Abstract
Purpose: In order to investigate the effects of hyperthermia conditions on human cognitive performance, functional magnetic resonance imaging (fMRI) was employed to investigate human brain activation during visual short-term memory (VSTM) tasks under modulated hyperthermia conditions.
Materials and methods: A total of 38 right-handed healthy young male participants were randomly divided into control and hyperthermia groups who underwent VSTM tasks by using block design.
Results: Compared with that of the control group, the reaction time of the hyperthermia group was longer in VSTM tasks (p = 0.019), but there was no significant difference between the two groups for the accuracy rate (p > 0.85). The fMRI data showed that the processing advantage of the right hemisphere was notably enhanced and that activity strength of the bilateral dorsolateral prefrontal cortex and right intra-parietal sulcus were significantly enhanced in the hyperthermia group.
Conclusions: These data provided neuroimaging evidence of the effect of hyperthermia conditions on the brain regions and also proved heat load decreases cognitive performance.
Introduction
The occupational heat stress exposure, such as firefighters or miners might undergo, for example, has been observed to be detrimentally affected by hyperthermia conditions [Citation1]. Passive heat exposure for a longer time could cause cognitive performance decrement by disturbing the central nervous system [Citation2–4]. In addition, electrophysiological studies showed that prolonged physical exercise in the heat slowed prefrontal electroencephalography (EEG) activity (e.g. a rise in the α/β index) [Citation5] and that passive heat exposure for a long time could damage pre-attentive processing reflected by mismatch negativity (MMN) of event-related potentials [Citation6].
As we know, when participants carry out various kinds of tasks, the metabolic activity of brain and regional cerebral blood flow in specific brain regions will be observed. Up to now, there has been little attempt to examine the perfusion changing of the brain regions when people were exposed to hyperthermia conditions with MR. In the present study, we developed a heat cabin and heat suit which would simulate a hyperthermia environment and maintain body temperature to carry out fMRI examination.
We will investigate the effect on the activation of the brain regions using visual short-term memory (VSTM) tasks under hyperthermia conditions, and this task has been successfully carried out in previous experiments. VSTM, a critical component of working memory, sustains the information on short-term storage and maintenance for the objects spatial location and their spatial relationships between objects [Citation7]. Over the last decade, various neuroimaging studies have confirmed that several regions such as the frontal/prefrontal, parietal and occipital cortices are involved in VSTM [Citation8–13]. For example, Todd and Marois [Citation9] have reported that the maintenance of information in VSTM is supported by the inferior intra-parietal sulcus (IPS) and intra-occipital sulcus (IOS). Similarly, Xu and colleagues [Citation12] have found that activations within these cerebral regions correlated most strongly with the amount of information held in the memory. Also, Grimault and colleagues [Citation13] have shown that oscillatory activity in the dorsolateral prefrontal cortex (DLPFC) in their magnetoencephalography (MEG) study. Furthermore, previous studies have shown that the right parietal lobe plays a very important role in processing of visual space conversion [Citation9,Citation14].Thus, in order to evaluate and extract fMRI responses, we defined that the brain regions such as DLPFC, IPS and IOS as the regions of interest (ROIs). We sought to examine brain activity changes in the three regions mentioned above in VSTM tasks during hyperthermia versus control conditions based on previous studies.
In hyperthermia conditions, participants require higher ability of reaction and spatial positioning, whereby, when the neural resources are insufficient to deal with both the tasks and thermal stress, the cognitive performance and activities will deteriorate [Citation15,Citation16]. Following this hypothesis, we thought the activity strength of brain regions may vary during the same tasks in the hyperthermia conditions, or as heat load increased, the scope of activation brain areas may be changed. However, the changing of the activation of brain regions is still unclear under hyperthermia conditions. Therefore, our study attempts to examine the scope of brain activation areas and further detect the change of activation intensity under hyperthermia conditions.
Materials and methods
Participants
In total 38 right-handed healthy young male volunteers (age range 20–29 years, mean age 24 ± 2.58 years) were enrolled in the test. All of the participants reported normal vision and had no history of current or past neurological or psychiatric illness and took no medications known to affect the central nervous system. They signed an informed consent form approved by the Ji Nan Ethical Committee and were paid for their participation. All the participants were randomly divided into two groups, a control group and a hyperthermia group.
