation via MHC class I molecules Citation[88]. APCs utilise the uptake of HSP-chaperoned peptides for the loading of MHC class I molecules and thus stimulate a specific T cell response Citation[89], Citation[9] (). Under oxidative stress, inducible Hsp70 was shown to be much more efficient in discriminating intracellular non-self-peptides than constitutive expressed Hsc70 Citation[90]. The UPR occurs as a stress response towards heat and results in activation of the transcription factor HSF1 which regulates the transcription of Hsp70 Citation[91]. The UPR was found to be responsible for the generation of new antigenic peptides since oncoproteins have unique characteristics and are processed via the proteasome.
Besides direct effects, activation of the immune system by HT also involves indirect effects mediated through release of HSP and HSP/peptide complexes Citation[92]. The mechanisms of Hsp70-mediated and DC-dependent induction of specific tumour cell killing by cytotoxic CD8+ T cells are summarised in Calderwood et al. Citation[93]. Active and passive release pathways of Hsp70 have been described Citation[94]. Passive release by necrotic cells and the consecutive uptake of HSP/peptide complexes by DCs can lead to efficient T cell priming Citation[95]. The current knowledge about the release of HSP by cells with intact membrane is based on the fact that intracellular Hsp70 is located in cholesterol-rich micro-domains and binds to globotriaosylceramide (Gb3) Citation[96]. It might be shuffled to the outside of the cellular membrane via regular flip-flop mechanisms Citation[97]. Released Hsp70 is often associated with exosomes Citation[98]. Hsp70-containing exosomes derived from heat stressed tumour cells have just recently been discovered to activate and attract DCs and T cells also via the chemokines CCL2, CCL3, CCL4, CCL5, and CCL20 Citation[99]. HSPs interact with multiple surface receptors of APCs Citation[93], such as CD91, LOX1, CD40, and TLR, thereby inducing the secretion of immune activatory cytokines Citation[100]. Released HSPs are therefore regarded as a danger signal that stimulate many steps in the induction of innate and adaptive immune reactions from DCs up to CTL activation Citation[101] (). Members of the Hsp70-related Hsp110 family cooperate with Hsp70 in protein folding in the cytosol Citation[102]. Heat-inducible proteins can be rendered more immunogenic by pre-treatment with heat, while heat-insensitive proteins were not modified in their vaccination efficacy. Hsp110 prepared from mice treated with WBHT were significantly better vaccines compared to those proteins isolated from non-heat treated animals. The authors concluded that Ag presentation pathways might be influenced by HT Citation[103].
HSPs are induced in target/tumour cells as well as in APCs. HSPs are part of intracellular aggregates with cytoskeletal proteins such as spectrin in immune cells. An exposure to mild HT (40°C) has the same effect on localisation changes of those aggregates within the cells as other stimuli leading to lymphocyte activation Citation[104]. DCs exposed to heat up-regulated Hsp70 and concomitantly the co-stimulatory markers CD80, CD83, and CD86. In addition, a significant increased capacity of heat stressed DCs to prime CD8+ T cells was observed Citation[105]. Fever-like temperature further leads to increased expression of Hsp90 in immune cells and thereby induces maturation of DCs Citation[106]. Since HSPs are strongly induced by thermal stress and their expression is only weakly modulated by electromagnetic fields Citation[107], the current notion is that heat acts as main stimulator of HSP expression and immune stimulation.
The exposure of Hsp70 on the tumour cell surface serves as a recognition signal for activated NK cells Citation[108]. SCID/beige mice are deficient for T and B cells and lack additionally functional NK cells. In those mice the growth of Hsp70-over-expressing tumours was not reduced compared with control tumours. In contrast, in SCID mice (having functional NK cells) bearing Hsp70-over-expressing tumours, NK cells were activated and killed tumour cells ex vivo that expressed NKG2D ligands Citation[98]. The migratory and killing activity of NK cells was found to be stimulated by Hsp70 positive exosomes derived from tumour cells Citation[109]. In general, the activity of NK cells can be increased by up-regulation of activating receptors and down-regulation or blocking of inhibitory ones. Heat also up-regulates Hsp60 that binds to HLA-E MHC molecules. This complex is no longer recognised by CD94/NKG2A inhibitory receptors Citation[110].
Taken together, cells of the innate (NK cells and DCs) and of the adaptive (CTL) immune system become activated by heat stress induced Hsp70. Importantly, the necessity of a cross-talk between NK cells and DCs for the induction of specific anti-tumour immune responses becomes more and more clear and Hsp70 is one mediator of this cell-to-cell contact-dependent interaction. For example, Hsp70 induced the expression of the NKG2D ligand MICA (the MHC class I chain-related protein A) on DCs Citation[111].
Immunogenic tumour cell death induced by combinatory treatments with HT
Many additive and even supra-additive anti-tumour effects have been described when combining RT with HT Citation[112]. As one example, HT increases the blood flow and thereby improves tumour tissue oxygenation leading to a better outcome of RT Citation[113]. The complementary modes of action of RT and HT are summarised in Schildkopf et al. and van der Zee Citation[1], Citation[112]. One major factor of the enhanced radiosensitivity of tumour cells after heat application is the inhibition of the repolymerisation step in the repair of base damages induced by RT Citation[114]. Just recently it was demonstrated that HT induces Breast Cancer 2 susceptibility protein (BRCA2) degradation and thereby inhibits homologous recombination Citation[115]. Besides radiosensitisation based on interference with cellular DNA, combinatory treatments of RT and HT may result in immune activation against tumour cells that have been modified by the therapy.
Combinations of RT with HT induce immunogenic necrotic tumour cells
Modification of the tumour cell surface or the release of danger signals via damaged tumour cell membranes may render the tumour cells as visible targets for immune attack. Heated cells modify their surface in many ways, like that antibodies bind differently. HT treatment (43.5°C) enhanced cytotoxicity by antibodies mono-specific to a certain tumour Ag, suggesting that HT is capable of augmenting specific immune reactions against tumour-associated cell membrane Ag Citation[116]. Fresh spleen cells of mice inoculated with heat-treated tumour cells (42°C for 30 min) showed a clear tumour-neutralising activity Citation[117]. Tumour cells that are exposed to two stress stimuli, such as RT and HT, die of apoptosis and necrosis Citation[13]. Heat-induced apoptosis is mediated via caspase-9 Citation[118]. Besides apoptotic cells, forms of programmed necrosis can also be induced in tumour cells after HT, RT or HT plus RT Citation[119]. Therefore, some effects of HT lie in killing of tumour cells and consecutive activation of the immune system Citation[120]. Already in the 1970s, Muckle and colleagues suggested that mode and form of tumour cell death and consecutive uptake of necrotic tumour cell material following treatment could be important in enabling the host to deal with metastatic cells. This indicates that local RT and HT treatment can induce systemic immune-mediated effects Citation[121]. Induction of tumour cell necrosis by local HT with 42°C resulted in complete disappearance of the tumour in one half of the mice. A long disease-free survival of the mice was observed (up to 18 months) suggesting that HT led to systemic tumour control. Of note, the non-surviving animals had defective immune systems Citation[122]. At temperatures below 41°C no necrosis occurred, whereas at temperatures between 42° and 45°C an increased rate of necrosis is observed Citation[123]. Necrotic cell death with release of danger signals can be induced by HT and finally leads to cross-priming of tumour Ag by DCs Citation[124]. In contrast, a chronic enhanced level of HMGB1 fosters inflammation, angiogenesis, evasion of cell death, and metastases Citation[125]. Of note is that even a resistance to tumour growth can be stimulated by pre-treatment of mice with two stimuli (heat plus X-ray) before tumour inoculation. In this model, the resistance was independent of T cells, as revealed by experiments with nude mice Citation[126]. One has not to conceal that WBHT with lower temperatures was not effective in inducing anti-tumour immunity and even led to compensation of anti-tumour immune responses triggered by whole tumour cell vaccines Citation[127].
