Abstract
Purpose: Collateral thermal injury can occur as a serious complication of microwave ablation. This study aimed to assess the insulating effect of a thermosensitive, chitosan-based hydrogel during the percutaneous microwave ablation of liver tissue abutting the stomach. Materials and methods: Thermometry needles (R1–R4) were positioned at 5-mm intervals from a thermal source to measure in vitro the temperature differences due to the hydrogel (R1 closest to the thermal source). Subsequently, two groups of eight rabbits each were injected with 10 mL of hydrogel solution or 410 ± 95 mL of 5% saline between the liver and stomach wall. A control group of eight rabbits received no ablation protection measures. The livers were ablated with 40 W for 300 s in 24 ablation zones. The severity of thermal injury to the stomach wall was assessed histologically. Results: In vitro, the mean maximum temperature difference between the R1 and R2 thermometry needles was 31.3° ± 0.1 °C. When R1 was over 60 °C, the mean temperatures at R2, R3, and R4 were 29.8° ± 0.1 °C, 18.6 ± 0.3 °C, and 18.1° ± 0.1 °C, respectively. After ablation for 300 s, the maximum temperature at R2 was 48.7° ± 0.2 °C. None of the rabbits injected with gel showed any injury after ablation; however, the other two groups showed varying degrees of thermal injury. Conclusion: The in situ gel protected the adjacent stomach wall from injury during percutaneous microwave ablation of liver tissue. Although the present technique appears promising, further studies are necessary prior to clinical application.
Introduction
Surgical candidacy for the excision of liver tumours is dependent on the size, number, location, and distribution of tumours [Citation1]. Local interventional therapies, including percutaneous tumour ablation, are therefore important in the treatment of patients with hepatocellular carcinoma (HCC) [Citation2,Citation3]. Microwave ablation (MWA) has shown high thermal efficiency and good long-term efficacy in the treatment of liver cancer, and minimally invasive ultrasound-guided percutaneous microwave coagulation therapy (PMCT) is increasingly used to treat patients with HCC. The underlying basis of this method is the induction of coagulation necrosis through treating the tumours at 54 °C for 3 min or by immediate heating to 60 °C [Citation4].
In the treatment of HCC, a very low energy level can result in incomplete ablation and local tumour progression. However, the failure to achieve successful ablation can be prevented by expanding the ablation range to 0.5–1.0 cm around the tumour. By contrast, very high energy levels can lead to the ablation zone temperature reaching 100 °C, thereby resulting in thermal injury to areas adjacent to the liver tumours, including the gallbladder, diaphragm, gastrointestinal tract, and bile duct because of heat conductance from the ablation site [Citation4,Citation5]. The incidence of severe complications after thermal ablation has been reported to be 2.2–8.9%, and several studies have observed an incidence of 0.2–0.3% in the occurrence of intestinal perforation resulting from ablation [Citation5,Citation6]. A mortality rate of 0.1–1.4% has also been reported [Citation4,Citation5,Citation7]. Of importance, 15% of liver tumours deemed as high risk are not suitable for thermal ablation [Citation8,Citation9]. For reducing the incidence of complications and the mortality rate, invasive artificial ascites [Citation8–12] have been used during the ablation of subcapsular hepatic lesions in order to minimise collateral thermal injury to adjacent organs [Citation8,Citation9]. However, the primary limitations of artificial ascites appear to be peritoneal adhesion due to previous treatments (surgical resection, transcatheter arterial chemoembolization (TACE), or thermal ablation) and the treatment of tumours in the bare area of the liver [Citation12,Citation13].
Chitosan is an amino-polysaccharide obtained from the alkaline deacetylation of chitin, an industrial by-product of the exoskeleton of shrimp and crabs [Citation14], and is an important natural polymer widely used in medical and pharmaceutical applications [Citation15]. Although chitosan derivatives may be useful in PMCT, their insulation effects must still be evaluated prior to their use in this procedure. Hence, the present study aimed to evaluate both in vitro and in vivo the insulating properties of a thermosensitive chitosan-based hydrogel during the percutaneous microwave ablation of liver tissue abutting the stomach in rabbits.
