Abstract
Purpose: The bone regenerative healing process is often prolonged, with a high risk of infection particularly in elderly and diseased patients. A reduction in healing process time usually requires mechanical stress devices, chemical cues, or laser/thermal therapies. Although these approaches have been used extensively for the reduction of bone healing time, the exact mechanisms involved in thermal stress-induced bone regeneration remain unclear. Methods: Photothermal stress (PTS) stimulation was carried out using a novel photothermal device, composed of an alginate gel (AG) including carbon nanotubes (CNT-AGs) and their irradiator with near-infrared (NIR) light. We investigated the effects of optimal hyperthermia on osteogenesis, its signalling pathway in vitro and mineral deposition in tooth-extracted sockets in vivo. Results: The PTS (10 min at 42 °C, every day), triggered by NIR-induced CNT, increased the activity of alkaline phosphatase (ALP) in mouse osteoblast MC3T3-E1 cells in a time-dependent manner compared with the non-thermal stress control. PTS significantly induced the expression of osteogenic-related molecules such as ALP, RUNX2 and Osterix in a time-dependent manner with phosphorylated mitogen-activated protein kinases (MAPK). PTS increased the expression of heat shock factor (HSF) 2, but not HSF1, resulting in activation of heat shock protein 27. PTS significantly up-regulated mineral deposition in tooth-extracted sockets in normal and ovariectomised osteoporotic model mice in vivo. Conclusions: Our novel CNT-based PTS up-regulated osteogenesis via activation of heat shock-related molecules, resulting in promotion of mineral deposition in enhanced tooth-extracted sockets.
Introduction
Heat stress response is the protective reaction of cells to a variety of environmental and pathological stimuli [Citation1]. The most obvious feature of the heat stress response is an increase in synthesis of heat shock proteins (HSPs) [Citation2]. HSPs act as chaperones [Citation3] and play a role in anti-apoptotic processes [Citation4]. They promote cell survival [Citation5] and are thought to function in the protection and recovery of cells from environmental and pathological stress [Citation2]. However, little information is available on the expression of HSPs during bone remodelling. Furthermore, the exact mechanisms involved during heat stress-induced osteogenesis remain unclear. It is therefore necessary to develop a new device capable of applying fast and fine controllable heat stress stimulation.
Carbon nanotubes (CNTs) possess exceptional mechanical [Citation6,Citation8], thermal [Citation9] and electrical properties [Citation10], making them useful and valuable materials in science and technology. The application of medical biomaterials using CNTs has been reported for clinical use [Citation11–13]. CNTs are predominantly reported for their use in drug delivery and cancer therapy [Citation14–16]. The application of CNTs in bone repair is expected to predominantly improve overall mechanical properties, for example in high-strength fixation plates and screws for arthroplasty prostheses. Interestingly, CNTs can be effectively heated at various wavelengths because of their strong absorbance over a wide range of the optical spectrum [Citation17]. This exothermic reaction, following irradiation by near-infrared (NIR) light, has been reported to be used successfully for hyperthermia in cancer therapy [Citation18,Citation19]. We therefore considered the use of CNT for photothermal stress (PTS) stimulation, composed of carbon nanotubes (CNT) and their irradiator, NIR light. It could potentially be used for photothermal therapy in the treatment of bone defects. We therefore considered that CNT could be used as an exothermic hyperthermic device for bone repair.
The aim of the present study was to clarify whether PTS, triggered by NIR-induced CNT, is an effective thermal therapy for osteogenesis and its signalling pathways in vitro. Furthermore, we examined the effect of PTS on bone mineral deposition in tooth-socket defects in vivo using normal and osteoporotic mice.
Materials and methods
Cell culture
Mouse osteoblast MC3T3-E1 cells were cultured in Dulbecco’s modified Eagle’s medium (DMEM) containing 10% fetal bovine serum (FBS) at a density of 1 × 105 cells/mL for 1, 2, 3, 5, 7 and 10 days at 37 °C in an atmosphere of 5% CO2. On reaching sub-confluence, cells were cultured in DMEM with 10% FBS supplement with bone morphogenic protein (BMP)-4 (10 ng/mL, PeproTech, Rocky Hill, NJ, USA) or osteogenic induction medium (OIM) (ascorbic acid (50 µg/mL), dexamethasone (10−6 M) and β-glycerophosphate (10 mM)).