Combinations of cytokines with HT induce immune activation
Researchers and clinicians became even more aware in the 1980s that cancer is a complex disease not only related to the tumour cells themselves. To treat the very heterogeneous neoplastic diseases, a multimodal approach should be followed consisting of surgery, radiotherapy, chemotherapy, immunotherapy and/or HT Citation[128], Citation[129]. How HT enhances the cytotoxicity of chemotherapeutic drugs at multiple levels is excellently summarised by Issels Citation[130].
The most prominent anti-tumour effect in mice bearing Lewis lung carcinoma was observed using IL-2 therapy combined with local HT Citation[131]. A beneficial targeting of metastatic tumours was also achieved with combinations of IL7, being important for B and T cell development, and HT Citation[132]. HT actually abrogated the inflammatory and thereby anti-immunotherapeutic effect of IL-8 Citation[133]. Application of HT with a further trigger results in increased TNF-alpha levels secreted by macrophages Citation[134]. Distinct sequences for macrophage triggering or treatment of tumour cells with tumour necrosis factor plus application of HT is capable of augmenting the cytotoxic actions of macrophages against the tumour cells Citation[135]. HT leads to fast, significantly increased, but timely restricted secretion of cytokines such as TNF-alpha and IL-1-beta fostering early activation of host defence immune mechanisms. This timely restricted pulsing of the immune system induced by HT is comparable to the new facts of action of HT where HMGB1 is released from tumour cells after RT plus HT Citation[13]. Combination therapies such as adding TNF-alpha to HT also resulted in massive tumour cell necrosis Citation[136]. As mentioned before, the pulsatile release of danger signals such as HMGB1 by necrotic cells fosters cross-presentation of Ag by DCs Citation[137].
Taken together, especially combinations of HT with further stimuli may lead to efficient anti-tumour immune responses. Sub-lethal damages induced by CT or RT are rendered lethal by additional application of HT Citation[138]. This is again another important reason for the recommended combined cancer treatment with HT plus RT and/or CT. In preclinical models it has been shown that HT added to RT increases local tumour control rates from 25% up to nearly 90% Citation[139], but also systemic tumour control is improved by combination of HT plus RT, as proven by longer survival rates of the patients Citation[140].
Combinations of HT with further immune therapies induce immune activation
Very effective anti-tumour responses were observed in preclinical models when HT was combined with further immune therapy, namely injection of DCs Citation[141], Citation[142]. DCs were activated and matured by Hsp70. In addition, HSPs fostered an increased CTL and NK cell activity Citation[143]. DCs loaded with melanoma cells that were heated to 42°C before killing were more efficient in priming of naive CD8+ T cells than DCs loaded with unheated melanoma cells Citation[64], indicating that HT fostered cross-priming of tumour Ag by DCs. In addition, heat shocked DCs themselves were potent stimulators of cytotoxic T cell responses against thyroid carcinoma Citation[144]. Treatment with Flt3L (which induced proliferation of DCs) and local RT led to abscopal anti-tumour effects Citation[145] and to eradication of small lung metastases Citation[146]. The induction of abscopal anti-tumour immunity and immunogenic tumour cell death by RT and further immune stimulation was recently summarised by Frey et al. Citation[147].
DC activation after combined HT therapies as key inductor of anti-tumour immune responses
In conclusion, local HT can lead to local, but also to systemic tumour control. A prerequisite for the latter is the migration of DCs that have taken up tumour Ag to LN (homing). Increased expression of CCR7 and decreased expression of CCR6 on DCs are required for their functional migration to regional LN. Exactly this expression profile was observed on mRNA level for Langerhans cells after local HT Citation[148] and also on protein level after contact of DCs with supernatants of tumour cells that have been exposed to HT plus RT Citation[46]. The release of Hsp70 is significantly enhanced when HT is added to RT and extracellular Hsp70 is one main player in inducing DC maturation and homing Citation[149]. We have to stress again that the release of immune activatory proteins such as Hsp70 and danger signals such as HMGB1, which are normally located inside the cells, after local treatment with HT plus RT mediate abscopal anti-tumour effects (see below and ). Radiotherapy regimens and certain chemotherapeutic agents trigger forms of cancer cell death that stimulate an active immune response against the tumour Citation[81]. RT plus HT stimulates the DC-mediated CTL response against the tumour, but also increases the expression of activating NKG2D ligands for NK cells on tumour cells thereby activating innate immune defence mechanisms Citation[150]. The number of cytotoxic T cells and NK cells is significantly increased after local HT treatment of melanoma in mice. In addition, increased amounts of activated monocytes (CD11b+, CD69+) were present in the tumour microenvironment after HT Citation[151].
Local HT applications result in systemic immune activation
First hints for an activation of the immune system after local tumour treatments were the improved survival times of patients with melanoma Citation[152]. A combination of IL-2 and local HT was beneficial in preclinical models for certain metastatic tumours Citation[131]. Tumour cells with high metastatic potential were more sensitive to HT treatment and the survival rate of mice bearing metastatic B16-F10 tumours was increased after HT Citation[153]. Infiltration of NK cells and macrophages into the tumour, containing necrotic melanoma cells induced by HT, takes place after local HT treatment Citation[154]. Notably, the addition of rIFN-beta to HT had no effect on NK cell infiltration but significantly increased the amount of T cells in the tumour Citation[155]. In another preclinical study using the MCA-105 sarcoma metastatic cell model, WBHT in adjunct to immune therapy had no significant effect on tumour growth. However, the combination of LHT and immune therapy with lymphokine-activated killer cells significantly decreased the number of pulmonary metastases Citation[156]. LHT in addition to further stress stimuli might be considered as an autologous in situ tumour vaccination, as it is also true for RT combined with immune activators such as AnxA5 Citation[157]. Zhang and colleagues recently summarised in detail how LHT alone and more importantly in combination with further immune therapy or RT is capable of rendering tumours immunogenic in situ Citation[158].
Immune defence mechanisms have always to be considered for the treatment of small tumour masses, recurrent tumours, metastases and micrometastases (being not displayed by current imaging techniques). Combinations of RT, CT or surgery with HT kill two birds with one stone: the primary tumour is reduced in size by the RT, CT or surgery, and residual tumour masses and metastases are killed via immune activation with the addition of HT ().
