Abstract
Purpose: Unresectable chest wall recurrences of breast cancer (CWR) in heavily pretreated patients are especially difficult to treat. We hypothesised that thermally enhanced drug delivery using low temperature liposomal doxorubicin (LTLD), given with mild local hyperthermia (MLHT), will be safe and effective in this population.
Patients and methods: This paper combines the results of two similarly designed phase I trials. Eligible CWR patients had progressed on the chest wall after prior hormone therapy, chemotherapy, and radiotherapy. Patients were to get six cycles of LTLD every 21–35 days, followed immediately by chest wall MLHT for 1 hour at 40–42 °C. In the first trial 18 subjects received LTLD at 20, 30, or 40 mg/m2; in the second trial, 11 subjects received LTLD at 40 or 50 mg/m2.
Results: The median age of all 29 patients enrolled was 57 years. Thirteen patients (45%) had distant metastases on enrolment. Patients had received a median dose of 256 mg/m2 of prior anthracyclines and a median dose of 61 Gy of prior radiation. The median number of study treatments that subjects completed was four. The maximum tolerated dose was 50 mg/m2, with seven subjects (24%) developing reversible grade 3–4 neutropenia and four (14%) reversible grade 3–4 leucopenia. The rate of overall local response was 48% (14/29, 95% CI: 30–66%), with. five patients (17%) achieving complete local responses and nine patients (31%) having partial local responses.
Conclusion: LTLD at 50 mg/m2 and MLHT is safe. This combined therapy produces objective responses in heavily pretreated CWR patients. Future work should test thermally enhanced LTLD delivery in a less advanced patient population.
Introduction
Women with unresectable chest wall recurrence (CWR) of breast cancer are challenging patients to treat. Many have been irradiated previously. They often receive doxorubicin, as it has considerable efficacy and is the most frequently used systemic agent for breast cancer [Citation1–4]. When patients’ treatments fail following prior radiation and anthracycline therapy, clinicians face a dilemma as to what systemic agents and/or treatment modalities to use.
Here we describe the combination of mild hyperthermia with a thermally sensitive doxorubicin containing liposome for patients with chest wall recurrences of breast cancer. The logic for this combination is multifold: 1) Low temperature sensitive liposomal formulations of doxorubicin (LTLD) have been engineered to rapidly release high concentrations of drug when exposed to temperatures ranging from 39.5° to 42.0 °C [Citation5–7]. These liposomes exhibit enhanced drug delivery to tumour tissue as a result of intravascular release [Citation8]. 2) Hyperthermia partially reverses drug resistance to doxorubicin [Citation9,Citation10] and increases perfusion, which should enhance drug delivery [Citation11]. 3) There is a strong clinical indication for increasing the local control rate in patients with CWR. Morbidities associated with local recurrence of breast cancer on the chest wall include pain, ulceration, odour, bleeding, lymphoedema and the psychological distress of having visible local disease [Citation12]. Therapeutic options for patients with chest wall recurrence are limited. Local therapies such as re-irradiation with hyperthermia can achieve control in some patients [Citation13]. Although many patients with chest wall recurrences present with metastatic disease, a proportion can achieve durable local control and alleviation of symptoms [Citation13].
The objectives of this study were 1) to identify the maximum tolerated dose (MTD) of LTLD, (ThermoDox®) in the setting of local regionally recurrent disease in a heavily pretreated population, 2) to analyse pharmacokinetics (PK) when combined with local hyperthermia in multiple cycle dosing, and 3) to provide initial assessment of anti-tumour activity. LTLD has been studied in phase I trials in hepatocellular carcinoma [Citation14]. The MTD was found to be 50 mg/m2, after two dose-limiting toxicities were seen at a dose of 60 mg/m2 (grade 3 ALT increase, and grade 4 neutropenia).
Patients and methods
Two similarly designed phase I trials were undertaken. Trial A was an investigator-sponsored trial at Duke University Medical Center which ran from May 2006 to January 2010 and treated 18 patients. One other patient discontinued without completing cycle 1 due to a grade 2 hypersensitivity reaction to LTLD that resolved without sequelae (described in detail in the Results section). Trial B was a multi-center phase I trial sponsored by Celsion, conducted from April 2009 to March 2011. Eleven subjects were treated. Both studies were approved by the institutional review board. Investigators obtained informed consent from each participant.
