Abstract
We investigated the effect of hyperthermia exposure on the early stages of face processing by recording event-related potentials (ERPs) elicited by faces and non-face stimuli presented in upright and inverted orientations. Across all conditions, both the peak latencies of P1 and N170 components were earlier in the hyperthermia group than in the control participants. Although no effects of P1 amplitudes were influenced by hyperthermia, the face effect (larger amplitude for faces relative to other object categories) of the N170 was modulated by hyperthermia, whereas the face effect was significant in the control group, it was minimised in the hyperthermia group. The inversion effect of faces on N170 amplitudes, however, was not affected by hyperthermia. These data suggest that the detection of faces in the visual field and their initial streaming to face-specific structural encoding mechanisms are impaired by hyperthermia. However, subsequent face-specific configural processing revealed by the N170 inversion effect is not affected by hyperthermia. In addition, hyperthermia accelerates the early stage of visual perception, regardless of faces or non-face objects.
Introduction
It has been known that hyperthermia negatively affects physical performance Citation[1], such as circulatory, metabolic, muscular, nutritional, immunological and thermoregulatory factors Citation[2–4]. While hyperthermia increases muscle metabolic rate and blood flow to the skin, there appears to be no effect on cardiac output, mean arterial pressure and muscle or skin blood flow or isometric contractions of muscle function, and the ability of muscle to extract oxygen seems to be well preserved Citation[1]. As well as the above physiological changes, hyperthermia also induces changes in the central nervous system, i.e. central fatigue Citation[3], Citation[5].
Although several hypotheses considered that hyperthermia may have effects on the synthesis and metabolism of serotonin, dopamine and noradrenaline, interleukins, β-endorphins and ammonia Citation[6–10], recently it was suggested that hyperthermia impaired cognitive function alterations Citation[11], Citation[12] and in particular, decreased memory capacity in both working memory and short-term memory, suggesting the dysfunction of frontal lobe activity in hyperthermia condition Citation[13]. By recording and analysing electroencephalogram (EEG) signals, it was found that increasing body temperature resulted in faster α rhythms in resting subjects Citation[14], There was also evidence that the α/β ratio increased with the increasing core temperature, and that these changes were generated in frontal motor areas, and the central motor cortex as well as the occipital cortex Citation[15], Citation[16]. In addition, a difference in brain temperature of only 1°C was sufficient to obtain a significant difference in peak θ frequency in the rapid-eye movement (REM) sleep EEG Citation[17], indicating that body temperature can cause changes in the EEG power spectra. Moreover, there was evidence that an increase in oesophageal temperature from 37.14 ± 0.25°C to 39.05 ± 0.15°C produced a significant reduction of auditory evoked potentials, which depend on brain stem temperature Citation[18], indicating that the low-level nervous system was affected by brain stem temperature. Another study relevant to finger shock stimulation revealed that P50 and P50-N70 amplitudes decreased regularly and significantly over the whole duration of the heating period, indicating that the hyperthermia could potentially disturb the somatosensory central nervous system Citation[19]. In particular, a recent study revealed that passive heat exposure for a long time (1 h) impaired pre-attentive processing reflected by the decrease of mismatch negativity (MMN) amplitudes Citation[20]. Particularly relevant to the current study, low-level visual functions are also impaired in heat-stress conditions. For example, there was evidence that the visual evoked potential (VEP) amplitude elicited by black and white checkerboard was reduced after heating, especially in patients with multiple sclerosis Citation[21], Citation[22], implying that conduction of more axons in the visual pathways was blocked. However, whether high-level visual perception abilities are affected by heat stress is less evident. To this end, in the present study we investigated possible heat effects on early stages of face processing.
Faces are ecologically important stimuli that provide essential cues relevant to a person's identity, mood, emotion, or intent; perceptual information that forms the basis of interpersonal communication. Ample evidence indicates that the processing of human faces relies both on local features and/or on the configural or holistic relations between these features Citation[23–29]. As mentioned above, there have been several studies showing that the early stage of information processing (P50-N70 of finger shock stimulation, P2 components of VEP, auditory MMN) was impaired in hyperthermia Citation[19], Citation[21], Citation[22]. It is possible, therefore, that heat stress may have a deleterious effect on the early stage of face processing that is independent of cognitive performance such as working memory Citation[13]. To address this question, in the present study we explored the early stages of face perception using the N170, an electrophysiological index of early face processing Citation[30]. In addition, the P1, which is an electrophysiological index related to the processing of basic visual stimuli, was also investigated.
The N170 is a robust negative deflection peaking normally between 160 and 180 ms at occipito-temporal sites with significantly larger amplitude for faces relative to other object categories Citation[30]. Previous study showed that the N170 is not sensitive to face identity Citation[31–33], is larger (and delayed) for face components (particularly eyes) than full faces Citation[30], Citation[34], Citation[35], larger (and delayed) for inverted faces Citation[30], Citation[36] and equally large for scrambled and normally configured faces Citation[37]. It was suggested that the N170 is triggered by the detection of global face structures as well as other face-related information in the visual field and initiates the perceptual processes that facilitate the individuation and identification of faces within category Citation[30], Citation[37], Citation[38]. In particular, converging behavioural data suggested that the face-inversion effect Citation[39], a disproportionate decrease in recognition performance when the face is inverted upside down, is due to the disruption of holistic/configural processing Citation[34], Citation[35]. Therefore, the N170 inversion effect (enhanced and delayed) has been the indicator of configural analysis of faces Citation[40], Citation[41].
In the present study we explored possible heat effects on face perception (as reflected by the N170 effect) and the application of configural processing strategies (reflected by the N170 inversion effect). An oddball paradigm was used, in which faces and non-face objects (tables) were randomly interspersed with butterfly pictures as visual target stimuli, and the participants kept a mental count of the occurrence of the target Citation[30], Citation[42]. A face-specific effect in early visual processing under heat-stress conditions should be reflected in the difference between the N170 elicited by faces and objects. Moreover, if heat stress does impair face configural processing, the N170 inversion effect should be decreased under heat conditions compared with normal temperature conditions.