Materials and methods
Drugs
Chitosan (degree of deacetylation 91%, Mw, 292 000) was obtained from Sigma-Aldrich (St Louis, MO, USA), β-glycerophosphate (β-GP), from Sinopharm Reagent (Shanghai, China), carboxymethyl cellulose sodium (Molecular weight (MW), 250 000, degree of substitution 0.80–0.95), from Beijing Chemical Reagent Company; polyvinyl chloride (PVC) powder (MW, 43 000), from Beijing Chemical Plant, and the ultrasound contrast reagent Sonovue, from Bracco (Milano, Italy).
Chitosan thermosensitive hydrogel
The chitosan/GP solution was prepared as described previously [Citation16]. Briefly, 200 mg of chitosan was dissolved in 9 mL of 0.1 N HCl, and 800 mg of β-GP was dissolved in 1 mL of distilled water. Both solutions were cooled in an ice bath for 15 min, and the β-GP solution was added dropwise to the chitosan solution with continuous stirring, followed by stirring for an additional 10 min to obtain a clear and homogeneous chitosan/β-GP solution ().
Figure 1. A demonstration of the gelling of the chitosan preparation. (a) In solution, (b) gelling after inversion, (c) photograph showing a 5-mm thick gel (black arrow) located between R1 and R2 and 5 mm from the microwave ablation needle fissure (red arrows). For interpretation of the references to colour in this figure legend, please refer to the web version of this article at informahealthcare.com.
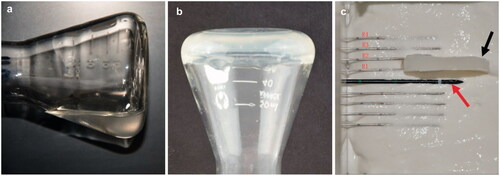
Microwave phantoms
The microwave phantoms (density, 1070 kg/m3; specific heat, 3700 J kg−1 °C−1), developed by Sichuan University, were composed of 3.5% sodium carboxymethyl cellulose, 25.95% PVC, 0.55% NaCl, and 70% water, with a dielectric constant (0.535 J m−1 s−1 °C−1) similar to that of liver tissue in a microwave field. The phantoms were prepared by mixing 105 g of sodium carboxymethyl cellulose (Beijing Chemical Reagent Company) with 778.5 g of polyvinyl chloride powder (Beijing Chemical Plant), to which 116.5 g of NaCl dissolved in 2100 g of water was slowly added, followed by continuous stirring for 3–5 min until it condensed into a white jelly-like microwave phantom.
Temperature measurement
The thermocouple device consisted of an iron–copper thermocouple wire, 0.0762 in diameter, with one end of each wire connected to an HP 34970A 16-channel multi-function data acquisition device (Agilent, Hewlett Packard, Englewood, United States), with 6 half-resolutions (22-bit), 0.004% basic DC voltage accuracy and low noise, and scan rates of up to 250 channels/s. This device was connected via an RS-232 interface to a computer, which was operated using an Agilent BenchLink Data Logger software configuration for data analysis and management.
Microwave ablation instrument
A KY-2000 water-cooled microwave ablation instrument was utilised (Nanjing Kang Yougong Secretary). This instrument provided a single-guide, single-source microwave emission at a microwave frequency of 2450 MHz and an output power of 1–l00 W. The water-cooling cycle was 40 mL/min. We utilised Model T11 and T5 (with an outer diameter of 15 G) microwave ablation needles, with distances of 11 mm and 5 mm from the front end of the gap to the tip, respectively.
In vitro experimental methods
The microwave ablation needle was placed horizontally, with the gaps as the origin of the coordinates. The horizontal and vertical directions of the microwave antenna T11 were defined as the X and Y axes, respectively. The thermometry needles R1, R2, R3, and R4 were placed in parallel with the X axis; the distal end of the needles were flush with the antenna gap at distances of 5 mm, 10 mm, 15 mm, and 20 mm along the Y axis from the origin. A film 5 mm in thickness was placed between R1 and R2 (). Microwaves were applied six times at 50 W for 300 s. The temperatures of R1 and R2 were measured every 30 s and used to draw a temperature difference curve (). Moreover, whenever R1 reached 60 °C, the temperature at each needle was measured ().