Patient selection
For both trials all women had histologically documented recurrent/metastatic adenocarcinoma of the breast, <3 cm thick, to allow adequate heating. Patients with distant metastatic disease and/or inflammatory disease were allowed. All had to have progressed after at least one course of hormonal therapy, provided their tumour was oestrogen (ER) or progesterone (PR) positive. All patients had to have also received prior radiotherapy to their chest wall or breast either in the adjuvant or metastatic setting; no radiotherapy was to be administered to the area of recurrence within 14 days prior to enrolment. Patients had to have had systemic chemotherapy for recurrent disease (≥1 regimen in Trial A, ≥2 regimens in Trial B). All women had to be ≥18 years of age, not pregnant, able to provide consent, and have a Zubrod performance status of 0–1. Haematological indices had to be within normal limits and baseline MUGA/echocardiogram assessed left ventricular ejection fraction (LVEF) must have been ≥50%. Previous doxorubicin and epirubicin must not have exceeded 450 mg/m2 and 900 mg/m2, respectively. This limit is based on the dose equivalency for cardiotoxicity being 2:1 for doxorubicin to epirubicin.
Study design
Both phase I clinical trials were standard ‘3 + 3’ dose finding studies. Each patient was continued on the same dose level that they started on. The only time dose escalated was when the prior level was found to be safe in a previous cohort. Then the entire next cohort of patients started and stayed at the next higher level dose increment. Prior to each treatment cycle, subjects received a 24-h prophylactic premedication regime designed to reduce immediate hypersensitivity reactions. Patients were to receive six cycles of LTLD every 21–35 days, followed immediately by local hyperthermia to the chest wall. In Trial A, the initial dose level was 20 mg/m2 and sequential dose levels were to be 30, 40, 50, and 60 mg/m2, given intravenously over 30 min. In Trial B, the two dose levels were 40 and 50 mg/m2; if no more than one of six patients had a dose-limiting toxicity (DLT) at 50 mg/m2, then that dose level would be the MTD. LTLD was not planned to exceed the established MTD of 50 mg/m2 dose level.
All patients were premedicated with a standardised regimen including dexamethasone, diphenhydramine and an antacid. The prophylactic regimen followed the standard doxorubicin package insert. Dexamethasone 10 mg per os twice a day × three doses (the third dose was the morning of treatment). Approximately 1 h prior to the administration of the study drug, 500 mL of normal saline was infused intravenously \(IV). Approximately 30 min prior to the administration of the study drug, all of the patients received dexamethasone, diphenhydramine, and ranitidine.
Hyperthermia treatments were to be given within 30–60 min after completion of the LTLD infusion, using FDA-approved microwave or ultrasound superficial hyperthermia devices able to cover the target volume with a maximum of two hyperthermia fields. We used 915 MHz microwave applicators, with the BSD 500 (Salt Lake City, UT) commercial hyperthermia system. The applicator sizes available for use were a 19 × 10 cm, 15 × 8 cm, and a circular 6 cm applicator. An area of 16 × 8 cm falls within the 25% iso-SAR (specific absorption rate) for the largest applicator. The hyperthermia dose goal was to maintain 40.0–42.0 °C in greater than 90% of measured points for approximately 60 min duration. Maximum allowable temperatures in normal and tumour tissues were 44.0 °C and 45.0 °C, respectively.
Toxicities were assessed using the US National Cancer Institute Common Terminology Criteria for Adverse Events, v3.0. To be evaluable for MTD, patients must have completed the first day of cycle 3 (days 1–43) or withdrawn due to a DLT. Haematological DLTs included ≥grade 4 thrombocytopenia, febrile neutropenia, or grade 4 neutropenia ≥5 days in duration (≥7 days in Trial B); in Trial B, ≥grade 4 anaemia was also a DLT. Non-hematological DLTs included ≥grade 3 toxicities (except alopecia, nausea, and vomiting); in Trial B, ≥grade 3 fatigue was not a DLT.
Patient evaluation
The primary end point was to ascertain the MTD. Secondary end points included characterising the safety and pharmacokinetic profile of LTLD in multiple cycle dosing and computing the local objective response rate.
All subjects were assessed for safety with treatment-emergent adverse events (AEs), MUGA scans or echocardiograms (each patient had the same test done on all occasions), physical exams, vital signs, and laboratory measurements (including clinical chemistry, haematology, and urinalysis). Subjects were evaluated for efficacy at baseline, at cycles 3 and 5, and 21–42 days after cycle 6. Measurement of lesions in the hyperthermia treatment field was by clinical assessment. CT scans were used to assess any disease outside the hyperthermia treatment field. Objective responses were graded according to RECIST criteria [Citation15].