Material and methods
Subjects
A total of 28 right-handed young male undergraduates (mean age 21.75 years, range 19–24 years) were divided into two groups, the control group (14 subjects) with 1 h exposure of 25°C, and the heat group with 1 h exposure of 50°C. All participants reported normal or corrected-to-normal vision and had no history of current or past neurological or psychiatric illness and took no medications known to affect the central nervous system. They signed an informed consent approved by the Jinan Ethical Committee and were paid for their participation.
Procedure
Subjects performed the task in a dimly lit room, whose indoor temperature could be controlled strictly within 1°C by four automatic heaters (one was located 1.2 m in front of the subject, one 1 m behind and one at each side at 0.5 m). Stimuli were presented on the computer screen (38.1 cm) at 60 cm from the subjects. The stimuli were 30 unfamiliar young faces, 30 tables, and 30 flowers. These stimuli were used to form five stimulus conditions: (1) upright faces, (2) inverted faces, (3) upright tables, (4) inverted tables and (5) targets (flowers) (). All images were presented by grey-scale 11.5 × 12.0 cm photographs. Half of the faces were male and half female, and were presented without hair, glasses, or other accessories. All images were equated for luminance and root mean square (RMS) contrast (not including the grey background in calculation), using Adobe Photoshop CS4 software.
Figure 1. Examples of target flowers and non-target stimuli (faces and tables) in the upright and inverted orientations.
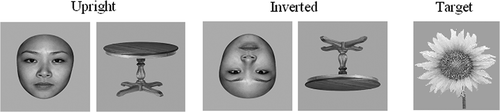
The stimuli were presented at fixation, seen from a distance of 60 cm and occupying a visual angle of 5.37°–6.25°. The subjects sat in a comfortable chair and were instructed to keep a mental count of flowers that occurred occasionally on the screen, while ignoring all other stimuli. This procedure has been frequently used in N170 research in order to assign similar task relevance to faces and non-face stimuli Citation[42]. The 900 stimuli were presented one at a time in six blocks, each consisting of 30 upright faces, 30 inverted faces, 30 upright tables, 30 inverted tables, and 30 flowers (targets). The order of trials within each block was randomised. Stimulus exposure time was 300 ms and separated by an inter-trial interval of 1000 ms (randomised between 800–1200 ms). There was a 1-min break between blocks, when the subject numbered off the counted flowers to the experimenter.
During the experiment, the temperature inside the room was kept constant to simulate a passive heat environment. Two electronic heaters were placed inside and four automatic thermal controllers were placed in the four corners of the room, respectively. Once the temperature inside the room reached the designated temperature, 50°C, the heater would stop working or the heaters would quickly work again. Thus, the temperature inside the room was kept constant at the expected level of 50°C.
For each participant, to avoid weight loss 100 mL water was given before the start of the experiment. Body weights and body core temperature (a thermocouple (Hohour, ZWJ-2, Shanghai, China) inserted beyond the anal sphincter) were measured before and after experiments. During the experiment, participants were asked to refrain from drinking and urinating.
Electrophysiological recording and measures
EEG was recorded continuously by a set of 32 Ag/AgCl electrodes placed according to the 10/20 system. The EEG recording sites were: FP1, FP2, F7, F3, Fz, F4, F8, FT7, FC3, FCz, FC4, FT8, T7, C3, Cz, C4, T8, TP7, CP3, CPz, CP4, TP8, P7, P3, Pz, P4, P8, O1, Oz, and O2 as well as left (M1) and right (M2) mastoids. Electrooculograms (EOG) were recorded via electrodes placed on the bilateral external canthi and the left infraorbital and supra-orbital areas to monitor for eye movements and blinks. Both EEG and EOG were sampled at 1000 Hz, with a 0.1–100 Hz band pass using a NuAmps digital amplifiers system (Neuroscan Labs, El Paso, TX). The tip of the nose was used for reference during recording. Electrode impedances were kept below 5 kΩ.
EOG artefacts were corrected using a correlation method proposed by Semlitsch and colleagues Citation[43] (Neuroscan Labs, El Paso, TX, USA). Then the recorded EEG data was segmented in epochs of 1000 ms beginning 200 ms prior to stimulus onset and averaged separately for each of the five conditions. Segments contaminated with artefacts exceeding an amplitude of ±100 µV were excluded from averaging. After this procedure, averaged event-related potentials (ERPs) included at least 55 trials for faces and tables and 30 trials for flowers. The averaged ERPs were low-pass filtered at 30 Hz (24 dB/octave).
Based on the visual inspection of grand-averaged ERPs and on the existing literature Citation[44], we analysed the N170 components only at a subset of sites including the lateral sites P7 and P8, M1 and M2, as well as more medially at the O1 and O2 electrodes. In addition, since group differences were observed for the preceding P1 component, we have also analysed these differences at the same locations. The peak amplitudes and latencies were measured automatically between 70 ms and 120 ms and between 120 ms and 200 ms for the P1 and N170, respectively (, –).
Figure 2. The diagrammatic drawing of peak amplitudes and latencies for P1 and N170, which were automatically measured between 70 ms and 120 ms and between 120 ms and 200 ms, respectively.
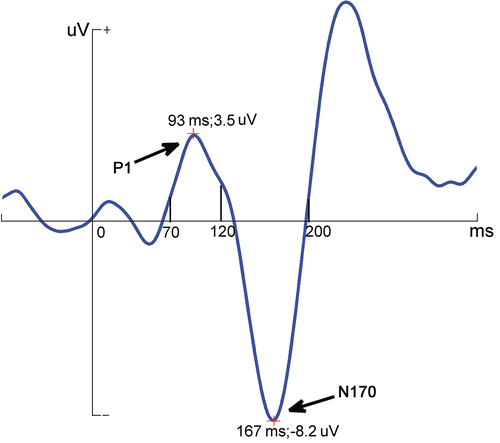
These measurements were analysed by using repeated ANOVA, with the Group (control and hyperthermia) as a between-subject factor, and Testing time (before heat, post-heat), Stimulus type (faces, tables), Orientation (upright, inverted), Hemispheres (left, right), and Sites (P7/8, M1/2, O1/2) as within-subject factors. Statistical probability from the ANOVAs was corrected using the Greenhouse-Geisser procedure. Post hoc tests (Bonferroni corrected for multiple comparisons) were performed when necessary.