Rabbits
The study protocol was approved by our institutional animal care committee. All animals were treated according to the standards set in the Guide for the Care and Use of Laboratory Animals. Twenty-four male and female healthy adult New Zealand white rabbits (mean weight 2.4 ± 0.1 kg (range 2.3–2.5 kg)) were randomly divided into three groups. Two groups of eight rabbits each were injected with 10 mL of gel solution between the liver and stomach wall or 410 ± 95 mL of 5% saline. The third group of rabbits (n = 8) received no injections. After induction of anaesthesia with ketamine (10 mg/kg, Yongguang, China) and diazepam (5 mg/kg, Jichuan, China), the rabbits were placed in a supine position and their ear veins were exposed and cannulated with 20-gauge (1.1 mm × 30.0 mm) catheters for administration of microbubbles, CT contrast agent, and fluids.
Microwave ablation
Each rabbit underwent percutaneous ultrasound scanning to choose the appropriate needle and to locate the area of puncture on the skin sites. After routine disinfection the skin of each rabbit was incised to 2 mm with a small sharp knife, and the microwave antenna was placed at the site indicated by ultrasound scanning and fixed with a haemostat. Ablation was performed for 240 s at a microwave output power of 40 W.
Outcome measures
All rabbits were monitored and underwent ultrasound contrast and/or enhanced CT examination to assess any thermal ablation injury to the liver and the surrounding stomach wall 3 days later. The animals were subsequently sacrificed and dissected, and any changes to the colour of the stomach wall adjacent to the ablation zone were recorded. Tissue samples were fixed in 10% formalin, embedded in paraffin, stained with haematoxylin and eosin (H&E), and assessed by light microscopy for stomach wall damage. Stomach wall damage was graded by two independent pathologists as 1 (no damage), 2 (damage to the serosal layer), 3 (damage to the outer muscular layer), 4 (damage to the inner muscular layer), 5 (damage to the submucosa), and 6 (damage to the mucosal layer).
Statistical analysis
Data were reported as the mean ± standard deviation. The damage level among the three groups was evaluated using multiple independent samples and non-parametric Kruskal-Wallis tests. The short-axis diameter (Ds) was evaluated using Student’s t-test, and the injury level (score) was evaluated using the Mann-Whitney U-test. All statistical analyses were performed using the SPSS 18.0 statistical package (Chicago, IL), with p < 0.05 defined as statistically significant.
Results
In vitro results
After microwave ablation for 150 s, the maximum temperature difference of 31.3 ± 0.1 °C was observed between R1 and R2. Following 300 s of microwave ablation, the temperature of R1 was 74.1° ± 0.8 °C, and the highest temperature at R2 was 48.7 °C (). When the temperature of R1 reached 60 °C, the mean temperatures at R2, R3, and R4 were 29.7° ±0.4 °C, 18.6° ± 0.2 °C, and 16.0° ± 0.3 °C, respectively, and the temperature difference between R1 and R2 on both sides of the gel was 30.3° ± 0.3 °C (). Thus, when the temperature on one side of the 5-mm thick gel was 60 °C, the mean maximum temperature on the other side was 29.7° ± 0.4 °C.
In vivo results
After in situ injection of 10 mL of gel between the liver and stomach (), the thickness of the gel was about 5 mm, and the distance between the ablation needle tip and the liver was about 5 mm. Ultrasound contrast and enhanced CT showed no apparent damage to the liver ablation zone close to the in situ gel (). Necrotic areas involving brown coagulation surrounded the visible red hyperaemia () and had an average thickness of 14.8 ± 0.6 mm (Supplementary Figure S1A), with no colour change in the hepatic ablation zone adjacent to the stomach wall ( and Supplementary Figure S1B). Histopathological analysis showed that the layers of the intestinal wall structure were clearly defined, with no abnormalities ().