Hyperthermia group: The fMRI was performed after all the participants were kept sitting quietly in the environmental chamber (50 °C, relative humidity 40%) for 30 min. In the fMRI trials, before the participants entered the MRI room, a set of self-developed special devices, a thermal heated suit (50 °C) with a protective layer (between the skin and the heating pipe) and a nose and mouth mask supplying ventilation (air set at 50 °C and 40% relative humidity) was used to simulate the passive heat environment while the temperature in the MRI room was only 21.5° ∼ 21.6 °C. The fMRI procedure lasted for about 11 min.
Control group: The procedure was mostly the same as the hyperthermia trial except that the condition of the room was set at 22 °C and 40% relative humidity respectively. Participants of the control group also wore the special device in order to keep the same environments as that of the hyperthermia group during the scanning.
Body weight and rectal temperature recording
Rectal temperatures were monitored by thermometer (ZWJ-2, Tianjin Honour Instruments Co., Ltd, Tianjin, China). Both the rectal temperatures and body weight data were recorded before and after each test session in the control and hyperthermia group.
Functional MRI data acquisition
The blood oxygen level-dependent (BOLD) fMRI measurements were obtained with a whole-body, 1.5 T Signa HD dual gradient scanner (GE Medical, Milwaukee, WI, USA). Each scan consisted of 114 contiguous EPI functional volumes (repetition time (TR) = 3000 ms; echo time (TE) = 40 ms; flip angle (FA) = 90°; slice thickness = 5 mm, space = 0; matrix = 64 × 64; FOV = 240 × 240 mm2). Additionally, a high resolution T1-weighted sequence was obtained: TR = 11.1 ms; TE = 4.9 ms; FA = 20°; slice thickness = 1.4 mm, space = 0; FOV = 240 × 240 mm; matrix 256 × 256.
fMRI stimuli and procedure
The stimuli were presented in separate blocks with a modified one-back paradigm. All stimuli pictures were presented with three spatial units lighting pseudo-randomly in the rectangular area which was composed of an array of spatial position: 3 × 3 (horizontal/vertical viewing 4°). Each trial began with target stimuli (600 ms), and probe stimuli for 1400 ms alternately with an inter-stimulus interval (ITI) of 1000 ms. Participants responded ‘yes’ or ‘no’ to indicate whether the probe was identical to the target, followed by an 800-ms blank delay period. It was designed so that 50% that the probe pictures were identical to the target pictures. One run contained 60 trials and each participant was tested for 1 run (). Each block lasted 30 s and consisted of 10 pairs of stimuli per trial. All stimuli pictures were projected on the screen positioned at the head side of participants via a laser projector. Participants were asked to look at the screen through the mirror attached to the head coil and requested to stare at on the cross shown on the screen during the first 12-s dummy scan and resting-state blocks.
Figure 1. Stimulus pattern for fMRI block design. There was a 12 s dummy scan, and 30 s in the beginning of the run when participants were asked to only fix on the cross point and no task was required, followed by stimulus images. Participants judged whether the probe matched the target in the sample display with the whole process being limited to 30 s. Another fixation followed was continued 30 s. The programme mentioned above went on alternately for five blocks with a dummy scan of 30 s as the ending. The total time of the test was 5 min 42 s.
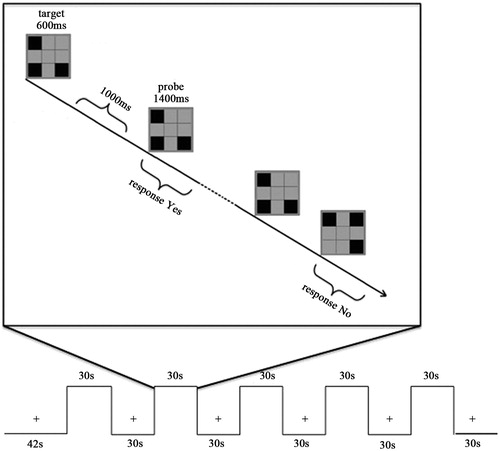
Data analysis
Body weight and rectal temperature
Body weight and rectal temperature acquisition were performed with SPSS 17.0 software. A two-way within-subject analysis of variance (ANOVA) for repeated measures (Time versus Group) was used to assess the main effects of the Group (control or hyperthermia) and Time (previous or post-experiment) with regard to changes in rectal temperatures and body weight.
