Abstract
Purpose: To evaluate the usefulness of combined treatment with continuous administration of a hypoxic cytotoxin, tirapazamine (TPZ), and mild temperature hyperthermia (MTH) in γ-ray irradiation in terms of local tumour response and lung metastatic potential, referring to the response of intratumour quiescent (Q) cells.
Materials and methods: B16-BL6 melanoma tumour-bearing C57BL/6 mice were continuously given 5-bromo-2′-deoxyuridine (BrdU) to label all proliferating (P) cells. The tumour-bearing mice then received γ-ray irradiation after a single intraperitoneal injection or 24 h continuous subcutaneous infusion of TPZ, either with or without MTH. Immediately after the irradiation, cells from some tumours were isolated and incubated with a cytokinesis blocker. The responses of the Q and total (= P + Q) cell populations were assessed based on the frequency of micronuclei using immunofluorescence staining for BrdU. In other tumour-bearing mice, 17 days after irradiation, macroscopic lung metastases were enumerated.
Results: Continuous administration elevated the sensitivity of both the total and Q cells, especially the total cells. MTH raised the sensitivity of Q cells more remarkably in both single and continuous administrations, probably because of more exposure to TPZ in intermediately hypoxic areas derived mainly from chronic hypoxia through MTH. With or without irradiation, TPZ, especially administered continuously and combined with MTH, decreased the number of lung metastases.
Conclusion: The combination of continuous long-term administration of TPZ and MTH in γ-ray irradiation was thought to be promising because of its potential to enhance local tumour response and repress lung metastatic potential.
Introduction
Many of the cancer cells in solid tumours are non-proliferating (quiescent), and many of the features of quiescent (Q) cells are still unknown Citation[1]. To improve cancer treatment, the response of Q cells to anticancer treatment should be determined, since often tumour cells that are quiescent in situ are still clonogenic Citation[1].
The development of bioreductive agents that are particularly toxic to hypoxic cells is considered a promising approach to solving the problem of radio-resistant tumour hypoxia in cancer radiotherapy Citation[2]. Tirapazamine (TPZ), a leading compound in the development of bioreductive hypoxic cytotoxins, in combination with radiation has been shown to be very useful for controlling solid tumours as a whole, especially for controlling Q tumour cell populations that are rich in hypoxic region Citation[2], Citation[3]. Tumour hypoxia results from either limited oxygen diffusion (chronic hypoxia) or limited perfusion (acute hypoxia, transient hypoxia or ischaemic hypoxia). Chronically hypoxic tumour cells existing at the rim of the oxygen diffusion distance can be killed by just a single administration of TPZ Citation[3]. Acutely hypoxic tumour cells occurring sporadically throughout solid tumours can be killed by TPZ during long-term continuous administration. Namely, the long-term continuous administration of TPZ can kill both chronically and acutely hypoxic tumour cells Citation[4].
Mild temperature hyperthermia (MTH) was reported to increase the response of tumours to radiation by improving oxygenation through an increase in tumour blood flow Citation[5]. Further, MTH was also shown to enhance the tumour response, especially of the intratumour Q cell population, to TPZ Citation[6].
Metastasis is a leading cause of cancer deaths and involves a complex, multistep process by which tumour cells disseminate to distant sites to establish discontinuous secondary colonies Citation[7], Citation[8]. It was reported that acute and cyclic, but not chronic, hypoxia significantly increased the number of spontaneous lung metastases in mice by a factor of about 2, and that this effect was due to the influence of the acute hypoxia treatment on the primary tumour and not to other potential effects of the treatment such as damage to the lung epithelium Citation[9], Citation[10]. Based on this report, we recently reported the significance of injections of an acute hypoxia-releasing agent, nicotinamide, into tumour-bearing mice as a combined treatment with high dose rate γ-ray irradiation in terms of reducing the number of lung metastatic nodules Citation[11].
Here, using a readily metastasising murine melanoma cell line, we tried to analyse the usefulness of combined treatment with continuous long-term administration of TPZ and MTH in radiotherapy with γ-rays in terms of local tumour response and lung metastatic potential. Concerning the local tumour response, the effect not only on the total (= proliferating (P) + Q) tumour cell population but also on the Q cell population was evaluated using our original method for selectively detecting the response of Q cells in solid tumours Citation[3].
