Abstract
Purpose: This study aimed to compare retrospectively bipolar RF ablation with multipolar RF ablation for the treatment of renal cell carcinoma.
Materials and methods: Between March 2009 and June 2012, 12 tumours (nine patients) treated with bipolar RF ablation (one applicator) and 14 tumours (11 patients) treated with multipolar RF ablation (two applicators) were compared systematically. Selection between bipolar RF ablation and multipolar RF ablation was operator choice considering tumour size. Study goals included differences in tumour and coagulation extent, and technical parameters (total RF energy delivery and RF ablation time per coagulation volume).
Results: Tumour maximum diameter was significantly larger for multipolar RF ablation compared with bipolar RF ablation (27.0 mm versus 19.4 mm; p < 0.01). This difference is partially dependent on operator choice. Coagulation length, width and volume were significantly larger for multipolar RF ablation compared with bipolar RF ablation (35.0 mm versus 26.5 mm, 27.5 mm versus 23.0 mm and 14.3 cm3 versus 8.1 cm3; p < 0.01, p < 0.05 and p < 0.05, respectively). Coagulation circularity was not significantly different between both study groups (0.8 versus 0.8; not significant). Total RF energy delivery was significantly higher and RF ablation time per coagulation volume was significantly shorter for multipolar RF ablation compared with bipolar RF ablation (52.0 kJ versus 28.6 kJ and 2.4 min/cm3 versus 4.1 min/cm3; p < 0.05 and p < 0.05, respectively).
Conclusions: Multipolar RF ablation creates a significantly larger coagulation width, but identical coagulation shape, compared with bipolar RF ablation. Additionally, multipolar RF ablation coagulates faster according to the shorter RF ablation time per coagulation volume.
Introduction
Radiofrequency (RF) ablation is common in the treatment of primary and secondary malignancies Citation[1], Citation[2]. Lung, liver, kidney, adrenal and bone are typical for image-guided percutaneous RF ablation. Due to special electrical and thermal conduction properties of tissue (e.g. air-filled spaces in the lung or urine-filled pelvic system in the kidney), the outcome of the different RF ablation technologies may be variable for the different organs Citation[3]. Basically, RF ablation can be performed in monopolar, bipolar or multipolar mode. Monopolar RF ablation uses one or more applicator(s), each with one electrode on the applicator shaft and a neutral electrode on the patient's skin Citation[4]. Thus, the current circuit is closed between the applicator and the neutral electrode. Whereas for bipolar RF ablation, only one bipolar applicator is used, multipolar RF ablation requires at least two bipolar applicators Citation[5]. Each bipolar applicator has two electrodes incorporated. In bipolar and multipolar RF ablation, the current does not cross the body but circulates in the tumour between the electrodes. From the technical point of view, current densities and energy delivery are supposed to be more effective compared with monopolar RF ablation Citation[6]. In experimental works, bipolar and multipolar RF ablation showed better performance with larger and more homogeneous coagulations Citation[6]. In very small series, the use of bipolar applicators showed promising clinical results Citation[7–9]. As distinct from monopolar RF ablation, bipolar and multipolar RF ablation is not established for the treatment of renal cell carcinoma. The purpose of our study was to compare retrospectively bipolar RF ablation with multipolar RF ablation for the treatment of renal cell carcinoma with evaluation of differences in technical and clinical parameters.
Materials and methods
This was a retrospective analysis of bipolar RF ablation (performed with one applicator) and multipolar RF ablation (performed with two applicators) for the treatment of renal cell carcinoma. Between March 2009 and June 2012, 26 consecutive RF ablations of 26 renal cell carcinomas were included. Twelve tumours were treated with bipolar RF ablation, and 14 tumours were treated with multipolar RF ablation. All procedures were performed as single-session treatments. The rate of complications according to the Society of Interventional Radiology classification was low with major and minor complication rates of 0% and 15.4% (two haematomas after bipolar RF ablation as well as one pneumothorax and one haematoma after multipolar RF ablation) Citation[10]. There was no significant deterioration of the renal function after the procedure. Two cases of local recurrence were observed after an imaging follow-up of 14 months (9.5; 23.5), one for bipolar RF ablation and one for multipolar RF ablation. Both cases are addressed in the “Discussion” section below. The study was in accordance with the ethical standards of the responsible committee on human experimentation and with the Helsinki Declaration. All patients gave written informed consent.