For the patients with recurrent chest wall disease there are limited imaging techniques that can assist clinicians in deciding the course of therapy and in evaluating the effectiveness of chemotherapeutic agents. In Trial A, digital infrared imaging (DII) was tested for its ability to estimate response and/or progression inside and outside the heated fields. Patients were imaged in a temperature controlled room with a digital infrared camera (IRSnapShot®, Fluke, Everett, WA) at baseline, prior to cycles 3, 5, and post-cycle 6. Regions of interest (ROI) were selected around the heated target lesions (TL) and non-heated target lesions (nTL) defined by the treating physician. The DII images were analysed using thresholding and basic averaging statistical tools. The average and maximum temperatures in each ROI were recorded for baseline and for each subsequent post-treatment image.
In Trial A, PK blood specimens were drawn in cycles 1 and 2 at approximately 0, 0.25, 0.5 (end of infusion), 0.75, 1.0, 1.25, 2, 3, 4, 6, 24 and 48 h and on days 4 and 8. We measured levels of doxorubicin and doxorubicinol, the latter being an active metabolite implicated in the cardiotoxicity of doxorubicin [Citation16]. Urine specimens were collected before the start of the infusion and at approximately 2, 4, and 24 h and on days 4 and 8. In Trial B, PK was assessed during the first two cycles of study drug administration by drawing four blood samples (4 mL each) at specified time intervals of 30 ± 2 min (i.e. at the end of the infusion) and at 5 ± 0.5 h, 10 ± 1 h, and 24 ± 1 h following the start of the infusion. Total urinary output was collected throughout the same 24-h period.
Thermal dosimetry was summarised as the tenth percentile of the temperature distribution of all sensors, or T90, meaning the temperature that is exceeded by 90% of all measurements (using fibreoptic thermometers) for each treatment [Citation17].
Two-tailed exact non-parametric tests are used for dose–response analysis and for comparing the two trials in demographics, treatments, and outcomes.
Results
Patient demographic and baseline characteristics
Patients in the two trials were similar on all 11 demographic and pretreatment characteristics except that Trial A subjects had fewer total prior treatments (, p = 0.0133) and a shorter time from CWR to first LTLD treatment (p = 0.0046).
Table 1. Summary of baseline characteristics.
Median age in the two trials was 57.4 years. Most subjects (55.2%) were triple negative and 44.8% had distant metastases at baseline. Patients recurred at a median of 7.4 months prior to their first study treatment.
Subjects in both trials were heavily pretreated. The mean and median prior anthracycline exposures were 304.1 mg/m2 and 256.5 mg/m2, respectively; the minimum total dose was 80.0 mg/m2 while the maximum was 570.0 mg/m2. The mean and median prior radiation exposures were 64.49 Gy and 61 Gy respectively; the minimum total dose was 19.80 Gy while the maximum was 168.10 Gy.
Study treatment
Both trials were similar in number of study treatments completed and in thermal doses (). Subjects completed a median of four study treatments. The thermal dose goal was to reach a temperature between 40° and 42 °C in greater than 90% of measured points (T90) for approximately 60 min duration. A total of 165 hyperthermia fields were treated. Of these 165 hyperthermia treatment fields, 58 (35.2%) had a T90 of less than 40.0 °C and the other 107 (64.8%) had a T90 between 40.0° and 42.9 °C. The minimum T90 was 36.0 °C and the maximum T90 was 42.6 °C. Of all 165 treatment fields, 33 (20%) had a T90 below 39.5 °C, the minimum temperature needed to release doxorubicin from LTLD. Eleven (37.9%) of 29 subjects had at least one treatment field with a T90 below 39.5 °C.
Table 2. Summary of study treatment.
Dose escalation and MTD
Thirteen subjects were evaluable for MTD in Trial A and 10 in Trial B. In Trial A, dose escalation continued to the 40 mg/m2 dose level, at which time Trial B initiated at 40 mg/m2 prior to reaching a MTD. In Trial A, one patient experienced a grade 2 hypersensitivity reaction that included a drug-related fever at 30 mg/m2; this was considered a DLT. Two of seven subjects at 40 mg/m2 experienced a DLT (grade 4 neutropenia lasting >5 days and grade 3 dehydration requiring hospitalisation for support for 27 days). In Trial B, one of six patients at the 50 mg/m2 dose level had a DLT (grade 3 hypokalaemia unrelated to study treatment), so the MTD was 50 mg/m2. Five of the six non-evaluable patients withdrew due to progression of disease during the first two treatment cycles and one for an unrelated adverse event.