Materials and methods
Mice and tumours
B16-BL6 murine melanoma cells (Institute of Development, Aging and Cancer, Tohoku University) derived from C57BL/6 mice were maintained in vitro in RPMI-1640 medium supplemented with 10% foetal bovine serum. Tumour cells (1.25 × 105) were inoculated subcutaneously into the left hind leg of 8-week-old syngeneic female C57BL/6 mice (Japan Animal Company, Osaka, Japan). Eighteen days later, the tumours, approximately 7 mm in diameter, were employed for γ-ray irradiation in this study, and the body weight of the tumour-bearing mice was 20.1 ± 2.3 g (mean ± standard error). Mice were handled according to the Recommendations for Handling of Laboratory Animals for Biomedical Research, compiled by the Committee on Safety Handling Regulations for Laboratory Animal Experiments at our university. The p53 of B16-BL6 tumour cells is the wild type Citation[12].
Labelling with 5-bromo-2′-deoxyuridine (BrdU)
Twelve days after the inoculation, mini-osmotic pumps (Durect Corporation, Cupertino, CA) containing 5-bromo-2′-deoxyuridine (BrdU) dissolved in physiological saline (250 mg/mL) were implanted subcutaneously into the animals’ backs for 6 days to label all P cells. The percentage of labelled cells after continuous labelling with BrdU was 54.3 ± 6.1%, and reached a plateau at this stage. Therefore, tumour cells not incorporating BrdU after continuous labelling were regarded as Q cells.
Treatment
After the labelling with BrdU, TPZ dissolved in physiological saline was administered at a dose of 224 µM/kg (40 mg/kg) singly by intra-peritoneal injection or continuously for 24 h by subcutaneously implanting mini-osmotic pumps (Durect) containing TPZ dissolved in physiological saline in the backs of the mice. Right after the intra-peritoneal injection or during the last hour of the 24 h of continuous subcutaneous infusion, the tumours grown in the left hind legs of mice were heated at 40°C for 60 min by immersing the tumour-bearing foot in a water bath. Right after the heating, solid tumours grown in the left hind legs of mice were irradiated with a cobalt-60 γ-ray irradiator.
Concerning MTH, the tumours grown in the left hind legs of mice were heated at 40°C for 60 min by immersing the tumour-bearing foot in a water bath. The mouse was held in a specially constructed device with the tail and right leg firmly fixed with an adhesive tape. The left tumour-bearing leg was pulled down by a special sinker (approximately 45 g) which was affixed to the skin of the paw with superglue (Arone-arufa, Konishi, Co., Osaka, Japan). The mice were then placed on a circulating water bath maintained at the desired temperature. The mice were air-cooled during the heat treatment Citation[13]. Temperatures at the tumour centre equilibrated within 3 to 4 min after immersion in the water bath and remained 0.2–0.3°C below the bath's temperature. The water bath's temperature was maintained at 0.3°C above the desired tumour temperature.
γ-Ray irradiation was performed with a cobalt-60 γ-ray irradiator at a dose rate of 2.75 Gy/min as conventionally employed for high dose-rate irradiation, with the tumour-bearing mice held in a specially constructed device with the tail firmly fixed with an adhesive tape. Lead blocks were used to avoid irradiating body parts other than the tumour-bearing left hind leg.
Each irradiation group also included mice that were not pretreated with BrdU.
Immunofluorescence staining of BrdU-labelled cells and micronucleus (MN) assay
Immediately after irradiation, the tumours excised from the mice given BrdU were minced and trypsinised (0.05% trypsin and 0.02% ethylenediaminetetraacetic acid (EDTA) in phosphate-buffered saline [PBS], 37°C, 15 min). Tumour cell suspensions were incubated for 72 h in tissue culture dishes containing complete medium and 1.0 µg/mL of cytochalasin-B to inhibit cytokinesis while allowing nuclear division. The cultures were trypsinised, and cell suspensions were fixed and resuspended with cold Carnoy's fixative (ethanol:acetic acid = 3:1 in volume). Each suspension was placed on a glass microscope slide, dried at room temperature and treated with 2 M hydrochloric acid for 60 min at room temperature to dissociate the histones and partially denature the DNA. The slides were immersed in borax-borate buffer (pH 8.5) to neutralise the acid. BrdU-labelled tumour cells were detected by indirect immunofluorescence staining using a monoclonal anti-BrdU antibody (Becton Dickinson, San Jose, CA) and a fluorescein isothiocyanate (FITC)-conjugated antimouse IgG antibody (Sigma, St Louis, MO). To distinguish the tumour cells stained with green-emitting FITC and observe them separately, cells on the slides were treated with red-emitting propidium iodide (PI, 2 µg/mL in PBS) as a background staining, and were monitored under a fluorescence microscope.
