Abstract
Background: Electrocautery (EC) is used during cytoreductive surgery (CRS) and hyperthermic intraperitoneal chemotherapy (HIPEC). Using a murine model, we studied the effect of HIPEC on small bowel EC lesions and surrounding normal tissues. Methods: Thirty-two rats were divided into five groups: a control group with EC lesions; EC plus intraperitoneal heated 5% dextrose (D5W); EC plus oxaliplatin (OXA, 460 mg/m2); EC plus mitomycin C 10 mg/m2 (MMC10); EC plus MMC 35 mg/m2 (MMC35). EC lesions and surrounding tissue microvasculature were analysed after intravenous injection of fluorescein. Results: In the ileum OXA significantly reduced EC lesions microvasculature compared with the control group; MMC10 caused greater reduction than the control, D5W and MMC35 groups. Surrounding tissue microvasculature was significantly reduced by MMC35 exposure when compared to the control, OXA or MMC10 groups. In the jejunum EC injuries exposed to OXA or MMC10 had significantly reduced microvasculature compared to the control, heated D5W and MMC35 groups. Surrounding tissue microvasculature was significantly reduced by MMC35 exposure when compared to the OXA group. There was no significant microvasculature difference between the EC lesions made before or after HIPEC. Conclusion: HIPEC with OXA and MMC10 potentiates small bowel wall EC injuries. MMC35 reduces surrounding unharmed tissue microvasculature. There was no effect of hyperthermia alone on microvasculature.
Introduction
Over the last two decades, cytoreductive surgery (CRS) combined with hyperthermic intraperitoneal chemotherapy (HIPEC) using mitomycin C (MMC) or oxaliplatin (OXA) has become the standard treatment for peritoneal carcinomatosis of colorectal origin. This approach has been associated with an increase in both disease-free and overall survival when compared to systemic chemotherapy and/or palliative surgery [Citation1–3]. Furthermore, some centres have begun using iterative treatments with CRS and HIPEC for peritoneal recurrences with promising results [Citation4].
However, CRS/HIPEC is associated with significant morbidity. The most commonly reported complications are severe gastrointestinal events, including bowel perforations and anastomotic leaks, which can occur in up to 19% of patients treated using this procedure [Citation5–10]. Multiple risk factors have been associated with gastrointestinal complications such as high histological grade, high peritoneal cancer index (PCI), small bowel resection, colorectal anastomosis, the number of anastomoses performed per patient, excessive blood loss and incomplete initial cytoreduction [Citation6,Citation9].
During CRS, electrocautery (EC) is often used on or near the bowel surface in order to destroy small tumour deposits. However, the effect of EC injuries to the bowel has never been studied in the context of CRS/HIPEC. Moreover, even though MMC and OXA pharmacokinetics have been studied, there is no data regarding the effect of these chemotherapy agents on intestinal microvasculature and whether HIPEC further worsens EC injuries on the intestinal wall [Citation7,Citation9,Citation11–13].
Therefore, the objective of this study was to evaluate the impact of EC injury on the small bowel microvasculature using fluorescence analysis in a murine model, in the context of a CRS/HIPEC procedure.
Materials and methods
Study design
Thirty-two male Sprague Dawley rats were used for the experiment, with a mean weight of 326 g (range 296–395 g) and a mean body surface area of 0.0431 m2 (range 0.0404–0.0490 m2). Only male rats were used for availability reasons and all animals were cared for according to the Canadian Council of Animal Care guidelines in the institution’s animal facility (Maisonneuve-Rosemont Hospital Animal Protection Committee approval no. 2011-37).
The rats were divided into five groups representing different experimental conditions. The number of rats per group is expressed in . For the control group (group A), a first set of cautery injuries was initially made on the small bowel (ileum and jejunum), the abdomen was left open and a second set was made after 35 min. The electrosurgical instrument was a monopolar device with a ball tip set on pure cut mode at high voltage (80 W). This was chosen in accordance with the technical description of tumour implant dissection by Sugarbaker [Citation14]. For groups with intraperitoneal perfusion we based our experimental set-up on the model developed by Piché et al. [Citation11]. Group B was exposed only to heated dextrose 5% solution (D5W) to isolate the effect of heat from the one caused by cytotoxic drugs. For group C, OXA was infused at a concentration of 460 mg/m2 as described by Elias et al. [Citation15,Citation16]. For groups D and E, MMC was infused at a dosage of 10 mg/m2 and 35 mg/m2 respectively as these values represent the most commonly used doses for MMC HIPEC [Citation3,Citation13,Citation17].