HT activates systemic anti-tumour immune responses in cancer patients
HT and immunity fit together Citation[80]. The clinical effectiveness of HT treatment in multimodal settings is described in detail in other papers of this special issue. Many randomised clinical trials have proven the effectiveness of HT when combined with RT, RCT, or CT. Recently, a randomised phase III multi-centre study has demonstrated that regional HT significantly increases the benefit of CT in adult cancer patients with high-risk soft-tissue sarcoma Citation[159]. In the following we briefly mention some further studies clearly indicating that application of LHT and WBHT mostly in addition to RT and/or CT contribute to systemic tumour immune defence mechanisms. Patients with advanced adenocarcinoma of the prostate receiving local HT treatment displayed a significant NK cell cytotoxic activity when compared to the pre-treatment status Citation[160]. An increased NK cell activity after local HT application was even observed in patients with liver cancer Citation[161], being a tumour hard to treat with HT because of its high perfusion. Preoperative radiochemotherapy combined with local HT led to an increased lymphocyte infiltration and increased survival rate of patients with oesophageal cancer compared to radiochemotherapy treatment alone Citation[140]. A prolonged T cell activation was observed after WBHT at higher temperatures (41.8°C) in addition to CT in patients with metastatic colorectal carcinoma Citation[162]. Currently, combinations of HT with DC-based immune therapy are tested for therapy of squamous cell carcinoma. Without RT, a three-step therapy setting (HT with 43° and 41°C and DC vaccination) seems to be most beneficial in inducing anti-tumour immunity Citation[163].
In cervical cancer, a study comparing RT with RT plus HT revealed that the percentage of patients with continuing pelvic control developing metastatic disease was significantly lower in the group with combined treatment Citation[164]. This might be due to the HSP-mediated induction of immunogenic tumour cell death Citation[112]. Clinical trials using HSP/peptide complexes have been carried out Citation[165], Citation[166]. Patients showed a longer disease-free survival indicating that systemic anti-tumour immune responses were induced. HSPs become hyperabundant under stressful conditions. We emphasise that HSPs induced by HT may contribute to anti-tumour immunity. Smaller tumour masses in early stage diseases or metastases are attacked by those immune-mediated mechanisms Citation[167].
A phase III trial showed that M1a and M1b stage IV melanoma patients receiving larger numbers of immunisation with autologous HSP gp96-peptide complexes survived longer compared to patients receiving fewer treatments Citation[168]. The clinical results are consistent with observations in mouse models examining the ‘immunological power’ of HSP-based vaccines Citation[169]. Another randomised phase III trial with adjuvant treatment in renal cell carcinoma revealed that patients in early stage disease have a hazard ration for recurrence of disease of 0.57 when receiving the HSP/peptide vaccine Citation[170]. Of note, both trials failed to meet their end points with respect to the intention-to-treat population (receiving the HSP/peptide vaccine). Nevertheless, retrospective sub-group analyses clearly showed that distinct groups of patients receiving multiple vaccinations significantly profited from the immune therapeutic ‘vaccitherapy’ Citation[167].
Short outlook
Repeated in situ induction of HSP tumour peptide complexes by HT treatment in combination with strong death stimuli for the tumour cells such as ionising radiation might result in great clinical benefit in the future Citation[147]. The in situ induction or vaccination with autologous HSP tumour material applies the strong immunogenic potential of the unique tumour composition of each individual patient as therapeutic option Citation[167], Citation[171]. A personalised cancer treatment approach that takes into account the individually unique tumour composition is feasible by combining HT with further standard and immune therapies (). The temporarily restricted mode of modifying the tumour microenvironment by HT likely prevents the development of immunological tolerance. Hence, repeated HT treatment cycles included in multimodal therapy settings should be arranged. HT and induction of local and systemic anti-tumour immunity are things that have and still fit together even closer.
Declaration of interest: This work was supported by the German Research Foundation (GA 1507/1-1), the German Federal Ministry of Education and Research (BMBF; m4 Cluster, 01EX1021R and GREWIS 02NUK017G), and the European Commission's DoReMi, European Network of Excellence (contract number 249689). The authors alone are responsible for the content and writing of the paper.
References
- Schildkopf P, Ott OJ, Frey B, Wadepohl M, Sauer R, Fietkau R, et al. Biological rationales and clinical applications of temperature controlled hyperthermia – Implications for multimodal cancer treatments. Curr Med Chem 2010; 17: 3045–3057
- Hoption Cann SA, van Netten JP, van Netten C. Dr William Coley and tumour regression: A place in history or in the future. Postgrad Med J 2003; 79: 672–680
- Berlin RD, Wood WB, Jr. Studies on the pathogenesis of fever. 13. The effect of phagocytosis on the release of endogenous pyrogen by polymorphonuclear leukocytes. J Exp Med 1964; 119: 715–726
- Duff GW, Durum SK. Fever and immunoregulation: Hyperthermia, interleukins 1 and 2, and T-cell proliferation. Yale J Biol Med 1982; 55: 437–442
- Jampel HD, Duff GW, Gershon RK, Atkins E, Durum SK. Fever and immunoregulation. III. Hyperthermia augments the primary in vitro humoral immune response. J Exp Med 1983; 157: 1229–1238
- Redmann K, Burmeister J, Jenssen HL. The influence of hyperthermia on the transmembrane potential, zeta-potential and metabolism of polymorphonuclear leukocytes. Acta Biol Med Ger 1974; 33: 187–196
- Rosen P. Random repression, immunological surveillance and hyperthermia. Cancer Lett 1976; 2: 59–61
