Abstract
Purpose: The ‘foetal origin hypothesis’ postulates that a number of organ structures and associated functions undergo programming during embryonic and foetal life and the neonatal period, which determines the set point of physiological and metabolic responses that carry into adulthood. We evaluate the relationship between high environmental temperatures and the reproductive function of male offspring to determine whether pregnant mammals and their infants are potentially vulnerable to the effects of climate change.
Methods: Rabbit pups were exposed to high temperatures during gestation and lactation. Results: Foetal and postnatal exposure to high temperatures did not alter semen characteristics and was associated with a similar fertility rate and number of pups born. Moreover, males showed reduced rate of maturing and carcass traits at adulthood.
Conclusion: Our findings suggest that male exposure during the foetal period to high temperatures did not affect sperm quality but permitted an adaptive phenotypic plasticity of growth in adulthood.
Introduction
Global surface temperature has increased by 0.75 °C since 1850 and is expected to increase another 1.1–6.4 °C by the end of the 21st century as a consequence of anthropogenic build-up of greenhouse gases in the atmosphere [Citation1]. Over the past 60 years, extremely high temperature events around the world have increased by 40% [Citation2], while forecasts predict an increase in the duration, frequency, and/or intensity of heat waves, with 90–99% probability [Citation1]. With global climate change becoming increasingly evident, understanding the physiological responses of organisms to these changes is a priority [Citation3].
Stressful heat exposure has effects on most aspects of reproductive function in mammals, such as spermatogenesis and oocyte development, oocyte maturation, early embryonic development, foetal and placental growth and lactation [Citation4]. It is likely that extreme temperatures (hyperthermia) cause problems ranging from embryonic death and abortion to teratogenically induced anomalies, which are heavily dependent on the dose and timing of the exposure [Citation5,Citation6]. Nevertheless, most animals can adapt physiologically and biochemically when exposed to altered temperatures for prolonged periods [Citation7]. The heat acclimation process involves two distinct phases: the first, short-term acclimation is an early, transient phase, characterised by an augmented autonomic signal output ratio in readiness to compensate for cellular perturbations. The second is a sustained stable phase called long-term heat acclimation, during which a new homeostasis is achieved [Citation8]. Nevertheless, a short duration and mild changes in environmental conditions are considered factors analogous to psychological stress [Citation9]. Chronic exposure to stressors increases the hypothalamic–pituitary–adrenal (HPA) axis activity and concomitantly reduces hypothalamic–pituitary–gonadal (HPG) axis activity. It has been proposed that the antagonistic relationship between these axes may underlie the inhibition of reproductive function due to stress [Citation10]. As an example, during pregnancy the mother’s HPA axis becomes hyporeactive to stressors [Citation11–14].
The last decade has witnessed the emergence and consolidation of a model based on evolutionary biology, in which foetal programming by maternal stress is seen as an evolved adaptive process [Citation14–22]. In this perspective, foetal programming is an instance of adaptive developmental plasticity [Citation23]. The theory of selection in utero suggests three hypotheses concerning the effect of environmental stressors on human reproduction [Citation24]. The first states that stressed populations will yield relatively low ratios of male to female live births [Citation25–32]. The second is that males in low sex-ratio birth cohorts will, on average, live longer than those from higher sex-ratio cohorts, because selection in utero has removed less robust individuals from the low sex-ratio birth cohorts [Citation30,Citation33,Citation34]. The third posits that relatively frail males gestating during warm periods who then confront cold climates in childhood, on average, live shorter lives than other males [Citation24,Citation30]. A standard assumption in the foetal programming literature is that higher levels of stress hormones signal a more dangerous and/or unpredictable environment [Citation18–20,Citation22].
Our aim was to evaluate how the high environmental temperatures in gestation and lactation periods affect growth pattern and reproductive function of male offspring and determine whether these periods are potentially vulnerable to effects of climate change.
Materials and methods
Animal protocols followed the ethical principles of animal care published by Spanish Royal Decree 53/2013 [Citation35] and were approved by the ethics committee of the Universidad Politécnica de Valencia. Animals were treated humanely and with regard for alleviation of suffering.
Experimental protocol and obtainment of offspring
The experiment was conducted at the Animal Science Department, Polytechnic University of Valencia (Valencia, Spain). Adult female rabbits (n = 26) were housed in conventional housing (with a light-alternating cycle of 16 h of light and 8 h of darkness under controlled environmental conditions: average daily minimum and maximum temperatures of 14 °C and 20 °C, respectively) using individual cages (700 mm × 500 mm × 320 mm) provided with a nest for litters from gestation day 28.
The female rabbits were randomly distributed into two different experimental groups: some under high environmental temperatures (ranged between 25° and 36 °C, n = 13) and the others under standard temperature (ranged between 14° and 20 °C, n = 13). Dams were allowed to acclimatise for 30 days before being artificially inseminated. The climatic chamber was equipped with a heating/cooling system which scheduled a sine function for the daily environmental temperature, with a minimum temperature of 25 °C early in the morning and a maximum of 36 °C in the afternoon. This system ensured environmental stress with a temperature up to 28 °C for 65% of the day [Citation36].