Quantitative reverse transcription-polymerase chain reaction
Total RNA was extracted from cells using TRIzol® reagent. First-strand cDNA was synthesised from 3 μg of total RNA using SuperScript II reverse transcriptase, according to the manufacturer’s instructions (Invitrogen, Carlsbad, CA). To detect mRNA expression, we selected specific primers based on the nucleotide sequence of cDNA (). The cDNA was amplified by polymerase chain reaction (PCR) under the following condition: 30 s denaturation at 94 °C, 30 s annealing at 52–55 °C, and 1 min extension at 72 °C, for 28–35 cycles. PCR products were subjected to electrophoresis on 2% agarose gels and visualised after staining with ethidium bromide. The signal intensity of each PCR product was quantitatively measured with Scion Image software (version 4.02, National Institutes of Health, Bethesda, MD) and normalised to β-actin mRNA. In some experiments quantitative reverse transcription (qRT)-PCR analyses of targeted mRNAs were performed using SYBR PrimeScript RT-PCR kit and ABI 7500 Real-Time PCR System and software (Applied Biosystems, Foster City, CA). Reaction conditions were: an initial step at 95 °C for 30 s, followed by 40 cycles of denaturation at 95 °C for 5 s, annealing at 60 °C for 10 s and extension at 72 °C for 35 s. Beta-actin was used as an internal standard to control for variability in amplification due to differences in starting mRNA concentrations. The relative expression of target mRNA was computed from the target threshold cycle (Ct) values and beta-actin Ct values by means of the standard curve method.
Table 1. RT- and qRT-PCR primers sequences.
Western blot analysis
Cells were lysed in Tris-NaCl-Tween (TNT) buffer containing 20 mM Tris-HCl (pH 7.5), 200 mM NaCl, 1% Triton-X 100, 1 mM DTT and protease inhibitors (Roche, Basel, Switzerland). The protein content of the samples was measured using Pierce reagents following the manufacturer’s protocol. Protein samples of 20 µg were subjected to sodium dodecyl sulphate-polyacrylamide gel electrophoresis and the proteins were then transferred to a PVDF membrane (100 V, 1 h, 4 °C). The membranes were then incubated with anti-alkaline phosphatase, anti-ALP, anti-Sp7/Osterix (Osx), anti-HSF1, anti-HSF2, anti-pHsp27 and anti-β-actin antibodies at optimal dilutions in 5% (w/v) skimmed milk solution, supplemented with 0.01% (w/v) azide, overnight at 4 °C. Blots were washed in TTBS (10 mM Tris-HCl, 50 mM NaCl, 0.25% Tween 20) and incubated with an appropriate secondary antibody for 30 min at room temperature. The immunoreactive proteins were visualised using enhanced ECL chemiluminescence reagents (GE Healthcare, Tokyo, Japan).
Alkaline phosphatase (ALP) assay
The protein content of the samples was measured using Pierce reagents following the manufacturer’s protocol. The protein samples of 1 µg were subjected to ALP assay. After incubation for each time period with OIM, ALP activity was measured with a phosphatase substrate kit (AnaSpec, Fremont, CA), according to the manufacturer’s instructions. Optical densities were measured on a plate reader at 405 nm as the detection wavelength. Results were obtained from five samples and data are presented as the mean ± standard error of the mean (SEM).
Preparation of the alginate gel including carbon nanotubes (CNT-AG)
To prepare water-soluble CNTs (400 mg, single-walled type, average diameter 1 nm, average length 800 nm, CG100, South West Nanotechnology, Norman, OK), CNTs were mixed with 300 mL of sulphuric acid and 100 mL of nitric acid, according to a previous report [Citation20]. The resulting solid was then washed with deionised water and dried in a vacuum oven for 24 h at 80 °C. Sodium alginate (2 g, Wako, Osaka, Japan) and CNTs (10 mg) were dissolved in 100 mL of deionised water. The solution was poured into a plastic mould and then covered by a filter paper wetted with 2% calcium chloride solution for 3 h to set the solution into a CNT-alginate gel (CNT-AG). The CNT-AG was then carefully removed from the mould and immersed in 2% calcium chloride solution for 24 h to achieve complete gel processing. The prepared CNT-AG was cut into disc-shaped specimens (10-mm diameter and 1-mm thickness). From preliminary evaluation, the CNT-AG discs showed strong heat emission following irradiation with NIR light. Because this exothermic generation could be controlled by the NIR irradiation conditions, the CNT-AG disc was considered a promising candidate as a photothermal device.