Patients
Demographics of the nine patients undergoing 12 bipolar RF ablations included seven men with an average age of 79.3 years (66.0–81.5 years), body mass index of 25.8 kg/m2 (24.0–28.5 kg/m2), three patients with a history of renal cell carcinoma in other locations than the tumours treated in this study, four patients with nephrectomy before RF ablation and six patients with impaired renal function before RF ablation. TNM stage was T1aN0M0 in ten tumours and T1aN0M1 in two tumours diagnosed on dynamic magnetic resonance imaging (MRI). Tumour location was exophytic in seven tumours and intraparenchymal in five tumours. Demographics of the 11 patients undergoing 14 multipolar RF ablations included: eight men, with an average age of 73.2 years (67.5–80.0 years), body mass index of 27.0 kg/m2 (26.1–27.3 kg/m2), three patients with a history of renal cell carcinoma in other locations than the tumours treated in this study, four patients with nephrectomy before RF ablation and five patients with impaired renal function before RF ablation. TNM stage was T1aN0M0 in 11 tumours and T1aN0M1 in three tumours diagnosed on dynamic MRI. Tumour location was exophytic in nine tumours and intraparenchymal in five tumours.
RF ablation procedure
RF ablations were performed under computerised tomography (CT) guidance (Siemens Somatom Definition Open 20, Forchheim, Germany) with local anaesthesia (mepivacaine hydrochloride) and analgosedation (piritramide and midazolam). All procedures were accomplished as planned. A 470-kHz generator RF system (CelonLabPOWER System, Olympus, Berlin, Germany) and internally cooled 16-gauge bipolar applicators (CelonProSurge, Olympus) were used. The generator works in the bipolar mode if one applicator is connected. If two applicators are connected, the procedure is automatically performed in the multipolar mode. All applicators had a straight needle design, whereas the length of the active part varied between 20 and 40 mm. The length of the active part of the applicator was selected individually in such a manner that the active part corresponded to or exceeded the tumour maximum diameter in the vast majority of cases. Selection between bipolar RF ablation and multipolar RF ablation was operator choice considering tumour size. Smaller tumours were treated with bipolar RF ablation, and larger tumours were treated with multipolar RF ablation. As there was a lack of sufficient clinical data, published experimental studies served as the basis for the operator choice. For bipolar RF ablation, a coagulation diameter of 20 mm was reported Citation[11]. For multipolar RF ablation, a coagulation diameter of approximately 30 mm was reported, whereas the distance of the applicators was 13.5 mm Citation[5]. The aim of all interventions was complete tumour destruction, and dependent on the intended coagulation extent, the following parameters were selected case by case: RF ablation mode, length of the active part of the applicator and distance of the applicators. All RF ablations were performed in the power control mode, and power output was stopped automatically when the tissue resistance exceeded 500 Ω Citation[12], Citation[13].
Study goals
Tumour extent
Dynamic MRI was used to determine the tumour extent before RF ablation. According to clinical practice, the diagnosis of renal cell carcinoma was made for a solid tumour with suspect contrast enhancement Citation[8], Citation[14]. Atypical tumours underwent biopsy. On transverse images, the tumour maximum extent was defined as length, and the tumour extent perpendicular to the length was defined as width. On coronal images, tumour maximum extent perpendicular to length and width was defined as depth. Tumour maximum and minimum diameters were defined considering the numerical values for length, width and depth. Tumour volume was calculated as follows Citation[15].
Tumour circularity as index for the tumour shape was determined as follows Citation[16].
Tumour circularity accounts for two dimensions, with “1” for perfect roundness and “<1” for oval shape.
Coagulation extent
Dynamic MRI was used to determine the extent of coagulation within 1 month after RF ablation according to reported criteria Citation[15]. Further MRI controls were scheduled every 6 months. Complete coagulation was defined as hypointense areas at T1w without contrast-enhancement, and uniformly hypointense areas at T2w. Hyperintense areas at T1w on non-enhanced MRI were also observed at the first MRI examination after RF ablation, and rated as regular finding as previously described Citation[17]. On transverse images, the coagulation maximum extent in the applicator shaft direction was defined as length, and the coagulation extent perpendicular to the length was defined as width. On coronal images, the coagulation maximum extent perpendicular to length and width was defined as depth. Coagulation maximum and minimum diameters were defined considering the numerical values for length, width and depth. Coagulation maximum and minimum diameters, coagulation volume and coagulation circularity were analysed in analogy to tumour maximum and minimum diameters, tumour volume and tumour circularity.