Results
Body weight and temperature responses
For the control group there were no significant changes for body weight (68.8 kg and 68.8 kg, p = 0.9) and body temperature (37.2°C and 37.3°C, p = 0.8). For the hyperthermia group, compared to control conditions before the test, body temperature was significantly increased (37.3°C and 38.4°C for control and heat conditions, respectively; p < 0.001) and the body weight losses were less than 0.1% less than control (69.6 kg and 68.9 kg for control and heat conditions, respectively; p = 0.89).
ERP data
Target monitoring was equally good in both groups (97.9% for control participants and 96.8% for hyperthemia participants, respectively; F(1, 26) < 1). Non-target stimuli, i.e. faces and tables, elicited clear P1 and N170 components with significant occipital temporal distribution in both control and hyperthermia participants ( and ). Although there were some slight latency differences of P1 (F(1, 26) < 1) and N170 (F(1, 26) < 1) between control and hyperthermia groups, according to the grand-averaged waveforms the P1 and N170 peaked at similar latency time windows. As mentioned in the Methods section above, therefore, we measured the peak latencies and amplitudes in the same time windows for both groups, between 70 ms and 120 ms and between 120 ms and 200 ms for the P1 (seen in , , , ) and N170 (seen in , , , ), respectively.
Figure 3. (A) The grand-averaged waveforms elicited by face and table stimuli before and after 1 h exposure in the heat and control groups, respectively. (B) Grand-averaged ERP topography elicited by upright faces and tables at the analysed sites for control and hyperthermia participants after exposure.
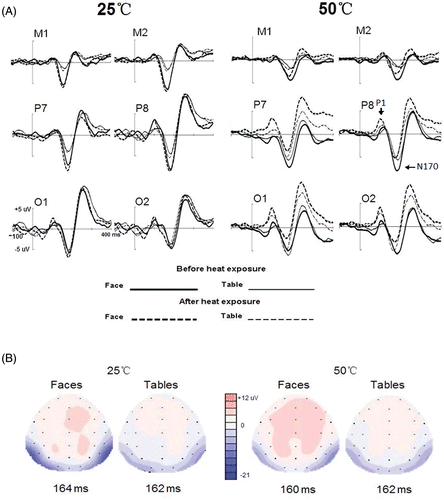
P1 component
In the P1 amplitude analysis, no main effect of Group was found (F(1, 26) < 1) (as presented in ). The analysis of the amplitude yielded several significant effects of factors with repeated measures, none of which, however, interacted with Group. The P1 elicited by faces (3.6 µV) was bigger than that elicited by tables (2.7 µV; F(1, 26) = 21.9, p < 0.001) and the amplitude was larger at the right hemisphere sites (3.4 µV) than the left hemisphere sites (2.8 µV; F(1, 26) = 19.4, p < 0.001). At the more posterior sites, the P1 was larger than at the more anterior sites (4.2 µV, 3.3 µV, and 1.9 µV for O1/2, P7/8, and M1/2, respectively; F(1, 26) = 13.2, p < 0.001), with Bonferroni comparisons showing significant differences between O1/2 and P7/8 (p = 0.005), between O1/2 and M1/2 (p < 0.001) and between M1/2 and P7/8 (p < 0.001). Although the P1 for faces was larger than for tables at all sites (F(1, 26) = 13.8, p < 0.05), this effect was smaller at M1/2 (0.4 µV) than O1/2 (1.1 µV, p < 0.001) and P7/8 (1.2 µV, p < 0.001). The inversion effect (inverted stimuli minus upright stimuli) () was not significant at all sites (p > 0.1 for each). For the analysis of P1 latency, there was no significant difference between two groups (108 ms and 104 ms for control and heat groups, respectively; F(1, 26) < 1, p = 0.447) and, interestingly, P1 latency was shorter after 1 h exposure (104 ms) than that before 1 h exposure (108 ms; F(1, 26) = 12.260, p = 0.002). The main effect of Site was also significant (F(2, 52) = 7.45, p = 0.03), with further Bonferroni comparisons showing shorter latency at O1/2 (103.5 ms) than at P7/8 (107.0 ms, p < 0.010) and M1/2 (107.9 ms, p < 0.001), with no difference for the latter two sites (p = 0.790). Testing time × Site interaction (F(2, 52) = 11.817, p < 0.001) indicated that whereas the Testing time effect was evident at O1/2 (before minus after 1 h heat exposure, 6.0 ms; p < 0.01) and M1/2 (3.7 ms; p < 0.05), it was not significant at P7/8 (1.6 ms; p = 0.19). What should be stressed is the interaction of Testing time × Group (F(1, 26) = 13.54, p < 0.001), indicating that the Testing time effect only existed in the hyperthermia group (109 ms and 100 ms for before and after 1 h exposure at 50°C, p < 0.001), while the latency of P1 was not modulated by Testing time in control conditions (107 ms and 108 ms for before and after 1 h exposure at 25°C, p = 0.4).
N170 component
As presented in and , during 100–300 ms post-stimuli onset, faces elicited more negative N170 than did tables, i.e. N170 face effect, regardless of heat or orientation conditions. After heat exposure, the N170 face effect was more conspicuous in the controls than in the heat group. Although there was no difference before and after 1 h exposure in the control group, the N170 declined significantly in the heat group after 1 h exposure.
In the N170 amplitude analysis, overall the N170 was similar between the two groups (−6.5 µV and −5.7 µV for the control and heat groups, respectively; F(1, 26) < 1). The following effects were significant and did not interact with Group: Faces elicited larger N170 (−7.3 µV) than did tables (−4.9 µV; F(1, 26) = 45.121, p < 0.001) but this effect was qualified by the two-way interaction of Orientation × Stimulus type, F(1, 26) = 40.183, p < 0.001. Further analysis revealed that whereas inversion enhanced the N170 of faces (−7.8 µV and −6.8 µV for inverted and upright faces, respectively; p < 0.001), the N170 amplitude in response to inverted tables was decreased (−4.6 µV) over upright tables (−5.2µV; p = 0.005). The Site × Stimulus type interaction was significant, F(1, 26) = 69.731, p < 0.001. Further analysis revealed that, whereas for faces the N170 was significantly larger at P7/8 (−8.2 µV) than at either M1/2 (−6.8 µV) (p < 0.05) or O1/2 (−6.9 µV) (p < 0.05), with no significant difference between the latter two sites (p = 0.882), for tables, the N170 was decreased significantly at M1/2 (−2.7 µV) than at either P7/8 (−5.9 uV, p < 0.001) and O1/2 (−6.1 uV, p < 0.001), with no difference between the latter two sites (p = 0.639). Furthermore, although significant at all sites, the Stimulus type effect was largest at the P7/8 sites and smallest at the O1/2 sites (−4.1 µV, −2.3 µV and −0.8 µV for the P7/8, M1/2, and O1/2 sites, respectively). Finally, the N170 was larger at the right hemisphere site (−6.5 µV) than at the left hemisphere site (−5.7 µV; F(1, 26) = 13.142, p < 0.05).