- Goldenberg DM, Langner M. Direct and abscopal antitumor action of local hyperthermia. Z Naturforsch B 1971; 26: 359–361
- Colaco C. Function of fever. J R Soc Med 1997; 90: 587–588
- Matzinger P. An innate sense of danger. Semin Immunol 1998; 10: 399–415
- Steinman RM, Cohn ZA. Identification of a novel cell type in peripheral lymphoid organs of mice. I. Morphology, quantitation, tissue distribution. J Exp Med 1973; 137: 1142–1162
- Seong SY, Matzinger P. Hydrophobicity: An ancient damage-associated molecular pattern that initiates innate immune responses. Nat Rev Immunol 2004; 4: 469–478
- Schildkopf P, Frey B, Mantel F, Ott OJ, Weiss EM, Sieber R, et al. Application of hyperthermia in addition to ionizing irradiation fosters necrotic cell death and HMGB1 release of colorectal tumor cells. Biochem Biophys Res Commun 2010; 391: 1014–1020
- Ljunggren HG, Karre K. In search of the ‘missing self’: MHC molecules and NK cell recognition. Immunol Today 1990; 11: 237–244
- Sauter B, Albert ML, Francisco L, Larsson M, Somersan S, Bhardwaj N. Consequences of cell death: Exposure to necrotic tumor cells, but not primary tissue cells or apoptotic cells, induces the maturation of immunostimulatory dendritic cells. J Exp Med 2000; 191: 423–434
- Basu S, Binder RJ, Suto R, Anderson KM, Srivastava PK. Necrotic but not apoptotic cell death releases heat shock proteins, which deliver a partial maturation signal to dendritic cells and activate the NF-kappa B pathway. Int Immunol 2000; 12: 1539–1546
- Somersan S, Larsson M, Fonteneau JF, Basu S, Srivastava P, Bhardwaj N. Primary tumor tissue lysates are enriched in heat shock proteins and induce the maturation of human dendritic cells. J Immunol 2001; 167: 4844–4852
- Schueller G, Stift A, Friedl J, Dubsky P, Bachleitner-Hofmann T, Benkoe T, et al. Hyperthermia improves cellular immune response to human hepatocellular carcinoma subsequent to co-culture with tumor lysate pulsed dendritic cells. Int J Oncol 2003; 22: 1397–1402
- Wong JL, Mailliard RB, Moschos SJ, Edington H, Lotze MT, Kirkwood JM, et al. Helper activity of natural killer cells during the dendritic cell-mediated induction of melanoma-specific cytotoxic T cells. J Immunother 2011; 34: 270–278
- Ferlazzo G, Pack M, Thomas D, Paludan C, Schmid D, Strowig T, et al. Distinct roles of IL-12 and IL-15 in human natural killer cell activation by dendritic cells from secondary lymphoid organs. Proc Natl Acad Sci USA. 2004; 101: 16606–16611
- Tomasovic SP, Klostergaard J. Hyperthermic modulation of macrophage-tumor cell interactions. Cancer Metastasis Rev 1989; 8: 215–229
- Manzella JP, Roberts NJ, Jr. Human macrophage and lymphocyte responses to mitogen stimulation after exposure to influenza virus, ascorbic acid, and hyperthermia. J Immunol 1979; 123: 1940–1944
- Postic B, DeAngelis C, Breinig MK, Monto HO. Effect of temperature on the induction of interferons by endotoxin and virus. J Bacteriol 1966; 91: 1277–1281
- Skeen MJ, Olkowski ZL, DuPre JR, McLaren JR. Mitogenesis in human lymphocytes following brief exposure to hyperthermia. Int J Radiat Oncol Biol Phys 1983; 9: 61–66
- von Ardenne M. Selective multiphase cancer therapy: Conceptual aspects and experimental basis. Adv Pharmacol Chemother 1972; 10: 339–380
- Ostberg JR, Patel R, Repasky EA. Regulation of immune activity by mild (fever-range) whole body hyperthermia: Effects on epidermal Langerhans cells. Cell Stress Chaperones 2000; 5: 458–461
- Peng JC, Hyde C, Pai S, O'sullivan BJ, Nielsen LK, Thomas R. Monocyte-derived DC primed with TLR agonists secrete IL-12p70 in a CD40-dependent manner under hyperthermic conditions. J Immunother 2006; 29: 606–615
- Yan X, Xiu F, An H, Wang X, Wang J, Cao X. Fever range temperature promotes TLR4 expression and signaling in dendritic cells. Life Sci 2007; 80: 307–313
- Wang WC, Goldman LM, Schleider DM, Appenheimer MM, Subjeck JR, Repasky EA, et al. Fever-range hyperthermia enhances L-selectin-dependent adhesion of lymphocytes to vascular endothelium. J Immunol 1998; 160: 961–969
- Evans SS, Wang WC, Bain MD, Burd R, Ostberg JR, Repasky EA. Fever-range hyperthermia dynamically regulates lymphocyte delivery to high endothelial venules. Blood 2001; 97: 2727–2733
- Azocar J, Yunis EJ, Essex M. Sensitivity of human natural killer cells to hyperthermia. Lancet 1982; 1: 16–17
- Zanker KS, Lange J. Whole body hyperthermia and natural killer cell activity. Lancet 1982; 1: 1079–1080
- Shen RN, Hornback NB, Shidnia H, Shupe RE, Brahmi Z. Whole-body hyperthermia decreases lung metastases in lung tumor-bearing mice, possibly via a mechanism involving natural killer cells. J Clin Immunol 1987; 7: 246–253
- Burd R, Dziedzic TS, Xu Y, Caligiuri MA, Subjeck JR, Repasky EA. Tumor cell apoptosis, lymphocyte recruitment and tumor vascular changes are induced by low temperature, long duration (fever-like) whole body hyperthermia. J Cell Physiol 1998; 177: 137–147
- Hattori T, Kokura S, Okuda T, Okayama T, Takagi T, Handa O, et al. Antitumor effect of whole body hyperthermia with alpha-galactosylceramide in a subcutaneous tumor model of colon cancer. Int J Hyperthermia 2007; 23: 591–598
- Dayanc BE, Beachy SH, Ostberg JR, Repasky EA. Dissecting the role of hyperthermia in natural killer cell mediated anti-tumor responses. Int J Hyperthermia 2008; 24: 41–56
- Takada Y, Sato EF, Nakajima T, Hosono M, Tsumura M, Inoue M, et al. Granulocyte-colony stimulating factor enhances anti-tumour effect of hyperthermia. Int J Hyperthermia 2000; 16: 275–286
- Kokura S, Yoshikawa T, Tainaka K, Kaneko T, Iinuma S, Nishimura S, et al. Anti-tumor effects of hyperthermia plus granulocyte colony-stimulating factor. Jpn J Cancer Res 1996; 87: 862–866
- Buhring M, Bork-Wolwer L, Krippner H, Pirlet K. Quantitative Veränderungen an peripheren T-Lymphozyten während der Hyperthermie [Quantitative changes in peripheral T-lymphocytes during hyperthermia (trans. author)]. MMW Munch Med Wochenschr 1977; 119: 1591–1594
- Grogan JB, Parks LC, Minaberry D. Polymorphonuclear leukocyte function in cancer patients treated with total body hyperthermia. Cancer 1980; 45: 2611–2615
- Rama Rao G, Cain CA, Lockwood J, Tompkins WA. Effects of microwave exposure on the hamster immune system. II. Peritoneal macrophage function. Bioelectromagnetics 1983; 4: 141–155