When cell division is disrupted, or the chromosomes are broken or damaged by chemicals or radiation, the distribution of genetic material between the two daughter nuclei during cell division is affected and pieces or entire chromosomes fail to be included in either of the daughter nuclei. The genetic material that is not incorporated into a new nucleus forms a ‘micronucleus’. Thus, the frequency with which micronuclei form (MN frequency) reflects the genotoxicity of a chemical compound and radiation very well. The MN frequency in cells not labelled with BrdU could be examined by counting the micronuclei in the binuclear cells that showed only red fluorescence. The MN frequency was defined as the ratio of the number of micronuclei in the binuclear cells to the total number of binuclear cells observed Citation[3].
The ratios obtained in tumours not pretreated with BrdU indicated the MN frequency at all phases in the total tumour cell population. More than 300 binuclear cells were counted to determine the MN frequency.
Clonogenic cell survival assay
The clonogenic cell survival assay was also performed for tumours implanted in mice given no BrdU using an in vivo-in vitro assay method immediately after irradiation. The tumours not labelled with BrdU were excised, weighed, minced, and disaggregated by stirring for 20 min at 37°C in PBS containing 0.05% trypsin and 0.02% EDTA. The cell yield was 1.2 ± 0.4 × 107/g of tumour weight. Appropriate numbers of viable tumour cells from the single cell suspension were plated on 60 or 100-mm tissue culture dishes, and, 12 days later, colonies were fixed with ethanol, stained with Giemsa, and counted. The cut-off cell number to be considered a colony was 50. For the tumours that received no irradiation, the surviving fractions (SFs) for the total tumour cell populations and the MN frequencies for the total and Q cell populations are shown in . The fraction of cells surviving a given radiation dose is determined by counting the number of macroscopic colonies as a fraction of the number of cells seeded, followed by allowance, that is, dividing by the fraction of cells surviving no radiation dose.
Table I. Surviving fractions and micronucleus frequencies at 0 Gy.
As stated above, the MN frequencies for Q cells were obtained from BrdU-unlabelled cells in tumours after continuous BrdU labelling in vivo. The MN frequencies and SFs for total tumour cell populations were obtained from cells in tumours not pretreated with BrdU. Thus, we could not detect any interaction between BrdU and irradiation in our data for the MN frequency and SF.
Growth of B16-BL6 tumours
After γ-ray irradiation at an absorbed dose of 0 or 16 Gy with or without TPZ in combination with MTH on day 18 after inoculation, the size of the tumours implanted in the left hind legs of some tumour-bearing mice was checked two to three times a week for about 20 days. Tumour volume was calculated using the formula V = π/6 × a × b2, where a and b are respectively the longest and shortest diameters of the tumour measured with calipers.
Metastasis assessment
Seventeen days after irradiation (= 35 days after the inoculation of B16-BL6 melanoma cells), the tumour-bearing mice were sacrificed by cervical dislocation, and their lungs were removed, briefly washed with distilled water, cleaned of extraneous tissue, fixed in Bouin's solution overnight (Sigma), and stored in buffered formalin 10% (Sigma) until metastases were counted. Macroscopically visible metastases were counted under a dissection microscope Citation[14]. Eighteen days after the inoculation and immediately before exposure to γ-rays, macroscopic lung metastases were also counted as background data. The average number was 5.2 ± 1.6.
Data analysis and statistics
Three mice with a tumour in the left hind leg were used to assess each set of conditions, and each experiment was repeated three times. Namely, nine mice were used for each set of conditions. To examine the differences between pairs of values, Student's t-test was used when variances of the two groups could be assumed to be equal with Shapiro-Wilk normality test; otherwise the Welch t-test was used; p values are from two-sided tests. The data on cell survival and MN frequencies were fitted to the linear quadratic dose relationship Citation[15].