More relevant to the scope of the present study, however, were the effects which were modulated by hyperthermia. For N170 amplitude, a significant two-way interaction of Testing time × Group, F(1, 26) = 5.911, p < 0.05, indicated that, while in the control group the N170 was maintained after 1 h exposure of 25°C (−6.3 µV and −6.6 µV for before and after 1 h exposure, respectively; p = 0.491), it was decreased by the heat stress (−6.4 µV and −5.0 µV for before and after 1 h heat exposure; p < 0.05). This two-way interaction was further qualified by two three-way interactions of Stimulus type × Testing time × Group (F(1, 26) = 6.127, p < 0.005) and Sites × Testing time × Group (F(2, 52) = 11.124, p < 0.005). Further analysis revealed that although the N170 face effect was not affected by 1 h exposure of 25°C (−3.2 and −3.0 µV for before and after 1 h exposure; p = 0.714), it was decreased significantly after 1 h exposure in heat stress (−2.5 and −0.9 µV for before and after 1 h exposure of 50°C, respectively; p < 0.05). However, for tables, N170 amplitude was not significantly changed before and after 1 h exposure of 25°C (F(1, 26) = , p = 0.530) or 50°C (F(1, 26)=, p = 0.411). Moreover, the decrease of N170 amplitude was significant at P7/8 (−7.5 µV and −5.8 µV for before and after 1 h exposure of 50°C, respectively; p < 0.05) and O1/2 (−7.3 µV and −5.2 µV for before and after 1 h exposure of 50°C, respectively; p < 0.05) not at M1/2 site (−4.3 µV and −4.1 µV for before and after 1 h exposure of 50°C, respectively; p = 0.89). Finally, the Hemisphere × Sites × Group interaction was also significant, F(2, 52) = 21.045, p < 0.001, showing that, while in the control group the N170 amplitudes were significantly increased on the right side at the M1/2 (right minus left: −1.0 µV, p < 0.05) and P7/8 sites (−2.0 µV, p < 0.05), this hemisphere effect, i.e. higher N170 over right hemisphere than left, was significant at P7/8(−1.1 µV, p < 0.05) and O1/2(−1.3 µV, p < 0.05) sites in the hyperthermia group.
Analysis of peak latency revealed that, overall, the N170 latency was similar in the control (167 ms) and hyperthermia (165 ms; F(1, 26) < 1) groups. Across groups, inversion delayed N170 latency significantly (165 ms and 167 ms for upright and inverted conditions, respectively; F(1, 26) = 8.159, p < 0.05) and the significant interaction of Orientation × Stimulus type (F(1, 26) = 4.696, p < 0.05) showed that the inversion effect was evident for faces (p = 0.002), not for tables (p = 0.38). Although faces elicited slightly but significantly shorter N170 latency (165 ms) than did tables (167 ms; F(1, 26) = 6.481, p < 0.05), this Stimulus type significantly interacted with Group (F(1, 26) = 9.242, p < 0.05), revealing that the Stimulus type effect was conspicuous in the control group (165 ms and 169 ms for faces and tables, respectively; p = 0.001), not in the hyperthermia group (166 ms and 165 ms for faces and tables, respectively; p = 0.73). The main effect of Testing time was also significant, F(1, 26) = 37.227, p < 0.001, indicating that compared to pre-test condition (170 ms), N170 peaked earlier after 1 h exposure (163 ms). Interestingly, this effect was qualified by the two-way interaction of Group × Testing time, F(1, 26) = 7.953, p < 0.05, revealing that the Testing time effect of N170 latency was larger in the hyperthermia group (11 ms; p < 0.001) than that in the control group (4 ms; p = 0.028), although the N170 latency was very similar for pre-test conditions between the two groups (169 ms and 170 ms for control and hyperthermia groups, respectively; p = 0.79). Furthermore, a significant Stimulus type × Orientation × Sites × Group interaction (F(2, 52) = 5.318, p < 0.05) followed by Bonferroni corrected pair wise comparison post hoc tests showed that, whereas in the control group inverted faces elicited a comparatively longer latency only at P7/8 (0.4 ms, 0.8 ms and 0.1 ms for M1/2, P7/8 and O1/2, respectively; p = 0.013 for P7/8, p > 0.05 for M1/2 and O1/2), in the hyperthermia group the delayed latency appeared at all sites (0.8 ms, 1.7 ms and 1.2 ms for M1/2, P7/8 and O1/2, respectively; p < 0.05 for M1/2, P7/8 and O1/2).
Discussion
In this study we investigated whether perceptual processes involved in the early stages of face processing were influenced by hyperthermia. Should such effects be found, we sought to determine their nature and time course. To achieve this goal, the hyperthermia effects on face detection and configural analysis were assessed by recording the early ERP components (P1 and N170) elicited by faces and objects (tables) presented in upright and upside down orientations.
The major findings could be summarised as follows: Compared with control conditions, body core temperature was significantly increased and the body weight loss was less than 0.1% lower for both groups. Across all experimental stimulus conditions, both the peak latencies of P1 and N170 components were earlier after 1 h exposure in both control group and hyperthermia group. In addition, we found a modulation of hyperthermia for P1 and N170 latency. Both the peak latencies of P1 and N170 components were earlier in the hyperthermia group than in the control participants. Furthermore, the hyperthermia effects on latency were modulated by stimulus type and orientation: the N170 latency difference between faces and tables disappeared after 1 h at 50°C heat exposure, and the scalp distribution of N170 inversion effect (delayed peak latency for inverted faces) was expanded.