PTS stimulation
PTS stimulation in vitro and in vivo was carried out by irradiation of MC3T3-E1 osteoblasts in 24-well plates and tooth-extracted sockets in mice, respectively. The cells in 24-well multi-plates were irradiated with CNT-AG (15 mm diameter) using the NIR apparatus (4.0 W/cm2, LA-100 IR, Hayashi, Tokyo), according to previous reports [Citation20]. The light tip of the NIR apparatus was located at a distance of 3 cm from the surface of the bottom of each well in the multi-plates (, Supplemental Figure 1). Each well was exposed to NIR light for 10 min at 42 °C every day for 10 consecutive days. For in vivo analysis, tooth-extracted sockets were also irradiated with CNT-AG using the NIR apparatus in control, sham-operated and ovariectomised ddY mice. The CNT-AG patch was set into the oral cavity and attached to the tooth-extracted sockets when mice were under deep anaesthesia using isoflurane (). The light tip of the NIR apparatus was located at a distance of 3 cm from the bottom of the tooth-extracted sockets and exposed to NIR light for 10 min at 42 °C every day for 8 weeks. All procedures using animals were approved by the Council on Animal Care of Fukuoka Dental College (No. 13007) and were performed in accordance with the Ethics Guidelines for Animal Experiments at Fukuoka Dental College.
Figure 1. Images of the experimental device arrangement for photothermal stress (PTS) stimulation under in vitro (A) and in vivo (B) conditions. The PTS device was composed of alginate gel (AG), comprising carbon nanotubes (CNT-AG) and a near-infrared ray (NIR) irradiator. In both conditions the top of the NIR irradiator was fixed 3 cm distant from the CNT-AG disc. (A) In vitro stimulation: the CNT-AG disc was attached to the bottom of the multi-well plates and NIR was irradiated for 10 min at 42 °C every day. (B) In vivo stimulation: the CNT-AG disc was placed into the extracted-tooth socket in the mouse oral cavity and irradiated for 10 min at 42 °C every day (reference to Supplemental Figure 1).
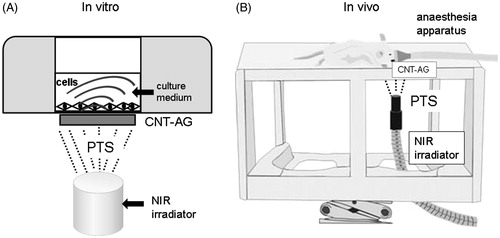
Micro-CT analysis
Right first maxilla molars were extracted in ten-week-old male ddY mice under deep anaesthesia using isoflurane. PTS stimulation was applied to the cavity at 42 °C for 10 min every day beginning at day1 after tooth extraction for 42 consecutive days. Maxilla bone-extracted teeth were collected at 0, 1, 2, 3, 4 and 6 weeks in the presence or absence of PTS stimulation and fixed by 4% paraformaldehyde for 24 h. In some experiments, six-week-old female ddY mice underwent either a sham-operation or were ovariectomised. After 4 weeks, right first maxilla molars were extracted under deep anaesthesia using isoflurane. Images were photographed by a micro-CT device (SkyScan 1176, TOYO, Tokyo). The image parameters were set at a 50 kV X-ray source with a 0.5 mm aluminium filter. Corrected images were set at 9 μm for one slice, which was reconstructed with NRecon software (SkyScan). The bone mineral density (BMD), bone volume/total volume (BV/TV), and trabecular Thickness (TbTh) in the samples were analysed using CTAN software (SkyScan).
Reagents
Recombinant human BMP4 was purchased from Peprotec (Minneapolis, MN). Anti-alkaline phosphatase, anti-Sp7/Osx, anti-HSF1, and anti-HSF2 antibodies were purchased from Abcam (Tokyo). All other antibodies were purchased from Cell Signaling Technologies (Denver, MA). All other chemicals were obtained from Sigma (St Louis, MO).
Statistics
Data are expressed as means ± standard error of the mean (SEM). Differences were analysed with one-way analysis of variance (ANOVA) and Scheffe’s multiple comparison tests. p Values of < 0.05 were considered to be significant.
Results
Osteogenesis up-regulation by CNT-base PTS
It is reported that mild heat stress induces ALP activity, representative of osteogenesis, in human osteoblast cell line MG63 cells [Citation21]. To clarify whether the PTS stimulation triggered using the CNT-AG and NIR device up-regulates osteogenesis, we examined the effects of PTS on the expression of osteogenic-related proteins and ALP activity using mouse osteoblast cell line MC3T3-E1 cells. Incubation of MC3T3-E1 cells with OIM gradually induced the expression of osteogenic-related molecules, including ALP, RUNX2 and Osx in a time-dependent manner (). Treatment with BMP-4 also increased expression of these osteogenic-related proteins in a time-dependent manner. These osteogenic-related proteins were expressed in order of ALP, RUNX2 and then Osx after exposure to OIM. Furthermore, PTS on day 1 significantly up-regulated the expression of osteogenic-related molecules at day 1 after osteogenic stimulation, compared with the control without PTS. Using Western blot analysis, ALP activity increased significantly in a time-dependent manner after PTS, compared with the control.