Behavioural data
Behavioural data, acquisition was performed with SPSS 17.0 software. Participants’ responses (including response time and accuracy) were recorded for analysis. Behavioural data that met with the following criteria were discarded: completion rate and accuracy rate <85%. After invalid and odd data outside 2.0 standard deviation were removed, the difference between groups of reaction time and accuracy was calculated with Greenhouse-Geisser correction (p < 0.05).
fMRI data processing
Brain Voyager QX software (Brain Innovation, Maastricht, the Netherlands) was used in data analysis. The following pre-processing steps were applied: slice scan timing correction, 3D motion correction and temporal filtering (high pass filter 0.006 Hz). Data with all participants’ head motion more than 1 mm or rotation more than l.5° were discarded. Participants who were in accordance with the standard above were as follows. Hyperthermia group: 14 participants (mean age 23 ± 2.46 years); control group: 14 participants (mean age 25 ± 2.05 years). The functional data were then aligned with the anatomical data and transformed into the Talairach space. The voxel size of functional data was resampled to 1 × 1 × 1 mm3. By using a multiple regression test based on a general linear model, the statistical activation maps were generated with the activation threshold range being limited to 10 voxels, while the statistical probability threshold was set to p < 0.01.
ROI analysis
We defined DLPFC, IPS and IOS as the ROIs based on earlier studies using the automated anatomical labelling of activations atlas (AAL) [Citation17] and the Brodmann area atlas. The DLPFC region is relatively difficult to anatomically delimit. Thus, in order to define the ROI as precisely as possible, the DLPFC region was generated by intersecting the BA9 in the Brodmann area atlas and the middle frontal gyrus in the AAL atlas. The IPS and IOS region were identified using the AAL atlas. Each individual participant’s data was extracted from the ROIs and computed for every subject using the same coordinate (detailed coordinate of each ROI can be seen in ). The activity strength for single voxels of all areas was submitted to t-test between the control and hyperthermia groups. A lateralisation index of brain response strength LI = (S-right − S-left)/(S-right + S-left) was calculated.
Table I. Intensity of DLPFC, IPS, IOS activation in ROI.
Results
Body weight and temperature
Compared to control condition, rectal temperatures were significantly increased (37.25 ± 0.33 °C and 38.26 ± 0.37 °C for control and heat conditions, respectively; p < 0.001). The body weight loss was controlled less than 1% (0.086 ± 0.21 kg and 0.69 ± 0.34 kg for control and heat conditions, respectively; p < 0.001) and did not differ between the two groups before the experiment (68.26 ± 8.15 kg and 67.19 ± 2.46 kg, p = 0.84).
Behavioural data
Compared with the control group (939 ± 22 ms), the reaction time in the present task for hyperthermia group was significantly longer (953 ± 14 ms, p = 0.019), whereas there was no significant difference for the accuracy rate (94% and 92% for control and hyperthermia groups, respectively; p > 0.85).
Data of fMRI
In the control and hyperthermia groups, activations in VSTM tasks were observed in bilateral DLPFC, IPS and IOS while activity strength showed significant group differences ( and ). Two-sample t-test analysis revealed that the activity strength increased more significantly than that of control group in the left DLPFC (t = −3.55, p < 0.002), the right DLPFC (t = −3.66, p < 0.001) and the right IPS (t = −2.08, p < 0.048). In the other areas, there were no significant group differences (ps > 0.1). The activity strength of DLPFC, IPS and IOS activation in ROI is shown in .
Figure 2. The statistical parametric map of an axial slice of the brain shows activation inversely proportional to visual short-term memory load in the bilateral DLPFC, IPS and IOS. The maps of upper row represented the control group and the lower row represented the hyperthermia group. Z, z axial in Talairach-Tournoux coordinates; T, t value; A, anterior; P, posterior; L, left; R, right.
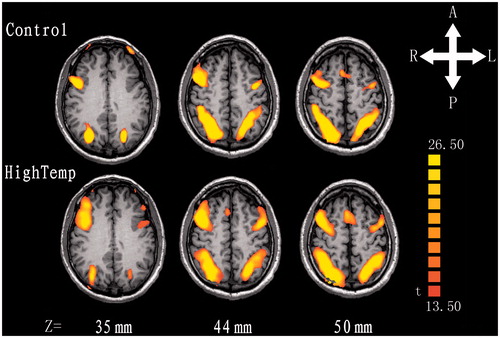
Figure 3. Activations were significantly enhanced in the bilateral dorsolateral prefrontal areas and right parietal lobe compared with that at regular temperature, and others were not significant between control and hyperthermia groups. Regions of interest for DLPFC, IPS and IOS are also shown, respectively.
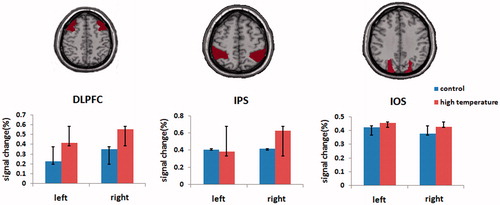
Based on the calculation of the lateralisation index (LI) of brain response strength, the right hemisphere processing advantage (e.g. LI = 0.07; the right DLPFC, t = −2.47, p = 0.021) was shown under control condition, while the advantage was enhanced significantly (LI = 0.16) after heat exposure.