Results
shows the SFs for the total tumour cell population and the MN frequencies without irradiation for the total and Q cell populations. TPZ treatment resulted in significantly lower SFs and higher MN frequencies in both the total and Q cell populations under each set of conditions. In addition, the continuous administration of TPZ produced significantly lower SFs and higher MN frequencies than a single intraperitoneal administration in the two cell populations under all conditions. Further, addition of MTH to the TPZ treatment led to lower SFs and higher MN frequencies in both cell populations under all conditions. On the other hand, the Q cell population showed significantly higher MN frequencies than the total cell population under each set of conditions.
shows cell survival curves for the total cell population as a function of the absorbed dose of γ-rays with or without TPZ in combination with MTH. shows net MN frequencies as a function of irradiated absorbed dose with or without TPZ in combination with MTH in the total and Q tumour cell populations. The net MN frequency was the MN frequency in tumours that received irradiation minus that in tumours that did not. Overall, the net MN frequencies were significantly smaller in Q cells than the total cell population.
Figure 1. Cell survival curves for the total cell population from B16-BL6 tumours irradiated with γ-rays following the single intraperitoneal (i.p.) or continuous subcutaneous (cont.) administration of tirapazamine (TPZ) in combination with mild temperature hyperthermia (MTH) on day 18 after tumour cell inoculation. ○ γ-ray irradiation only; Δ γ-ray irradiation after single intraperitoneal administration of TPZ; ▴ γ-ray irradiation after single intraperitoneal administration of TPZ with MTH; □ γ-ray irradiation after continuous subcutaneous administration of TPZ; ▪ γ-ray irradiation after continuous subcutaneous administration of TPZ with MTH. Bars represent standard errors (n = 9).
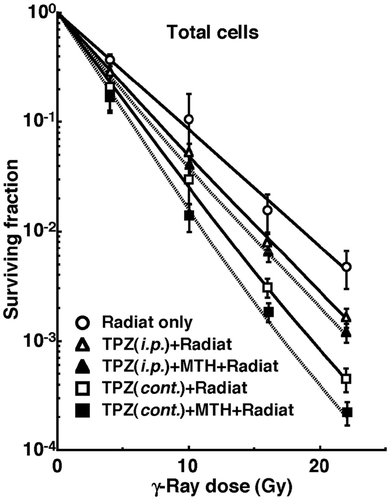
Figure 2. Dose response curves of the net micronucleus frequency for total (left panel) and quiescent (right panel) cell populations from B16-BL6 tumours irradiated with γ-rays following the single intraperitoneal (i.p.) or continuous subcutaneous (cont.) administration of tirapazamine (TPZ) in combination with mild temperature hyperthermia (MTH) on day 18 after tumour cell inoculation. ○ γ-ray irradiation only; △ γ-ray irradiation after single intraperitoneal administration of TPZ; ▴ γ-ray irradiation after single intraperitoneal administration of TPZ with MTH; □ γ-ray irradiation after continuous subcutaneous administration of TPZ; ▪ γ-ray irradiation after continuous subcutaneous administration of TPZ with MTH. Bars represent standard errors (n = 9).
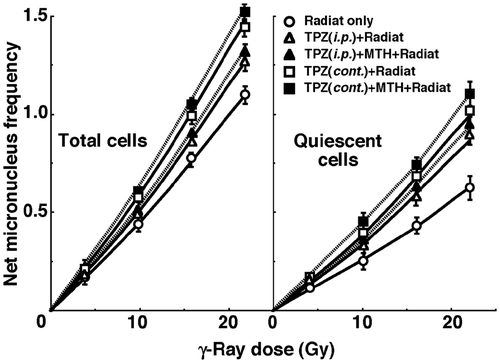
To estimate the radio-enhancing effect of TPZ, irradiation with TPZ in both the total and Q cell populations was compared with irradiation only, using the data obtained without MTH shown in and (). The radio-enhancing effect of TPZ was significantly larger than 1.0, and TPZ enhanced the sensitivity of the Q cell population significantly more than that of the total cell population. Further, continuous administration increased the sensitivity of both cell populations significantly more than single intraperitoneal administration.
Table II. The effect* of tirapazamine on each end point.
