Abstract
Non-muscle-invasive bladder cancer is a challenging disease, even given its superficial nature. It is prone to multiple recurrences and progression to muscle-invasive cancer. These features of this disease contribute significantly to reduced quality of life as well as creating significant morbidity and even mortality. Randomised trials demonstrate that when hyperthermia is added to conventional mitomycin-C treatment that local control rates and progression-free survival are substantially improved. In this review we consider how hyperthermia can exert such beneficial effects. Some of the mechanisms presented are theoretical, while others are facts. It is hoped that this review will contribute rationale for further examination of mechanisms, because an understanding of such mechanisms may lead to even better chemotherapeutic approaches, as well as potential biomarkers for predicting and monitoring treatment success.
Introduction
A series of previous studies in bladder cancer have shown that the non-muscle-invasive bladder cancer (NMIBC) recurrence rate is substantially reduced following radiofrequency-induced hyperthermia plus mitomycin-C (MMC), compared with ‘conventional’ intravesical MMC instillation [Citation1]. Furthermore, the progression rate is low, even in patients presenting with carcinoma in situ (CIS), grade 3 (G3) tumours and patients failing BCG prior to radiofrequency-induced chemo-hyperthermia (RF-CH) treatment [Citation2]. There are multiple potential reasons for the enhanced effects of this combination therapy. In this review we consider effects of hyperthermia on drug transport and on cell-killing efficiency. We believe that both play an important role in the success of this therapeutic combination.
Drug transport barriers
There are multiple barriers to effective delivery of drugs to cancers at cytotoxic concentrations. First, there are substantial barriers to deliver adequate concentrations of drug throughout the tumour volume. Second, cells have delivery barriers that must be circumvented. Drug has to be taken up by the cell in sufficient quantity to hit the intracellular target in order to be cytotoxic. Mechanisms of cellular drug resistance have been identified by many investigators and will be discussed.
Barriers to transport of drugs from intravesical administration
The challenges of drug delivery following intravenous administration have been discussed in excellent reviews [Citation3,Citation4]; but the basic concepts have not been discussed previously with reference to treatment of NMIBC, where drugs are typically administered intravesically. In this review we examine those features of drug transport from intravesical administration that are influenced by hyperthermia. We will focus mainly on discussion of MMC because it is a standard of care. However, the principles discussed are appropriate to any small molecule drug. In the discussion we will briefly examine issues related to intravenous drug administration, which is not currently used for NMIBC. Intravenous drug could be used if a method could be developed that could reduce systemic toxicity while maintaining cytotoxic drug levels in the bladder. We discuss thermosensitive liposomes as a platform through which this goal could theoretically be achieved.
Sites of growth of NMIBC and rationale for standards of care
NMIBC is located in the mucosa and can extend into the submucosa, but has not penetrated into the muscle layer. There is low probability for regional or distant metastasis with this presentation; therefore, it is considered a localised disease. Intravesical chemotherapy is used for NMIBC, since the highest concentration of drug will be delivered directly to the site of tumour growth. In addition, one would not want to subject a patient who has local disease to the toxicities associated with systemically administered drug. However, there are substantial hurdles to achieving uniform cytotoxic drug delivery with NMIBC. Here we discuss the barriers and then how hyperthermia can improve drug delivery.
The standard of care for newly diagnosed NMIBC is transurethral resection of the tumour, followed by intravesical chemotherapy in low or intermediate risk, and immunotherapy with bacillus Calmette-Guérin (BCG) in intermediate- or high-risk NMIBC patients [Citation5]. Although these therapies are initially effective, the 5-year recurrence rate is up to 80%, depending on the stage and grade of the tumour. The consequences of these recurrences are twofold. First, recurrences may require many resections. Eventually, this will lead to inability of the bladder to retain acceptable volumes of urine because the elasticity becomes compromised. This may require complete removal of the bladder, known as a cystectomy, because of functional problems. Cystectomy is associated with substantial morbidity. Even in experienced centres the 30 and 90 days perioperative mortality rate is respectively around 2 and 7% [Citation6]. Second, approximately 15% of patients with high grade NMIBC will progress to muscle-invasive cancer, which also has a much worse prognosis compared to de novo muscle-invasive disease [Citation7,Citation8]. Thus, there is strong rationale to develop therapies that can lower recurrences and prevent progression to muscle-invasive disease.
One might assume that treatment efficacy would be maximised by using intravesical chemotherapy for NMIBC, since the drug will be in high concentration immediately adjacent to the mucosal surface. However, there are impediments to efficient drug delivery by intravesical means.