Discussion
The behavioural tests in the present study demonstrated impairments in VSTM performance over a long period (>40 min) of heat exposure. Compared to the control group, reaction time of the hyperthermia group was prolonged significantly in VSTM tasks, indicating that the present hyperthermia impaired visual short memory function. Previous studies have confirmed that impairments in cognitive function with passive hyperthermia are task dependent [Citation18]. Complex cognitive functions such as short-term memory, working memory, vigilance performance, and visual recognition were impaired by hyperthermia [Citation19,Citation20], whereas simple functions such as reaction time and mental transformation tasks were not [Citation21]. In the present study, participants were assigned to VSTM tasks requiring a capacity of high state of vigilance, cooperation and coordination to overcome possible negative effects of thermal stress that can result in a significant impairment of cognitive function.
In the current study, activation of brain areas in the hyperthermia group did not exceed the scope of the control group, that is, there was no evidence that heat load led to additional brain activity regions during hyperthermia conditions compared to control. According to the ROI analysis, however, an increase in activity strength was observed in bilateral DLPFC and right IPS at high temperatures. Previous studies reported that increased brain electrical activity (frontal regions, occipito-parietal regions) was associated with the increasing of the core temperature, which is similar to the present studies’ results [Citation16]. Combined with the impaired behavioural performance in the present fMRI study, we might speculate that the increase of neural activity in the above regions could be due to the more occupation of cognitive resources (such as attention or emotional response) to complete VSTM tasks.
Our study revealed that the right hemisphere (DLPFC) processing advantage (RHPA) was shown under control conditions. Furthermore, on the basis of the increased activity strength of the right DLPFC, the RHPA was significantly enhanced after heat exposure. Previous studies have reported that activation intensity of brain areas was predominant in the right hemisphere in the working memory task [Citation22,Citation23], and the right DLPFC played a very important role in manipulating and organizing information [Citation10,Citation14,Citation24]. However, Reuter-Lorenz [Citation25] found that RHPA in the right prefrontal lobe in VSTM disappeared when human beings got older. He proposed that this reflected the functional compensation of the left side due to the decline in nervous system function. Different from the aging effect, the enhanced RHPA under hyperthermia condition could reflect the hyperfunction of this area in order to validly conduct the task.
Heat load, as mentioned above, increased the activity strength in the bilateral DLPFC and right IPS. Similarly, it was also observed that increasing memory load led to activation of the posterior parietal/superior occipital cortex in VSTM tasks [Citation11,Citation24,Citation26]. Previous studies have reported that the posterior parietal cortex was involved in the storage of visuospatial information and the occipital cortex may contribute to individual differences in VSTM capacity [Citation24,Citation27]. Meanwhile, the frontal/prefrontal cortices play an important role in maintain and organise information in VSTM performance [Citation10]. Thus, different from memory load, in order to maintain their performance level, the corresponding brain regions may pay more energy particularly in the bilateral DLPFC and right IPS for storage, control and maintenance information under heat load. In addition, no compensation of other brain regions was observed in our study; therefore, the reaction to heat load may have come about by the increasing activation in the corresponding brain regions in VSTM processing.
In summary, this study examined the effect of hyperthermia conditions on the activation of the brain regions using the VSTM tasks. Behavioural data showed that the heat stress prolonged the reaction time in VSTM tasks. For the first time it is possible to make a connection between behaviour change of cognitive function and the neural activity of specific brain regions under heat stress using fMRI. More specifically, the bilateral DLPFC, right IPS processing advantage came out under hyperthermia conditions.
Declaration of interest
The authors report no conflicts of interest. The authors alone are responsible for the content and writing of the paper.