Technical data
Length of the active part of the applicator(s), course of the applicator(s) within the renal parenchyma/tumour, tip position of the applicator(s) beyond the tumour margin and distance of the applicators (for multipolar RF ablation only) were analysed. The applicator/tumour sizing index was calculated:
A positive numerical value for the applicator/tumour sizing index indicates oversizing of the length of the active part of the applicator in relation to the tumour maximum diameter. A negative numerical value for the applicator/tumour sizing index indicates undersizing of the length of the active part of the applicator in relation to the tumour maximum diameter. The applicator/coagulation sizing index was calculated as follows.
A positive numerical value for the applicator/coagulation sizing index indicates that the length of the active part of the applicator was larger compared with the coagulation maximum diameter. A negative numerical value for the applicator/coagulation sizing index indicates that the length of the active part of the applicator was smaller compared with the coagulation maximum diameter. Maximum RF power output, total RF ablation time and total RF energy delivery were analysed. RF ablation time per tumour volume, RF energy delivery per tumour volume, RF ablation time per coagulation volume and RF energy delivery per coagulation volume were calculated as follows Citation[13].and
and
and
Statistics
Statistics were performed with a commercially available software package (Prism 4.00, GraphPad Software, La Jolla, CA, USA). Quantitative data is presented as median and percentiles (25th; 75th). Statistical differences between bipolar RF ablation and multipolar RF ablation were analysed with the Mann-Whitney U test. p = 0.05 was regarded as the level of statistical significance. Plots were created using a commercially available software package (Microsoft Excel 14.0.0, Bellevue, WA, USA).
Results
Tumour extent
Detailed data is listed in . Tumour maximum diameter was significantly larger for multipolar RF ablation compared with bipolar RF ablation (27.0 mm versus 19.4 mm; p < 0.01) ( and ). Tumour volume was significantly larger for multipolar RF ablation compared with bipolar RF ablation (9.7 cm3 versus 3.2 cm3; p < 0.01). Tumour circularity was not significantly different between both study groups (0.9 versus 0.9, not significant). Tumour characteristic differences are partially dependent on operator choice and may not relate directly to the RF ablation mode itself.
Figure 1. Clinical example. Bipolar RF ablation was performed in a T1a renal cell carcinoma. (A, B) Dynamic MRI (T2w coronal image and T1w transverse image) for the diagnosis of an exophytic contrast-enhancing renal cell carcinoma in the lower segment of the left kidney (white arrowheads). (C) CT (transverse image) for documentation of correct positioning of the applicator (active tip length of 30 mm) – note the tip of the applicator was positioned slightly beyond the tumour margin. (D, E, F) Dynamic MRI (T2w coronal image, T1w transverse image and T1w coronal image) 22 months after bipolar RF ablation without evidence of local recurrence – note signs of complete tumour destruction with fat tissue necrosis and lack of suspect contrast-enhancement (white arrowheads).
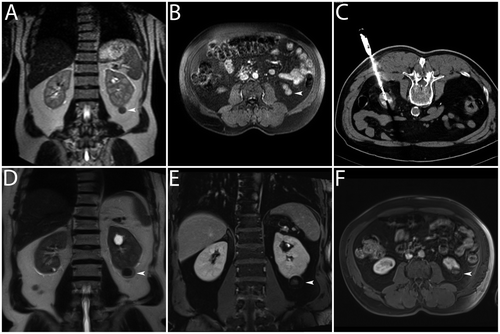
Figure 2. Clinical example. Multipolar RF ablation with two applicators was performed in a T1a renal cell carcinoma. (A, B) Dynamic MRI (T2w coronal image and T1w coronal image) for the diagnosis of a renal cell carcinoma with an exophytic tumour in the lower segment of the right kidney showing peripheral nodular contrast-enhancement (white arrowheads). (C) CT (curved maximum intensity projection) for documentation of correct positioning of the applicators (active tip lengths of 40 mm) – note exact parallel positioning of the applicators with a distance of approximately 20 mm. (D) CT (transverse image) directly after multipolar RF ablation – note regular hyperacute appearance of the coagulation with gas bubbles within the treated tumour (white arrowhead). (E, F) Dynamic MRI (T2w coronal image and T1w coronal image) one month after multipolar RF ablation without evidence of local recurrence – note signs of complete tumour destruction without suspect contrast-enhancement (white arrowheads).