Safety
Grade 4 toxicity was only observed in three (10.3%) patients with neutropenia and two (6.9%) with a neutrophil count decrease (). Grade 3 toxicity included six patients (20.7%) with a neutrophil count decrease, five (17.2%) with neutropenia, four (13.8%) with leucopenia, and three (10.3%) with a white blood cell count decrease. All myelotoxicities were reversible. All other grade 3 toxicities consisted of a single case of each of the following: anaemia, lymphopenia, thrombocytopenia, dehydration, axillary pain, fatigue, cellulitis, third degree burn adjacent to a silicone implant (Trial B), activated partial thromboplastin time-prolonged, haemoglobin decreased, hypokalaemia, back pain, musculoskeletal chest pain, musculoskeletal pain, and lymphoedema. Two subjects, both in Trial B, experienced first degree thermal burns that were managed conservatively and healed.
Table 3. Combined summary of grade 3–4 adverse events. (Subjects are counted only once within each system-organ class and preferred term, at the highest severity grade experienced. N = 29.).
There were no clinically significant cardiac toxicities; however, in Trial B, one asymptomatic decline in LVEF from 55% to 45% was recorded after six LTLD treatments and a prior anthracycline exposure of 240 mg/m2. In addition, there were no cases of hand–foot syndrome, which has been reported with the use of non-temperature-sensitive liposomal doxorubicin [Citation18].
Pharmacokinetics: Trial A
Plasma concentrations of doxorubicin achieved their maximum at the end of the 30-min infusion and subsequently declined rapidly (). As expected, plasma concentration of doxorubicin increased with each subsequent dose level (). The near superimposition of curves for cycles 1 and 2 demonstrate that there was no accumulation of drug between cycles. The average plasma clearance of doxorubicin was 1.3 L/min/m2, but there was considerable heterogeneity among patients. The average terminal plasma half-life for all patients was 35.15 min, but ranged from 9.26 to 45.27 min. There was no statistically significant difference between these variables among the various dose cohorts.
Figure 1. Pharmacokinetic profiles for cycles 1 and 2 at each dose level for doxorubicin and its cardiotoxic metabolite, doxorubicinol. The half-life of the drug was the same for cycle 1 and cycle 2 and was not dependent upon doxorubicin dose.
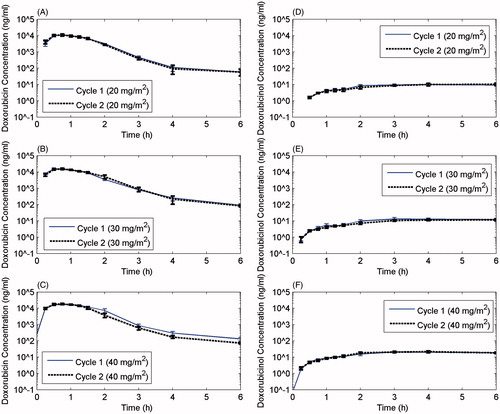
Table 4. Total doxorubicin and doxorubicinol plasma PK parameters for LTLD – Trial A.
Pharmacokinetics: Trial B
For Trial B sparse sampling results include the mean Cmax of doxorubicin ranged from 19 800 to 25 400 ng/mL in the 40 to the 50 mg/m2 dose range and between cycles. The mean Cmax of doxorubicinol ranged from 19.6 to 21.9 ng/mL in the 40–50 mg/m2 dose range and between cycles. Both doxorubicin and doxorubicinol exhibit linear (dose-independent) pharmacokinetics following a single dose in the 40–50 mg/m2 dose range. The within-subject variability in doxorubicin and doxorubicinol exposure was small between each administration cycle with mean cycle 2 vs cycle 1 ratios ranging from 0.99 to 1.06.
Efficacy
The local objective tumour response was similar in the two trials (, and Supplementary Tables 1–3). When combined, five (17.2%) complete local responses and nine (31%) partial local responses were seen. The rate of local response in these heavily pretreated patients was 48.3% (14/29, 95% CI: 30.1–66.5%). No dose–response relationship was observed (p = 0.4991). depicts response during therapy in one subject.