Table 1. Experimental groups and electrocautery lesions per site.
Surgical procedure
All procedures were performed under general anaesthesia using isoflurane inhalation. Each rat received pre-anaesthesia sedation to facilitate abdominal shaving and surgical set-up using ketamine (50 mg/kg), acepromazine (2.5 mg/kg), glycopyrrulate (0.02 mg/kg) and butorphanol (5 mg/kg).
The small bowel was exposed by performing a midline incision on the anterior abdominal wall. Using the ball tip on the monopolar electrocautery at 80 W in pure cut mode, 5-mm-long injuries were made on both ileal and jejunal surfaces on the anti-mesenteric side. One to three injuries were made on both jejunum and ileum before (groups A–E) and after the perfusion (groups B–E). Jejunum and ileum were studied separately because of their difference in thickness and vasculature. During the course of the study the number of EC lesions per rat increased in order to lower the number of animals to be sacrificed and reduce the chemotherapy to be used. The total number of EC lesions made before and after experimental exposure in each group is shown in .
In groups B–E, inflow and outflow intra-peritoneal catheters (5 mm diameter) were inserted after the first set of cautery lesions and the abdominal wall was closed in a watertight fashion (running locked silk suture). Both catheters were connected by a standard intravenous line to an injection port, creating a closed loop circuit containing 27 mL of D5W. The circuit was passed through a water tank heated at 45 °C at a rate of 27 cm3/min. Digital temperature probes were installed on the inflow and outflow catheters and perfusion temperature was maintained at 43 °C (±1 °C) during the experiment (groups B–E). OXA or MMC was subsequently injected at the appropriate dose in the circuit. HIPEC was conducted for a total duration of 25 min (groups B–E). The time difference between groups A (no HIPEC; 35 min) and B–E was applied to reflect a similar delay between the first and second set of EC lesions, thus allowing time for HIPEC set-up in the latter groups.
Next, the circuit and the abdominal cavity were washed with 30 cm3 of a D5W solution. The abdominal wall was reopened and a second set of EC lesions was made on the small bowel. Then, fluorescein (5 mg/kg) was injected into the inferior vena cava. An interval of 1 min was allowed for tissue distribution before euthanasia with intracardiac thiopental injection. The small bowel was harvested and the tissue samples were spread on specimen trays.
Photos of every EC lesion with normal surrounding tissues were taken using a Fuji camera, set on fluorescence mode (Cy3, Green – EpiRGB). Exposure time was 0.25 s. Fluorescence of both the EC lesions and normal tissues were calculated with AlphaEaseFc software (version 3.1.2; Alpha Innotech, San Leandro, CA, USA), using the spot density tool. demonstrates a jejunum tissue sample with an EC injury (central dark zone).
Statistical analysis
The fluorescence reduction (FR) was determined for every EC injury. FR was defined as the fluorescence of each electrocautery lesion (EL) related to the surrounding unharmed tissue (UT): FR = (UT – EL)/UT. A higher FR (i.e. closer to 1) signifies more tissue damage as fluorescence of severely damaged tissue is lower, and vice versa. Normality of distribution was confirmed using Kolmogorov–Smirnov test. Mean within-group differences in FR were analysed using Student’s t-test (unpaired) for lesions made before and after the experimental condition. The FR and the fluorescence of the surrounding unharmed tissues of the five groups were compared using one-way ANOVA followed by Tukey’s test for multiple comparisons. Statistical significance was defined as a p-value <0.05. All analyses were performed using Prism 5 (GraphPad software, La Jolla, CA).
Results
A total of 331 EC injuries (166 pre-experimental conditions, 165 post-experimental conditions) were induced in 32 rats (). There was no mortality. There was no significant difference in FR between the cautery lesions made before and after perfusion in any of the five groups. For the ANOVA analysis, only data from lesions made before the perfusion were used as it more closely represented the CRS/HIPEC procedure in the human context ().