Figure 2. PTS promoted osteogenesis in mouse osteoblasts MC3T3-E1 cells. (A) Cells were cultured prior to performing Western blot analysis for alkaline phosphatase (ALP), RUNX2, Osx, and osteocalcin with or without PTS at 0, 1, 3, 5 and 7 days after replacement of medium with osteogenic induction medium (OIM) or BMP-4 (10 ng/mL). (B) ALP activity of MC3T3-E1 cells cultured with or without PTS at 0, 1, 3, 5, 7 and 10 days after replacement with OIM. Data shown are the mean from six wells (mean ± SEM). **p < 0.01, unstimulated versus PTS-stimulated group.
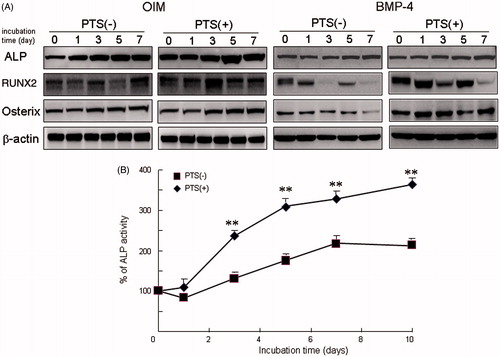
PTS induced heat shock-related molecules
HSPs are reported to be abundant within cells and their levels increase rapidly in response to heat stress [Citation22]. Furthermore, heat shock factors (HSFs) are master regulators of HSP expression during heat stress in mammals. We therefore examined the effect of PTS on the expression of HSFs during osteoblast differentiation. HSF1 mRNA and protein were constantly expressed during osteogenesis, following exposure to OIM. Interestingly, PTS had no effect on the expression of HSF1 ( and ). In contrast, HSF2 mRNA and protein were slightly increased during osteogenesis. Furthermore, PTS significantly up-regulated expression of HSF2 at day after PTS stimulation. Although OIM exposure without PTS barely induced phosphorylated Hsp27 (pHsp27) on day 7, PTS greatly increased the expression of pHsp27 in a time-dependent manner, which peaked at day 1, compared with the control. Although OIM exposure transiently increased Mitogen-activated protein kinase (MAPK) activity, PTS gradually up-regulated expression of phosphorylated MAPK proteins such as p-p38 and p-ERK1/2.
Figure 3. Effect of PTS on expression of heat shock factors (HSFs) after replacement with OIM. (A) Expression of HSF1 and HSF2 mRNAs were analysed semi-quantitatively by RT-PCR in MC3T3-E1 cells. (B) Effect of PTS on expression levels of HSF1 and HSF2 mRNAs were quantitatively analysed by qRT-PCR. Data shown are the mean from six wells (mean ± SEM). **p < 0.01, unstimulated versus PTS-stimulated group.
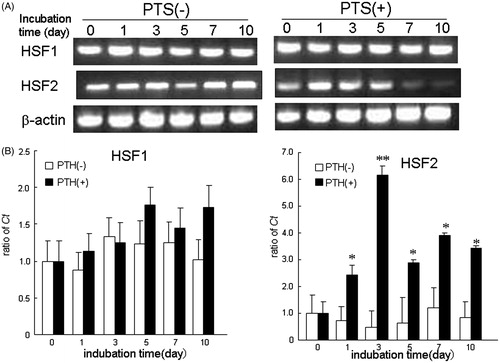
Figure 4. PTS up-regulated the expression of heat-shock-related MAPK molecules in MC3T-E1 cells. Total cell lysates were prepared and Western blot analysis was carried out using targeted and anti-β actin antibodies. MC3T3-E1 cells were stimulated with PTS every day for the indicated culture periods. Similar results were obtained from four independent experiments.

PTS induced mineral deposition in tooth-extracted sockets
To clarify the effects of PTS on alveolar bone deposition, we examined the effect of PTS on mineral deposition in tooth-extracted sockets using normal mice and an osteoporosis mouse model. PTS was applied to the tooth-extracted sockets every day from day 1 after extraction of the right first maxilla molars until day 21. Bone mineral was gradually deposited in the control mice and restored to the original level by day 21 after tooth extraction (). In contrast, PTS transiently increased the BMD, BV/TV, and TbTh in the tooth-extracted sockets on day 7 after extraction compared with control mice (). Furthermore, the ovariectomy-induced osteoporotic (OVX) mice treated had no deposition on day 7 after extraction and a significantly decreased BMD in the sockets on days 7, 14 and 28 after extraction compared with control mice ( and ). In contrast, simultaneous PTS stimulation in ovariectomised mice significantly increased the BMD in the sockets on day 7 after extraction, indicating that PTS significantly restored bone deposition even in osteoporotic mice ().