On birth day (considered day 0), pups were weighed, sexed and microchipped. Litters were culled to 10 pups with an equivalent sex ratio if possible. The results of this study pertain to the male offspring only. All experimental manipulations involving the allocation of pups into their respective experimental groups were performed on day 0 (). Pups were allocated into groups as follows:
Pups underwent both gestation (30 days) and lactation (30 days) in high temperatures (25° to 36 °C; group G+&L+)
Pups gestated in high temperatures then underwent lactation in standard temperatures (group G+&L−)
Pups gestated in standard temperatures (14–20 °C) then underwent lactation in high temperatures (group G−&L+)
Pups maintained in standard temperatures throughout gestation and lactation (group G−&L−).
Figure 1. Schematic illustration of gestation and lactation period and time of exposure to high temperatures in the experimental groups. G−&L−, control animals raised in standard laboratory temperatures during both gestation and lactation; G+&L−, animals exposed to high temperatures during gestation; G−&L+, animals exposed to high temperatures during lactation; G−&L+, animals exposed to high temperatures during both gestation and lactation.
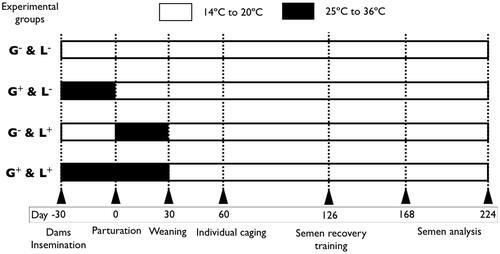
All litters were kept under each experimental environment until weaning at 30 days. Then, litters were moved to the control environment with a photoperiod of 16 h of light and fed a commercial diet ad libitum (15.5% crude protein [CP], 2.3% fat, 17.2% fibre; dry matter [DM] basis) until day 60. After this period, the male rabbits were individually housed and fed with another commercial diet (180 g/day; 16% CP, 4.3% fat, 17% fibre; DM basis). Fresh water was always available.
Evaluation of male offspring: reproductive traits
Determination of plasma concentration of total testosterone
Blood samples from the marginal ear vein were collected for 40 male rabbits randomly sampled at 16, 24, 36 weeks of age. These ages take into account changes in both pubertal (16 weeks of age) and mature, sexually active animals (24 and 36 weeks of age). Plasma was isolated by blood centrifugation at 3000 g for 20 min at 4 °C and then stored at −80 °C until used. Plasma testosterone concentration was determined by direct enzyme immunoassay technique following the manufacturer’s instructions (DRG International, Marburg, Germany). Test sensitivity was 0.1 ng/mL.
Semen collection and sperm evaluation
A total of 53 male rabbits began the training period with an artificial vagina at 18 weeks of age. From 24 to 36 weeks of age, two ejaculates per male and per week were collected, with an interval of 30 min between collections. Ejaculates from the same male each day were pooled and three 20 μL aliquots were taken. The first and second were diluted 1:20 with Tris-citrate-glucose extender (250 mM tris-hydroxymethylaminomethane, 83 mM citric acid, 50 mM glucose, pH 6.8–7.0, 300 mOsmkg−1). The first was assessed for individual sperm motility and motion parameters using the Integrated Semen Analysis System version 1.0.17 (ISAS; Projectes i Serveis R + D). The system was set to record images at 25 frames/s. Then 10 µL of the sample was placed in a 10 -µm deep Makler counting chamber. Sperm motility was assessed at 37 °C at ×20 using a negative phase contrast microscope. For each sample, four microscopic fields were analysed and a minimum of 200 sperm evaluated. The following sperm activity variables were assessed: motile sperm cells (%), curvilinear velocity (VCL, μm s−1), linear velocity (VSL, μm s−1), average velocity (VAP, μm s−1), linearity coefficient (LIN; calculated as (VSL/VCL) × 100, %), straightness coefficient (STR), wobble coefficient (WOB; VSL/VAP × 100), amplitude of lateral head movement (ALH, μm) and track crossing frequency (BCF, Hz). The second sample was assessed for the percentage of live and dead spermatozoa using the LIVE/DEAD sperm viability kit (Molecular Probes), which consists basically of two DNA-binding fluorescent stains: a membrane-permeant stain, SYBR-14, and a conventional dead-cell stain, propidium iodide [Citation37]. The third sample was also diluted 1:20 with 0.5% of glutaraldehyde solution in phosphate buffered saline to calculate the concentration in a Thoma-Zeiss counting cell chamber and evaluate both the percentages of intact apical ridge and abnormal sperm (spermatozoa with morphological abnormalities of head, neck, mid-piece and tail) by phase contrast at ×400 magnification.
Collection of reproductive organs: testis tissue sampling
A total of 103 male rabbits in pubertal (16 weeks of age) and mature, sexually active stages (36 weeks of age) were randomly sampled. Male rabbits were euthanised by sodium pentobarbital overdose (∼150–200 mg/kg administered intravenously) for testis recovery. Gonads were weighed and the length and width (measured at the point of maximum width) were taken with a calliper. The average of left and right measurements for weight, length and width were calculated to obtain averaged testis weight, averaged testis length and averaged testis width. The right testes were fixed in 4% (water/vol) neutral formalin solution and pieces were embedded in paraffin wax and sectioned at 5 μm. The sections were stained with haematoxylin and eosin and observed by light microscopy, in a blind assay for histological examination. Fifty seminiferous tubule cross sections per animal were evaluated as the percentage of seminiferous tubules with presence of lumen, presence of spermatids in the seminiferous epithelium or the presence of spermatozoa in the lumen of the seminiferous tubules. A representative stained image of a testis section is shown in .