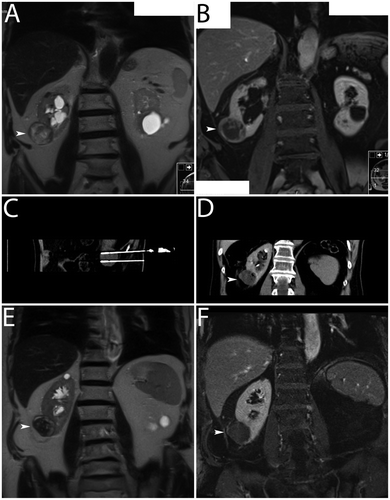
Table I. Tumour and coagulation extent.
Coagulation extent
Detailed data is listed in . Coagulation length, width and volume were significantly larger for multipolar RF ablation compared with bipolar RF ablation (35.0 mm versus 26.5 mm, 27.5 mm versus 23.0 mm and 14.3 cm3 versus 8.1 cm3; p < 0.01, p < 0.05 and p < 0.05, respectively) (, and ). Coagulation circularity was not significantly different between both study groups (0.8 versus 0.8, not significant). Coagulation characteristic differences are procedure-related data, and relate directly to the RF ablation mode itself.
Figure 3. Graphic representation of coagulation extent. (A) Plot of length, width and volume – note there were statistically significant differences between bipolar RF ablation and multipolar RF ablation regarding medians (p < 0.01, p < 0.05 and p < 0.05, respectively). (B) Scatterplot of distance of the applicators versus width for multipolar RF ablation – note dependence of both parameters.
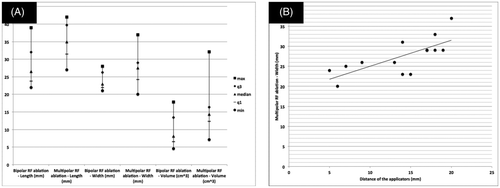
Technical data
Detailed data is listed in . Length of the active part of the applicator was not significantly different between both study groups (30.0 mm versus 30.0 mm, not significant). The corresponding percentiles (25th, 75th), however, suggested a trend to larger length of the active part of the applicator for multipolar RF ablation. Applicator/tumour sizing index and application/coagulation sizing index were not significantly different between both study groups (37.2% versus 15.1% and −11.1% versus −11.7%, not significant, respectively) ( and ). Total RF energy delivery was significantly higher for multipolar RF ablation compared with bipolar RF ablation (52.0 kJ versus 28.6 kJ; p < 0.05). RF ablation time per tumour volume was significantly shorter for multipolar RF ablation compared with bipolar RF ablation (3.2 min/cm3 versus 16.9 min/cm3; p < 0.01). RF ablation time per coagulation volume was significantly shorter for multipolar RF ablation compared with bipolar RF ablation (2.4 min/cm3 versus 4.1 min/cm3; p < 0.01).
Table II. Technical data.
Discussion
The study described herein detected significant differences between bipolar RF ablation (performed with one applicator) and multipolar RF ablation (performed with two applicators) for the treatment of renal cell carcinoma. Coagulation length, width and volume were significantly larger for multipolar RF ablation. Coagulation circularity was not significantly different. There was a trend to larger length of the active part of the applicator for multipolar RF ablation. Applicator/tumour sizing index and applicator/coagulation sizing index were not significantly different. Total RF energy delivery was significantly higher and RF ablation time per coagulation volume was significantly shorter for multipolar RF ablation. Our results refer to the specific devices used, and cannot be translated to compare bipolar RF ablation and multipolar RF ablation in general.