Glycosaminoglycans present a barrier to drug penetration
The inner lining of the bladder is covered by a thick layer of glycosaminoglycan (GAG), which is an effective barrier that limits passage of drugs to the tumour bladder cell surface [Citation9,Citation10]. In order to increase drug penetration across the GAG layer, one must either remove the GAG or enhance drug transport across it. A number of methods have been used to temporarily disrupt the GAG layer, as a means to enhance drug delivery. Examples include instillation of mild detergents and dimethylsufoxide (DMSO) [Citation9]. Additionally, drugs that inhibit GAG synthesis have been used to reduce the thickness of the layer [Citation11]. Alternatively, glycosaminoglycan drug carriers have been shown to be taken up into GAG layers, increasing penetration of drug into tumour interstitium [Citation12,Citation13]. GAG has also been considered a barrier to effective therapy with the immunotherapeutic drug BCG [Citation14]. Although these methods have resulted in improvements in antitumour effects of drugs in pre-clinical models, none have been approved for use in conjunction with intravesical chemotherapy.
Urothelium is the next layer of defence against drug penetration
The urothelium is composed of three types of cells: basal, intermediate and umbrella cells (). The umbrella cells are very specialised to add another tight barrier to prevent urine and bacteria from passing from the bladder into adjacent tissues [Citation15]. Tight protein-based junctions form between adjacent cells, which contribute to the impenetrability of this layer [Citation16]. The lipid composition is comprised of cholesterol, phosphatidyl choline, phosphatidyl ethanolamine, and cerebroside. This composition makes the layer very water impermeable. To be able to deliver drugs to NMIBC, one must penetrate this impermeable layer.
Figure 1. Structure of bladder wall. This wall is very impermeable to water. The umbrella cells contain a high percentage of highly hydrophobic lipids in addition to having very tight junctions between cells. Drugs must traverse through the glycosaminoglycan layer, three layers of cells, the basal lamina and underlying stroma to reach to the depth of tumour cells. NMIBC cells do not penetrate into the first muscle layer. It has been reported that hyperthermia can damage this mucosal layer, thereby increasing drug penetrability.
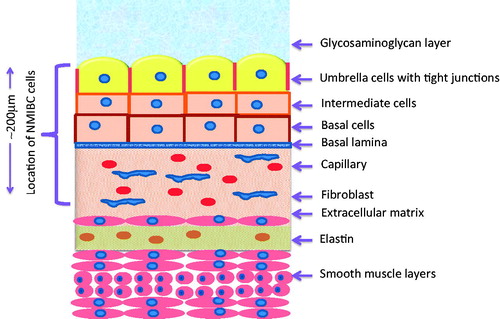
Drug must penetrate through the mucosal surface to reach all tumour cells
MMC penetration across the bladder wall has been measured following intravesical chemotherapy in human patients [Citation17]. Concentrations dropped from 120 μg/mL in the urine, to 5.6 μg/mL in the urothelium and 0.9 μg/mL in the muscle layer. Thus, there is a 20-fold drop in drug concentration just to the urothelium and a 100-fold drop to the muscularis. The distance over which the drug concentration in the bladder wall drops by 50% is 500 μm. The unfolded mucosal cell layer is 200 μm thick [Citation17]. However, if the bladder is partially collapsed the drug may have to penetrate through several folded mucosal layers to reach all cells, as described next.
Folded mucosal layer and penetration depth
In the constricted state, the bladder wall is folded on itself, much like a collapsed hot air balloon () [Citation15]. When the bladder is fully stretched, these folds disappear. At this point, the depth that drug must diffuse to be effective is minimal. When drug is instilled into the bladder, the volume is much smaller than the bladder capacity. This is done to allow for the bladder to fill during drug administration. A typical treatment lasts 1–2 h, during which time, the patient will produce urine. Whether or not the bladder reaches full capacity is unknown, but it is safe to say that for much of the time that drug is present, the folded mucosal layers present a barrier to effective diffusion.