- Harris JW, Meneses JJ. Effects of hyperthermia on the production and activity of primary and secondary cytolytic T-lymphocytes in vitro. Cancer Res 1978; 38: 1120–1126
- Shah SA, Dickson JA. Effect of hyperthermia on the immune response of normal rabbits. Cancer Res 1978; 38: 3518–3522
- Fuggetta MP, Alvino E, Tricarico M, D’Atri S, Pepponi R, Prete SP, et al. In vitro effect of hyperthermia on natural cell-mediated cytotoxicity. Anticancer Res 2000; 20: 1667–1672
- Yang H, Lauzon W, Lemaire I. Effects of hyperthermia on natural killer cells: Inhibition of lytic function and microtubule organization. Int J Hyperthermia 1992; 8: 87–97
- Schildkopf P, Frey B, Ott OJ, Rubner Y, Multhoff G, Sauer R, et al. Radiation combined with hyperthermia induces Hsp70-dependent maturation of dendritic cells and release of pro-inflammatory cytokines by dendritic cells and macrophages. Radiother Oncol 2011; 101: 109–115
- Liburdy RP. Radiofrequency radiation alters the immune system: Modulation of T- and B-lymphocyte levels and cell-mediated immunocompetence by hyperthermic radiation. Radiat Res 1979; 77: 34–46
- Alfieri AA, Hahn EW, Kim JH. Role of cell-mediated immunity in tumor eradication by hyperthermia. Cancer Res 1981; 41: 1301–1305
- Shah SA. Participation of the immune system in regression of a rat Mc7 sarcoma by hyperthermia. Cancer Res 1981; 41: 1742–1747
- Dickson JA, Shah SA. Hyperthermia: The immune response and tumor metastasis. Natl Cancer Inst Monogr 1982; 61: 183–192
- Cippitelli M, Fionda C, Di Bona D, Piccoli M, Frati L, Santoni A. Hyperthermia enhances CD95-ligand gene expression in T lymphocytes. J Immunol 2005; 174: 223–232
- Russell JH, Ley TJ. Lymphocyte-mediated cytotoxicity. Annu Rev Immunol 2002; 20: 323–370
- Park MM, Hornback NB, Endres S, Dinarello CA. The effect of whole body hyperthermia on the immune cell activity of cancer patients. Lymphokine Res 1990; 9: 213–223
- Ostberg JR, Repasky EA. Comparison of the effects of two different whole body hyperthermia protocols on the distribution of murine leukocyte populations. Int J Hyperthermia 2000; 16: 29–43
- Ostberg JR, Gellin C, Patel R, Repasky EA. Regulatory potential of fever-range whole body hyperthermia on Langerhans cells and lymphocytes in an antigen-dependent cellular immune response. J Immunol 2001; 167: 2666–2670
- Skitzki JJ, Repasky EA, Evans SS. Hyperthermia as an immunotherapy strategy for cancer. Curr Opin Investig Drugs 2009; 10: 550–558
- Overgaard K, Overgaard J. Investigation on the possibility of a thermic tumour therapy. II. Action of combined heat-roentgen treatment on a transplanted mouse mammary carcinoma. Eur J Cancer 1972; 8: 573–575
- Cavaliere R, Ciocatto EC, Giovanella BC, Heidelberger C, Johnson RO, Margottini M, et al. Selective heat sensitivity of cancer cells. Biochemical and clinical studies. Cancer 1967; 20: 1351–1381
- Suzuki M, Shinkai M, Honda H, Kobayashi T. Anticancer effect and immune induction by hyperthermia of malignant melanoma using magnetite cationic liposomes. Melanoma Res 2003; 13: 129–135
- Mondovi B, Santoro AS, Strom R, Faiola R, Fanelli AR. Increased immunogenicity of Ehrlich ascites cells after heat treatment. Cancer 1972; 30: 885–888
- Schechter M, Stowe SM, Moroson H. Effects of hyperthermia on primary and metastatic tumor growth and host immune response in rats. Cancer Res 1978; 38: 498–502
- Davies CD, Lindmo T. Hyperthermia-induced shedding and masking of melanoma-associated antigen. Int J Hyperthermia 1990; 6: 1053–1064
- Schildkopf P, Holmer R, Sieber R, Ott OJ, Janko C, Mantel F, et al. Hyperthermia in combination with X-irradiation induces inflammatory forms of cell death. Autoimmunity 2009; 42: 311–313
- Shi H, Cao T, Connolly JE, Monnet L, Bennett L, Chapel S, et al. Hyperthermia enhances CTL cross-priming. J Immunol 2006; 176: 2134–2141
- Agarwal SS, Katz EJ, Loeb LA. Effect of hyperthermia on the survival of normal human peripheral blood mononuclear cells. Cancer Res 1983; 43: 3124–3126
- Johnston RL, Rao GR, Tompkins WA, Cain CA. Effects of in vivo ultrasound hyperthermia on natural killer cell cytotoxicity in the hamster. Bioelectromagnetics 1986; 7: 283–293
- Harada H, Murakami T, Tea SS, Takeuchi A, Koga T, Okada S, et al. Heat shock suppresses human NK cell cytotoxicity via regulation of perforin. Int J Hyperthermia 2007; 23: 657–665
- Roberts NJ, Jr. Differential effects of hyperthermia on human leukocyte production of interferon-alpha and interferon-gamma. Proc Soc Exp Biol Med 1986; 183: 42–47
- Borkamo ED, Dahl O, Bruland O, Fluge O. Kinetics study on markers of the immune system by gene expression profiling of an in vivo heated tumor. Int J Hyperthermia 2009; 25: 41–46
- Moore TC, Nur K, Storm FK. Localized deep hyperthermia increases the traffic of lymphocytes through peripheral lymph nodes of sheep in vivo. J Surg Oncol 1987; 35: 75–81
- Yoshioka A, Miyachi Y, Toda K, Imamura S, Hiraoka M, Abe M. Effects of local hyperthermia on natural killer activity in mice. Int J Hyperthermia 1990; 6: 261–267
- Mitsudo K, Kobayashi M, Tohnai I, Ueda M, Kotani H, Hoshino T. Electron-microscopic and immunohistochemical studies of Langerhans cells and Thy-1-positive cells in mouse tongue epithelium subjected to local hyperthermia. Arch Oral Biol 1995; 40: 533–538
- Bruggmoser G, Bauchowitz S, Canters R, Crezee H, Ehmann M, Gellermann J, et al. Quality assurance for clinical studies in regional deep hyperthermia. Strahlenther Onkol 2011; 187: 605–610
- Milani V, Noessner E. Effects of thermal stress on tumor antigenicity and recognition by immune effector cells. Cancer Immunol Immunother 2006; 55: 312–319
- Beachy SH, Repasky EA. Toward establishment of temperature thresholds for immunological impact of heat exposure in humans. Int J Hyperthermia 2011; 27: 344–352
- Sciandra JJ, Subjeck JR. Heat shock proteins and protection of proliferation and translation in mammalian cells. Cancer Res 1984; 44: 5188–5194
- Nylandsted J, Gyrd-Hansen M, Danielewicz A, Fehrenbacher N, Lademann U, Hoyer-Hansen M, et al. Heat shock protein 70 promotes cell survival by inhibiting lysosomal membrane permeabilization. J Exp Med 2004; 200: 425–435