Figure 3. (a) Ultrasonographic view showing placement of the ablation needle (white arrow), with the in situ gel (red arrow) located between the liver and stomach wall (blue arrows). (b) Enhanced CT scan after ablation showing in situ gel (red arrow) between the lesion (white arrow) and the stomach wall (blue arrows). (c) Contrast-enhanced ultrasound CEUS, showing the gel (red arrow) located between the lesion (blue arrow) and the stomach wall (white arrow). (d) Photograph showing the microwave ablation area (white arrow) and the adjacent stomach wall (black arrow). (e) Histopathology, showing no stomach wall damage (H&E staining). For interpretation of the references to colour in this figure legend, please refer to the web version of this article at informahealthcare.com.
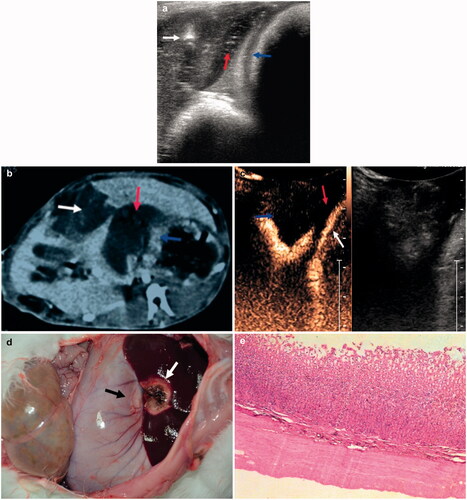
Following the injection of 410 ± 95 mL of 5% saline (315–510 mL), which had properties similar to that of artificial ascites, the saline solution was 5 mm in thickness, and the distance from the ablation needle tip to the surface of the liver was about 5 mm (). The average thickness of the ablation zone was 14.9 ± 0.7 mm (Figure S2B). Three of the rabbits showed colour changes in the liver ablation zone adjacent to the intestinal wall (). Histopathological examination showed that the gastric parietal part of the structures was not well defined. We also observed coagulation necrosis, destruction of cellular nuclei, and a pink unstructured region in the deepest part of the outer muscular layer (). Three rabbits had grades 1 and 2 damage.
Figure 4. (a) Photograph showing the ablated areas (white arrow) and the injured stomach wall (black arrow). (b) Ultrasonographic image showing the artificial ascites were 0.6 cm wide (white arrow) between the liver (red arrows) and the diaphragm (black arrow). (c) Ultrasonographic image showing successful injection of artificial ascites, ultrasound-guided placement of ablation needle (white arrow) between the liver and the stomach wall (red arrow), and the formation of ascites 0.2 mm in width (blue arrows). (d) Histopathological examination showing thermal damage to the outer muscular layer of the stomach wall (black arrow). For interpretation of the references to colour in this figure legend, please refer to the web version of this article at informahealthcare.com.
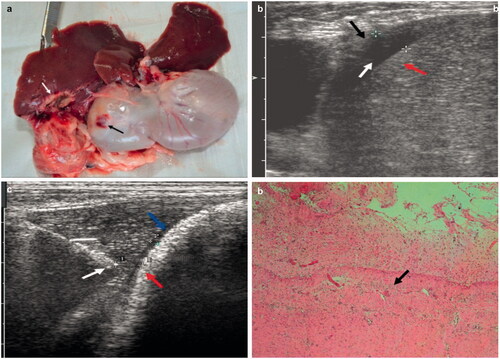
The control group of eight rabbits did not receive any ablation protection measures. The tip of the ablation needle was approximately 5 mm from the edge of the liver (). The average ablation zone was 14.1 ± 0.7 mm (Figure S3), with colour changes observed in the intestinal wall surrounding the ablation zone (). Histopathological examination showed that the gastric parietal part of the structures was not well defined. Coagulation necrosis, structural damage to cell nuclei, and a pink unstructured district were observed in the deepest of the mucosal layer (). One rabbit had a grade 1 injury; 2 had grades 3, 4 and 5 injuries; and 1 had a grade 6 injury.