Figure 2. Folded mucosal surface of unfilled rabbit bladder. In this state there are multiple mucosal layers folded on top of each other. The multiple layers will restrict access of drug to all tumour cell locations. Intravesical therapy involves instilling 40 mL of MMC-containing solution. This volume does not stretch the bladder to its full capacity. Over time, the bladder will fill, which will stretch the bladder, reducing the size and number of folds. It has been reported that intravesical hyperthermia increases urine production. In a clinical trial involving intravesical MMC and radiofrequency hyperthermia, 82–95 mL of urine was recovered from unheated control patients versus 130 mL was recovered from patients who received the hyperthermia treatment [Citation19]. There is a trade-off regarding the value of bladder filling. On the one hand, stretching of the wall will reduce the folded layers, making the mucosal surface more exposed to drug. On the other hand, the filling dilutes the drug.
![Figure 2. Folded mucosal surface of unfilled rabbit bladder. In this state there are multiple mucosal layers folded on top of each other. The multiple layers will restrict access of drug to all tumour cell locations. Intravesical therapy involves instilling 40 mL of MMC-containing solution. This volume does not stretch the bladder to its full capacity. Over time, the bladder will fill, which will stretch the bladder, reducing the size and number of folds. It has been reported that intravesical hyperthermia increases urine production. In a clinical trial involving intravesical MMC and radiofrequency hyperthermia, 82–95 mL of urine was recovered from unheated control patients versus 130 mL was recovered from patients who received the hyperthermia treatment [Citation19]. There is a trade-off regarding the value of bladder filling. On the one hand, stretching of the wall will reduce the folded layers, making the mucosal surface more exposed to drug. On the other hand, the filling dilutes the drug.](/cms/asset/2be427d4-368d-4777-bfc9-e78b704bc1f5/ihyt_a_1155761_f0002_b.jpg)
Positive effects of hyperthermia on drug transport
Hyperthermia may exert beneficial effects related to the delivery limitations listed above. Some of these mechanisms will work directly on tumour cells as well as uroepithelial cells. It will become apparent that hyperthermia exerts beneficial effects to enhance drug transport across multiple barriers that emanate from intravesical therapy.
Effects on GAG and urothelial layers
Hyperthermia has been shown to transiently damage the urothelium, which can increase the rate of drug uptake into the bladder wall [Citation18]. This results in increased drug absorption when radiofrequency-induced hyperthermia is applied [Citation19]. This increase is even more pronounced in patients with unresected tumours.
Potential mechanisms for enhancement of drug transport across urothelium
Cytoskeleton
Hyperthermia has been reported to cause transient changes in the cytoskeleton of cells; this is largely attributed to the disassembly microtubules, microfilaments and actin fibres [Citation20]. The cytoskeleton is known to be involved in maintenance of cell shape. If it collapses, this may weaken the tight junctions between the uroepithelial layers, thereby increasing permeability. We reported previously that hyperthermia increases microvascular permeability, opening up pores between endothelial cells of 100–400 microns in size [Citation21]. Similar effects may occur with the urothelium. It is important to know that these cytoskeletal changes are transient and recover as cells induce the production of heat shock proteins in response to thermal stress [Citation20].
Hyperthermia Enhances drug diffusion
The delivery of a small molecule through a tissue layer is dependent upon the diffusion coefficient. Diffusion coefficients are temperature dependent. According to the Stokes-Einstein equation, the diffusion coefficient increases by 14% when temperature increases from 37 to 43 °C, with this rise in temperature the hydraulic conductivity is also increased by 12% [Citation22]. The increase in hydraulic conductivity is the result of enhanced permeability in the interstitium and reduced fluid viscosity. Hyperthermia (42 °C) has been shown to decrease urine viscosity by approximately 10%, as compared with 37 °C [Citation23]. This combination of effects could contribute to increased drug penetration into the bladder wall.
Hyperthermia affects tissue structure
The effects of hyperthermia on cell density and shape may also contribute to enhanced drug transport. It has been reported that increased drug uptake into tumours can occur if efforts are made to reduce tumour cell density [Citation3]. There are several reports indicating that hyperthermia can increase efficacy of a variety of chemotherapeutic agents. Apoptosis is a common mode of death following such treatments [Citation24]. Ware et al. observed a decrease of 15% in cell volume 24 hours after radiofrequency (RF) treatment [Citation25]. They concluded that decreases in individual cell area do play an important role in alteration of cell–cell junctions. There was also a loss of cell adhesion. Furthermore, radiofrequency alters the surface roughness of malignant cells. This change will influence the interactions between chemotherapy and the cell membrane. Further, hyperthermia increased tunnelling nanotube formation, which results in more cell-to-cell interactions. Tunnelling nanotube formation has been shown to enhance particle trafficking in cancer cells. These observations by Ware were performed in circumstances where temperature was controlled to <39 °C. It is unclear whether similar effects would be observed if therapeutic temperatures were induced with RF. Induction of a wave of apoptosis after thermochemotherapy could reduce tumour cell packing density, thereby enabling more effective administration of a second drug [Citation3]. To our knowledge, this therapeutic strategy has not been attempted for treatment of NMIBC. Similarly, use of agents that can modify the density of the extracellular matrix can also improve drug penetration [Citation3]. To our knowledge, however, there have not been any reports indicating whether hyperthermia changes extracellular matrix to improve drug transport.