Bipolar RF ablation and multipolar RF ablation is not established. Although some preclinical studies exist, it is not quite clear where this technology may fit in the ablation armamentarium. Häcker et al. performed bipolar RF ablation in ex vivo porcine kidneys Citation[11]. Length of the active part of the applicator (20 mm), RF ablation time (9 min) and maximum power output (20 W), resulting in a coagulation diameter of 20 mm, seem to be inappropriate for the treatment of renal cell carcinoma. In our study, bipolar RF ablation was performed with markedly longer RF ablation time and higher maximum RF power output, but coagulation diameter was barely 20 mm. This may be due to the fact that viable tumour in the perfused kidney was treated. Neuhaus et al. performed detailed histopathological analysis of the coagulation extent in an in vivo porcine kidney study Citation[18]. Multipolar RF ablation resulted in a larger coagulation volume (2.7 cm3) compared with bipolar RF ablation (0.8 cm3). Total RF energy delivery was 15 kJ and 7 kJ, respectively. Our coagulation volume was significantly larger for both RF ablation modes, most likely due to higher total RF energy delivery. Another important finding in the aforementioned study was the coagulation shape. Neuhaus et al. found significantly lower coagulation circularity for multipolar RF ablation compared with bipolar RF ablation, and the authors concluded that more homogeneous ablation could be achieved with bipolar RF ablation. In our study, coagulation shape was identical between both study groups. Higher total RF energy delivery may have compensated for heterogeneous coagulation shapes, especially between both applicators for multipolar RF ablation. In clinical settings, more homogeneous coagulation may result in a reduced rate of incomplete tumour destruction.
For bipolar RF ablation and multipolar RF ablation for the treatment of renal cell carcinoma, only case reports and small series were published. Tacke et al. reported first clinical results for the same RF system we used Citation[9]. They treated successfully one renal cell carcinoma (tumour diameter of 18 mm) applying bipolar RF ablation. After a total RF energy delivery of 58 kJ and a maximum RF power output of 30 W, the coagulation maximum diameter was 30 mm. Salagierski et al. reported two cases of bipolar RF ablation without giving detailed technical parameters Citation[8]. In this study, the operators followed the intuitive strategy to ablate kidney tumours smaller than 30 mm with one applicator, and larger tumours with two applicators at a maximum distance of 10 mm. Boss et al. performed multipolar RF ablation for the treatment of two renal cell carcinomas with tumour volumes of 14.1 cm3 and 37.6 cm3, respectively Citation[7]. For the smaller tumour two applicators were used, and for the larger tumour three applicators were used. Coagulation geometry, however, is not only influenced by the number of applicators but also by the length of the active part of the applicator. In our study the coagulation length was significantly larger for multipolar RF ablation compared with bipolar RF ablation. It is likely that the length of the active part of the applicator itself was the major factor contributing to this observed difference. It seems that coagulation length may have been comparable if applicators with an active length of 30.0 mm were used for all bipolar ablations (). Regarding RF energy delivery per coagulation volume, our study showed almost identical results compared with Boss et al. for the case with multipolar RF ablation with two applicators Citation[7].
Our study is the first systematic evaluation of differences between bipolar RF ablation and multipolar RF ablation with a considerable number of cases. Both cases of local recurrence, however, have to be addressed. There was one case of local recurrence diagnosed 19 months after bipolar RF ablation of a renal cell carcinoma (tumour maximum diameter of 20 mm). Total RF ablation time (63 min) and total RF energy delivery (43.5 kJ) was markedly higher compared with the respective medians of the study group. Since the coagulation zone was only slightly larger compared with the tumour extent, eccentric applicator position in relation to the tumour centre may explain incomplete tumour destruction (). In this context, an adequate safety margin for intraparenchymal renal cell carcinoma with unsharp demarcation may be relevant. The second case of local recurrence occurred 7 months after multipolar RF ablation of a renal cell carcinoma (tumour maximum diameter of 36 mm). Total RF ablation time was 52.3 min and total RF energy delivery was 65.3 kJ. Extrapolation of the medians for RF ablation time per tumour volume and RF energy delivery per tumour volume would result in a total RF ablation time of 69.8 min and a total RF energy delivery of 102.5 kJ. One may speculate whether the applied RF ablation parameters were inadequate for this concrete case. Additionally, the number of applicators (n = 2) and the length of the active part of the applicator (30 mm) may have been suboptimal for the relatively large tumour extent.
Figure 4. Clinical example. Bipolar RF ablation was performed in a T1a renal cell carcinoma. (A) CT (transverse image) for the diagnosis of an intraparenchymal renal cell carcinoma in the upper segment of the right kidney (white arrowhead). (B) CT (transverse image) for documentation of the applicator (active tip length of 20 mm) – note eccentric applicator position in relation to the tumour centre. (C, D) Dynamic CT (transverse images) 19 months after bipolar RF ablation with evidence of local recurrence – note signs of incomplete tumour destruction with suspect contrast-enhancement (white arrowheads).