Figure 2. Evaluation of treatment course for a patient who achieved a complete response. (A) Photo of thermometry placement, and (B) position of the 18 × 10 cm applicator over the lesion. This lesion was treated in a single field using this applicator. The 25% isoSAR line encompassed the tumour region. (C) Appearance of the involved region prior to initiation of treatment. The margins of the tumour are easily seen by the red colour against the normal skin. (D) Appearance of the tumour area prior to cycle 3. The patient had achieved a partial response by this time point. (E) Thermographic camera image of chest well shows temperature distribution on the surface of the tumour region, prior to therapy. (F) Thermographic image of the chest wall prior to cycle 4 shows reduced surface temperature compared with baseline. (G) Thermographic images of the chest wall prior to cycle 5. By this time, the temperature of the involved region had reduced by 1 °C. This was most likely the result of reduced perfusion and metabolism, associated with tumour regression. This patient went on to achieve a complete response (not shown).
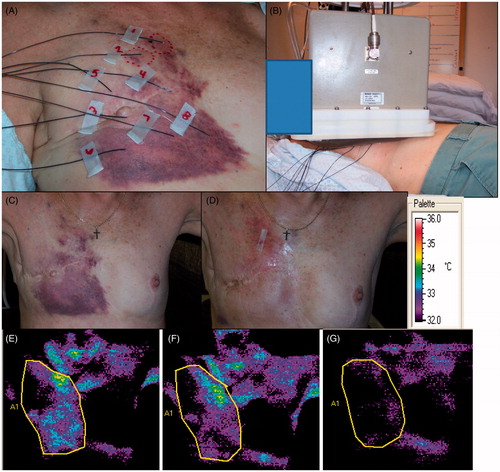
Table 5. Efficacy: Local objective response.
Table 6. Efficacy: Local objective response rates in the combined trials.
In Trial A, time to local progression (TTLP) and overall survival were additional end points (Supplementary Tables 2 and 3). There was a modest dose–response trend for TTLP, but it was not statistically significant.
In Trial B, ‘quality of life’ (QoL) was also an end point. LTLD and approved hyperthermia therapy resulted in clinically significant (≥8 point) improvements in patient self-assessed QoL on the FACT-B scale. Six of 11 subjects (54.5%, 95% CI: 25.1–83.9) had a clinically significant QoL improvement.
Discussion
LTLD was tolerated well by our heavily pretreated patients with four DLTs observed between two trials. Grade 3–4 neutropenia occurred in eight patients (27.6%) and was afebrile in all patients. Severe neutropenia and leucopenia can occur with doxorubicin. Such myelotoxicity was to be expected with anthracycline therapy. There were no dose-limiting cardiac toxicities seen in our patients and there were no symptomatic LVEF decreases seen on serial echo/MUGA imaging. This is similar to the cardiotoxicity profile commonly seen with non-temperature-sensitive liposomal doxorubicin, with very few cases of heart failure, and if any cardiac morbidity is seen it is often asymptomatic declines in LVEF [Citation18–24]. Two Trial B subjects experienced thermal burns, one grade 2 with possible radiation recall and one grade 3 event in a subject with a silicone breast implant. Mild to severe thermal injury is reported as a known side effect of hyperthermia [Citation25,Citation26].
While not the primary end point of the trials, the combined local response rate of 48% is quite impressive. However, the median time to local progression in Trial A was not long (4.9 months) and might be lengthened with technical improvements in delivery of hyperthermia and by treating future patients at the 50 mg/m2 MTD.
Twenty per cent of all treatment fields had a T90 below 39.5 °C, the minimum temperature needed to release doxorubicin from LTLD. Eleven (37.9%) of 29 subjects had at least one treatment field with a T90 below 39.5 °C. However, the local objective response rate of these 11 subjects was similar to that for the other 18 subjects (55% and 44% respectively, p = 0.7104). Higher temperatures throughout the tumour region would normally be expected to increase drug delivery. The maximal release rate of the commercial drug occurs at 41.3 °C. At temperatures > 43 °C, it remained elevated above baseline, but below peak [Citation27]. The performance of the commercial drug was the same as the original formulation [Citation6]. Thus, a modest improvement in minimum tumour temperatures without significantly increasing the maximum temperatures should prove useful. Moreover, it has been predicted mathematically and shown that maximal drug delivery with this drug formulation occurs when the drug is administered during heating, as opposed to heating after drug administration [Citation28,Citation29].