Potential negative effects of hyperthermia on drug transport
Tissue oedema
Transient oedema was observed in sheep bladder tissues after hyperthermia [Citation18]. The diffusion distance of a small molecule is proportional to L2/D, where L is the distance and D is the diffusion coefficient. Bladder oedema could increase the thickness of the bladder wall, which could reduce drug delivery by increasing the distance over which drug must diffuse to reach target tumour cells. Oedema would also reduce the local drug concentration, which could reduce the drug concentration gradient. These two effects could reduce drug transport across the bladder wall. On the other hand, the diffusion of drug can increase because tissue density is decreased in the presence of oedema. This would tend to increase the diffusion distance. It is difficult to predict the net effect of hyperthermia on drug diffusion, given these offsetting forces.
Urine production during heating dilutes drug concentration in bladder
Dilution of drug by urine can reduce the exposure of the bladder wall to drug. In a human clinical trial, urine concentrations of MMC were measured during and after treatment. Patients were treated with and without intravesical hyperthermia with a microwave antenna. Patients who received hyperthermia produced more urine, thereby resulting in more dilution of drug than the control patients who were not heated. For example, at an intravesical dose of 40 mg the urine concentration dropped by fourfold in the heated group, compared with 2.2-fold in the control group [Citation19].
Increased perfusion may remove drug from target volume
Even though NMIBC is superficial, these tumours can be vascularised [Citation26]. There is additional vasculature in the muscle layer, deep in relation to the position of the tumour. Hyperthermia at 43 °C for up to 2 h increases skeletal muscle perfusion of rats by 3.5- to sixfold [Citation27]. Similar effects were observed in canine thigh muscle in response to thermal stress [Citation28]. The tissue response to thermal stress follows a biphasic curve, where perfusion increases by approximately twofold between 37 and 42 °C. Above 42 °C, perfusion increases by approximately a factor of 3 for every 1 °C rise in temperature [Citation28]. Multiple mechanisms have been postulated to be involved in the regulation of perfusion upon thermal stress. These include enhanced release of vasoactive substances, such as bradykinin and prostacyclin. In addition, enhanced NO production by vascular endothelium could contribute [Citation28]. The muscularis of the bladder is composed of smooth muscle, so one has to consider whether there is precedent for hyperthermia having a direct effect on perfusion of this organ. Activation of TRP (transient receptor potential) channels may be involved in regulating smooth muscle vasodilation upon heat stress [Citation29,Citation30]. Perfusion in tumours can increase as well by as much as 2-fold [Citation27]. The increase in perfusion in both tumour and muscle of the bladder wall could enhance drug removal from the tumour, where it could be redistributed systemically. Paroni et al. reported that systemic levels of MMC were increased following intravesical administration in patients who received local heating of the bladder [Citation19]. On the one hand, this observation is consistent with the concept that systemic removal of drug is increased with local hyperthermia delivered to the bladder. On the other hand, the fact that there is an increase in systemic levels of drug means that the drug was penetrating deep enough to be picked up by the blood vessels. Presumably, this is indirect evidence for greater tumour cell exposure to drug as well, since the drug would have to pass through multiple cell layers to reach the deeper vasculature. The systemic levels of drug achieved with hyperthermia were below those that are known to cause normal tissue toxicity.