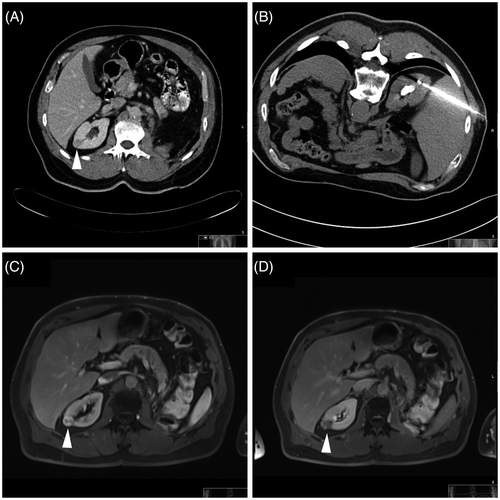
Our results may serve as a baseline observation. Future work is necessary to describe what differentiated bipolar RF ablation and multipolar RF ablation from alternative ablation modalities, namely microwave (MW) ablation, cryoablation and irreversible electroporation (IRE). Potential advantages of multipolar RF ablation include a more precise adaptation of the coagulation zone in relation to the tumour extent. After exact spatial positioning of the appropriate applicators, energy delivery is concentrated within the tumour, and the safety margin may be more predictable. MW ablation has a much broader zone of heating and its transmission in living tissue is not limited Citation[19]. Since dedicated ablation protocols for MW ablation are not established yet, over-ablation and complications may result. According to meta-analyses, cryoablation shows an acceptable oncological outcome at intermediate-term follow-up Citation[20], Citation[21]. Standard cryoablation freeze–thaw–freeze cycle time is half of our 75th percentile for total RF ablation time. Accordingly, cryoablation is also quite time-consuming. Moreover, cryoablation does not coagulate vessels, and the risk of haemorrhage directly after applicator removal is increased compared to heat-based thermal ablation systems with a track ablation mode. IRE is the latest technology for focal tumour ablation. High-voltage and high-current electrical pulses applied via percutaneous applicators induce irreversible nanopores within the cell membrane Citation[22]. This results in disturbed cell homeostasis, followed by controlled cell death (apoptosis). Drawbacks of this technology are the need for multiple applicators, general anaesthesia and full relaxation as well as unknown oncologic long-term results Citation[23], Citation[24].
A limitation of our study is the small number of tumours and mid-term follow-up. Moreover, there was no randomisation, and some of the presented data may be partially dependent on operator choice, and may not relate directly to the RF ablation mode itself. The role of multipolar RF ablation with more than two applicators is unclear. A prospective study including larger tumours may give additional information for when and how to use multiple applicators. Finally, bipolar RF ablation and multipolar RF ablation was not compared with alternative ablation technologies, and it is still not quite clear where this technology fits in the ablation armamentarium.
Conclusion
Multipolar RF ablation creates a significantly larger coagulation width, but identical coagulation shape, compared with bipolar RF ablation. Additionally, multipolar RF ablation coagulates faster according to the shorter RF ablation time per coagulation volume.
Declaration of interest: P.L.P. received grants from Celon AG (Celon AG Olympus, Berlin, Germany). The authors alone are responsible for the content and writing of the paper.