Figure 5. PTS transiently up-regulated mineral deposition in extracted-tooth sockets. (A) Right first maxilla molars were extracted from male ddY mice at 10 weeks of age under deep anaesthesia using isoflurane. PTS was applied to the extracted-tooth sockets until 21 days after tooth extraction. (B) Bone mineral deposition (BMD) in the samples was continuously analysed with or without PTS stimulation in the extracted-tooth sockets using CTAN software. The percentage of bone volume versus total bone volume (BV/TV) and trabecular bone thickness (TbTh.) were also analysed at day 7 after tooth extraction using CTAN software. Data are means ± SEM from six culture wells. *p < 0.05.
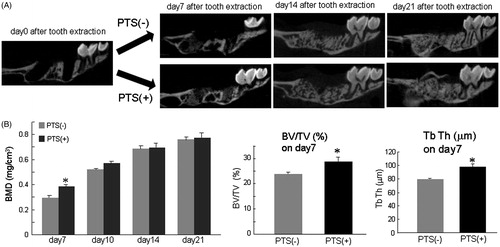
Figure 6. Ovariectomised osteoporotic mice had decreased mineral deposition in extracted-tooth sockets and PTS suppresses this inhibition. (A) Female ddY mice at 6 weeks of age were ovariectomised and the right first maxilla molars were extracted 4 weeks after ovariectomy under deep anaesthesia using isoflurane. PTS was applied to the extracted-tooth sockets until 28 days after tooth extraction. (B) BMD in the samples was analysed using CTAN software. Data are means ± SEM from six culture wells. *p < 0.05. OVX, ovariectomy.
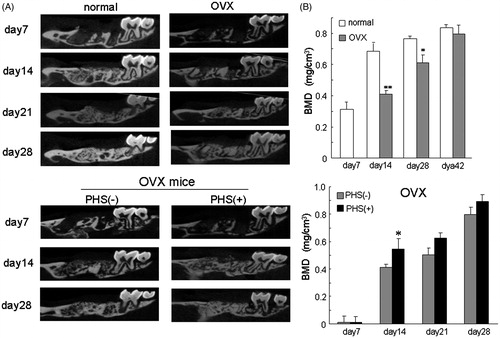
Discussion
Bone regeneration is reported to be facilitated by extracellular stimuli, including mechanical stress devices, chemical cues, or laser/thermal therapies [Citation23,Citation24]. In the present study we investigated the effect of a novel photothermal device, composed of AG with CNTs and an NIR irradiator, used for hyperthermia therapy, for osteogenic stimulation in vitro and in vivo. The PTS triggered by the device up-regulated alveolar bone repair with enhanced expression of osteogenic-related genes in osteoblasts via HSP activation.
It has been reported that using only one heating cycle fails to elicit any significant heat-shock enhancement of osteogenesis [Citation25]. In contrast, mild repetitive heat stress (39–42 °C) induces osteogenesis, measured by increased ALP activity in MG63 cells [Citation21]. An optimal temperature of 42 °C has also been reported to induce heat shock promoter-mediated gene expression in NIH-3T3 cells [Citation26]. Therefore, repetitive PTS was applied to maintain a thermal effect for 10 min at 42 °C every day, to assess bone repair and osteoblast differentiation.
HSFs are reported to be recognised and bound to heat shock elements present in the promoter regions of the HSP family [Citation27]. HSF1 and HSF2 differ in their pathways of activation and the nature of their transcriptional responses. HSF1 is reported to be activated upon exposure to a multitude of physiological and environmental stresses [Citation28]. However, HSF2 activity appears to be more selective during differentiation [Citation29,Citation30] and in early development [Citation31,Citation32].