Thermal environment can produce different effects such as dehydration, physical and fatigue stressors Citation[45]. There was evidence that a 2% or more decrease in body weight could result in dehydration which could lead to deterioration of cognitive abilities Citation[46], and, possibly due to adequate fluid replacement, cognitive performance was indeed unaffected when body weight changes did not exceed 0.5% Citation[47]. In the present study, to avoid dehydration effects all participants ingested water before the experiment and, finally, body weight loss was less than 0.1% of control. Hence, the observed face detection changes were not caused by weight changes per se. Compared to the controls, in heat conditions the increment of body core temperature was significant and mean body temperature was increased 1.1°C by thermal stress in our experiment. In line with the current findings, previous studies reported when deep body temperature was disturbed with thermal stress exposures away from both normal and steady-state conditions, vigilance could be impaired (Hancock, 1986). In addition, a study evaluating psychological stress reactions during hyperthermia treatments showed that hyperthermia treatments are not excessively stressful, either systemically or psychologically Citation[48], which implies that the underlying mechanism responsible for the measured ERP changes is hyperthermia, rather than the psychological changes elicited by hyperthermia exposure.
Across the two groups the scalp distribution of P1 and N170 demonstrated that these two components are dissociated: P1 was largest at the occipital sites (O1 and O2), whereas N170 was largest at the posterior lateral sites (P7 and P8). Moreover, whereas the N170 elicited was right lateralised only to faces, P1 was larger at the right hemisphere for both faces and tables. Nevertheless, the amplitude of P1 was sensitive to stimulus manipulations as well as N170. It was higher in response to faces than tables. Importantly, however, unlike N170, none of these effects of P1 amplitudes were influenced by hyperthermia, and the P1 amplitudes were equal for inverted and upright stimulus. The face effect (larger amplitude for faces relative to other object categories) of the N170, on the other hand, was modulated by hyperthermia: whereas the distinction between faces and non-face stimuli was significant in the control group, the face effect was minimised in the hyperthermia group. The inversion effect of faces on amplitude, however, was not affected by hyperthermia.
Although anticipating no P1 modulations, the present study focused primarily on the N170. One of the important findings is that the face effect decreased after 1 h heat exposure. Studies about the cognitive mechanisms of face processing have proven that faces are recognised in configural processing, while the tables were perceived in a featural manner Citation[25], Citation[49–52]. The visual N170 component peaks at occipito-temporal electrode sites are believed to be a marker to investigate non-invasively the time course of configural face recognition in the human brain and characterise their sensitivity to various stimulus manipulations. The larger amplitude of N170 for faces than for tables seems to lie in the recruitment of face-specific cortex Citation[53]. Generally, there are three face-selective regions which respond to changes in different aspects of faces Citation[54]: the fusiform face area (FFA) Citation[55–57] found in the mid-fusiform gyrus, the occipital face area (OFA) Citation[58] found in the lateral inferior occipital gyri, and a face-selective region in the posterior part of the superior temporal sulcus (fSTS) Citation[59], Citation[60]. Several studies revealed that the face-selective responses in FFA and fSTS were highly correlated with the face-selective N170 component Citation[61]. Following this hypothesis, the decreased face effect of the N170 in hyperthermia conditions may be caused by the weakened activation of face-selective cortex, while the neurons for non-faces were not affected significantly.
In contrast to the N170 face effect, the inversion effect on the N170 was not modulated by high temperature atmosphere. Conclusive evidence has shown that faces presented upside-down are harder to perceive, memorise and recognise than upright faces, and the inversion effect primarily reflects the recruitment of object-selective neurons needed to process inverted faces, due to the disruption of the face configuration Citation[34], Citation[35]. Given that the featural processing of tables is not influenced by hyperthermia, it is not so surprising that the activation of object-selective neurons for inverted faces, like that of tables, is not modulated by hyperthermia. The maintained inversion effect in the hyperthermia group may be due to the proportionally decreased activation in FFA and STS for upright and inverted faces and minor effect on object-selective neurons, which leads to a maintained inversion effect of faces. Combining the decreased face effect and maintained inversion effect, we can conclude that hyperthermia mainly disrupts the face-selective neurons while the non-faces based activation is less affected.
P1 is an early index of endogenous processing of visual stimuli, with sources in the striate and extrastriate cortex Citation[62]. It is thought to represent the psychophysical differences in the stimuli or top-down or attentional modulations Citation[63] as well as to reflect physical variations in the stimuli used such as luminance, colour, contrast or spatial frequencies of the stimulus Citation[64–66]. Although most ERP studies of face processing did not find categorical sensitivity to faces at P1 Citation[67–71], other studies have reported larger P1 in response to faces than to objects Citation[53], Citation[72], Citation[73]. At the same time, there is evidence that the P1 are sensitive to violation of the first-order configuration of a face, such as orientation and Mooney faces defined by shape from shading Citation[42], Citation[66], Citation[73–75], which may barely be accounted for by low-level factors in vision. The present study is not the first to report higher P1 to faces. The inversion effect of P1 for faces, however, was not found. What is more, the face effects of P1, without any changes of luminance, colour, contrast or spatial frequencies of the stimulus were not modulated by heat stress. This phenomenon supports that P1 may be just a component reflecting physical variations in the stimuli, rather than a component representing the configural processing Citation[64], Citation[71], as it is not modulated in the same pattern as N170 during long exposure at high temperature. The face effect of P1 may be due to differences in the global or local low-level parameters between stimulus categories generally left uncontrolled in ERP studies; although in the present study all images used in this study were equated for luminance and contrast, the area subtended by tables was much smaller than the area occupied by faces Citation[42]. Clearly determining the exact processing mechanism of P1 awaits additional research. Importantly, however, P1 is not modulated by hyperthermia, which may suggest that the low-level processing of faces is not influenced by heat stress.