Table 2. Fluorescence reduction and fluorescence of unharmed tissues.
Ileal EC injuries
The ANOVA showed a significant difference in FR for pre-HIPEC EC lesions in the ileum between the five groups (p < 0.0001). Post hoc tests showed that FR was significantly higher in the OXA (mean ± SD 0.44 ± 0.09, p < 0.01) and MMC 10 mg/m2 (0.50 ± 0.08, p < 0.001) groups compared to the control group (0.29 ± 0.09) (). The MMC 10 mg/m2 group also had a higher FR compared to the heated D5W (0.34 ± 0.07, p < 0.001) and MMC 35 mg/m2 groups (0.33 ± 0.19, p < 0.01) ().
Figure 2. Fluorescence reduction (FR) of ileum lesions. Significant difference with oxaliplatin (#). Significant difference with mitomycin 10 mg/m2 (*). D5%: Dextrose 5%; Oxali: Oxaliplatin; Mito 10: Mitomycin 10 mg/m2; Mito 35: Mitomycin 35 mg/m2.
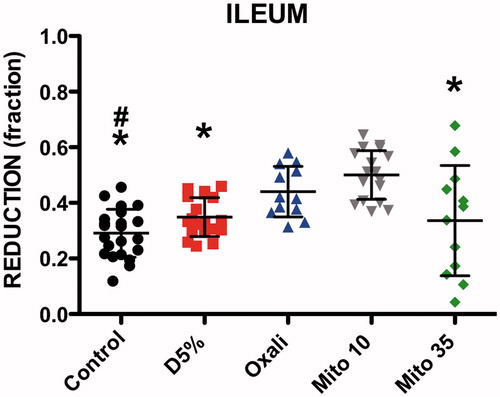
There were also significant differences between the five groups regarding the fluorescence of the surrounding unharmed tissues (ANOVA p = 0.0017). The post hoc comparisons between groups showed that the MMC 35 mg/m2 group (79.13 ± 15.80) had lower values than the control (94.08 ± 12.65, p < 0.01), OXA (95.40 ± 20.22, p < 0.01) and MMC 10 mg/m2 (91.08 ± 12.65, p < 0.05) groups ().
Jejunal EC injuries
The FR value for pre-HIPEC-induced EC lesions in the jejunum was significantly different between the five groups (ANOVA p < 0.0001). The FR value of EC lesions exposed to OXA (0.46 ± 0.09) was higher than those obtained in the control (0.29 ± 0.08, p < 0.001), heated D5W (0.26 ± 0.12, p < 0.001) and MMC 35 mg/m2 (0.29 ± 0.15, p < 0.01) groups. There was also a difference between the MMC 10 mg/m2 results (0.45 ± 0.08) and the same three groups (control p < 0.001, heated D5W p < 0.001 and MMC 35 mg/m2 p < 0.01) ().
Figure 4. Fluorescence reduction (FR) of jejunum lesions. Significant difference with oxaliplatin (#). Significant difference with mitomycin 10 mg/m2 (*). D5%: Dextrose 5%; Oxali: Oxaliplatin; Mito 10: Mitomycin 10 mg/m2; Mito 35: Mitomycin 35 mg/m2.
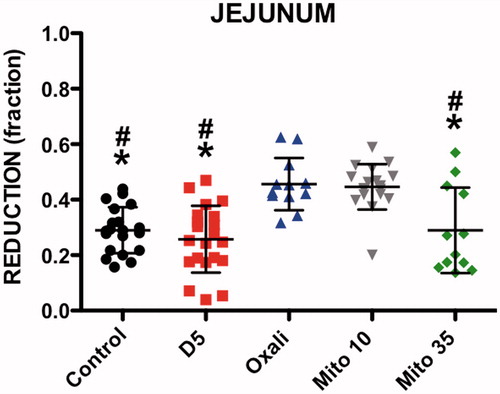
The MMC 35 mg/m2 group had significantly less fluorescence in the surrounding unharmed jejunal tissue (83.58 ± 14.22) than OXA (102.80 ± 19.08, p < 0.001) ().