Figure 2. Representative images of haematoxylin and eosin stained sections from rabbit seminiferous tubules. (A) and (B) seminiferous tubules without a lumen; (C) seminiferous tubules without a lumen with presence of spermatids in the seminiferous epithelium; (D) seminiferous tubules without a lumen; (E) presence of spermatids in the seminiferous epithelium; (F) presence of spermatozoa in the lumen of the seminiferous tubules. Microphotographs (A) and (D) were observed under a light microscope at ×100. Microphotographs (B), (C), (E) and (F) were observed under a light microscope at ×400.
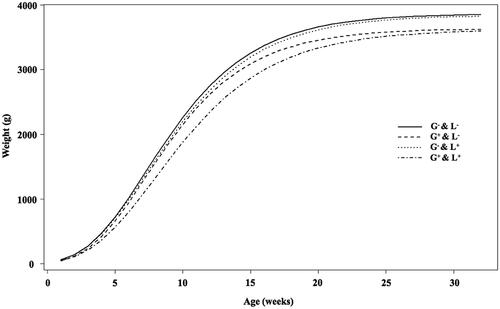
Fertility rate and number of pups born
Fertility and number of pups born outcomes were compared among male rabbits that underwent both gestation and lactation in high temperatures (group G+&L+) and males that underwent both gestation and lactation in standard temperatures (group G−&L−). Ejaculates for each experimental group were pooled and the concentration adjusted to 20 × 106 cells/mL in a pool, balancing the sperm contribution of each male to eliminate individual differences. A total of 321 inseminations were carried out with semen from 16 pools (two pools per week). Each female was inseminated with 0.5 mL (about 10 × 106 cells per doe), which was performed within 2–4 h of semen collection. At insemination time, each female was injected intramuscularly with 1 μg of buserelin acetate (Hoechst Marion Roussel, Madrid, Spain) to induce ovulation. Only receptive does (red colour of vulvar lips) were inseminated, using a standard curved plastic pipette (Imporvet, Barcelona, Spain). The number of dams that gave birth by number of inseminations (fertility rate) and number of live pups per litter were recorded.
Evaluation of male offspring: growth traits
Growth study
A total of 53 animals were weighed weekly from 1 to 36 weeks old. The Gompertz curve, well suited for rabbits [Citation38], was used as the non-linear function describing growth of rabbits: y = a exp[−b exp(−kt)], where y is the observed body weight of one individual at a specific age; a, b and k are the parameters of the Gompertz function for the one animal, which have a biological interpretation; a can be interpreted as the mature body weight, maintained independently of short-term fluctuations; b is a timescale parameter related to the initial body weight; k is a parameter related to the rate of maturing (growth rate).
Carcass characteristics
Male rabbits were euthanised by sodium pentobarbital overdose (∼150–200 mg/kg administered intravenously) for carcass study on week 36. After scarification, skin and full gastrointestinal tract were removed and the carcasses stored at 3 °C for 24 h. The carcass traits were determined according to the recommendations made by Blasco and Ouhayoun [Citation39]. Various measurements were taken after slaughtering: chilled carcass weight (CCW), reference carcass weight – carcass without head and organs (RCW), head weight (HW), liver weight (LvW), kidneys weight (KiW), dissectible fat weight of the chilled carcass, perirenal (PFaW), and scapular (SFaW). Reference carcasses were divided into technological joints as indicated by the World Rabbit Science Association (WRSA) [Citation39]. Joints obtained were weighed and consisted of forelegs (FLW), thoracic cage (TW), loin (LoW), and hind part (HPW). From the hind part, a hind leg (HLW) was carefully dissected to separate the bone (HLBW) from the muscle (HLMW).
Statistics
All statistical analyses were performed by the SPSS statistical software package, version 16.0 (Chicago, IL, USA), except for determination of the parameters of the Gompertz function, performed by the SAS statistical software package, version 9.2 (SAS Institute, Cary, NC).
The model used for the seminal traits was a factorial randomised design (2 × 2). There were two gestation environments (G− versus G+) and two lactation environments (L− versus L+). Otherwise, prenatal and postnatal environments were compared with a mixed linear model including as fixed effects the gestation (high temperatures, G+ or standard laboratory temperatures, G−) and lactation (high temperatures, L+ or standard laboratory temperatures, L−) and their interaction (G−&L+, G−&L−, G+&L− and G+&L+) and male as random effect. The weight at birth was included as a covariate.
For fertility rate, a probit link with binomial error distribution was used according to a mixed model including the environment during gestation and lactation as fixed effect (G+&L+ versus G−&L−) and pool as random effect. A mixed linear model was used to analyse the number of kits born live and number of stillborn kits according with the model used to analyse fertility.