Most of the above interpretations are based on the pattern of amplitude modulations. It is noteworthy that, overall, we found adequate evidence for hyperthermia-related latency modulation for either P1 or N170. Although the Test–time effect was not evident in the control group, the latencies of early components were significantly shortened after 1 h of 50°C heat stress, regardless of faces or objects. We considered that the speed perception for visual stimuli could be due to the increased conduction velocity of the optic nerve in hyperthermic conditions. Supporting this hypothesis, several studies on the effects of temperature on the conduction velocity for myelinated nerve fibres, including the phrenic nerve Citation[76], auditory nerves Citation[77], sural nerve Citation[78], median motor and sensory nerves Citation[79], and median nerve Citation[80], concluded that the conduction velocity of nerves increased non-linearly with increase in skin temperature Citation[79]. Although we did not directly test the conduction velocity of the optic nerve, it is still reasonable that hyperthermia can increase optic nerve conduction velocity significantly, which shortens the intervals between the onset of stimulus and early ERP components.
Before concluding, we should reiterate three procedural decisions that constrain the interpretation of the present findings. In the present study we investigated hyperthermia effects on humans under a degree of thermal stress with a designated 50°C, approximately 1 h heat exposure. First, the exposure timing and temperatures were fixed at the same values, i.e. 50°C, approximately 1 h heat exposure. It has been shown that the extent of cognitive performance deficits was associated with the extent of heat exposure, including the duration and the intensity of heat exposure Citation[12]. Therefore, it is necessary to further explore the relationship between face perception and the extent of heat exposure. Second, all the participants in this study were young men. The effects of gender on face perception have been established, for instance a lesser degree of lateralisation of brain functions related to face coding in women than in men Citation[81–83]. In addition, ample evidence indicates the aging modulation of the early stage of face perception. For example, the N170 inversion effect of faces, which was evident in younger people, was indeed absent in elderly people Citation[42]. Hence, further investigation using gender and age subgroups may explicate the adverse effect of hyperthermia more clearly. Finally, we did not investigate the physiological basis, such as plasma osmolarity, hormonal concentrations, neurotransmitter or redistribution of fluids, of the influenced cognitive performance. Though previous studies showed that hyperthermia-mediated central fatigue may include cerebral perturbations such as reduced perfusion of the brain, induced hypoglycaemia, accumulation of ammonia or depletion of neuronal energy stores, with a clear deteriorating effect on motor performance and prolonged exercise Citation[3], Citation[84], the true mechanism was still unclear as this evidence is mainly based on the blood, rather than the true internal environment of the central nervous system. As the technique develops in the future, the impact of physiological changes of the central nervous system on cognition should be able to shed light on the hyperthermia-induced effect on cognition.
These limitations notwithstanding, our study findings suggested that the detection of faces in the visual field and their initial streaming to face-specific structural encoding mechanisms are affected by hyperthermia revealed by decreased N170 face effect. However, subsequent face-specific configural processing revealed by the N170 inversion effect is not affected by hyperthermia. In addition, hyperthermia accelerates the early stage of visual perception, regardless of faces or non-face objects.
Table 1. The detailed latency (ms) and amplitude (uV) of P1 in the control group under pre-test conditions.
Table 2. The detailed latency (ms) and amplitude (uV) of P1 in the control group under post-test conditions.
Table 3. The detailed latency (ms) and amplitude (uV) of N170 in the control group under pre-test conditions.
Table 4. The detailed latency (ms) and amplitude (uV) of N170 in the control group under post-test conditions.
Table 5. The detailed latency (ms) and amplitude (uV) of P1 in the hyperthermia group under pre-test conditions.
Table 6. The detailed latency (ms) and amplitude (uV) of P1 in the hyperthermia group under post-test conditions.
Table 7. The detailed latency (ms) and amplitude (uV) of N170 in the hyperthermia group under pre-test conditions.
Table 8. The detailed latency (ms) and amplitude (uV) of N170 in the hyperthermia group under post-test conditions.
Declaration of interest: The authors report no conflicts of interest. The authors alone are responsible for the content and writing of the paper.
References
- Romer LM, Polkey MI. Fatigue mechanisms determining exercise performance. J Appl Physiol 2008; 104: 879–888
- Kreider R, Miriel V, Bertun E. Amino acid supplementation and exercise performance. Analysis of the proposed ergogenic value. Sports Med 1993; 16: 190–209
- Nybo L, Secher NH. Cerebral perturbations provoked by prolonged exercise. Prog Neurobiol 2004; 72: 223–261
- Frey B, Weiss E-M, Rubner Y, Wunderlich R, Ott OJ, Sauer R, et al. Old and new facts about hyperthermia-induced modulations of the immune system. Int J Hyperthermia 2012; 28(6)528–542
- Mundel T, Bunn SJ, Hooper PL, Jones DA. The effects of face cooling during hyperthermic exercise in man: Evidence for an integrated thermal, neuroendocrine and behavioural response. Exp Physiol 2007; 92: 187–195
- Nybo L, Nielsen B, Blomstrand E, Møller K, Secher N. Neurohumoral responses during prolonged exercise in humans. J Appl Physiol 2003; 95: 1125–1131
- Meeusen R, De Meirleir K. Exercise and brain neurotransmission. Sports Med 1995; 20: 160–188
- Gleeson M. Interleukins and exercise. J Physiol 2000; 529: 1