Theoretically, exposure of tumour cells to drug could be increased if hyperthermia causes vascular damage in tumours. Such damage would reduce systemic uptake of drug. In early studies there was the suggestion that the vasculature of tumours is more thermally sensitive than that of normal tissue, with thresholds of thermal damage in the range of 42–43 °C for 1 h exposure [Citation27]. Most of those data were based on observations in rodents. More recent studies have indicated that the vasculature of human/canine tumours is more resilient and that vascular shut down does not occur below temperatures of 44 °C for 1 h. For example, in companion canine soft tissue sarcomas, intratumoural temperatures in the range of 40–43 °C resulted in increases in tumour perfusion and oxygenation at 24 h post-hyperthermia treatment [Citation31]. In contrast, temperatures >44 °C resulted in reduced perfusion and oxygenation, most likely as a result of vascular damage. Similar results were observed in women with locally advanced breast cancer who were treated with a combination of hyperthermia, radiotherapy and chemotherapy. Further, reoxygenation was associated with better clinical response in the locally advanced breast cancer study [Citation32]. The prescribed temperature range for bladder heating is in the range of 42–43 °C [Citation33,Citation34]. Thus it is unlikely that vascular damage to the tumour or normal tissue vasculature is a consequence. If vascular damage did occur, the consequence could easily result in less drug uptake by the tumour. This could occur because such damage would not maintain the concentration gradient of drug across the tumour – should this occur, Fickian diffusion could be reduced.
Hyperthermia increases drug uptake by, and cytotoxicity of, tumour cells
Hyperthermia has been reported to increase cellular uptake of some drugs; this increase has been considered one of the factors that leads to enhanced antitumour effects. Cisplatin, MMC, gemcitabine, doxorubicin and epirubicin, are all used in intravesicle treatment of bladder cancer. We will discuss the effects of hyperthermia on cellular transport of these drugs in more detail.
The permeability of lipid bilayers is affected by the order of acyl chains of phospholipids. Increasing temperature reduces the order of the acyl chains, thereby increasing permeability. Increases in membrane fluidity have been associated with increases in drug uptake by cells, including doxorubicin [Citation35], and cisplatin [Citation36]. However, the effects of hyperthermia on cell membrane fluidity are not the entire explanation for enhanced uptake for all drugs.
Hyperthermia inhibits DNA damage repair. The most important lethal target is the double strand break, which is repaired by two mechanisms: non-homologous end joining and homologous recombination. Both of these mechanisms of repair are inhibited by hyperthermia [Citation37]. Important thermal targets include DNA polymerases and poly(ADP-ribose)polymerase-1 (PARP) [Citation38,Citation39]. PARP functions to direct DNA repair enzymes to the location of strand breaks. Thermal inhibition of BRCA2, an enzyme responsible for initiation of DNA repair, combined with inhibition of PARP is synthetically lethal to target cells. Hyperthermia has also been reported to inhibit recombination repair of stalled DNA replication forks, which are the primary target lesion of gemcitabine [Citation40].
Cisplatin
Alberts was one of the first investigators to report that hyperthermia (42 °C) increased cytotoxicity of cisplatin, in vivo, using a mouse leukaemia line [Citation41]. Using radiolabelled cisplatin, he demonstrated that hyperthermia increased cellular uptake of cisplatin. Meyn et al. reported that hyperthermia (43 °C) of CHO cells increased the extent of lethal DNA crosslinks created by cisplatin [Citation42]. The dose modifying factor, which is the ratio of drug doses to achieve an equal level of cytotoxicity at 37 °C versus 43 °C, was nearly a factor of 6. They hypothesised that the increase in crosslinks could be a result of inhibition of DNA damage repair, but the rate of disappearance of crosslinks was the same for both temperatures. They concluded that the increase in cytotoxicity of cisplatin by hyperthermia was most likely caused by an increase in drug uptake. Los et al., demonstrated that regional hyperthermia (41 °C) increased drug uptake by over fourfold, increased DNA crosslinking by 2.5-fold and increased in vivo efficacy of cisplatin, using a rat colon carcinoma line [Citation43]. Los also reported that the threshold for enhancement of cisplatin uptake by hyperthermia was 38.5 °C [Citation44]. Using isolated DNA, they were able to show that the rate of cisplatin binding to DNA was not temperature dependent. Thus, it is reasonable to conclude that the enhancement in cytotoxicity caused by hyperthermia is a result of increased cellular uptake, leading to an increase in DNA crosslinks. It has been recently reported that the mechanism of enhanced cisplatin uptake is related to the function of the copper transporter, CTR1. Hyperthermia increases the extent of CTR1 multimerisation, which creates a membrane pore to facilitate cisplatin uptake [Citation45]. Wallner and Li demonstrated that the greatest effectiveness of cisplatin with hyperthermia occurs when the two are given simultaneously, with a hyperthermia treatment period of 1 h [Citation46]. Longer heating times were less effective. This observation fits well with the expected use of cisplatin with hyperthermia, since the drug would be instilled into the bladder and hyperthermia could commence quickly after instillation.