In these phase I studies, drug was administered before heating for practical and logistical reasons. It was not possible to perform drug infusion where the heating device was located. Unfortunately, due to the differences in time from when each patient received their infusion and subsequent heating, it was not possible to make meaningful interpretation of the heterogeneous pharmacokinetic values. Technical advances that would permit heating during chemotherapy administration could achieve as much as a two-fold increase in drug delivery [Citation28]. Doxorubicin has a terminal half-life reported in the 20–48-h range, a non-temperature-sensitive liposomal formulation of doxorubicin (Doxil®) has a half-life of approximately 55 h, whereas LTLD has a half-life considerably shorter, in the order of 35 min, regardless of dose. For LTLD, drug delivery could be substantially increased by heating during drug administration, one might be able to maximise the therapeutic benefit. Gasselhuber has considered these factors theoretically to demonstrate the influence of pharmacokinetics, release kinetics and rate of extravasation of drug on drug accumulation in the heated volume. Such models could be used in the design of future trials [Citation30].
This trial included many patients with disease that extended beyond the outer perimeter of the applicators available with the current microwave hyperthermia system. In order to improve average temperatures and corresponding thermal doses, large conformal water boluses were custom fitted to many patients with water–skin interface temperatures as high as 45°C for cases with only superficial disease (< 1 cm deep), in order to extend high temperatures out to the margins of disease. Larger microwave applicators that can conform to contoured patient anatomy and adjust the heating pattern to accommodate irregularly shaped diffuse disease would be expected to raise thermal dose and minimum tumour temperatures substantially compared to the centrally peaked heating patterns of rectangular box-shaped waveguide antennas used in this study.
Needham et al. have shown that drug release occurs at 40°C although maximal drug release rates occur nearer to 41.3°C, which is the transition temperature for this liposome [Citation6]. Of all 165 treatment fields, 33 (20%) had a T90 below 39.5 °C, the minimum temperature needed to release doxorubicin from LTLD. Thus, moving to larger conformal array microwave applicators that can effectively heat much larger areas of contoured anatomy above the required threshold temperature for drug release should significantly improve drug delivery to large area locoregional disease. Such applicators are becoming available for clinical use.
Prolonging time to local progression may also be possible with the addition of concurrent radiotherapy to LTLD. Radiotherapy plus hyperthermia can provide local control of superficial tumours [Citation25]; in recurrent disease, previously irradiated patients may benefit most [Citation26]. Treating with concurrent doxorubicin and radiotherapy has largely been abandoned due to the potent radiosensitisation and resultant morbidities seen with it in the past. However, there may be significant concerns with soft tissue and bone injury along with potential cardiac toxicity in the setting of left side CWR. But with careful planning and execution, safe administration may be possible. One possible solution is to use a thermobrachytherapy surface applicator to deliver a conformal radiation dose with restricted depth of penetration in combination with superficial heating and LTLD to localise treatment to the chest wall [Citation31–34].
Unfortunately, as is the case with many women who experience CWR, distant metastases are frequent and the majority of patients die of their disease. Trial A’s median TTLP of 4.9 months and OS of 9.0 months highlight the often poor prognosis of these patients; in Trial B, the subjects had had even more prior treatment. One might also consider heat sensitive liposomal formulations of other agents that might also be combined with local radiotherapy. Over half (55.2%) of our patients had triple negative CWRs, and there is ongoing interest in using PARP inhibitors in this cohort [Citation35]; the delivery of these agents might be enhanced with temperature-sensitive liposomal formulations.
Conclusion
Over half of the subjects had been diagnosed with a breast cancer recurrence at the chest wall more than 6 months (median 7.4 months, mean 13.7 months) prior to their first study treatment and 44.8% had distant metastases at baseline. Despite the encouraging rate of local response, seven of 29 subjects (24.1%) progressed outside the study treatment field. We were able to achieve local control in nearly half the patients as a salvage therapy with LTLD/hyperthermia treatment and it is reasonable to expect better results in a population with less advanced disease.
This thermally enhanced therapy is safe and produces objective responses in heavily pretreated CWR patients. It should be tested in a less advanced population.
Declaration of interest
Mark Dewhirst owns stock in Celsion, and David Needham is the inventor of ThermoDox®. Trial A was funded by US National Institutes of Health grant P01 NIH NCI CA42745 and Celsion. Trial B was funded by Celsion, who also supplied the LTLD (ThermoDox®). Clinical trials registration numbers are NCT00346229, NCT00826085. The authors alone are responsible for the content and writing of the paper.
Supplementary Material
Download PDF (25.5 KB)Acknowledgements
The authors would like to thank William L. Simonich, PhD, for his support in the manuscript, and Chelsea D. Landon, PhD, for editorial assistance.