- Sgherza AL, Axen K, Fain R, Hoffman RS, Dunbar CC, Haas F. Effect of naloxone on perceived exertion and exercise capacity during maximal cycle ergometry. J Appl Physiol 2002; 93: 2023–2028
- Nybo L. CNS fatigue and prolonged exercise: Effect of glucose supplementation. Med Sci Sport Exer 2003; 35: 589–594
- Cian C, Barraud P, Melin B, Raphel C. Effects of fluid ingestion on cognitive function after heat stress or exercise-induced dehydration. Int J Psychophysiol 2001; 42: 243–251
- Hocking C, Silberstein RB, Lau WM, Stough C, Roberts W. Evaluation of cognitive performance in the heat by functional brain imaging and psychometric testing. Comp Biochem Physiol A Mol Integr Physiol 2001; 128: 719–734
- Racinais S, Gaoua N, Grantham J. Hyperthermia impairs short-term memory and peripheral motor drive transmission. J Physiol 2008; 586: 4751–4762
- Deboer T. Brain temperature dependent changes in the electroencephalogram power spectrum of humans and animals. J Sleep Res 1998; 7: 254–262
- Nybo L, Nielsen B. Middle cerebral artery blood velocity is reduced with hyperthermia during prolonged exercise in humans. J Physiol 2001; 534: 279–286
- Nielsen B, Nybo L. Cerebral changes during exercise in the heat. Sports Med 2003; 33: 1–11
- Deboer T. Electroencephalogram theta frequency changes in parallel with euthermic brain temperature. Brain Res 2002; 930: 212–215
- Nielsen B, Jessen C. Evidence against brain stem cooling by face fanning in severely hyperthermic humans. Pflugers Archiv Eur J Physiol 1992; 422: 168–172
- Dubois M, Coppola R, Buchsbaum MS, Lees DE. Somatosensory evoked potentials during whole body hyperthermia in humans. Electroencephalogr Clin Neurophysiol 1981; 52: 157–162
- Hancock PA, Vasmatzidis I. Effects of heat stress on cognitive performance: The current state of knowledge. Int J Hyperthermia 2003; 19: 355–372
- Bajada S, Mastaglia F, Black J, Collins D. Effects of induced hyperthermia on visual evoked potentials and saccade parameters in normal subjects and multiple sclerosis patients. J Neurol Neurosur Ps 1980; 43: 849–852
- Matthews W, Read D, Pountney E. Effect of raising body temperature on visual and somatosensory evoked potentials in patients with multiple sclerosis. J Neurol Neurosur Ps 1979; 42: 250–255
- Goffaux V, Hault B, Michel C, Vuong QC, Rossion B. The respective role of low and high spatial frequencies in supporting configural and featural processing of faces. Perception 2005; 34: 77–86
- Schwaninger A, Lobmaier J, Collishaw S. Role of featural and configural information in familiar and unfamiliar face recognition. Biologically Motivated Computer Vision 2010; 2: 245–258
- Itier RJ, Taylor MJ. Face recognition memory and configural processing: A developmental ERP study using upright, inverted, and contrast-reversed faces. J Cogn Neurosci 2004; 16: 487–502
- Cheung OS, Richler JJ, Palmeri TJ, Gauthier I. Revisiting the role of spatial frequencies in the holistic processing of faces. J Exp Psychol 2008; 34: 1327–1336
- Gauthier I, Curran T, Curby KM, Collins D. Perceptual interference supports a non-modular account of face processing. Nat Neurosci 2003; 6: 428–432
- Richler JJ, Gauthier I, Wenger MJ, Palmeri TJ. Holistic processing of faces: Perceptual and decisional components. Learn Memory 2008; 34: 328–342
- Richler J, Tanaka J, Brown D, Gauthier I. Why does selective attention to parts fail in face processing?. J Exp Psychol Learn 2008; 34: 1356–1368
- Bentin S, Allison T, Puce A, Perez E, McCarthy G. Electrophysiological studies of face perception in humans. J Cogn Neurosci 1996; 8: 551–565
- Anaki D, Zion-Golumbic E, Bentin S. Electrophysiological neural mechanisms for detection, configural analysis and recognition of faces. Neuroimage 2007; 37: 1407–1416
- Bentin S, Deouell LY. Structural encoding and identification in face processing: ERP evidence for separate mechanisms. Cognitive Neuropsych 2000; 17: 35–55
- Eimer M. The face-specific N170 component reflects late stages in the structural encoding of faces. Neuroreport 2000; 11: 2319–2324
- Itier RJ, Alain C, Sedore K, McIntosh AR. Early face processing specificity: It's in the eyes!. J Cogn Neurosci 2007; 19: 1815–1826
- Itier RJ, Batty M. Neural bases of eye and gaze processing: The core of social cognition. Neurosci Biobehav Rev 2009; 33: 843–863
- Rossion B, Gauthier I. How does the brain process upright and inverted faces?. Behav Cogn Neurosci Rev 2002; 1: 63–75
- Zion-Golumbic E, Bentin S. Dissociated neural mechanisms for face detection and configural encoding: Evidence from N170 and induced gamma-band oscillation effects. Cereb Cortex 2007; 17: 1741–1749
- Bentin S, Golland Y, Flevaris A, Robertson LC, Moscovitch M. Processing the trees and the forest during initial stages of face perception: Electrophysiological evidence. J Cogn Neurosci 2006; 18: 1406–1421
- Diamond R, Carey S. Why faces are and are not special: An effect of expertise. J Exp Psychol Gen 1986; 115: 107–117
- Nederhouser M, Yue X, Mangini MC, Biederman I. The deleterious effect of contrast reversal on recognition is unique to faces, not objects. Vision Res 2007; 47: 2134–2142
- Boehm SG, Dering BR, Thierry G. Category-sensitivity in the N170 range: A question of topography and inversion, not one of amplitude. Neuropsychologia 2011; 49(7)2082–2089. Epub 2011 Apr 6
- Gao L, Xu J, Zhang B, Zhao L, Harel A, Bentin S. Aging effects on early-stage face perception: An ERP study. Psychophysiology 2009; 46: 970–983
- Semlitsch HV, Anderer P, Schuster P. A solution for reliable and valid reduction of ocular artifacts applied to the P300 ERP. Psychophysiology 1986; 23: 695–703
- Dahl CD, Wallraven C, Bulthoff HH, Logothetis NK. Humans and macaques employ similar face-processing strategies. Curr Biol 2009; 19: 509–513
- Wetsel WC. Hyperthermic effects on behavior. Int J Hyperthermia 2011; 27: 353–373
- Szinnai G, Schachinger H, Arnaud MJ, Linder L, Keller U. Effect of water deprivation on cognitive-motor performance in healthy men and women. Am J Physiol Regul Integr Comp Physiol 2005; 289: R275–280