Mitomycin C
There are several references related to the combination of hyperthermia with MMC, dating back to the early 1980s [Citation47–52]. Wallner et al. examined the effects of hyperthermia on cytotoxicity of MMC in drug-sensitive and drug-resistant Chinese hamster ovary (CHO) cell lines [Citation53]. Dose-modifying factors for 42° and 43 °C heating with MMC ranged from 1.3–2.0 and 2.6–3.8, respectively. The magnitude of the dose-modifying factor was independent of whether the cells were drug sensitive or drug resistant. Hyperthermia (43.5 °C) increased cellular uptake of MMC by 78% versus 27% for drug-sensitive versus drug-resistant cell lines. Like cisplatin, simultaneous administration of drug and hyperthermia was the most efficacious.
Teicher et al. examined the effects of hyperthermia (41, 42 and 43 °C) on cytotoxicity of MMC, under aerobic and hypoxic conditions [Citation49]. Although they did not measure drug uptake, they found that the rate of formation of active drug increased remarkably when hyperthermia was added to drug. Further, they demonstrated that the thermal enhancement of MMC killing was greater in hypoxic cells than in aerobic cells. Considering that bladder cancer can be hypoxic, this provides very strong rationale for using MMC in combination with hyperthermia [Citation54].
Gemcitabine
We were able to identify one paper in which intratumoural gemcitabine concentrations were measured after hyperthermia treatment (42 °C). These authors did not observe any increase in gemcitabine uptake with hyperthermia [Citation55]. We were unable to identify any in vitro studies that examined the question of whether hyperthermia augmented drug uptake.
Hyperthermia (43 °C) has been shown to enhance the cytotoxicity of gemcitabine, however. In one study, two pancreatic cell lines were assessed for cell viability by the WST-8 assay, which reports on cell mass after treatment [Citation56]. This assay reflects the balance between cell death and cell proliferation. Reduction in WST-8 signal occurred in two scenarios: when heat was administered 24 h prior to drug, or when the two treatments were given simultaneously. The enhancement in cell killing was associated with thermal inhibition of NFĸB activation by tumour cells.
Vertrees et al. examined the combination of gemcitabine and hyperthermia (temperatures between 40 and 44 °C for periods ranging from 60 to 180 min) using a human non-small-cell lung cancer line [Citation57] .The optimal sequence was as discussed above, where hyperthermia was administered 24 h prior to drug treatment. The optimal temperature was found to be 43 °C. Using isobologram analysis of in vitro survival data, they demonstrated that this combination led to synergistic killing. The mechanism of killing appeared to be related to activation of apoptosis. Interestingly, however, they were able to demonstrate a significant prolongation of tumour regrowth when tumour-bearing animals were exposed to 40 °C for 1 h, 24 h prior to gemcitabine treatment. Haveman also reported that a 24-h delay between hyperthermia treatment and gemcitabine yielded the greatest level of cell killing as assessed by clonogenic assay [Citation58].
Mohamed et al. reported that administration of gemcitabine immediately prior to heating at 41.5 °C led to enhanced antitumour effect [Citation59]. Close examination of these data, however, shows that the enhancement was quite small in magnitude.
In contrast to most of the results reported above suggesting that a 24-h period between heating and administration of gemcitabine was required for optimal enhanced cytotoxicity, Raoof et al. recently reported that enhanced antitumour effect in a hepatoma tumour line was seen regardless of whether gemcitabine was given 22 h after or immediately after heating [Citation40].
Given the recent results of Raoof et al., it would seem prudent to conduct additional studies, using thermal conditions seen with bladder heating, to determine whether heating immediately prior to drug administration would yield positive effects. Logistically, this would much easier to translate to the clinic, than requiring a 24-h period between the two treatments.
Doxorubicin
There are several reports in the literature demonstrating that hyperthermia increases doxorubicin uptake and retention by cells. In some reports, hyperthermia enhanced doxorubicin cytotoxicity. Further, hyperthermia facilitated uptake into both drug-sensitive and drug-resistant tumour cells. Key conceptual papers are discussed. Unlike cisplatin and MMC, there is some controversy as to what the ideal temperature would be to facilitate enhanced drug uptake and cell killing.
Hahn was the first investigator to report that hyperthermia increases doxorubicin uptake by tumour cells [Citation60]. Using the CHO cell line, they observed little enhancement of doxorubicin cytotoxicity at 41 °C, but substantial enhancement at 42 °C. Using an in vivo, in vitro clonogenic assay of the EMT6 tumour grown in mice, they demonstrated that 43 °C heating yielded a dose-modifying factor for cell survival of nearly six. Concomitantly, they observed a threefold increase in fluorescence intensity of doxorubicin in 43 °C heated cells, compared with 37 °C controls.