Discussion
During CRS, peritoneal implants on the small bowel surface can be destroyed using electrocautery and thermal energy. This technique reduces the need for intestinal resection but also increases the risk of gastrointestinal complications, especially anastomotic leak and intestinal perforations [Citation8,Citation18]. Furthermore, HIPEC agents have been associated with a harmful effect on tissue healing. Other animal studies using rats have shown that intraperitoneal MMC and cisplatin impair anastomotic healing [Citation19–22]. Lower hydroxyproline content and smaller collagen fibre diameter at the anastomosis were observed after MMC and OXA use [Citation20–22]. Our study evaluates the impact of intraperitoneal chemotherapy on microvasculature associated with thermal damage of EC lesions on the small bowel.
In our study, EC lesions were made before and after HIPEC to evaluate the effect of the technical sequence on the amount of microvasculature damage. In the operating room, CRS is usually performed before HIPEC. However, remaining intestinal implants can also be cauterised after the perfusion. The statistical analysis of our data has not shown any significant difference between the FR of EC lesions made before and after HIPEC.
The method used to measure this effect was perfusion fluorometry using intravenous sodium fluorescein. While there is still a debate about the accuracy of this technique [Citation23], it has been tested in a previous study at the authors’ institution. It was found to be reliable and reproducible when testing small bowel vascularisation after various EC injuries (Tremblay et al., unpublished data). To summarise the results, there was a decrease in bowel wall micro-vascularisation with increasing duration and intensity of injuries.
The decision to perform EC lesions on the jejunum and on the ileum was made in light of the anatomical differences in the small bowel wall thickness and terminal vascularisation [Citation24] The results of the present study demonstrate that OXA and MMC 10 mg/m2 increased the FR on both the ileum and jejunum, compared to the control group. No EC lesions were made on the colon wall as implants on the colon are often resected. Compared to the small intestine, there is less risk of short-gut syndrome if the colon is resected extensively and minimal effort is usually made to preserve colonic length [Citation25].
OXA and MMC 10 mg/m2 significantly diminished the vascularisation of EC lesions on jejunum (fluorescence reduction) when compared to the control, heated D5W solution or MMC 35 mg/m2 groups. For the ileal EC lesions, this significant difference with OXA was limited to the control group, whereas mean FR of MMC 10 mg/m2 was significantly greater than the three other groups (control, D5W, MMC 35 mg/m2). This difference between MMC 10 mg/m2 and OXA could result from different effects on vascularisation, wall thickness or from insufficient power.
It was surprising that MMC 35 mg/m2 did not have a deleterious effect on the microvasculature of EC lesions with HIPEC as for the MMC 10 mg/m2 results. One potential explanation for this finding can be obtained from the fluorescence of unharmed tissues: ileum exposed to MMC at 35 mg/m2 had significantly less fluorescence intensity than control, D5W and OXA groups. This could have lowered the calculated fluorescence reduction. At 35 mg/m2 dosage, MMC appears to lower the overall fluorescence of unharmed tissues, and thereby its vascularisation. This effect is more pronounced on the ileum where a significant difference was observed with the control, OXA and MMC 10 mg/m2 groups. In the jejunum, this difference was only observed between the OXA and the MMC 35 mg/m2 groups. These data suggest that the ileum could be more susceptible to MMC than the jejunum. This may also partially explain previous studies results showing negative effect of MMC and OXA on anastomotic healing [Citation19–22].
Hyperthermia alone (heated D5W solution) did not show a significant difference in FR or unharmed tissue fluorescence compared to the control group. To our knowledge, no study has examined the impact of hyperthermia on bowel vascularisation. A study on intestinal anastomosis breaking strength in rats showed no difference for hyperthermia up to 44 °C [Citation26]. Another study showed no difference in anastomotic healing after preoperative exposure to hyperthermia or radiation alone; however, the combination of both was detrimental [Citation27]. The results of the current study seem to agree with the available literature, as harmful effects on tissue microvasculature after EC lesions were only seen after certain HIPEC agents. The mechanism by which OXA and MMC could affect the microvasculature of unharmed tissue and EC injuries is unknown. We hypothesise that there could be an effect of the cytotoxic drugs on the regulation of blood flow, whether by causing tissue oedema or vasoconstriction.