HSPs are present in significant amounts, even in several unstressed cells, where they may have essential functions [Citation33,Citation34]. Hsp27, Hsp47 and Hsp70 are reported to be highly expressed in osteoblasts in newly formed bone areas [Citation35]. Heat stress stimulation and pretreatment with oestrogen has been found to induce the expression of Hsp27 in osteoblasts [Citation36,Citation37]. Furthermore, treatment with FGF-2 and prostaglandins has also been reported to induce proliferation of MC3T3-E1 cells, with Hsp27 accumulation [Citation38,Citation39]. Interestingly, treatment with endothelin-1 and sphingosine 1-phosphate are reported to induce Hsp27, not through ERK1/2 MAPK, but through p38 MAPK activation in these cells [Citation40,Citation41]. The transient increase in Hsp27 mRNA has been shown to down-regulate proliferation in osteoblasts [Citation36,Citation37]. We found that PTS induced the expression of Hsp27 with phosphorylation of MAPK molecules p-38 and ERK1/2 in MC3T3-E1 cells, resulting in promotion of osteogenesis. The up-regulation of Hsp27 suggests that protection is offered from external stress, whilst also modulating several important molecules relevant to osteogenesis. These results suggest that PTS may up-regulate the expression of heat-shock-related molecules, such as HSP and HSF, as well as osteogenic-related molecules in osteoblasts, resulting in promotion of alveolar mineral deposition in tooth-extracted sockets. However, the detailed mechanism of PTS-induced bone repair remains unclear. Further studies will address the transcriptional and/or epigenetic mechanism of PTS-activation in the up-regulation of bone formation in vivo and in vitro.
Devices of exothermic generation have been developed using ultra violet (UV), short-wavelength visible, and IR laser light heat to induce a thermal response. Although these light sources have poor penetration into the body, NIR light can penetrate at least 10 cm through deep tissue [Citation42,Citation43]. Remarkably, CNTs possess electrical, chemical, mechanical, and thermal properties, indicating them as useful and valuable materials in science and technology. Furthermore, CNTs can be effectively heated at various wavelengths because of their strong absorbance over a wide range of the optical region. The novel device used in this study, composed of CNT-AG and its NIR, has beneficial characteristics as follows; (1) The CNT-AG material can change its arbitrary shape after cutting, (2) The device temperature set-up point is easily controlled, (3) The device is convenient to move, (4) The PTS stimulation triggered by the device penetrates the deep tissues. Thus, for first time we have shown that CNTs can be used as an exothermic molecular device for hyperthermia in alveolar bone regeneration in extracted-tooth sockets. Furthermore, our data indicate that PTS stimulation strongly up-regulated bone deposition in normal osteoporotic mice following ovariectomy. However, little is known about why PTS transiently enhanced bone deposition only at day 7 in normal mice and day 14 in ovariectomied mice, but not other days. HSF1 and phosphorylated Hsp27 proteins transiently increased on day 5–7 and returned to basal expression level over day 7 after PTS, suggesting in the enhancement of the alveolar bone deposition in the early phase during bone regeneration.
Hyperthermia therapy has been shown to stimulate bone remodelling and the formation of new bone, thus increasing cortical bone density [Citation44–46]. The hyperthermal stimulation (1.5–3.0 °C above normal) stimulates the longitudinal and concentric growth of the femur and tibia in growing rats and dogs [Citation47]. These reports suggest that hyperthermia accelerates local bone formation. Mild heat shock is also reported to induce proliferation, ALP activity and mineralisation of bone marrow stromal cells [Citation21]. Our results, taken together with those of previous reports, indicate that the CNT-based PTS also facilitates osteogenesis via activation of heat-shock-related molecules, and appears to be a promising hyperthermia therapy for bone repair treatment. The present study used a novel device which is easy to control and is portable, both of which have a great advantage in hyperthermia therapy.
In summary, osteogenesis and bone mineral deposition are temperature dependent. Exposure to optimal PTS stimulation transiently promoted osteoblast differentiation via heat shock-related molecules, including HSF2 and Hsp27, which might be key components involved in the osteogenesis of cells during temperature changes. Furthermore, exposure to PTS potentiated alveolar bone mineralisation. This temperature-induced osteogenesis suggests there may be a potential use for thermotherapy in patients with osteoporosis and periodontal disease to promote bone healing.
Supplementary material available online
Supplemental File
Download TIFF Image (575.3 KB)Declaration of interest
This work was supported by Grants-in-Aid for Scientific Research from the Ministry of Education, Culture, Sports, Science and Technology of Japan (Nos. 24592823 and 23390455) and by a Grant-in-Aid for Strategic Study Base Formation Support Business (S1001059) from the Japan Society for the Promotion of Science.
All authors declare no conflict interest.