References
- Ramsey JD, Burford CL, Beshir MY, Jensen RC. Effects of workplace thermal conditions on safe work behavior. J Safety Res 1983;14:105–14
- Mustafa KY, Aneja IS, Khogali M, Nasreldin A, Arar I. Effect of hyperthermia on brain auditory evoked potentials in the conscious sheep. Electroencephalogr Clin Neurophysiol. 1988;71:133–41
- Grandjean AC, Grandjean NR. Dehydration and cognitive performance. J Am Coll Nutr 2007;26:549S–54S
- Sun G, Yang X, Jiang Q, Liu K, Li B, Li L, et al. Hyperthermia impairs the executive function using the Attention Network Test. Int J Hyperthermia 2012;28:621–6
- Nybo L, Nielsen B. Hyperthermia and central fatigue during prolonged exercise in humans. J Appl Physiol 2001;91:1055–60
- Sun G, Li L, Li M, Jiang Q. Hyperthermia impaired pre-attentive processing: An auditory MMN study. Neurosci Lett 2011;502:94–8
- Baddeley A. Working memory. Curr Biol 2010;20:R136–140
- Linden DE, Bittner RA, Muckli L, Waltz JA, Kriegeskorte N, Goebel R, et al. Cortical capacity constraints for visual working memory: Dissociation of fMRI load effects in a fronto-parietal network. Neuroimage 2003;20:1518–30
- Todd JJ, Marois R. Capacity limit of visual short-term memory in human posterior parietal cortex. Nature 2004;428:751–4
- Curtis CE, D’Esposito M. Persistent activity in the prefrontal cortex during working memory. Trends Cogn Sci 2003;7:415–23
- Sheremata SL, Bettencourt KC, Somers DC. Hemispheric asymmetry in visuotopic posterior parietal cortex emerges with visual short-term memory load. J Neurosci 2010;30:12581–8
- Xu Y, Chun MM. Dissociable neural mechanisms supporting visual short-term memory for objects. Nature 2006;440:91–5
- Grimault S, Robitaille N, Grova C, Lina JM, Dubarry AS, Jolicoeur P. Oscillatory activity in parietal and dorsolateral prefrontal cortex during retention in visual short-term memory: Additive effects of spatial attention and memory load. Hum Brain Mapp 2009;30:3378–92
- Harris IM, Egan GF, Sonkkila C, Tochon-Danguy HJ, Paxinos G, Watson JD. Selective right parietal lobe activation during mental rotation: A parametric PET study. Brain 2000;123:65–73
- Hancock PA, Vasmatzidis I. Effects of heat stress on cognitive performance: The current state of knowledge. Int J Hyperthermia 2003;19:355–72
- Hocking C, Silberstein RB, Lau WM, Stough C, Roberts W. Evaluation of cognitive performance in the heat by functional brain imaging and psychometric testing. Comp Biochem Physiol A Mol Integr Physiol 2001;128:719–34
- Tzourio-Mazoyer N, Landeau B, Papathanassiou D, Crivello F, Etard O, Delcroix N, et al. Automated anatomical labeling of activations in SPM using a macroscopic anatomical parcellation of the MNI MRI single-subject brain. Neuroimage 2002;15:273–89
- Gaoua N, Racinais S, Grantham J, El Massioui F. Alterations in cognitive performance during passive hyperthermia are task dependent. Int J Hyperthermia 2011;27:1–9
- Cian C, Barraud PA, Melin B, Raphel C. Effects of fluid ingestion on cognitive function after heat stress or exercise-induced dehydration. Int J Psychophysiol 2001;42:243–51
- Racinais S, Gaoua N, Grantham J. Hyperthermia impairs short-term memory and peripheral motor drive transmission. J Physiol 2008;586:4751–62
- Amos D, Hansen R, Lau WM, Michalski JT. Physiological and cognitive performance of soldiers conducting routine patrol and reconnaissance operations in the tropics. Mil Med 2000;165:961–6
- Smith EE, Jonides J. Working memory: A view from neuroimaging. Cogn Psychol 1997;33:5–42
- McCarthy G, Puce A, Constable RT, Krystal JH, Gore JC, Goldman-Rakic P. Activation of human prefrontal cortex during spatial and nonspatial working memory tasks measured by functional MRI. Cereb Cortex 1996;6:600–11
- Todd JJ, Marois R. Posterior parietal cortex activity predicts individual differences in visual short-term memory capacity. Cogn Affect Behav Neurosci 2005;5:144–55
- Reuter-Lorenz PA, Jonides J, Smith EE, Hartley A, Miller A, Marshuetz C, et al. Age differences in the frontal lateralization of verbal and spatial working memory revealed by PET. J Cogn Neurosci 2000;12:174–87
- Magen H, Emmanouil TA, McMains SA, Kastner S, Treisman A. Attentional demands predict short-term memory load response in posterior parietal cortex. Neuropsychologia 2009;47:1790–8
- Robitaille N, Grimault S, Jolicoeur P. Bilateral parietal and contralateral responses during maintenance of unilaterally encoded objects in visual short-term memory: Evidence from magnetoencephalography. Psychophysiology 2009;46:1090–9