Figure 5. (a) Ultrasonographic image showing that the distance between the ablation needle (white arrow) and the stomach wall (blue arrow) was approximately 5 mm. (b) Photograph showing the ablation areas (white arrow) and the adjacent stomach wall (black arrow). (c) Histopathological examination showing injury to the mucous layer of the stomach wall (black arrow). For interpretation of the references to colour in this figure legend, please refer to the web version of this article at informahealthcare.com.
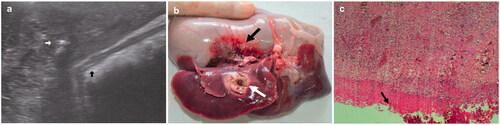
shows the levels of pathological thermal damage to the stomach wall in the three study groups. Statistical analysis showed that thermal damage to the stomach wall among the three groups of rabbits differed significantly (p < 0.01, and ). The injury was significantly greater in the group receiving saline than in the group receiving the gel (p = 0.01), although the Ds values did not differ significantly (p = 0.7) (). Similarly, the damage in the control group was significantly greater than in the group receiving the gel (p < 0.01); however, the Ds values were similar (p = 0.36) ().
Table I. Levels of pathological thermal damage to the stomach wall in the three groups of New Zealand white rabbits.
Table II. Comparisons of the maximum short-axis diameter of the ablation lesions and the thermal injury level of the stomach wall between the two experimental groups.
Table III. Comparison of the short-axis diameter of the ablation area and the injury level of the stomach wall between rabbits injected with chitosan gel and control rabbits.
Discussion
Microwave ablation is currently the most commonly used thermal ablation method for the treatment of hepatic malignancy in China [Citation17]. However, microwave ablation of the liver may have a deleterious effect on the integrity of adjacent temperature-sensitive structures such as the gallbladder and gastrointestinal tract, thereby resulting in post-operative complications. This excessive thermal injury can occur because the temperature within the ablated lesion can reach up to 100 °C [Citation18], and the heat in the ablated lesion may be conducted to adjacent organs [Citation19–21]. In particular, collateral thermal damage can occur in adjacent extrahepatic organs during the treatment of subcapsular tumours [Citation20,Citation22]. Of 1136 patients who underwent MWA for subcapsular tumours, 30 (2.6%) experienced major complications, including two (0.2%) who experienced perforation of the colon. Complications of the colon likely result because it is relatively fixed in position and has a thinner wall than the stomach [Citation3,Citation23]. Bowel perforation is also a major complication of RF ablation and has been reported to occur at an incidence of 0.2–0.3% [Citation4–6,Citation24]. Moreover, fistulous tracts may develop between the skin and gastric wall because of delayed gastric perforation and hepatic abscess formation after RF ablation [Citation5,Citation25,Citation26]. The rate of local tumour progression after the ablation of hepatic tumours adjacent to extrahepatic organs has been reported to range from 2% to 15% [Citation27,Citation28]. Ablation is not recommended for large, infiltrating tumours located near the gastrointestinal tract due to the risk of bowel perforation; instead, these patients should be treated by chemoembolisation or laparoscopic RF ablation [Citation4,Citation24].
Several techniques can be used to overcome these limitations of ablation, including the use of a different approach (laparoscopic or open) or guiding modality (CT or MR). Other methods involve using a balloon catheter or an artificial fluid, or air, changing the position of the patient, or altering the position of the electrode [Citation29]. Artificial ascitic fluid represents an additional option in reducing thermal injury to adjacent tissues, since ascitic fluid is believed to reduce the severity of ablation treatment through washing away thrombogenic material at the puncture site and decreasing the ‘tamponade effect’ from the parietal peritoneum opposite to the liver. However, the main obstacle in introducing the use of artificial ascites is the occurrence of peritoneal adhesion due to prior treatments, including surgical resection, TACE, or thermal ablation [Citation30]. Moreover, the thickness of the artificial ascitic layer can vary depending on the location of the liver tumour.