Growth curves for male rabbits were fitted following the Gompertz model as described by Laird [Citation40] since the Gompertz curve is appropriate to describe rabbit growth [Citation38]. First, the parameters of the Gompertz curve were estimated by line using the NLIN procedure of SAS software. The differences between experimental groups for the Gompertz curve parameters were tested using an ANOVA as previously (randomised design with 2 × 2 factorial arrangement), considering a normal distribution of the parameters. For all statistical tests, results were considered significant at p < 0.05.
Results
Semen collection and sperm evaluation
All male rabbits were adapted to ejaculate into an artificial vagina. Over the course of the experiment, only one male from group G+&L− died. The environment affected semen characteristics (). Male rabbits that underwent gestation in high temperatures showed a higher viability rate (86.3 ± 2.18% versus 78.7 ± 2.13%, p < 0.05) and the intact apical ridge (82.9 ± 1.83% versus 77.2 ± 1.83%, p < 0.05). Additionally, the environment affected motion parameters (). Male rabbits that underwent gestation in high temperatures showed a higher VSL (35.1 ± 1.80 μm s−1 versus 30.1 ± 1.65 μm s−1, p < 0.05), VAP (62.3 ± 3.12 μm s−1 versus 51.6 ± 2.81 μm s−1, p < 0.05) and ALH (2.9 ± 0.11 μm versus 2.5 ± 0.11 μm, p < 0.05), while males that underwent lactation in high temperatures showed a lower VSL (29.3 ± 1.83 μm s−1 versus 35.7 ± 1.52 μm s−1, p < 0.05) and WOB (58.1 ± 1.28% versus 62.4 ± 1.07%, p < 0.05). However, interactions were not significant.
Table 1. Ejaculates and sperm parameters and motility assessment of male rabbits exposed to high temperatures during pregnancy and the postnatal period.
Table 2. Sperm motility assessment of male rabbits exposed to high temperatures during pregnancy and postnatal period.
Testosterone concentration
The testosterone concentration of G+&L+ male group was significantly higher compared with the other experimental groups at 16 weeks of age, but did not persist until 24 weeks of age (). Specifically, warm lactation period induced higher testosterone concentration at 16 weeks of age (5.6 ± 0.77 versus 2.8 ± 0.77 for L+ versus L−, respectively, see ).
Table 3. Serum testosterone levels of male rabbits exposed to high temperatures during gestation and/or lactation at pubertal age (16 weeks old) and mature, sexually active ages (24 and 36 weeks old).
Testis tissue sampling
We observed no statistical differences in the male histometric measures and histological parameters between experimental groups and the age evaluated ().
Figure 3. Analysis of testicular histometry and histology of male rabbits exposed to high temperatures during gestation (G+&L−), lactation (G−&L+), both gestation and lactation (G+&L+), and control group (G−&L−) at pubertal age (16 weeks old) and mature, sexually active ages (24 and 36 weeks old). (A) Histometry: testis weight, length and width. (B) Histology: percentage of seminiferous tubules with presence of lumen, percentage of seminiferous tubules with presence of spermatids and the percentage of seminiferous tubules with presence of spermatozoa.
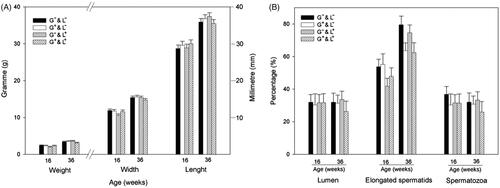
Fertility and number of neonates per litter
We assessed male offspring fertility by the total number of litters per dam and the number of pups per dam for the G+&L+ and G−&L− groups (radical extremes) between 28 to 36 weeks of age (). We observed no statistical differences in the fertility (91.3% versus 89.4% for G+&L+ versus G−&L−, respectively). In the same way, the number of pups per dam did not differ between both groups (8.6 ± 0.38 versus 8.4 ± 0.38 for G+&L+ versus G−&L−, respectively).
Table 4. Fertility and prolificacy of male rabbits exposed to high temperatures during pregnancy and postnatal period.
Growth and carcass traits
The growth curves estimated using Gompertz function for experimental groups are shown in . The G−&L− and G−&L+ groups had similar growth curves, while differences in growth rates between both G−&L− and G−&L+ groups versus G+&L− and G+&L+ groups can be observed. The four groups had similar estimates of Gompertz growth curve b and k parameters (). However, male rabbits that underwent gestation in high temperatures had a lower mature body weight (3576.2 ± 44.82 versus 3748.2 ± 39.15, for G+ versus G−, respectively, ). Additionally, males that underwent gestation in high temperatures had a lower body weight at 16 weeks of age (pubertal) and 24 and 36 weeks of age (mature, sexually active animals) ().
Figure 4. Average growth curves estimated with the Gompertz function during the first 36 weeks for male rabbits exposed to high temperatures during gestation (G+&L−), lactation (G−&L+), both gestation and lactation (G+&L+) and control group (G−&L−).
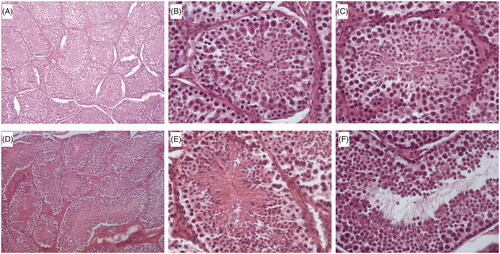
Table 5. Comparison Gompertz growth curve parameters of male rabbits exposed to high temperatures during pregnancy and the postnatal period weighed weekly from 1 to 36 weeks old.