- Jaattela M, Wissing D. Heat-shock proteins protect cells from monocyte cytotoxicity: Possible mechanism of self-protection. J Exp Med 1993; 177: 231–236
- Ciocca DR, Fanelli MA, Cuello-Carrion FD, Castro GN. Heat shock proteins in prostate cancer: From tumorigenesis to the clinic. Int J Hyperthermia 2010; 26: 737–747
- Baronzio G, Gramaglia A, Fiorentini G. Hyperthermia and immunity. A brief overview. In Vivo 2006; 20: 689–695
- Hannani D, Sistigu A, Kepp O, Galluzzi L, Kroemer G, Zitvogel L. Prerequisites for the antitumor vaccine-like effect of chemotherapy and radiotherapy. Cancer J 2011; 17: 351–358
- Srivastava PK. Purification of heat shock protein-peptide complexes for use in vaccination against cancers and intracellular pathogens. Methods 1997; 12: 165–171
- Hantschel M, Pfister K, Jordan A, Scholz R, Andreesen R, Schmitz G, et al. Hsp70 plasma membrane expression on primary tumor biopsy material and bone marrow of leukemic patients. Cell Stress Chaperones 2000; 5: 438–442
- Gehrmann M, Marienhagen J, Eichholtz-Wirth H, Fritz E, Ellwart J, Jaattela M, et al. Dual function of membrane-bound heat shock protein 70 (Hsp70), Bag-4, and Hsp40: Protection against radiation-induced effects and target structure for natural killer cells. Cell Death Differ 2005; 12: 38–51
- Multhoff G. Heat shock protein 72 (Hsp72), a hyperthermia-inducible immunogenic determinant on leukemic K562 and Ewing's sarcoma cells. Int J Hyperthermia 1997; 13: 39–48
- Hurwitz MD, Kaur P, Nagaraja GM, Bausero MA, Manola J, Asea A. Radiation therapy induces circulating serum Hsp72 in patients with prostate cancer. Radiother Oncol 2010; 95: 350–358
- Melcher A, Todryk S, Hardwick N, Ford M, Jacobson M, Vile RG. Tumor immunogenicity is determined by the mechanism of cell death via induction of heat shock protein expression. Nat Med 1998; 4: 581–587
- Binder RJ, Blachere NE, Srivastava PK. Heat shock protein-chaperoned peptides but not free peptides introduced into the cytosol are presented efficiently by major histocompatibility complex I molecules. J Biol Chem 2001; 276: 17163–17171
- Suto R, Srivastava PK. A mechanism for the specific immunogenicity of heat shock protein-chaperoned peptides. Science 1995; 269: 1585–1588
- Menoret A, Chaillot D, Callahan M, Jacquin C. Hsp70, an immunological actor playing with the intracellular self under oxidative stress. Int J Hyperthermia 2002; 18: 490–505
- Baler R, Zou J, Voellmy R. Evidence for a role of Hsp70 in the regulation of the heat shock response in mammalian cells. Cell Stress Chaperones 1996; 1: 33–39
- Calderwood SK, Theriault JR, Gong J. How is the immune response affected by hyperthermia and heat shock proteins?. Int J Hyperthermia 2005; 21: 713–716
- Calderwood SK, Theriault JR, Gong J. Message in a bottle: Role of the 70-kDa heat shock protein family in anti-tumor immunity. Eur J Immunol 2005; 35: 2518–2527
- Mambula SS, Calderwood SK. Heat induced release of Hsp70 from prostate carcinoma cells involves both active secretion and passive release from necrotic cells. Int J Hyperthermia 2006; 22: 575–585
- Milani V, Noessner E, Ghose S, Kuppner M, Ahrens B, Scharner A, et al. Heat shock protein 70: Role in antigen presentation and immune stimulation. Int J Hyperthermia 2002; 18: 563–575
- Gehrmann M, Liebisch G, Schmitz G, Anderson R, Steinem C, De Maio A, et al. Tumor-specific Hsp70 plasma membrane localization is enabled by the glycosphingolipid Gb3. PLoS One 2008; 3: e1925
- Frey B, Gaipl US. The immune functions of phosphatidylserine in membranes of dying cells and microvesicles. Semin Immunopathol 2011; 33: 497–516
- Elsner L, Muppala V, Gehrmann M, Lozano J, Malzahn D, Bickeboller H, et al. The heat shock protein Hsp70 promotes mouse NK cell activity against tumors that express inducible NKG2D ligands. J Immunol 2007; 179: 5523–5533
- Chen T, Guo J, Yang M, Zhu X, Cao X. Chemokine-containing exosomes are released from heat-stressed tumor cells via lipid raft-dependent pathway and act as efficient tumor vaccine. J Immunol 2011; 186: 2219–2228
- Multhoff G. Activation of natural killer cells by heat shock protein 70. 2002, 18: 576–585, Comment: Int J Hyperthermia 2009;25:169–175
- Torigoe T, Tamura Y, Sato N. Heat shock proteins and immunity: Application of hyperthermia for immunomodulation. Int J Hyperthermia 2009; 25: 610–616
- Polier S, Dragovic Z, Hartl FU, Bracher A. Structural basis for the cooperation of Hsp70 and Hsp110 chaperones in protein folding. Cell 2008; 133: 1068–1079
- Wang XY, Kazim L, Repasky EA, Subjeck JR. Characterization of heat shock protein 110 and glucose-regulated protein 170 as cancer vaccines and the effect of fever-range hyperthermia on vaccine activity. J Immunol 2001; 166: 490–497
- Di YP, Repasky EA, Subjeck JR. Distribution of Hsp70, protein kinase C, and spectrin is altered in lymphocytes during a fever-like hyperthermia exposure. J Cell Physiol 1997; 172: 44–54
- Knippertz I, Stein MF, Dorrie J, Schaft N, Muller I, Deinzer A, et al. Mild hyperthermia enhances human monocyte-derived dendritic cell functions and offers potential for applications in vaccination strategies. Int J Hyperthermia 2011; 27: 591–603
- Basu S, Srivastava PK. Fever-like temperature induces maturation of dendritic cells through induction of Hsp90. Int Immunol 2003; 15: 1053–1061
- Wang J, Koyama S, Komatsubara Y, Suzuki Y, Taki M, Miyakoshi J. Effects of a 2450 MHz high-frequency electromagnetic field with a wide range of SARs on the induction of heat-shock proteins in A172 cells. Bioelectromagnetics 2006; 27: 479–486
- Stangl S, Gross C, Pockley AG, Asea AA, Multhoff G. Influence of Hsp70 and HLA-E on the killing of leukemic blasts by cytokine/Hsp70 peptide-activated human natural killer (NK) cells. Cell Stress Chaperones 2008; 13: 221–230
- Gastpar R, Gehrmann M, Bausero MA, Asea A, Gross C, Schroeder JA, et al. Heat shock protein 70 surface-positive tumor exosomes stimulate migratory and cytolytic activity of natural killer cells. Cancer Res 2005; 65: 5238–5247
- Michaelsson J, Teixeira de Matos C, Achour A, Lanier LL, Karre K, Soderstrom K. A signal peptide derived from Hsp60 binds HLA-E and interferes with CD94/NKG2A recognition. J Exp Med 2002; 196: 1403–1414