Bates and McKillop examined the effects of hyperthermia (39–45 °C) on doxorubicin cytotoxicity of drug-sensitive and drug-resistant CHO cells [Citation61]. Hyperthermia (43 °C) enhanced the rate of uptake of radiolabelled doxorubicin by approximately 80%. Enhancement of doxorubicin cytotoxicity of the drug sensitive line was seen at temperatures >39 °C (two- to threefold increase in cell killing compared with 37 °C), but the greatest enhancements in cytotoxicity were seen at temperatures of ≥42 °C (relative increase in cell killing by factors of 10, 50 and 100 at T = 42, 43 and 45 °C, respectively). In contrast, hyperthermia administration, even up to temperatures of 47 °C, did not increase drug cytotoxicity of the drug-resistant line.
Using an elegant confocal approach, Kawai et al., demonstrated that 43 °C hyperthermia increased the accumulation of doxorubicin into the nuclei of both drug-sensitive and drug-resistant oesophageal carcinoma cell lines [Citation62]. The enhancement in uptake was 4.3 and 7.2-fold for the drug-sensitive and drug-resistant lines. Hyperthermia enhanced the cytotoxicity of doxorubicin in both the wild-type and drug-resistant cell lines. In fact, the enhancement of cytotoxicity was equivalent between the two cell types in this study.
Epirubicin
We were unable to identify any papers that examined the effects of hyperthermia on epirubicin drug uptake. The toxicity of epirubicin-treated cells under hyperthermic conditions was examined in a paper that is described more fully below.
Hyperthermia inactivates membrane drug pumps and intracellular detoxification mechanisms
Inactivation of detoxification mechanisms could increase drug uptake by urothelium for transport to deeper tumour cell layers. These same mechanisms will be important for enhancing tumour cell killing as well. Such mechanisms include ATP-binding cassette (ABC) drug pumps, such as p-glycoprotein [Citation63], and antioxidant mechanisms such as glutathione [Citation64,Citation65].
Hyperthermia has been reported to inactivate multiple drug detoxification mechanisms. From a phenomenological point of view, Wallner et al. were among the first to report that hyperthermia could at least partially reverse drug resistance to MMC. They demonstrated that drug uptake was increased by heat in wild-type and MMC-resistant CHO cells [Citation53]. The increases in drug uptake were associated with enhanced cytotoxicity. It has also been reported that hyperthermia reduces cell membrane localisation of multidrug resistance proteins, when combined with MMC [Citation66]. If cellular uptake of drug is increased as a result of inactivating pumps, this will contribute to maintaining a drug concentration gradient in the tissue. Small molecules such as MMC diffuse through tissue, driven by their concentration gradient [Citation67]. Once the drug enters a cell, it no longer contributes to the gradient.
Glutathione is the primary intracellular modifier of oxidative stress. It has been shown to play a role in reducing cytotoxicity of a variety of drugs, including MMC [Citation68]. Laskowitz et al. demonstrated that hyperthermia reduced intracellular glutathione levels and that this was associated with enhanced in vivo activity of melphalan against a drug-resistant rhabdomyosarcoma xenograft [Citation69]. The reduction in glutathione levels may in part be due to an increase in oxidative stress that has been associated with hyperthermia treatment [Citation70,Citation71].
Rationale for intravenous drug administration in the treatment of NMIBC
Intravenous drug administration has not been considered clinically viable for NMIBC, because of the low probability that patients that present with NMIBC would have spread of their disease beyond the confines of the bladder wall. Under the mantra of ’doing no harm‘, it is difficult to justify administering a toxic drug to such a population of patients, since such drugs have substantial systemic toxicities. Furthermore it is also important to realise that the drug dose administered in the bladder is much higher than the dose that can be used intravenously.