In the future it would be interesting to compare the effects of monopolar electrocautery and argon beam coagulation on intestinal microvasculature. The latter is known for lower energy penetration and thermal diffusion. However, in our institution, the argon device is not used on intestinal surfaces.
A limitation of our study is the number of rats per group, which could result in a lack of power or bias. Causing and harvesting many EC lesions per bowel site was used to overcome this issue. Another limitation of our study is its experimental nature, and whether the results can be translated from rats to human beings remains to be proven. Also, the relationship between delayed intestinal perforation or anastomotic leaks and the immediate thermal injury remains unknown. More studies are required to further investigate this area and potentially find solutions to lower CRS/HIPEC morbidity.
Conclusion
HIPEC with OXA and MMC at 10 mg/m2 amplifies the effect of EC lesions on small bowel microvasculature. MMC at 35 mg/m2 dose does not seem to convey a similar result but does reduce the fluorescence in the surrounding unharmed tissues. There was no effect of hyperthermia alone on small bowel microvasculature. These data suggest that EC on bowel wall must be used with caution during CRS/HIPEC.
Acknowledgements
J.S.T., L.S. and P.Du. took part in the overall design of the study. J.S.T. wrote the first draft of the manuscript and conducted the experiments. J.F.T. participated in the experimental set-up. P.Dr. conducted the statistical analyses. L.L., J.F.T., L.S. and P.Du. critically revised the manuscript. All authors have read and approved the final version of the manuscript.
Disclosure statement
The authors report no conflicts of interest. The authors alone are responsible for the content and writing of the paper.
References
- Verwaal VJ, van Ruth S, de Bree E, van Sloothen GW, van Tinteren H, Boot H, et al. Randomized trial of cytoreduction and hyperthermic intraperitoneal chemotherapy versus systemic chemotherapy and palliative surgery in patients with peritoneal carcinomatosis of colorectal cancer. J Clin Oncol 2003;21:3737–43.
- Glehen O, Kwiatkowski F, Sugarbaker PH, Elias D, Levine EA, De Simone M, et al. Cytoreductive surgery combined with perioperative intraperitoneal chemotherapy for the management of peritoneal carcinomatosis from colorectal cancer: A multi-institutional study. J Clin Oncol 2004;22:3284–92.
- Esquivel J, Sticca R, Sugarbaker P, Levine E, Yan TD, Alexander R, et al. Cytoreductive surgery and hyperthermic intraperitoneal chemotherapy in the management of peritoneal surface malignancies of colonic origin: A consensus statement. Ann Surg Oncol 2006;14:128–33.
- Vaira M, Robella M, Mellano A, Sottile A, De Simone M. Iterative procedures combining cytoreductive surgery and hyperthermic intraperitoneal chemotherapy for isolated peritoneal recurrence. Int J Hyperthermia 2014;30:565–9.
- Smeenk RM, Verwaal VJ, Zoetmulder FAN. Learning curve of combined modality treatment in peritoneal surface disease. Br J Surg 2007;94:1408–14.
- Casado-Adam A, Alderman R, Stuart OA, Chang D, Sugarbaker PH. Gastrointestinal complications in 147 consecutive patients with peritoneal surface malignancy treated by cytoreductive surgery and perioperative intraperitoneal chemotherapy. Int J Surg Oncol 2011;2011:1–10.
- Kusamura S, Younan R, Baratti D, Costanzo P, Favaro M, Gavazzi C, et al. Cytoreductive surgery followed by intraperitoneal hyperthermic perfusion: Analysis of morbidity and mortality in 209 peritoneal surface malignancies treated with closed abdomen technique. Cancer 2006;106:1144–53.
- Hansson J, Graf W, Påhlman L, Nygren P, Mahteme H. Postoperative adverse events and long-term survival after cytoreductive surgery and intraperitoneal chemotherapy. Eur J of Surg Oncol 2009;35:202–8.
- Younan R, Kusamura S, Baratti D, Oliva G, Favaro M, Gavazzi C, et al. Bowel complications in 203 cases of peritoneal surface malignancies treated with peritonectomy and closed-technique intraperitoneal hyperthermic perfusion. Ann Surg Oncol 2005;12:910–18.