References
- Rastogi P, Anderson SJ, Bear HD, Geyer CE, Kahlenberg MS, Robidoux A, et al. Preoperative chemotherapy: Updates of National Surgical Adjuvant Breast and Bowel Project Protocols B-18 and B-27. J Clin Oncol 2008;26:778–85
- Mamounas EP, Bryant J, Lembersky B, Fehrenbacher L, Sedlacek SM, Fisher B, et al. Paclitaxel after doxorubicin plus cyclophosphamide as adjuvant chemotherapy for node-positive breast cancer: Results from NSABP B-28. J Clin Oncol 2005;23:3686–96
- Citron ML, Berry DA, Cirrincione C, Hudis C, Winer EP, Gradishar WJ, et al. Randomized trial of dose-dense vs conventionally scheduled and sequential vs concurrent combination chemotherapy as postoperative adjuvant treatment of node-positive primary breast cancer: First report of Intergroup Trial C9741/Cancer and Leukemia Group B Trial 9741. J Clin Oncol 2003;21:1431–9
- Effects of chemotherapy and hormonal therapy for early breast cancer on recurrence and 15-year survival: An overview of the randomised trials. Lancet 2005;365:1687–717
- Kong G, Anyarambhatla G, Petros WP, Braun RD, Colvin OM, Needham D, et al. Efficacy of liposomes and hyperthermia in a human tumor xenograft model: Importance of triggered drug release. Cancer Res 2000;60:6950–7
- Needham D, Anyarambhatla G, Kong G, Dewhirst MW. A new temperature-sensitive liposome for use with mild hyperthermia: Characterization and testing in a human tumor xenograft model. Cancer Res 2000;60:1197–201
- Landon CD, Park J-Y, Needham D, Dewhirst MW. Nanoscale drug delivery and hyperthermia: The materials design and preclinical and clinical testing of low temperature-sensitive liposomes used in combination with mild hyperthermia in the treatment of local cancer. Open Nanomed J 2011;3:24–37
- Manzoor AA, Lindner LH, Landon CD, Park JY, Simnick AJ, Dreher MR, et al. Overcoming limitations in nanoparticle drug delivery: Triggered, intravascular release to improve drug penetration into tumors. Cancer Res 2012;72:5566–75
- Gaber MH. Modulation of doxorubicin resistance in multidrug-resistance cells by targeted liposomes combined with hyperthermia. J Biochem Mol Biol Biophys 2002;6:309–14
- Issels RD. Hyperthermia adds to chemotherapy. Eur J Cancer 2008;44:2546–54
- Dewhirst MW, Vujaskovic Z, Jones E, Thrall D. Re-setting the biologic rationale for thermal therapy. Int J Hyperthermia 2005;21:779–90
- Clemons M, Danson S, Hamilton T, Goss P. Locoregionally recurrent breast cancer: Incidence, risk factors and survival. Cancer Treat Rev 2001;27:67–82
- Zagar TM, Oleson JR, Vujaskovic Z, Dewhirst MW, Craciunescu OI, Blackwell KL, et al. Hyperthermia combined with radiation therapy for superficial breast cancer and chest wall recurrence: A review of the randomised data. Int J Hyperthermia 2010;26:612–17
- Poon RT, Borys N. Lyso-thermosensitive liposomal doxorubicin: A novel approach to enhance efficacy of thermal ablation of liver cancer. Expert Opin Pharmacother 2009;10:333–43
- Therasse P, Arbuck SG, Eisenhauer EA, Wanders J, Kaplan RS, Rubinstein L, et al. New guidelines to evaluate the response to treatment in solid tumors. J Natl Cancer Inst 2000;92:205–16
- Salvatorelli E, Menna P, Paz OG, Chello M, Covino E, Singer JW, et al. The novel anthracenedione, pixantrone, lacks redox activity and inhibits doxorubicinol formation in human myocardium: Insight to explain the cardiac safety of pixantrone in doxorubicin-treated patients. J Pharmacol Exp Therapeut 2013;344:467–78
- Dewhirst MW, Phillips TL, Samulski TV, Stauffer P, Shrivastava P, Paliwal B, et al. RTOG quality assurance guidelines for clinical trials using hyperthermia. Int J Radiat Oncol Biol Phys 1990;18:1249–59
- O’Brien ME, Wigler N, Inbar M, Rosso R, Grischke E, Santoro A, et al. Reduced cardiotoxicity and comparable efficacy in a phase III trial of pegylated liposomal doxorubicin HCl (CAELYX/Doxil) vs conventional doxorubicin for first-line treatment of metastatic breast cancer. Ann Oncol 2004;15:440–9