Table 6. Live weight of male rabbits exposed to high temperatures during pregnancy and postnatal period at pubertal age (16 weeks old) and mature, sexually active ages (24 and 36 weeks old).
Similarly, when carcass traits were evaluated only males of the G+ group were affected (). Males that underwent gestation in high temperatures had a lower HPW, HLMW, LOW, SFaW, PFaW, RCW and CCW at 36 weeks of age.
Table 7. Comparison carcass traits at 36 weeks old of male rabbits exposed to high temperatures during pregnancy and postnatal period..
Discussion
Environmental stress has played a major role in the evolution of living organisms [Citation41]. Climatic stress can lead to disruptions in reproductive processes through two general mechanisms: homeokinetic changes and failure of homeokinetic systems [Citation4]. Here, we investigated how the impact of embryonic, foetal and postnatal exposure to high environmental temperatures might affect a male child on into adulthood. Developmental plasticity has been a central theme in embryology [Citation42], and is currently being explored in medicine and public health due to increasing evidence from studies in animal models and humans that various adult-onset diseases, such as hypertension, obesity, diabetes, and altered HPA axis, may have their origins during foetal life [Citation43–46]. The ‘foetal origins hypothesis’ postulates that a number of organ structures and associated functions undergo programming during the embryonic, foetal and neonatal periods, which determines the set point of physiological and metabolic responses that carry into adulthood [Citation47–52]. Specifically, pre- and postnatal exposure to stressful conditions prior to sexual maturity can have negative fitness consequences [Citation53–57]. Although important differences in physiology, metabolism, placental structure and function necessitate caution in the interpretation of animal experiments in their application to humans [Citation58], the difficulty of a cohesive framework in human studies means that controlled experiments with non-human animals may have to serve to illuminate this aetiology [Citation59].
In our model, under a realistic scenario to which populations at the Mediterranean coast are exposed during summer, embryonic and foetal and postnatal exposure to high temperatures did not result in a decline in reproductive capacity in adult male rabbits, but disrupted maturing and growth rates. We also observed that both gestation and lactation exposure to high temperatures disturbs the testosterone concentration at puberty (16 weeks), but this effect did not persist at the sexually active ages of early maturity (24 weeks) and maturity (36 weeks). To our knowledge, this is the first report showing an association between exposure to high temperatures during the gestation and lactation periods and reproductive function in adult males. Although there is clear evidence of effects of early programming by nutrition and endocrine disruptors on adult reproductive physiology, less is known about thermal stress [Citation60].
It is known that foetuses exposed to environmental factors or pharmacological agents suffer alterations that inhibit normal gonadal activity, which in turn affects sexual development and behaviour in adulthood [Citation61]. Although few studies focus on the effect of intrauterine growth conditions on male sexual development and sperm quality later in life, previous studies suggested an impairment of both Sertoli and Leydig cell functions [Citation62]. We did not find any disorder in sex organ weights, length and width and the pattern of testicular histology –regardless of both experimental group and age (16, 24 and 32 weeks of age). Moreover, an exposure to high temperature during lactation period did not affect the adaptation of male rabbits to an artificial vagina to collect semen. Some authors have reported that prenatal stress disrupts the normal maternal-foetal hormonal environmental and suppresses the foetal testosterone peak on gestational days 18 and 19, a peak necessary for later expression and maintenance of male sexual behaviour [Citation63–64]. In the present study, serum testosterone increased in male rabbits exposed to high temperatures during lactation at 16 weeks of age (pubertal) compared with other groups. However, it is important to note that this effect did not persist into adulthood. We cannot yet explain this observation, and unfortunately, testosterone levels were not being measured during early life, but it can be postulated that adaptations to high temperatures suggest a testosterone hyperproduction at pubertal age to enhance the growth-promoting effect of growth hormone or a growth compensation mechanism [Citation65], but without detrimental reproductive performance. During the pubertal growth spurt, onset of gonadal production of sex steroids increases growth rate, especially in males [Citation66]. However, in the current study, birth weight was unaffected and there were no significant differences between groups of dams (mean ± standard error, 59.6 ± 2.38 g versus 63.1 ± 2.15 g, for heat environment versus control environment dams, respectively; data not included in tables). In this sense, epidemiological studies in human and animal experiments have shown that nutrition during foetal and neonatal life may lead to related disorders in adulthood [Citation67]. Extreme heat during pregnancy is related to lower birth weight [Citation68]. Specifically, size at birth reflects the product of the foetus’s growth trajectory and maternoplacental capacity to supply sufficient nutrients to maintain that trajectory [Citation67]. However, body weight does not always reflect the qualitative growth and maturity of the newborn [Citation67]. Moreover, programming effects are thought to be maximised during critical windows of rapid organ growth, resulting in asymmetrical growth. In our study, male rabbits exposed to high temperatures only during gestation had similar rates of maturing and growth compared to lactation and control groups. Nevertheless, exposure to heat stress during only the gestation period resulted in a significant decline in the live weight, carcass traits and scapular and perirenal adipose tissue at mature age. Thus, our results suggest that heat stress in the prenatal period is crucial to programming size in the adult. Prenatal programming of postnatal plasticity may be an adaptive response such that stressful environmental experiences during pregnancy contribute to the foetal programming of developmental plasticity and/or enhance adjustment to the postnatal environment [Citation21]. Specifically, it has been reported that climatic change induces phenotypic shifts [Citation69]. Indeed, our data are in line with the original foetal programming hypothesis that the foetus adapts its phenotype to the anticipated postnatal environment based on maternal cues regarding the quality of the outside world in order to function optimally in that specific environment [Citation21,Citation44,Citation70]. However, it is important to emphasise again that no reproductive alterations appeared until the early stage of adulthood studied in the work (8 months of age).