To estimate the radio-enhancing effect of combined treatment with MTH in both the total and Q cell populations, the data shown in and were used (). Whether TPZ was administered singly or continuously, the sensitivity of the Q cell population seemed to be slightly more enhanced with MTH than with that of the total cell population, although there were no significant differences. There were also no significant differences in the radio-enhancing effects between single and continuous administration of TPZ.
Table III. The effect* of mild temperature hyperthermia on each end point.
To examine the difference in radiosensitivity between the total and Q cell populations, dose-modifying factors were calculated using the data in and (). Overall, all the values were significantly larger than 1.0. In combination with TPZ, the difference was significantly decreased. In further combination with MTH, the difference in radiosensitivity seemed to be smaller, although there were no significant differences. There were also no significant differences in the values between single and continuous administration of TPZ.
Table IV. Dose-modifying factors for quiescent cells relative to the total cell population.*
shows tumour growth curves after γ-ray irradiation with or without TPZ administration in combination with MTH 18 days after the tumour cell inoculation. To evaluate tumour growth, the period required for each tumour to become twice as large as on day 18 was obtained using the data shown in (). Without irradiation, the period required for TPZ combination was significantly prolonged with, as compared to without, TPZ. Continuous administration further lengthened the period with significant differences, with or without MTH. Whether TPZ was administered singly or continuously, MTH seemed to prolong the period without any significant differences. With irradiation at an absorbed dose of 16 Gy, overall, the period required was significantly prolonged compared with no irradiation, and the treatments ranked in the following order; without TPZ < with singly administered TPZ < with continuously administered TPZ, with or without MTH. Again, whether TPZ was administered singly or continuously, MTH seemed to prolong the period without any significant differences.
Figure 3. Tumour growth curves for B16-BL6 tumours with (solid lines) or without (dotted lines) γ-ray irradiation at a dose of 16 Gy following the single intraperitoneal (i.p.) or continuous subcutaneous (cont.) administration of tirapazamine (TPZ) in combination with mild temperature hyperthermia (MTH) on day 18 after tumour cell inoculation. ○ γ-ray irradiation only; △ γ-ray irradiation aftersingle intraperitoneal administration of TPZ; ▴ γ-ray irradiation after single intraperitoneal administration of TPZ with MTH; □ γ-ray irradiation after continuous subcutaneous administration of TPZ; ▪ γ-ray irradiation after continuous subcutaneous administration of TPZ with MTH. Bars represent standard errors (n = 9).
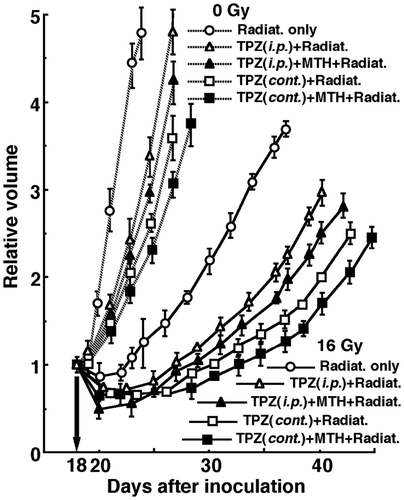
Table V. The period (days) required for each tumour to become twice as large as on day 18 after tumour cell inoculation.
shows the numbers of lung metastases on day 35 after inoculation as a function of the absorbed dose of γ-ray irradiation with or without TPZ administration in combination with MTH. Without irradiation, TPZ, especially when administered continuously, seemed to decrease the numbers of macroscopic metastases. Whether TPZ was administered singly or continuously, MTH seemed to further decrease the number. With irradiation, as the absorbed dose increased, the numbers decreased. Further, the numbers decreased more remarkably with TPZ than without. Again, especially when TPZ was administered continuously, the numbers decreased further, and MTH seemed to cause a greater decrease. With or without MTH, the curves for the tumours treated by radiation with TPZ were slightly steeper than those for the tumours treated by radiation only. However, there were no significant differences between the slopes of the curves for tumours treated by radiation with TPZ and those for tumours treated by radiation only. Consequently, this means there was no apparent radiosensitising effect of TPZ on repressing lung metastases from treated tumours. Meanwhile, there was an almost parallel shift in the curves and no apparent changes in the slopes of the curves for the tumours treated with TPZ with or without MTH. This means there was no apparent difference in the radiosensitising or protecting effect between single intraperitoneal and continuous subcutaneous administration with or without MTH in terms of the numbers of lung metastases. With or without irradiation, continuous administration of TPZ combined with MTH, which was the most cytotoxic as an initial effect, seemed to reduce the numbers of lung metastases from the local tumours the most efficiently.