- Qiao Y, Liu B, Li Z. Activation of NK cells by extracellular heat shock protein 70 through induction of NKG2D ligands on dendritic cells. Cancer Immun 2008; 8: 12
- van der Zee J. Heating the patient: A promising approach?. Ann Oncol 2002; 13: 1173–1184
- Song CW, Shakil A, Osborn JL, Iwata K. Tumour oxygenation is increased by hyperthermia at mild temperatures. 1996, 12: 367–373, Int J Hyperthermia 2009;25:91–95
- Iliakis G, Wu W, Wang M. DNA double strand break repair inhibition as a cause of heat radiosensitization: Re-evaluation considering backup pathways of NHEJ. Int J Hyperthermia 2008; 24: 17–29
- Krawczyk PM, Eppink B, Essers J, Stap J, Rodermond H, Odijk H, et al. Mild hyperthermia inhibits homologous recombination, induces BRCA2 degradation, and sensitizes cancer cells to poly (ADP-ribose) polymerase-1 inhibition. Proc Natl Acad Sci USA. 2011; 108: 9851–9856
- Tompkins WA, Rama Rao GV, Pantasatos P, Cain CA. Hyperthermia enhancement of antibody-complement cytotoxicity for human colon tumor cells. J Natl Cancer Inst 1981; 66: 453–459
- Mise K, Kan N, Okino T, Nakanishi M, Satoh K, Teramura Y, et al. Effect of heat treatment on tumor cells and antitumor effector cells. Cancer Res 1990; 50: 6199–6202
- Shelton SN, Dillard CD, Robertson JD. Activation of caspase-9, but not caspase-2 or caspase-8, is essential for heat-induced apoptosis in Jurkat cells. J Biol Chem 2010; 285: 40525–40533
- Mantel F, Frey B, Haslinger S, Schildkopf P, Sieber R, Ott OJ, et al. Combination of ionising irradiation and hyperthermia activates programmed apoptotic and necrotic cell death pathways in human colorectal carcinoma cells. Strahlenther Onkol 2010; 186: 587–599
- Kawai N, Ito A, Nakahara Y, Futakuchi M, Shirai T, Honda H, et al. Anticancer effect of hyperthermia on prostate cancer mediated by magnetite cationic liposomes and immune-response induction in transplanted syngeneic rats. Prostate 2005; 64: 373–381
- Muckle DS, Dickson JA. Hyperthermia (42 degrees C) as an adjuvant to radiotherapy and chemotherapy in the treatment of the allogeneic VX2 carcinoma in the rabbit. Br J Cancer 1973; 27: 307–315
- Muckle DS, Dickson JA. The selective inhibitory effect of hyperthermia on the metabolism and growth of malignant cells. Br J Cancer 1971; 25: 771–778
- Elkon D, Fechner RE, Homzie MJ, Baker DG, Constable WC. Response of mouse kidney to hyperthermia: Pathology and temperature-dependence of injury. Arch Pathol Lab Med 1980; 104: 153–158
- Ito A, Honda H, Kobayashi T. Cancer immunotherapy based on intracellular hyperthermia using magnetite nanoparticles: A novel concept of ‘heat-controlled necrosis’ with heat shock protein expression. Cancer Immunol Immunother 2006; 55: 320–328
- Tang D, Kang R, Zeh HJ, III, Lotze MT. High-mobility group box 1 and cancer. Biochim Biophys Acta 2010; 1799: 131–140
- Yerushalmi A, Weinstein Y. Stimulation of resistance to tumor growth of athymic nude mice pretreated by combined local hyperthermia and X-irradiation. Cancer Res 1979; 39: 1126–1128
- Roszkowski W, Wrembel JK, Roszkowski K, Janiak M, Szmigielski S. Does whole-body hyperthermia therapy involve participation of the immune system?. Int J Cancer 1980; 25: 289–292
- Robins HI. Role of whole-body hyperthermia in the treatment of neoplastic disease: Its current status and future prospects. Cancer Res 1984; 44: S4878–4883
- Gridley DS, Nutter RL, Kettering JD, Mantik DW, Slater JM. Mouse neoplasia and immunity: Effects of radiation, hyperthermia, 2-deoxy-D-glucose, and Corynebacterium parvum. Oncology 1985; 42: 391–398
- Issels RD. Hyperthermia adds to chemotherapy. Eur J Cancer 2008; 44: 2546–2554
- Shen RN, Lu L, Wu B, Shidnia H, Hornback NB, Broxmeyer HE. Effects of interleukin 2 treatment combined with local hyperthermia in mice inoculated with Lewis lung carcinoma cells. Cancer Res 1990; 50: 5027–5030
- Wu B, Shen RN, Wang WX, Broxmeyer HE, Lu L. Antitumor effect of interleukin 7 in combination with local hyperthermia in mice bearing B16a melanoma cells. Stem Cells 1993; 11: 412–421
- Geehan DM, Fabian DF, Lefor AT. Local hyperthermia abrogates the anti-immunotherapeutic effect of interleukin-8. J Surg Oncol 1994; 56: 102–106
- Klostergaard J, Barta M, Tomasovic SP. Hyperthermic modulation of tumor necrosis factor-dependent monocyte/macrophage tumor cytotoxicity in vitro. J Biol Response Mod 1989; 8: 262–277
- Tomasovic SP, Barta M, Klostergaard J. Temporal dependence of hyperthermic augmentation of macrophage-TNF production and tumor cell-TNF sensitization. Int J Hyperthermia 1989; 5: 625–639
- Srinivasan JM, Fajardo LF, Hahn GM. Mechanism of antitumor activity of tumor necrosis factor alpha with hyperthermia in a tumor necrosis factor alpha-resistant tumor. J Natl Cancer Inst 1990; 82: 1904–1910
- Apetoh L, Ghiringhelli F, Tesniere A, Obeid M, Ortiz C, Criollo A, et al. Toll-like receptor 4-dependent contribution of the immune system to anticancer chemotherapy and radiotherapy. Nat Med 2007; 13: 1050–1059
- Miller RC, Roizin-Towle L, Komatsu K, Richards M, Hall EJ. Interaction of heat with X-rays and cis-platinum: Cell lethality and oncogenic transformation. Int J Hyperthermia 1989; 5: 697–705
- Baker D, Sager H, Constable W. The influence of levamisole and hyperthermia on the incidence of metastases from an X-irradiated tumor. Cancer Invest 1986; 4: 287–292
- Morita M, Kuwano H, Araki K, Egashira A, Kawaguchi H, Saeki H, et al. Prognostic significance of lymphocyte infiltration following preoperative chemoradiotherapy and hyperthermia for esophageal cancer. Int J Radiat Oncol Biol Phys 2001; 49: 1259–1266
- Guo J, Zhu J, Sheng X, Wang X, Qu L, Han Y, et al. Intratumoral injection of dendritic cells in combination with local hyperthermia induces systemic antitumor effect in patients with advanced melanoma. Int J Cancer 2007; 120: 2418–2425
- Mukhopadhaya A, Mendecki J, Dong X, Liu L, Kalnicki S, Garg M, et al. Localized hyperthermia combined with intratumoral dendritic cells induces systemic antitumor immunity. Cancer Res 2007; 67: 7798–7806