References
- Lindquist S, Craig EA. The heat-shock proteins. Annu Rev Genet 1988;22:631–77
- Sorger PK. Heat shock factor and the heat shock response. Cell 1991;65:363–6
- Jakob U, Gaestel M, Engel K, Buchner J. Small heat shock proteins are molecular chaperones. J Biol Chem 1993;268:1517–20
- Mehlen P, Mehlen A, Godet J, Arrigo AP. Hsp27 as a switch between differentiation and apoptosis in murine embryonic stem cells. J Biol Chem 1997;272:31657–65
- Lavoie JN, Gingras-Breton G, Tanguay RM, Landry J. Induction of Chinese hamster Hsp27 gene expression in mouse cells confers resistance to heat shock. Hsp27 stabilization of the microfilament organization. J Biol Chem 1993;268:3420–9
- Ruoff, RS, Lorents, DC. Mechanical and thermal properties of carbon nanotubes. Carbon 1995;33:925–30
- Treacy, MJ, Ebbesen TW, Gibson JM. Exceptionally high Young’s modulus observed for individual carbon nanotubes. Nature 1996;81:678–80
- Miyagawa H, Misra M, Mohanty AK. Mechanical properties of carbon nanotubes and their polymer nanocomposites. J Nanosci Nanotechnol 2005;5:1593–615
- Berber S, Kwon YK, Tomanek D. Unusually high thermal conductivity of carbon nanotubes. Phys Rev Lett 2000;84:4613–16
- Ebbesen TW, Lezec HJ, Hiura H, Bennett JW, Ghaemi HF, Thio T. Electrical conductivity of individual carbon nanotubes. Nature 1996;382:54–6
- Pagona G, Tagmatarchis N. Carbon nanotubes: Materials for medicinal chemistry and biotechnological applications. Curr Med Chem 2006;13:1789–98
- Wang W, Omori M, Watari F, Yokoyama A. Novel bulk carbon nanotube materials for implant by spark plasma sintering. Dent Mater J 2005;24:478–86
- Marrs B, Andrews R, Rantell T, Pienkowski D. Augmentation of acrylic bone cement with multiwall carbon nanotubes. J Biomed Mater Res A 2006;77:269–76
- Sun TP, Shieh HL, Ching CT, Yao YD, Huang SH, Liu CM, et al. Carbon nanotubes composites for glucose biosensor incorporated with reverse iontophoresis function for noninvasive glucose monitoring. Int J Nanomedicine 2010;5:343–9
- Fisher JW, Sarkar Sm Buchanan CF, et al. Photothermal response of human and murine cancer cells to multiwalled carbon nanotubes after laser irradiation. Cancer Res 2010;70:9855–64
- Zanello LP, Zhao B, Hu H, Haddon RC. Bone cell proliferation on carbon nanotubes. Nano Lett 2006;6:562–7
- Weissleder R, Kelly K, Sun EY, Shtatland T, Josephson L. Cell-specific targeting of nanoparticles by multivalent attachment of small molecules. Nat Biotechnol 2005;23:1418–23
- Kam NW, O’Connell M, Wisdom JA, Dai H. Carbon nanotubes as multifunctional biological transporters and near-infrared agents for selective cancer cell destruction. Proc Natl Acad Sci USA 2005;102:11600–5
- Ji SR, Liu C, Zhang B, Yang F, Xu J, Long J, Jin C, et al. Carbon nanotubes in cancer diagnosis and therapy. Biochim Biophys Acta 2010;1806:29–35
- Kawaguchi M, Fukushima T, Hayakawa T, Nakashima N, Inoue Y, Takeda S, et al. Preparation of carbon nanotube-alginate nanocomposite gel for tissue engineering. Dent Mater J 2006;25:719–25
- Shui C, Scutt A. Mild heat shock induces proliferation, alkaline phosphatase activity, and mineralization in human bone marrow stromal cells and Mg-63 cells in vitro. J Bone Miner Res 2001;16:731–41
- Morimoto RI. Regulation of the heat shock transcriptional response: Cross talk between a family of heat shock factors, molecular chaperones, and negative regulators. Genes Dev 1998;12:3788–96
- Sharp LA, Lee YW, Goldstein AS. Effect of low-frequency pulsatile flow on expression of osteoblastic genes by bone marrow stromal cells. Ann Biomed Eng 2009;37:445–53
- Yoshida K, Uoshima K, Oda K, Maeda T. Influence of heat stress to matrix on bone formation. Clin Oral Implants Res 2009;20:20782–90
- Lim CL, Byrne C, Lee JK. Human thermoregulation and measurement of body temperature in exercise and clinical settings. Ann Acad Med Singapore 2008;37:347–53
- Lindquist S, Craig EA. The heat-shock proteins. Annu Rev Genet 1988;22:631–77