- Youssef H, Newman C, Chandrakumaran K, Mohamed F, Cecil TD, Moran BJ. Operative findings, early complications, and long-term survival in 456 patients with pseudomyxoma peritonei syndrome of appendiceal rigin. Dis Colon Rectum 2011;54:293–9.
- Piché N, Leblond FA, Sidéris L, Pichette V, Drolet P, Fortier L-P, et al. Rationale for heating oxaliplatin for the intraperitoneal treatment of peritoneal carcinomatosis. Ann Surg 2011;254:138–44.
- Ceelen WP, Peeters M, Houtmeyers P, Breusegem C, Somer F, Pattyn P. Safety and efficacy of hyperthermic intraperitoneal chemoperfusion with high-dose oxaliplatin in patients with peritoneal carcinomatosis. Ann Surg Oncol 2007;15:535–41.
- van Ruth S, Mathôt RA, Sparidans RW, Beijnen JH, Verwaal VJ, Zoetmulder FA. Population pharmacokinetics and pharmacodynamics of mitomycin during intraoperative hyperthermic intraperitoneal chemotherapy. Clin Pharmacokinet 2004;43:131–43.
- Sugarbaker PH. Dissection by electrocautery with a ball tip. J Surg Oncol 1994;56:246–8.
- Elias D, Bonnay M, Puizillou JM, Antoun S, Demirdjian S, Otmany El A, et al. Heated intra-operative intraperitoneal oxaliplatin after complete resection of peritoneal carcinomatosis: Pharmacokinetics and tissue distribution. Ann Oncol 2002;13:267–72.
- Elias DM, Sidéris L. Pharmacokinetics of heated intraoperative intraperitoneal oxaliplatin after complete resection of peritoneal carcinomatosis. Surg Oncol Clin N Am 2003;12:755–69.
- Sugarbaker P, Chang D. Results of treatment of 385 patients with peritoneal surface spread of appendiceal malignancy. Ann Surg Oncol 1999; 6:727–31.
- Glockzin G, Schlitt HJ, Piso P. Peritoneal carcinomatosis: Patients selection, perioperative complications and quality of life related to cytoreductive surgery and hyperthermic intraperitoneal chemotherapy. World J Surg Oncol 2009;7:1–8.
- Makrin V, Lev-Chelouche D, Even Sapir E, Paran H, Rabau M, Gutman M. Intraperitoneal heated chemotherapy affects healing of experimental colonic anastomosis: an animal study. J Surg Oncol 2005;89:18–22.
- Fumagalli U, Trabucchi E, Soligo M, Rosati R, Rebuffat C, Tonelli C, et al. Effects of intraperitoneal chemotherapy on anastomotic healing in the rat. J Surg Res 1991;50:82–7.
- Pelz JOW, Doerfer J, Decker M, Dimmler A, Hohenberger W, Meyer T. Hyperthermic intraperitoneal chemoperfusion (HIPEC) decrease wound strength of colonic anastomosis in a rat model. Int J Colorectal Dis 2007;22:941–47.
- Kanellos D, Pramateftakis MG, Mantzoros I, Zacharakis E, Raptis D, Despoudi K, et al. The effects of the intraperitoneal administration of oxaliplatin and 5-FU on the healing of colonic anastomoses: An experimental study. Tech Coloproctol 2011;15:111–15.
- Urbanavičius L. How to assess intestinal viability during surgery: a review of techniques. World J Gastrointest Surg 2011;3:59–69.
- Abbott AM, Armstrong L, Jensen EH. Small intestine. In: Yeo CJ, McFadden DW, Pemberton JH, Peters JH, eds. Shackelford’s surgery of the alimentary tract. 7th ed. Vol. 1. Philadephia: Elsevier Saunders, 2013, pp. 839–63.
- Sugarbaker PH. Intraperitoneal chemotherapy and cytoreductive surgery for the prevention and treatment of peritoneal carcinomatosis and sarcomatosis. Semin Surg Oncol 1998;14:254–61.
- Shimizu T, Maeta M, Koga S. Influence of local hyperthermia on the healing of small intestinal anastomoses in the rat. Br J Surg 1991;78:57–9.
- Biert J. Combined preoperative irradiation and local hyperthermia delays early healing of experimental colonic anastomoses. Arch Surg 1996;131:1037–42.