- Tanaka K, Ito A, Kobayashi T, Kawamura T, Shimada S, Matsumoto K, et al. Intratumoral injection of immature dendritic cells enhances antitumor effect of hyperthermia using magnetic nanoparticles. Int J Cancer 2005; 116: 624–633
- Bachleitner-Hofmann T, Strohschneider M, Krieger P, Sachet M, Dubsky P, Hayden H, et al. Heat shock treatment of tumor lysate-pulsed dendritic cells enhances their capacity to elicit antitumor T cell responses against medullary thyroid carcinoma. J Clin Endocrinol Metab 2006; 91: 4571–4577
- Demaria S, Ng B, Devitt ML, Babb JS, Kawashima N, Liebes L, et al. Ionizing radiation inhibition of distant untreated tumors (abscopal effect) is immune mediated. Int J Radiat Oncol Biol Phys 2004; 58: 862–870
- Chakravarty PK, Guha C, Alfieri A, Beri V, Niazova Z, Deb NJ, et al. Flt3L therapy following localized tumor irradiation generates long-term protective immune response in metastatic lung cancer: Its implication in designing a vaccination strategy. Oncology 2006; 70: 245–254
- Frey B, Rubner Y, Wunderlich R, Weiss EM, Pockley AG, Fietkau R, et al. Induction of abscopal anti-tumor immunity and immunogenic tumor cell death by ionizing irradiation – Implications for cancer therapies. Curr Med Chem 2012; 19: 1751–1764
- Li X, Gao XH, Jin L, Wang Y, Hong Y, McHepange UO, et al. Local hyperthermia could induce migrational maturation of Langerhans cells in condyloma acuminatum. J Dermatol Sci 2009; 54: 121–123
- Chen T, Guo J, Han C, Yang M, Cao X. Heat shock protein 70, released from heat-stressed tumor cells, initiates antitumor immunity by inducing tumor cell chemokine production and activating dendritic cells via TLR4 pathway. J Immunol 2009; 182: 1449–1459
- Kim JY, Son YO, Park SW, Bae JH, Chung JS, Kim HH, et al. Increase of NKG2D ligands and sensitivity to NK cell-mediated cytotoxicity of tumor cells by heat shock and ionizing radiation. Exp Mol Med 2006; 38: 474–484
- Kubes J, Svoboda J, Rosina J, Starec M, Fiserova A. Immunological response in the mouse melanoma model after local hyperthermia. Physiol Res 2008; 57: 459–465
- Stehlin JS, Giovanella BC, de Ipolyi PD, Muenz LR, Anderson RF. Results of hyperthermic perfusion for melanoma of the extremities. Surg Gynecol Obstet 1975; 140: 339–348
- Nakayama J, Moroi Y, Toshitani A, Taniguchi S, Okamoto-Inoue M, Hori Y. Responses of B16 melanoma cell lines, F1 and F10, to hyperthermia, lymphokine-activated killer cells and a combination of both in vitro. Br J Dermatol 1992; 126: 131–136
- Nakayama J, Urabe A, Terao H, Taniguchi S, Hori Y. In situ detection of immunocompetent cells in murine B16 melanoma locally treated with interleukin-2 or microwaval hyperthermia. Pigment Cell Res 1993; 6: 111–116
- Nakayama J, Toyofuku K, Urabe A, Taniguchi S, Hori Y. A combined therapeutic modality with hyperthermia and locally administered rIFN-beta inhibited the growth of B16 melanoma in association with the modulation of cellular infiltrates. J Dermatol Sci 1993; 6: 240–246
- Strauch ED, Fabian DF, Turner J, Lefor AT. Combined hyperthermia and immunotherapy treatment of multiple pulmonary metastases in mice. Surg Oncol 1994; 3: 45–52
- Frey B, Schildkopf P, Rodel F, Weiss EM, Munoz LE, Herrmann M, et al. AnnexinA5 renders dead tumor cells immunogenic-implications for multimodal cancer therapies. J Immunotoxicol 2009; 6: 209–216
- Zhang HG, Mehta K, Cohen P, Guha C. Hyperthermia on immune regulation: A temperature's story. Cancer Lett 2008; 271: 191–204
- Issels RD, Lindner LH, Verweij J, Wust P, Reichardt P, Schem BC, et al. Neo-adjuvant chemotherapy alone or with regional hyperthermia for localised high-risk soft-tissue sarcoma: A randomised phase 3 multicentre study. Lancet Oncol 2010; 11: 561–570
- Szmigielski S, Sobczynski J, Sokolska G, Stawarz B, Zielinski H, Petrovich Z. Effects of local prostatic hyperthermia on human NK and T cell function. Int J Hyperthermia 1991; 7: 869–880
- Ostapenko VV, Tanaka H, Miyano M, Nishide T, Ueda H, Nishide I, et al. Immune-related effects of local hyperthermia in patients with primary liver cancer. Hepatogastroenterology 2005; 52: 1502–1506
- Atanackovic D, Nierhaus A, Neumeier M, Hossfeld DK, Hegewisch-Becker S. 41.8 degrees C whole body hyperthermia as an adjunct to chemotherapy induces prolonged T cell activation in patients with various malignant diseases. Cancer Immunol Immunother 2002; 51: 603–613
- Matsumoto K, Yamamoto N, Hagiwara S, Saito M, Furue H, Shigetomi T, et al. Optimization of hyperthermia and dendritic cell immunotherapy for squamous cell carcinoma. Oncol Rep 2011; 25: 1525–1532
- van der Zee J, Gonzalez GD. The Dutch Deep Hyperthermia Trial: Results in cervical cancer. Int J Hyperthermia 2002; 18: 1–12
- Belli F, Testori A, Rivoltini L, Maio M, Andreola G, Sertoli MR, et al. Vaccination of metastatic melanoma patients with autologous tumor-derived heat shock protein gp96-peptide complexes: Clinical and immunologic findings. J Clin Oncol 2002; 20: 4169–4180
- Li Z, Qiao Y, Liu B, Laska EJ, Chakravarthi P, Kulko JM, et al. Combination of imatinib mesylate with autologous leukocyte-derived heat shock protein and chronic myelogenous leukemia. Clin Cancer Res 2005; 11: 4460–4468
- Srivastava PK, Callahan MK, Mauri MM. Treating human cancers with heat shock protein-peptide complexes: The road ahead. Expert Opin Biol Ther 2009; 9: 179–186
- Testori A, Richards J, Whitman E, Mann GB, Lutzky J, Camacho L, et al. Phase III comparison of vitespen, an autologous tumor-derived heat shock protein gp96 peptide complex vaccine, with physician's choice of treatment for stage IV melanoma: The C-100-21 Study Group. J Clin Oncol 2008; 26: 955–962
- Hilf N, Singh-Jasuja H, Schild H. The heat shock protein Gp96 links innate and specific immunity. Int J Hyperthermia 2002; 18: 521–533
- Wood C, Srivastava P, Bukowski R, Lacombe L, Gorelov AI, Gorelov S, et al. An adjuvant autologous therapeutic vaccine (HSPPC-96; vitespen) versus observation alone for patients at high risk of recurrence after nephrectomy for renal cell carcinoma: A multicentre, open-label, randomised phase III trial. Lancet 2008; 372: 145–154
- Srivastava PK, Old LJ. Individually distinct transplantation antigens of chemically induced mouse tumors. Immunol Today 1988; 9: 78–83