Several previous studies have reported the preparation of β-GP thermosensitive hydrogel from chitosan solutions containing β-GP [Citation16,Citation31]. Of particular interest, these solutions show a temperature-controlled, pH-dependent transition from solution to gel at a temperature close to 37 °C [Citation16,Citation31]. Accordingly, the properties of the gel allow it to remain liquid at room temperature and physiological pH but to convert into a gel when heated to body temperature [Citation32]. Additionally, these aqueous formulations can be easily injected. Indeed, injectable in situ gel-forming systems have received increased interest in drug delivery and tissue engineering [Citation33]. Chitosan thermosensitive hydrogels possess a number of favourable properties, including non-toxicity [Citation34], biocompatibility, and biodegradability. In addition, these hydrogels show antimicrobial, wound healing, mucoadhesive, and tissue regeneration properties [Citation35]. Clinically, the use of injectable scaffolds offers distinct advantages, including the minimising of patient discomfort, risk of infection, scar formation, and the cost of treatment. We therefore tested a chitosan polymer as an injectable hydrogel scaffold that forms in situ and shows a good insulation effect.
As observed in the present study, chitosan hydrogel injection into the ablation zone to a thickness of 5 mm can result in a temperature difference of about 30 °C between both sides of the gel. This insulative capacity can protect areas adjacent to the treatment zone and reduce post-operative complications. However, because the chitosan material becomes dehydrated and contracts as the temperature increases, it was tested over a range of 30–200 °C [Citation36]. The highest temperature was close to the boiling point of water, and the average weight loss was 14%. At temperatures between 80–200 °C, the structure of the chitosan material did not change significantly, indicating that chitosan does not undergo significant thermal degradation at these temperatures. In the present study, during ablation at temperatures corresponding to the one of tissue necrosis (74.1 ± 0.8 °C), the maximum temperature on the other side of the chitosan hydrogel was 48.7 °C.
In the present study, contrast-enhanced ultrasound, enhanced CT, and post-operative anatomical analysis showed that the area of liver coagulation necrosis around the microwave ablation area was located at the edge of the liver. After injection of the gel, none of the rabbits experienced ablation injury, partly because the gel had been placed into a predetermined position and remained relatively fixed during microwave ablation (e.g. the gel was not shifted as a result of the heat). However, the level of thermal damage in the rabbits that received the artificial ascites was high, despite injection into a predetermined position. A possible explanation for the thermal damage is that the microwave ablation process, as well as breathing and gastrointestinal motility, caused a shift in the position of the ascites adjacent to the stomach, resulting in thermal damage. Artificial ascites provided some protection to the adjacent stomach wall during the ablation of the rabbit liver tissue. However, because of the inherent fluidity, the artificial ascites may not have reached their predetermined position or may have flowed away from it, resulting in thermal injury.
The most severe injury to the stomach wall was observed in the control group. Animal studies of heat damage after RF ablation showed thermal injury in all examined layers in areas within 1 cm of the liver surface [Citation37]. The temperature of the coagulative necrotic area increased rapidly to 100 °C, resulting in the rapid disintegration of cell structure and damage to the stomach wall. Our in vitro and in vivo results suggest that chitosan hydrogel can reduce thermal damage resulting from microwave ablation. Chitosan gel of a suitable thickness can provide protection to the structural organisation close to the ablation zone. Moreover, in situ injection of this gel protected rabbit stomach walls from damage during the microwave ablation of rabbit liver tissue. Future animal and clinical experiments are required to establish the effectiveness of chitosan gel.
A study limitation is that the protective effects of the chitosan gel were tested in normal animals and not in an established tumour model. Moreover, the thermal characteristics of the tumour and normal tissues may differ, including vascularity and thermal conductivity, and the post-surgery effects of chitosan were not investigated in the present study. Nevertheless, these results may contribute to a comprehensive understanding of the effects of chitosan during microwave ablation of liver tumours adjacent to gastrointestinal organs.
Declaration of interest
This study was supported by the National Nature Science Foundation of China (81171358). The authors alone are responsible for the content and writing of the paper.