Figure 4. Counted numbers of macroscopic metastases in the lung on day 35 after tumour cell inoculation as a function of the dose of γ-ray irradiation following the single intraperitoneal (i.p.) or continuous subcutaneous (cont.) administration of tirapazamine (TPZ) in combination with mild temperature hyperthermia (MTH) on day 18 after tumour cell inoculation. ○ γ-ray irradiation only; △ γ-ray irradiation after single intraperitoneal administration of TPZ; ▴ γ-ray irradiation after single intraperitoneal administration of TPZ with MTH; □ γ-ray irradiation after continuous subcutaneous administration of TPZ; ▪ γ-ray irradiation after continuous subcutaneous administration of TPZ with MTH. Bars represent standard errors (n = 9).
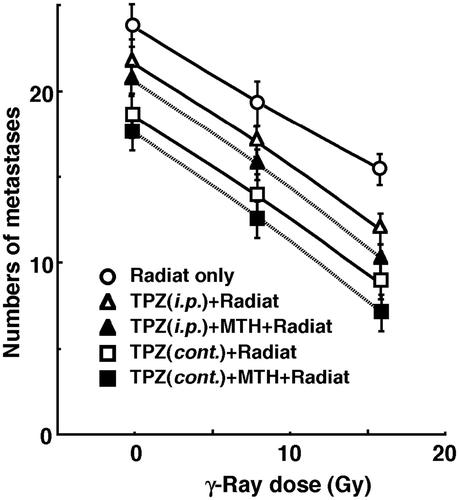
The numbers of lung metastases from local tumours that received irradiation under each set of conditions, which produced an identical SF of 0.03 as an initial effect (), were estimated using the data shown in (). Overall, the combination with TPZ tended to decrease the numbers more than γ-ray irradiation only. Especially when TPZ was administered continuously, the number was significantly smaller than that for γ-ray irradiation only. Further combination of MTH with TPZ resulted in a slightly smaller number than without MTH, although not significantly. Thus, when TPZ was also administered continuously combined with MTH, the number was significantly smaller than that for γ-ray irradiation only.
Table VI. The numbers of metastases from the irradiated tumours that received cytotoxic treatment producing a similar initial local effect.*
Discussion
Tumour microvasculatures frequently have structural and functional abnormalities, such as a disorganised vascular network, dilations, an elongated and tortuous shape, an incomplete endothelial lining, a lack of physiological/pharmacological receptors, an absence of flow regulation, and intermittent stasis Citation[1], Citation[16]. Perfusion-related O2 delivery leads to ischaemic hypoxia, which is often transient. Thus, perfusion-limited acute hypoxic areas are distributed throughout the tumour depending on these causative factors Citation[1], Citation[16], Citation[17]. Diffusion-related chronic hypoxia is caused by an increase in diffusion distances with tumour expansion. This results in an inadequate O2 supply for cells distant (>70 µm) from the nutritive blood vessels. Diffusion-limited hypoxia may also be caused by a deterioration of diffusion ‘geometry’, for example, concurrent vs. countercurrent blood flow within the tumour microvessel network Citation[1], Citation[16], Citation[17]. MTH before irradiation decreased the hypoxic fraction (HF), even combined with nicotinamide treatment that prevents transient fluctuations in tumour blood flow that lead to the development of acute hypoxia Citation[18], Citation[19]. In contrast, MTH did not decrease the HF when tumour-bearing mice were placed in a circulating carbogen (95% O2/5% CO2) chamber during irradiation Citation[20]. Thus, MTH was shown to increase the tumour response to radiation by improving tumour oxygenation through an increase in tumour blood flow, thereby preferentially overcoming chronic hypoxia rather than acute hypoxia Citation[20].