References
- Carraway WA, Raman JD, Cadeddu JA. Current status of renal radiofrequency ablation. Curr Opin Urol 2009; 19: 143–147
- Kim KH, Yoon YS, Yu CS, Kim TW, Kim HJ, Kim PN, et al. Comparative analysis of radiofrequency ablation and surgical resection for colorectal liver metastases. J Korean Surg Soc 2011; 81: 25–34
- Gillams A. Tumour ablation: Current role in the kidney, lung and bone. Cancer Imaging 2009; 9: 68–70
- Nakada SY, Jerde TJ, Warner TF, Wright AS, Haemmerich D, Mahvi DM, Lee FT, Jr. Bipolar radiofrequency ablation of the kidney: Comparison with monopolar radiofrequency ablation. J Endourol 2003; 17: 927–933
- Hacker A, Vallo S, Weiss C, Stein T, Alken P, Knoll T, Michel MS. Technical characterization of a new bipolar and multipolar radiofrequency device for minimally invasive treatment of renal tumours. BJU Int 2006; 97: 822–828
- Haemmerich D, Wright AW, Mahvi DM, Lee FT, Jr, Webster JG. Hepatic bipolar radiofrequency ablation creates coagulation zones close to blood vessels: A finite element study. Med Biol Eng Comput 2003; 41: 317–323
- Boss A, Rempp H, Martirosian P, Clasen S, Schraml C, Stenzl A, et al. Wide-bore 1.5 Tesla MR imagers for guidance and monitoring of radiofrequency ablation of renal cell carcinoma: Initial experience on feasibility. Eur Radiol 2008; 18: 1449–1455
- Salagierski M, Salagierska-Barwinska A, Sosnowski M. Percutaneous ultrasound-guided radiofrequency ablation for kidney tumors in patients with surgical risk. Int J Urol 2006; 13: 1375–1379
- Tacke J, Mahnken A, Roggan A, Günther RW. Multipolar radiofrequency ablation: First clinical results. RöFo 2004; 176: 324–329
- Sacks D, McClenny TE, Cardella JF, Lewis CA. Society of Interventional Radiology clinical practice guidelines. J Vasc Interv Radiol 2003; 14: 199–202
- Hacker A, Risse F, Peters K, Fink C, Weiss C, Huber PE, et al. Magnetic resonance imaging for assessment of radiofrequency lesions in kidney tissue immediately after ablation: An experimental study. J Endourol 2006; 20: 312–317
- Clasen S, Rempp H, Schmidt D, Schraml C, Hoffmann R, Claussen CD, Pereira PL. Multipolar radiofrequency ablation using internally cooled electrodes in ex vivo bovine liver: Correlation between volume of coagulation and amount of applied energy. Eur J Radiol 2012; 81: 111–113
- Clasen S, Schmidt D, Dietz K, Boss A, Kröber SM, Schraml C, et al. Bipolar radiofrequency ablation using internally cooled electrodes in ex vivo bovine liver: Prediction of coagulation volume from applied energy. Invest Radiol 2007; 42: 29–36
- Ferakis N, Bouropoulos C, Granitsas T, Mylona S, Poulias I. Long-term results after computed-tomography-guided percutaneous radiofrequency ablation for small renal tumors. J Endourol 2010; 24: 1909–1913
- Awad MM, Devgan L, Kamel IR, Torbensen M, Choti MA. Microwave ablation in a hepatic porcine model: Correlation of CT and histopathologic findings. HPB (Oxford) 2007; 9: 357–362
- Laeseke PF, Lee FT, Jr, Sampson LA, van der Weide DW, Brace CL. Microwave ablation versus radiofrequency ablation in the kidney: High-power triaxial antennas create larger ablation zones than similarly sized internally cooled electrodes. J Vasc Interv Radiol 2009; 20: 1224–1229
- Kawamoto S, Solomon SB, Bluemke DA, Fishman EK. Computed tomography and magnetic resonance imaging appearance of renal neoplasms after radiofrequency ablation and cryoablation. Semin Ultrasound CT MR 2009; 30: 67–77
- Neuhaus J, Blachut L, Rabenalt R, Stein T, König F, Wehner M. Efficiency analysis of bipolar and multipolar radiofrequency ablation in an in vivo porcine kidney model using three-dimensional reconstruction of histologic section series. J Endourol 2011; 25: 859–867
- Feng B, Liang P. Local thermal ablation of renal cell carcinoma. Eur J Radiol 2012; 81: 437–440
- Kutikov A, Kunkle DA, Uzzo RG. Focal therapy for kidney cancer: A systematic review. Curr Opin Urol 2009; 19: 148–153
- Venkatesan AM, Wood BJ, Gervais DA. Percutaneous ablation in the kidney. Radiology 2011; 261: 375–391
- Deodhar A, Monette S, Single GW, Jr, Hamilton WC, Jr, Thornton R, Maybody M, et al. Renal tissue ablation with irreversible electroporation: Preliminary results in a porcine model. Urology 2011; 77: 754–760
- Pech M, Janitzky A, Wendler JJ, Strang C, Blaschke S, Dudeck O, et al. Irreversible electroporation of renal cell carcinoma: C first-in-man phase I clinical study. Cardiovasc Intervent Radiol 2011; 34: 132–138
- Davalos RV, Mir IL, Rubinsky B. Tissue ablation with irreversible electroporation. Ann Biomed Eng 2005; 33: 223–231