Supplementary Material
Download PDF (1.2 MB)References
- Tanaka K, Shimada H, Togo S, Ota M, Yamagichi S, Ike H. Is hepatic resection for multiple liver metastases from colorectal carcinoma acceptable treatment? Hepatogastroenterology 2001;48:803–7
- McGhana JP, Dodd GD III. Radiofrequency ablation of the liver: Current status. Am J Roentgenol 2001;176:3–16
- Poon RT, Fan ST, Tsang FH, Wong J. Locoregional therapies for hepatocellular carcinoma: A critical review from the surgeon’s perspective. Ann Surg 2002;235:466–86
- Rhim H, Yoon KH, Lee JM, Cho Y, Cho JS, Kim SH, et al. Major complications after radio-frequency thermal ablation of hepatic tumors: Spectrum of imaging findings. Radiographics 2003;23:123–34
- Livraghi T, Solbiati L, Meloni MF, Gazelle GS, Halpern EF, Goldberg SN. Treatment of focal liver tumors with percutaneous radio-frequency ablation: Complications encountered in a multicenter study. Radiology 2003;226:441–51
- de Baère T, Risse O, Kuoch V, Dromain C, Sengel C, Smayra T, et al. Adverse events during radiofrequency treatment of 582 hepatic tumors. Am J Roentgenol 2003;181:695–700
- Mulier S, Mulier P, Ni Y, Miao Y, Dupas B, Marchal G, et al. Complications of radiofrequency coagulation of liver tumours. Br J Surg 2002;89:1206–22
- Wong SN, Lin CJ, Lin CC, Chen WT, Cua IH, Lin SM. Combined percutaneous radiofrequency ablation and ethanol injection for hepatocellular carcinoma in high-risk locations. Am J Roentgenol 2008;190:W187–95
- Teratani T, Yoshida H, Shiina S, Obi S, Sato S, Tateishi R, et al. Radiofrequency ablation for hepatocellular carcinoma in so-called high-risk locations. Hepatology 2006;43:1101–8
- Huang FY, Huang LK, Lin WY, Luo TY, Tsai CS, Hsieh BT. Development of a thermosensitive hydrogel system for local delivery of (188)Re colloid drugs. Appl Radiat Isot 2009;67:1405–11
- Lee J, Lim HK, Choi D, Kim SH, Min K, Jeon YH. Radiofrequency ablation of the liver abutting stomach: In vivo comparison of gastric injury before and after intragastric saline administration in a porcine model. Eur J Radiol 2009;72:154–9
- Miele V, Andreoli C, De Cicco ML, Adami L, David V. Hemoretroperitoneum associated with liver bare area injuries: CT evaluation. Eur Radiol 2002;12:765–9
- Raman SS, Lu DS, Vodopich DJ, Sayre J, Lassman C. Minimizing diaphragmatic injury during radio-frequency ablation: Efficacy of subphrenic peritoneal saline injection in a porcine model. Radiology 2002;222:819–23
- Austin PR, Brine CJ, Castle JE, Zikakis JP. Chitin: New facets of research. Science 1981;212:749–53
- Lee JY, Nam SH, Im SY, Park YJ, Lee YM, Seol YJ, et al. Enhanced bone formation by controlled growth factor delivery from chitosan-based biomaterials. J Control Release 2002;78:187–97
- Chenite A, Chaput C, Wang D, Combes C, Buschmann MD, Hoemann CD, et al. Novel injectable neutral solutions of chitosan form biodegradable gels in situ. Biomaterials 2000;21:2155–61
- Qiu-Jie S, Zhi-Yu H, Xiao-Xia N, Wen-Yuan S, Yuan-Yuan S, Liu H, et al. Feasible temperature of percutaneous microwave ablation of dog liver abutting the bowel. Int J Hyperthermia 2011;27:124–31
- Goldberg SN. Comparison of techniques for image-guided ablation of focal liver tumors. Radiology 2002;223:304–7
- Rossi S, Buscarini E, Garbagnati F, Di Stasi M, Quaretti P, Rago M, et al. Percutaneous treatment of small hepatic tumors by an expandable RF needle electrode. Am J Roentgenol 1998;170:1015–22