In this study, the enhancing effect of the hypoxic cytotoxin TPZ with or without MTH was more remarkable in Q cells than in the total cell population () mainly because of the much larger size of the hypoxic fraction (HF) in the Q cell population Citation[3], Citation[11]. When administered continuously, TPZ can produce DNA breaks in larger areas of solid tumours where not only chronic hypoxia exists but also acute hypoxia has occurred, whereas a single administration mainly kills chronically hypoxic tumour cells Citation[4]. In the previous study using theoretical predictions Citation[21], it was also shown that fluctuating pO2 increases the efficacy of TPZ, and that the longer time frame of TPZ delivery causes slow fluctuations in pO2, resulting in increasing the efficacy of TPZ.
According to our previous report Citation[11], in the B16-BL6 tumour, the HF of the total cell population includes a large acutely HF and small chronically HF. In contrast, the HF of Q cells is made up of a large chronically HF and small acutely HF. Consequently, when MTH was combined with TPZ before irradiation, more TPZ could be exposed to intermediately hypoxic areas derived mainly from chronic hypoxia in solid tumours through the increase in tumour blood flow induced by MTH Citation[4], resulting in greater enhancing effects preferentially on chronic hypoxia-rich Q cell fractions. Anyway, the use of TPZ, especially in combination with MTH, is very useful for enhancing the sensitivity of tumour cells, especially of radio-resistant Q tumour cells.
Meanwhile, tumour growth as a whole showed a close correlation with the initial response of the total cell population in a tumour. Thus, it is reasonable that the changes in tumour growth as a whole (, ) were consistent with and well supported the changes in the radiosensitivity of total tumour cell populations in cell survival curves () and dose-response curves of the net MN frequency ().
The presence of Q cells is probably due, at least in part, to hypoxia and the depletion of nutrition, a consequence of poor vascular supply Citation[1], Citation[16]. As a result, Q cells are viable and clonogenic, but have ceased dividing. This might promote the formation of micronuclei at 0 Gy in Q tumour cells (). Q cells were shown to have significantly less radiosensitivity than the total cell population Citation[1], Citation[3], Citation[16], that is, more Q cells survive radiotherapy than P cells (, ). Thus, the control of chronic hypoxia-rich Q cells has a great impact on the outcome of conventional radiotherapy for controlling local tumours, resulting in the superiority of the combination of TPZ and MTH in radiotherapy. As a result, the combined use of TPZ and MTH led to a decrease in the difference in radiosensitivity (). After all, the use of TPZ as a hypoxic cytotoxin in combination with MTH is thought to be very promising in terms of local tumour response in conventional radiotherapy.
Hypoxia is suggested to enhance metastasis by increasing genetic instability Citation[10]. Acute but not chronic hypoxia increased the number of macroscopic metastases in mouse lungs Citation[9], Citation[10]. We recently reported the significance of the administration of an acute hypoxia-releasing agent, nicotinamide, into tumour-bearing mice as a combined treatment with γ-ray irradiation in terms of repressing lung metastasis Citation[11]. Also in this study, continuous administration of TPZ, by which acutely hypoxic tumour cells are effectively killed, decreased the number of lung metastases, especially when combined with MTH that increased the dose of TPZ delivered. Meanwhile, as the delivered γ-ray dose increased with irradiation, the number of macroscopic lung metastases decreased, reflecting the decrease in the number of clonogenically viable tumour cells in the primary tumour (). Consequently, continuous administration of TPZ in combination with MTH in γ-ray irradiation showed a little more potential to reduce the number of metastases.
It was elucidated that control of the chronic hypoxia-rich Q cell population in primary solid tumours has the potential to impact the control of local tumours as a whole, while control of the acute hypoxia-rich total tumour cell population has the potential to impact the control of lung metastases. Namely, in conventional radiotherapy, continuous TPZ administration combined with MTH is thought to have a great potential to control both local solid tumours and lung metastases from the local tumours.
Declaration of interest
This study was supported in part by Grants-in-aid for Scientific Research (B) (23300348 and 23390355) from the Japan Society for the Promotion of Science. The authors alone are responsible for the content and writing of the paper.