- Fernandes M, O'Brein T, Lis JT. The Biology of Heat Shock Proteins and Molecular Chaperones. Chapter 15 (375--93): The Structure and Regulation of Heat Shock Gene Promoter
- Sarge KD, Murphy SP, Morimoto RI. Activation of heat shock gene transcription by heat shock factor 1 involves oligomerization, acquisition of DNA-binding activity, and nuclear localization and can occur in the absence of stress. Mol Cell Biol 1993;13:1392–407
- Sarge KD, Park-Sarge OK, Kirby JD, Mayo KE, Morimoto RI. Expression of heat shock factor 2 in mouse testis: Potential role as a regulator of heat-shock protein gene expression during spermatogenesis. Biol Reprod 1994;50:1334–43
- Pirkkala L, Nykänen P, Sistonen L. Roles of the heat shock transcription factors in regulation of the heat shock response and beyond. FASEB J 2001;15:1118–31
- Mezger V, Renard JP, Christians E, Morange M. Detection of heat shock element-binding activities by gel shift assay during mouse preimplantation development. Dev Biol 1994;165:627–38
- Eriksson M, Jokinen E, Sistonen L, Leppä S. Heat shock factor 2 is activated during mouse heart development. Int J Dev Biol 2000;44:471–7
- Ang D, Liberek K, Skowyra D, Zylicz M, Georgopoulos C. Biological role and regulation of the universally conserved heat shock proteins. J Biol Chem 1991;266:24233–6
- Udelsman R, Blake MJ, Stagg CA, Li DG, Putney DJ, Holbrook NJ. Vascular heat shock protein expression in response to stress. Endocrine and autonomic regulation of this age-dependent response. J Clin Invest 1993;91:465–73
- Tiffee JC, Griffin JP, Cooper LF. Immunolocalization of stress proteins and extracellular matrix proteins in the rat tibia. Tissue Cell 2000;32:141–7
- Shakoori AR, Oberdorf AM, Owen TA, Weber LA, Hickey E, Stein JL, et al. Expression of heat shock genes during differentiation of mammalian osteoblasts and promyelocytic leukemia cells. J Cell Biochem 1992;48:277–87
- Cooper LF, Uoshima K. Differential estrogenic regulation of small M(r) heat shock protein expression in osteoblasts. J Biol Chem 1994;269:7869–73
- Kozawa O, Niwa M, Matsuno H, Ishisaki A, Kato K, Uematsu T. Stimulatory effect of basic fibroblast growth factor on induction of heat shock protein 27 in osteoblasts: Role of protein kinase C. Arch Biochem Biophys 2001;388:237–42
- Hakeda Y, Hotta T, Kurihara N, Ikeda E, Maeda N, Yagyu Y, et al. Prostaglandin E1 and F2 alpha stimulate differentiation and proliferation, respectively, of clonal osteoblastic MC3T3-E1 cells by different second messengers in vitro. Endocrinology 1987;121:1966–74
- Kawamura H, Otsuka T, Matsuno H, Niwa M, Matsui N, Kato K, et al. Endothelin-1 stimulates heat shock protein 27 induction in osteoblasts: Involvement of p38 MAP kinase. Am J Physiol 1999;277:E1046–54
- Kozawa O, Niwa M, Matsuno H, Tokuda H, Miwa M, Ito H, et al. Sphingosine 1-phosphate induces heat shock protein 27 via p38 mitogen-activated protein kinase activation in osteoblasts. J Bone Miner Res 1999;14:1761–7
- Weissleder R, Kelly K, Sun EY, Shtatland T, Josephson L. Cell-specific targeting of nanoparticles by multivalent attachment of small molecules. Nat Biotechnol 2005;23:1418–23
- Huang H, Delikanli S, Zeng H, Ferkey DM, Pralle A. Remote control of ion channels and neurons through magnetic-field heating of nanoparticles. Nat Nanotechnol 2010;5:602–6
- van der Zee J. Heating the patient: A promising approach? Ann Oncol 2002;13:1173–84
- Jeyamohan P, Hasumura T, Nagaoka Y, Yoshida Y, Maekawa T, Kumar DS. Accelerated killing of cancer cells using a multifunctional single-walled carbon nanotube-based system for targeted drug delivery in combination with photothermal therapy. Int J Nanomedicine 2013;8:2653–67
- Leon SA, Asbell SO, Arastu HH, Edelstein G, Packel AJ, Sheehan S, et al. Effects of hyperthermia on bone. II. Heating of bone in vivo and stimulation of bone growth. Int J Hyperthermia 1993;9:77–87
- Doyle JR. Narrowing of the intervertebral-disc space in children. Presumably an infectious lesion of the disc. J Bone Joint Surg Am 1960;42:1191–200