- Livraghi T, Goldberg SN, Lazzaroni S, Meloni F, Solbiati L, Gazelle GS. Small hepatocellular carcinoma: Treatment with radio-frequency ablation versus ethanol injection. Radiology 1999;210:655–61
- Gazelle GS, Goldberg SN, Solbiati L, Livraghi T. Tumor ablation with radio-frequency energy. Radiology 2000;217:633–46
- Akahane M, Koga H, Kato N, Yamada H, Uozumi K, Tateishi R, et al. Complications of percutaneous radiofrequency ablation for hepato-cellular carcinoma: Imaging spectrum and management. Radiographics 2005;25:S57–68
- Liang P, Wang Y, Yu X, Dong B. Malignant liver tumors: Treatment with percutaneous microwave ablation – Complications among a cohort of 1136 patients. Radiology 2009;251:933–40
- Meloni MF, Goldberg SN, Moser V, Piazza G, Livraghi T. Colonic perforation and abscess following radiofrequency ablation treatment of hepatoma. Eur J Ultrasound 2002;15:73–6
- Yamakado K, Nakatsuka A, Ohmori S, Shiraki K, Nakano T, Ikoma J, et al. Radiofrequency ablation combined with chemoembolization in hepatocellular carcinoma: Treatment response based on tumor size and morphology. J Vasc Interv Radiol 2002;13:1225–32
- Frich L, Edwin B, Brabrand K, Rosseland AR, Mala T, Mathisen O, et al. Gastric perforation after percutaneous radiofrequency ablation of a colorectal liver metastasis in a patient with adhesions in the peritoneal cavity. Am J Roentgenol 2005;184:S120–2
- Choi D, Lim HK, Kim MJ, Kim SH, Lee WJ, Kim SH, et al. Therapeutic efficacy and safety of percutaneous radiofrequency ablation of hepatocellular carcinoma abutting the gastrointestinal tract. Am J Roentgenol 2004;183:1417–24
- Chen MH, Wei Y, Yan K, Gao W, Dai Y, Huo L, et al. Treatment strategy to optimize radiofrequency ablation for liver malignancies. J Vasc Interv Radiol 2006;17:671–83
- Rhim H, Lim HK. Radiofrequency ablation for hepatocellular carcinoma abutting the diaphragm: The value of artificial ascites. Abdom Imaging 2009;34:371–80
- Rhim H, Lim HK, Kim YS, Choi D. Percutaneous radiofrequency ablation with artificial ascites for hepatocellular carcinoma in the hepatic dome: Initial experience. Am J Roentgenol 2008;190:91–8
- Ruel-Gariépy E, Leclair G, Hildgen P, Gupta A, Leroux JC. Thermosensitive chitosan-based hydrogel containing liposomes for the delivery of hydrophilic molecules. J Control Release 2002;82:373–83
- Molinaro G, Leroux JC, Damas J, Adam A. Biocompatibility of thermosensitive chitosan-based hydrogels: An in vivo experimental approach to injectable biomaterials. Biomaterials 2002;23:2717–22
- Jeong B, Bae YH, Lee DS, Kim SW. Biodegradable block copolymers as injectable drug-delivery systems. Nature 1997;388:860–2
- Rao SB, Sharma CP. Use of chitosan as a biomaterial: studies on its safety and hemostatic potential. J Biomed Mater Res 1997;34:21–8
- Ma ZW, Zhang YJ, Wang R, Wang QT, Dong GY, Wu ZF. An animal experiment for the regeneration of periodontal defect by application of the dual-release chitosan thermosensitive hydrogel system. Zhonghua Kou Qiang Yi Xue Za Zhi 2008;43:273–7
- de Britto D, de Assis OB. Synthesis and mechanical properties [in Chinese] of quaternary salts of chitosan-based films for food application. Int J Biol Macromol 2007;41:198–203
- Hansen PD, Rogers S, Corless CL, Swanstrom LL, Siperstien AE. Radiofrequency ablation lesions in a pig liver model. J Surg Res 1999;87:114–21