Variations in maternal care over the first week of life were associated with marked differences in the reproductive physiology and behaviour of adult female offspring [Citation71]. Recently, it was reported that exposure to elevated stress hormones early in postnatal life markedly reduced adult longevity [Citation72]. In our study this effect was not observed, because all the male rabbits were mature (eight months of age) or fully grown, but it was not possible to predict their life expectancy. Specifically, only one of the males died during our experiment. Chronic exposure to restraint stress in utero reprograms the foetal HPA axis [Citation73,Citation74]. Specifically, chronic exposure to stressors increases the HPA axis activity and concomitantly reduces HPG axis activity. It has been proposed that this antagonistic relationship between both of these axes may underlie the inhibition of reproductive function due to stress [Citation10]. It is important that in the hypothalamus, heat acclimation involves substantial but transient central genomic responses in the course of the acclimation period [Citation7]. Nevertheless, stress effects on the HPG axis hormones are relatively complex [Citation75].
Under a realistic scenario of high temperatures during summer we observed significant and unequivocal shifts in the growth rate of adult male rabbits exposed to high temperatures during the gestation period, although sperm quality was not affected. Work is continuing on female rabbit pre- and postnatal exposure to the same high temperatures in order to understand the effects of heat stress on female plasticity. Moreover, our findings in rabbit males confirmed that exposure to heat stress during foetal period induces an adaptive phenotypic plasticity of growth in adulthood.
Declaration of interest
This work was supported by the Spanish Research Projects AGL2008–03274 and AGL2011-30170-C02-01 (CICYT) and funding from the Generalitat Valenciana Research Programme (Prometeo 2009/125). Estrella Jiménez-Trigos and Carmen Naturil-Alfonso were supported by a research grant from the Education Ministry of the Valencian Regional Government (programme VALi+d. ACIF/2010/262 and ACIF/2013/296, respectively). The English text version was revised by N. Macowan English Language Service. The authors declare they have no actual or potential competing financial interests. The authors alone are responsible for the content and writing of the paper.
References
- Intergovernmental Panel on Climate Change. Summary for policymakers. In: Intergovernmental Panel on Climate Change. Climate Change 2007. Cambridge: Cambridge University Press, 2007, pp. 2–18
- Donat MG, Alexander LV. The shifting probability distribution of global daytime and night-time temperatures. Geophys Res Lett 2012;39:L14707
- Mydlarz LD, McGinty ES, Harvell CD. What are the physiological and immunological responses of coral to climate warming and disease? J Exp Biol 2010;213:934–45
- Hansen PJ. Effects of heat stress on mammalian reproduction. Philos Trans R Soc Lond B Biol Sci 2009;364:3341–50
- Graham JM Jr, Edwards MJ, Edwards MJ. Teratogen update: Gestational effects of maternal hyperthermia due to febrile illnesses and resultant patterns of defects in humans. Teratology 1998;58:209–21
- Edwards MJ. Review: Hyperthermia and fever during pregnancy. Birth Defects Res A Clin Mol Teratol 2006;76:507–16
- Schwimmer H, Eli-Berchoer L, Horowitz M. Acclimatory-phase specificity of gene expression during the course of heat acclimation and superimposed hypohydration in the rat hypothalamus. J Appl Physiol 2006;100:1992–2003
- Horowitz M. Do cellular heat acclimation responses modulate central thermoregulatory activity? News Physiol Sci 1998;13:218–25
- Baran A, Jakiel G, Wójcik G. Changes in ambient temperature and oxygenation during the proestrus do not affect duration, regularity and repeatability of the estrus cycle in female rats. Folia Histochem Cytobiol 2009;47:215–19
- Retana-Márquez S, Bonilla-Jaime H, Vázquez-Palacios G, Domínguez-Salazar E, Martínez-García R, Velázquez-Moctezuma J. Body weight gain and diurnal differences of corticosterone changes in response to acute and chronic stress in rats. Psychoneuroendocrinology 2003;28:207–27
- de Weerth C, Buitelaar JK. Physiological stress reactivity in human pregnancy – A review. Neurosci Biobehav Rev 2005;29:295–312
- Russell JA, Douglas AJ, Brunton PJ. Reduced hypothalamo–pituitary–adrenal axis stress responses in late pregnancy: Central opioid inhibition and noradrenergic mechanisms. Ann NY Acad Sci 2008;1148:428–38