References
- Vaupel P. Tumor microenvironmental physiology and its implications for radiation oncology. Semin Radiat Oncol 2004; 14: 198–206
- Masunaga S, Ono K, Hori H. Exploiting tumor hypoxia in the treatment of solid tumors. Jpn J Hyperthermic Oncol 2001; 17: 13–22
- Masunaga S, Ono K. Significance of the response of quiescent cell populations within solid tumors in cancer therapy. J Radiat Res 2002; 43: 11–25
- Masunaga S, Nagasawa H, Uto Y, Hori H, Suzuki M, Nagata K, et al. The usefulness of continuous administration of hypoxic cytotoxin combined with mild temperature hyperthermia, with reference to effects on quiescent tumor cell populations. Int J Hyperthermia 2005; 21: 305–318
- Griffin RJ, Okajima K, Ogawa A, Song CW. Radiosensitization of two murine tumours with mild temperature hyperthermia and carbogen breathing. Int J Radiat Biol 1999; 75: 1299–1306
- Masunaga S, Ono K, Hori H, Kinashi Y, Suzuki M, Takagaki M, et al. Modification of tirapazamine-induced cytotoxicity in combination with mild hyperthermia and/or nicotinamide: Reference to effect on quiescent tumour cells. Int J Hyperthermia 1999; 15: 7–16
- Boyd D. Invasion and metastasis. Cancer Metastasis Rev 1996; 15: 77–89
- Fidler IJ. The pathogenesis of cancer metastasis: The ‘seed and soil’ hypothesis revisited. Nat Rev Cancer 2003; 3: 453–845
- Cairns RA, Kalliomaki T, Hill RP. Acute (cyclic) hypoxia enhances spontaneous metastasis of KHT murine tumors. Cancer Res 2001; 61: 8903–8908
- Rofstad EK, Galappathi K, Mathiesen B, Ruud E-BM. Fluctuating and diffusion-limited hypoxia in hypoxia induced metastasis. Clin Cancer Res 2007; 13: 1971–1978
- Masunaga S, Matsumoto Y, Hirayama R, Kashino G, Tanaka H, Suzuki M, et al. Significance of hypoxia manipulation in solid tumors in the effect on lung metastases in radiotherapy, with reference to its effect on the sensitivity of intratumor quiescent cells. Clin Exp Metastasis 2009; 26: 693–700
- Duan X, Zhang H, Liu B, Li XD, Gao QX, Wu ZH. Apoptosis of murine melanoma cells induced by heavy-ion radiation combined with Tp53 gene transfer. Int J Radiat Biol 2008; 84: 211–217
- Nishimura Y, Ono K, Hiraoka M, Masunaga S, Jo S, Shibamoto Y, et al. Treatment of murine SCC VII tumors with localized hyperthermia and temperature-sensitive liposomes containing cisplatin. Radiat Res 1990; 122: 161–167
- De Jaeger K, Kavanagh M-C, Hill RP. Relationship of hypoxia to metastatic ability in rodent tumours. Br J Cancer 2001; 84: 1280–1285
- Hall EJ, Giaccia AJ. Time, dose, and fractionation in radiotherapy. Radiobiology for the Radiologist7th, EJ Hall, AJ Giaccia. Lippincott Williams & Wilkins, Philadelphia, PA 2012; 391–411
- Vaupel P, Kelleher DK. Pathophysiological and vascular characteristics of tumours and their importance for hyperthermia: Heterogeneity is the key issue. Int J Hyperthermia 2010; 26: 211–223
- Brown JM. Evidence of acutely hypoxic cells in mouse tumours, and a possible mechanism of reoxygenation. Br J Radiol 1979; 2: 650–656
- Chaplin DJ, Horsman MR, Trotter MJ. Effect of nicotinamide on the microregional heterogeneity of oxygen delivery within a murine tumor. J Natl Cancer Inst 1990; 82: 672–676
- Sun X, Xing L, Ling CC, Li G. The effect of mild temperature hyperthermia on tumour hypoxia and blood perfusion: Relevance for radiotherapy, vascular targeting and imaging. Int J Hyperthermia 2010; 26: 224–231
- Masunaga S, Ono K, Suzuki M, Nishimura Y, Hiraoka M, Kinashi Y, et al. Alteration of the hypoxic fraction of quiescent cell populations by hyperthermia at mild temperatures. Int J Hyperthermia 1997; 13: 401–411
- Cardenas-Navia LI, Secomb TW, Dewhirst MW. Effects of fluctuating oxygenation on tirapazamine efficacy: Theoretical predictions. Int J Radiat Oncol Biol Phys 2007; 67: 581–586