- Simmons SE, Saxby BK, McGlone FP, Jones DA. The effect of passive heating and head cooling on perception, cardiovascular function and cognitive performance in the heat. Eur J Appl Physiol 2008; 104: 271–280
- Puchinger M, Meinitzer A, Stettin M, Rehak PH. Psychological and systemic stress reactions of patients during hyperthermia treatments. Int J Hyperthermia 2009; 25: 488–497
- Lobmaier JS, B lte J, Mast FW, Dobel C. Configural and featural processing in humans with congenital prosopagnosia. Adv Cogn Psychol 2010; 6: 23–34
- Behrmann M, Avidan G, Leonard GL, Kimchi R, Luna B, Humphreys K, et al. Configural processing in autism and its relationship to face processing. Neuropsychologia 2006; 44: 110–129
- Mondloch CJ, Le Grand R, Maurer D. Configural face processing develops more slowly than featural face processing. Perception 2002; 31: 553–566
- Schwarzer G, Zauner N, Jovanovic B. Evidence of a shift from featural to configural face processing in infancy. Dev Sci 2007; 10: 452–463
- Itier RJ, Taylor MJ. N170 or N1? Spatiotemporal differences between object and face processing using Erps. Cereb Cortex 2004; 14: 132–142
- Haxby JV, Hoffman EA, Gobbini MI. The distributed human neural system for face perception. Trends Cogn Sci 2000; 4: 223–232
- Kanwisher N, McDermott J, Chun MM. The fusiform face area: A module in human extrastriate cortex specialized for face perception. J Neurosci 1997; 17: 4302–4311
- Kanwisher N, Yovel G. The fusiform face area: A cortical region specialized for the perception of faces. Philos T Roy Soc B 2006; 361: 2109–2128
- McCarthy G, Puce A, Gore JC, Allison T. Face-specific processing in the human fusiform gyrus. J Cogn Neurosci 1997; 9: 605–610
- Gauthier I, Tarr MJ, Moylan J, Skudlarski P, Gore JC, Anderson AW. The fusiform ‘face area’ is part of a network that processes faces at the individual level. J Cogn Neurosci 2000; 12: 495–504
- Allison T, Puce A, McCarthy G. Social perception from visual cues: Role of the sts region. Trends Cogn Sci 2000; 4: 267–278
- Hoffman EA, Haxby JV. Distinct representations of eye gaze and identity in the distributed human neural system for face perception. Nat Neurosci 2000; 3: 80–84
- Sadeh B, Podlipsky I, Zhdanov A, Yovel G. Event-related potential and functional mri measures of face-selectivity are highly correlated: A simultaneous ERP-FMRI investigation. Hum Brain Mapp 2010; 31: 1490–1501
- Di Russo F, Martinez A, Sereno MI, Pitzalis S, Hillyard SA. Cortical sources of the early components of the visual evoked potential. Hum Brain Mapp 2002; 15: 95–111
- Halit H, de Haan M, Johnson MH. Modulation of event-related potentials by prototypical and atypical faces. Neuroreport 2000; 11: 1871–1875
- Rossion B, Jacques C. Does physical interstimulus variance account for early electrophysiological face sensitive responses in the human brain? Ten lessons on the N170. Neuroimage 2008; 39: 1959–1979
- Mangun GR. Neural mechanisms of visual selective attention. Psychophysiology 1995; 32: 4–18
- Itier RJ, Taylor MJ. Inversion and contrast polarity reversal affect both encoding and recognition processes of unfamiliar faces: A repetition study using ERPs. Neuroimage 2002; 15: 353–372
- Rossion B, Campanella S, Gomez C, Delinte A, Debatisse D, Liard L, et al. Task modulation of brain activity related to familiar and unfamiliar face processing: An ERP study. Clin Neurophysiol 1999; 110: 449–462
- Taylor MJ, Edmonds GE, McCarthy G, Allison T. Eyes first! Eye processing develops before face processing in children. Neuroreport 2001; 12: 1671–1676
- Bentin S, Allison T, Puce A, Perez E, McCarthy G. Electrophysiological studies of face perception in humans. J Cognitive Neurosci 1996; 8: 551–565
- Boutsen L, Humphreys GW, Praamstra P, Warbrick T. Comparing neural correlates of configural processing in faces and objects: An ERP study of the thatcher illusion. Neuroimage 2006; 32: 352–367
- Rossion B, Joyce CA, Cottrell GW, Tarr MJ. Early lateralization and orientation tuning for face, word, object processing in the visual cortex. Neuroimage 2003; 20: 1609–1624
- Goffaux V, Gauthier I, Rossion B. Spatial scale contribution to early visual differences between face and object processing. Cognitive Brain Res 2003; 16: 416–424
- Herrmann M, Ehlis AC, Ellgring H, Fallgatter A. Early stages (p100) of face perception in humans as measured with event-related potentials (erps). J Neural Transm 2005; 112: 1073–1081
- Itier RJ, Taylor MJ. Source analysis of the N170 to faces and objects. Neuroreport 2004; 15: 1261–1265
- Linkenkaer-Hansen K, Palva JM, Sams M, Hietanen JK, Aronen HJ, Ilmoniemi RJ. Face-selective processing in human extrastriate cortex around 120 ms after stimulus onset revealed by magneto-and electroencephalography. Neurosci Lett 1998; 253: 147–150
- Mills G, Khan Z, Moxham J, Desai J, Forsyth A, Ponte J. Effects of temperature on phrenic nerve and diaphragmatic function during cardiac surgery. Br J Anaesth 1997; 79: 726–732
- Starr A, Sininger Y, Winter M, Derebery M, Oba S, Michalewski H. Transient deafness due to temperature-sensitive auditory neuropathy. Ear Hearing 1998; 19: 169–179
- Trojaborg WT, Moon A, Andersen BB, Trojaborg NS. Sural nerve conduction parameters in normal subjects related to age, gender, temperature, and height: A reappraisal. Muscle Nerve 1992; 15: 666–671
- Todnem K, Knudsen G, Riise T, Nyland H, Aarli J. The non-linear relationship between nerve conduction velocity and skin temperature. J Neurol Neurosurg Ps 1989; 52: 497–501
- Dioszeghy P, Stalberg E. Changes in motor and sensory nerve conduction parameters with temperature in normal and diseased nerve. Electroen Clin Neuro 1992; 85: 229–235
- Proverbio AM, Riva F, Martin E, Zani A. Face coding is bilateral in the female brain. PLoS One 2010; 5: e11242
- Rilea SL, Roskos-Ewoldsen B, Boles D. Sex differences in spatial ability: A lateralization of function approach. Brain Cognition 2004; 56: 332–343
- Kemp AH, Silberstein RB, Armstrong SM, Nathan PJ. Gender differences in the cortical electrophysiological processing of visual emotional stimuli. Neuroimage 2004; 21: 632–646
- Nybo L. CNS fatigue provoked by prolonged exercise in the heat. Front Bioscience (Elite). 2010; 2: 779–792