- Brunton PJ, Russell JA. Neuroendocrine control of maternal stress responses and fetal programming by stress in pregnancy. Prog Neuro-Psychoph 2011;35:1178–91
- Del Giudice M. Fetal programming by maternal stress: Insights from a conflict perspective. Psychoneuroendocrinology 2012;37:1614–29
- Matthews SG. Early programming of the hypothalamo–pituitary–adrenal axis. Trends Endocrinol Metab 2002;13:373–80
- Kaiser S, Sachser N. The effects of prenatal social stress on behaviour: Mechanisms and function. Neurosci Biobehav Rev 2005;29:283–94
- Kaiser S, Sachser N. Effects of prenatal social stress on offspring development: pathology or adaptation? Curr Direct Psychol Sci 2009;18:118–21
- Kapoor A, Dunn E, Kostaki A, Andrews MH, Matthews SG. Fetal programming of hypothalamo–pituitary–adrenal function: Prenatal stress and glucocorticoids. J Physiol 2006;572:31–44
- Talge NM, Neal C, Glover V. Antenatal maternal stress and long-term effects on child neurodevelopment: How and why? J Child Psychol Psychiatry 2007;48:245–61
- Glover V. Prenatal stress and the origins of psychopathology: An evolutionary perspective. J Child Psychol Psychiatry 2011;52:356–67
- Pluess M, Belsky J. Prenatal programming of postnatal plasticity? Dev Psychopathol 2011;23:29–38
- Sandman CA, Davis EP, Glynn LM. Prescient human fetuses thrive. Psychol Sci 2012;23:93–100
- West-Eberhard MJ. Developmental Plasticity and Evolution. New York: Oxford University Press, 2003
- Catalano R, Goodman J, Margerison-Zilko CE, Saxton KB, Anderson E, Epstein M. Selection against small males in utero: A test of the Wells hypothesis. Hum Reprod 2012;27:1202–8
- Hansen D, Moller H, Olsen J. Severe periconceptional life events and the sex ratio in offspring: Follow up study based on five national registers. BMJ 1999;319:548–9
- Kemkes A. Secondary sex ratio variation during stressful times: The impact of the French revolutionary wars on a German parish (1787–1802). Am J Hum Biol 2006;18:806–21
- Lyster WR. Altered sex ratio after the London smog of 1952 and the Brisbane flood of 1965. J Obstet Gynaecol Br Commonw 1974;81:626–31
- Obel C, Henriksen TB, Secher NJ, Eskenazi B, Hedegaard M. Psychological distress during early gestation and offspring sex ratio. Hum Reprod 2007;22:3009–12
- Saadat M. Decline in sex ratio at birth after Bam (Kerman Province, Southern Iran) earthquake. J Biosoc Sci 2008;40:935–7
- Catalano R, Bruckner T, Smith KR. Ambient temperature predicts sex ratios and male longevity. Proc Natl Acad Sci USA 2008;105:2244–7
- Grech V, Savona-Ventura C, Vassallo-Agius P. Research pointers: Unexplained differences in sex ratios at birth in Europe and North America. BMJ 2002;324:1010–11
- Helle S, Helama S, Jokela J. Temperature-related birth sex ratio bias in historical Sami: Warm years bring more sons. Biol Lett 2008;4:60–2
- Catalano R, Bruckner T. Male lifespan and the secondary sex ratio. Am J Hum Biol 2006;18:783–90
- Catalano R, Bruckner T. Secondary sex ratios and male lifespan: Damaged or culled cohorts. Proc Natl Acad Sci USA 2006;103:1639–43
- BOE. Official Spanish State Gazette 2013;11370–421
- García-Diego FJ, Pascual JJ, Marco-Jiménez F. Technical note: Design of a large variable temperature chamber for heat stress studies in rabbits. World Rabbit Sci 2011;19:225–31
- Garner DL, Johnson LA, Yue ST, Roth BL, Haugland RP. Dual DNA staining assessment of bovine sperm viability using SYBR-14 and propidium iodide. J Androl 1994;15:620–9
- Blasco A, Gomez E. A note on growth curves of rabbit lines selected on growth rate or litter size. Anim Prod 1993;57:332–4
- Blasco A, Ouhayoun J. Harmonisation of criteria and terminology in rabbit meat research. Revised proposal. World Rabbit Sci 1996;4:93–9
- Laird AK. Postnatal growth of birds and mammals. Growth 1965;30:349–63
- Hoffman AA, Parsons PA. Evolutionary Genetics and Environmental Stress. Oxford: Oxford University Press, 1991
- Gilbert SF, Epel D. Ecological Developmental Biology: Integrating Epigenetics, Medicine, and Evolution. Sunderland, MA: Sinauer, 2009
- Barker DJP. The fetal origins of adult disease. Fetal Maternal Med Rev 1994;6:71–80
- Bateson P, Barker D, Clutton-Brock T, Deb D, D’Udine B, Foley RA, et al. Developmental plasticity and human health. Nature 2004;430:419–21
- Fowden AL, Forhead AJ. Encocrine mechanisms of intrauterine programming. Reproduction 2004;127:515–26
- Newbold RR, Padilla-Banks E, Jefferson WN, Heindel JJ. Effects of endocrine disruptors on obesity. Int J Androl 2008;31:201–8
- Lau C, Rogers JM. Embryonic and fetal programming of physiological disorders in adulthood. Birth Defects Res C Embryo Today 2004;72:300–12