- Lorusso V, Manzione L, Silvestris N. Role of liposomal anthracyclines in breast cancer. Ann Oncol 2007;18:vi70–3
- Keller AM, Mennel RG, Georgoulias VA, Nabholtz JM, Erazo A, Lluch A, et al. Randomized phase III trial of pegylated liposomal doxorubicin vs vinorelbine or mitomycin C plus vinblastine in women with taxane-refractory advanced breast cancer. J Clin Oncol 2004;22:3893–901
- Chia S, Clemons M, Martin LA, Rodgers A, Gelmon K, Pond GR, et al. Pegylated liposomal doxorubicin and trastuzumab in HER-2 overexpressing metastatic breast cancer: A multicenter phase II trial. J Clin Oncol 2006;24:2773–8
- Coleman RE, Biganzoli L, Canney P, Dirix L, Mauriac L, Chollet P, et al. A randomised phase II study of two different schedules of pegylated liposomal doxorubicin in metastatic breast cancer (EORTC-10993). Eur J Cancer 2006;42:882–7
- Al-Batran SE, Bischoff J, von Minckwitz G, Atmaca A, Kleeberg U, Meuthen I, et al. The clinical benefit of pegylated liposomal doxorubicin in patients with metastatic breast cancer previously treated with conventional anthracyclines: A multicentre phase II trial. Br J Cancer 2006;94:1615–20
- Verma S, Dent S, Chow BJ, Rayson D, Safra T. Metastatic breast cancer: The role of pegylated liposomal doxorubicin after conventional anthracyclines. Cancer Treat Rev 2008;34:391–406
- Jones EL, Oleson JR, Prosnitz LR, Samulski TV, Vujaskovic Z, Yu D, et al. Randomized trial of hyperthermia and radiation for superficial tumors. J Clin Oncol 2005;23:3079–85
- Vujaskovic Z, Kim DW, Jones E, Lan L, McCall L, Dewhirst MW, et al. A phase I/II study of neoadjuvant liposomal doxorubicin, paclitaxel, and hyperthermia in locally advanced breast cancer. Int J Hyperthermia 2010;26:514–21
- Mills JK, Needham D. Lysolipid incorporation in dipalmitoylphosphatidylcholine bilayer membranes enhances the ion permeability and drug release rates at the membrane phase transition. Biochim Biophys Acta 2005;1716:77–96
- Ponce AM, Viglianti BL, Yu D, Yarmolenko PS, Michelich CR, Woo J, et al. Magnetic resonance imaging of temperature-sensitive liposome release: Drug dose painting and antitumor effects. J Natl Cancer Inst 2007;99:53–63
- Gasselhuber A, Dreher MR, Rattay F, Wood BJ, Haemmerich D. Comparison of conventional chemotherapy, stealth liposomes and temperature-sensitive liposomes in a mathematical model. PLOS ONE 2012;7:e47453
- Gasselhuber A, Dreher MR, Partanen A, Yarmolenko PS, Woods D, Wood BJ, et al. Targeted drug delivery by high intensity focused ultrasound mediated hyperthermia combined with temperature-sensitive liposomes: Computational modelling and preliminary in vivo validation. Int J Hyperthermia 2012;28:337–48
- Arunachalam K, Craciunescu OI, Maccarini PF, Schlorff JL, Markowitz E, Stauffer PR. Progress on thermobrachytherapy surface applicator for superficial tissue disease. Proc SPIE 2009;7181. doi: 10.1117/12.809493
- Arunachalam K, Maccarini PF, Craciunescu OI, Schlorff JL, Stauffer PR. Thermal characteristics of thermobrachytherapy surface applicators for treating chest wall recurrence. Phys Med Biol 2010;55:1949–69
- Stauffer PR, Schlorff JL, Juang T, Neuman JDG, Johnson JE, Maccarini PF, et al. Progress on system for applying simultaneous heat and brachytherapy to large-area surface disease. Proc SPIE 2005;5698:82–96
- Taschereau R, Stauffer PR, Hsu IC, Schlorff JL, Milligan AJ, Pouliot J. Radiation dosimetry of a conformal heat-brachytherapy applicator. Technol Cancer Res Treat 2004;3:347–58
- Krawczyk PM, Eppink B, Essers J, Stap J, Rodermond H, Odijk H, et al. Mild hyperthermia inhibits homologous recombination, induces BRCA2 degradation, and sensitizes cancer cells to poly (ADP-ribose) polymerase-1 inhibition. Proc Natl Acad Sci 2011;108:9851–6