- McMillen IC, Muhlhausler BS, Duffield JA, Yuen BSJ. Prenatal programming of postnatal obesity: Fetal nutrition and the regulation of leptin synthesis and secretion before birth. Proc Nutr Soc 2004;63:405–12
- Rolland-Cachera MF, Thibault H, Souberbielle JC, Soulié D, Carbonel P, Deheeger M, et al. Massive obesity in adolescents: Dietary interventions and behaviours associated with weight regain at 2 y follow-up. Int J Obes Relat Metab Disord 2004;28:514–19
- Kinra S, Baumer JH, Davey Smith G. Early growth and childhood obesity: A historical cohort study. Arch Dis Child 2005;90:1122–7
- Langley-Evans SC, Bellinger L, McMullen S. Animal models of programming: Early life influences on appetite and feeding behaviour. Maternal Child Nutr 2005;1:142–8
- Guilloteau P, Waterland RA. Does nutrition during infancy and early childhood contribute to later obesity via metabolic imprinting of epigenetic gene regulatory mechanisms? Nestle Nutr Workshop Ser Pediatr Program 2005;56:157–71
- Mousseau TA, Fox CW. The adaptive significance of maternal effects. Trends Ecol Evol 1998;13:403–7
- Gilbert SF. Mechanisms for the environmental regulation of gene expression: Ecological aspects of animal development. J Bioscience 2005;30:65–74
- Monaghan P. Early growth conditions, phenotypic development and environmental change. Proc R Soc B 2008;363:1635–45
- Harris A, Seckl J. Glucocorticoids, prenatal stress and the programming of disease. Horm Behav 2011;59:279–89
- Henriksen R, Rettenbacher S, Groothuis TGG. Prenatal stress in birds: Pathways, effects, function and perspectives. Neurosci Behav R 2011;35:1484–501
- Harding JE. The nutritional basis of the fetal origins of adult disease. Int J Epidemiol 2001;30:15–23
- Benderlioglu Z, Dow E, Pyter LM. Neonatal exposure to short days and low temperatures blunts stress response and yields low fluctuating asymmetry in Siberian hamsters. Physiol Behav 2007;90:459–65
- Rhind SM, Rae MT, Brooks AN. Effects of nutrition and environmental factors on the fetal programming of the reproductive axis. Reproduction 2001;122:205–14
- Ward IL, Ward OB, Winn RJ, Bielawski D. Male and female sexual behavior potential of male rats prenatally exposed to the influence of alcohol, stress or both factors. Behav Neurosci 1994;108:1188–95
- Main KM, Mortensen GK, Kaleva MM, Boisen KA, Damgaard IN, Chellakooty Schmidt IM, et al. Human breast milk contamination with phthalates and alterations of endogenous reproductive hormones in infants three months of age. Environ Health Perspect 2006;114:270–6
- Menéndez-Patterson A, Fernandez F, Marin B. In uterus, immobilization stress and its effects on the development, behavior and sexual maturity of the rat. Revista Española de Fisiología 1982;38:433–40
- Ward IL, Weisz J. Differential effects of maternal stress on circulating levels of corticosterone, progesterone, and testosterone in male female rat fetuses and their mothers. Endocrinology 1984;114:1635–44
- Rogol AD, Roemmich JN, Clark PA. Growth at puberty. J Adolesc Health 2002;31:192–200
- Kuzawa CW, Bragg JM. Plasticity in human life history strategy: Implications for contemporary human variation and the evolution of genus Homo. Curr Anthropol 2012;53:S369–82
- Guilloteau P, Zabielski R, Hammon HM, Metges CC. Adverse effects of nutritional programming during prenatal and early postnatal life, some aspects of regulation and potential prevention and treatments. J Physiol Pharmacol 2009;60:17–35
- Sheffield PE, Landrigan PJ. Global climate change and children’s health: threats and strategies for prevention. Environ Health Perspect 2011;119:291–8
- Nevo E. Evolution under environmental stress at macro- and microscales. Genome Biol Evol 2011;2:1039–52
- Barker DJ. In utero programming of chronic disease. Clin Sci 1998;95:115–28
- Cameron N, Del Corpo A, Diorio J, McAllister K, Sharma S, Meaney MJ. Maternal programming of sexual behavior and hypothalamic–pituitary–gonadal function in the female rat. PLOS One 2008;21:e2210
- Monaghan P, Heidinger BJ, D’Alba L, Evans NP, Spencer KA. For better or worse: Reduced adult lifespan following early-life stress is transmitted to breeding partners. Proc Biol Sci 2012;279:709–14
- Owen D, Andrews MH, Matthews SG. Maternal adversity, glucocorticoids and programming of neuroendocrine function and behaviour. Neurosci Biobehav Rev 2005;9:209–26
- Weinstock M. The potential influence of maternal stress hormones on development and mental health of the offspring. Brain Behav Immun 2005;19:296–308
- Rodríguez N, Mayer N, Gauna HF. Effects of prenatal stress on male offspring sexual maturity. Biocell 2007;31:67–74