Theoretically, however, administration of systemic drug has some merit, particularly considering the fact that NMIBC often has a vascular supply. If one were able to administer a drug systemically, but stimulate its action selectively in the bladder, then systemic toxicities could be minimised. One such approach might be to use a thermally sensitive drug carrier, such as a liposome or other nanoparticle [Citation72,Citation73]. In this case, hyperthermia could be used to stimulate enhanced drug release in the bladder. Clinical trials with a doxorubicin-containing thermosensitive liposome indicate that the systemic toxicities are no higher or different than those of free drug, when given at the maximally tolerated dose [Citation74,Citation75]. However, local hyperthermia triggers intravascular drug release in the heated zone. This mechanism enhances drug delivery to the tumour many fold, increases drug diffusion distance from the vasculature and enhances antitumour activity of several drugs, including doxorubicin, cisplatin and gemcitabine [Citation76–80]. Because of the differential in systemic versus local effects of these formulations, one could administer less than the maximally tolerated dose and still enhance drug delivery to the bladder tumour. However, this approach still has to be considered experimental.
Summary regarding temperature dependence of drug uptake and cytotoxicity
Since the target temperature for intravesical hyperthermia is 41–42 °C, we will summarise the salient points about how temperatures in this range can alter drug uptake and cytotoxicity. For both cisplatin and MMC, heating in this temperature range will increase drug uptake and enhance cell killing by substantial amounts. The most efficient cell killing occurs if the drugs and heat are given simultaneously.
The effects of hyperthermia on doxorubicin accumulation and cytotoxicity are somewhat more complicated. The literature shows that minor enhancements in cell killing can be seen at 41 °C, but the enhancement is much greater at >42 °C. Therefore, when using doxorubicin it may be advised to push intravesical temperatures to 42 °C or even slightly above.
Currently, one report indicates that hyperthermia does not increase gemcitabine uptake; but there are several reports showing that gemcitabine cytotoxicity is enhanced by hyperthermia. In most studies, the greatest enhancement occurs if the drug is given 24 h after heating. However, recent data suggest that similar antitumour effects can be seen when gemicitabine is given immediately after hyperthermia.
Direct comparison of hyperthermia combined with four drugs
In 2005 we reported the cytotoxicity results of MMC, epirubicin, gemcitabine and EO9 with or without hyperthermia in four human bladder cancer cell lines (RT4, RT112, 253J and T24) [Citation81]. MMC is an antibiotic antitumour agent (molecular weight (MW) 334 g/mol) that interacts with DNA. Cytotoxicity is a direct result of DNA damage. MMC is the most frequently used intravesical chemotherapeutic agent. Epirubicin is also a frequently used agent in NMIBC. It is an analogue of the antibiotic antitumour agent doxorubicin (MW 544 g/mol). It binds to DNA and inhibits nucleic acid synthesis and function. An intravesically less frequently used drug is gemcitabine, a deoxycytidine analogue (MW 300 g/mol). After intracellular activation, the active metabolite is incorporated in the DNA and results in DNA synthesis inhibition. The fourth agent studied, EO9, is a bio-reductive alkylating indoloquinone (MW 288 g/mol). The activity of EO9 is presumably related to the presence of DT-diaphorase enzyme in tumour tissue. This bioreductive drug showed good activity in vitro but failed when given intravenously. The reason for this poor efficacy in vivo is the rapid clearance from the blood stream. However, this is of no relevance when used intravesically [Citation82].
In our preclinical study, cells were treated for 60 min with increasing concentrations of the four chemotherapeutic agents mentioned at 37 or 43 °C. Cell survival was determined by 3-(4,5-dimethylthiazol-2-yl)-2,5-diphenyltetrazoliumbromide (MTT) assay. An inverse relation between cell proliferation and drug concentrations was demonstrated. EO9 proved to be the most potent agent at both temperatures (). In this experiment hyperthermia alone did not decrease cell proliferation. However, a synergistic effect of hyperthermia on decreased cell proliferation was demonstrated in all cell lines and chemotherapeutic agents used, although each reached a maximum at a different drug concentration (). The extent of inhibition was different between the drugs. The highest level of synergism was seen in cells treated with epirubicin, and this occurred at lower drug concentrations. One of the explanations for increased effect was a higher uptake of the drugs. A counterargument is the limited effect on cell death shown in gemcitabine, a drug with a much lower molecular weight than epirubicin. A more plausible explanation for the increased effect is a drug-specific activation under hyperthermic conditions.
Figure 3. Cell survival curves using increasing concentrations EO9, the most potent drug in our preclinical study. Effect of 1 h hyperthermia (43 °C) and EO9 treatment (red line, triangle) on the growth of four human bladder cancer cell lines compared to cells treated 1 h with EO9 at 37 °C (blue line, square).The dotted line indicates the LD50 axis. The vertical axis represents the cell survival (scale 0–1) and the horizontal axis represents the concentration EO9 in μg/mL.
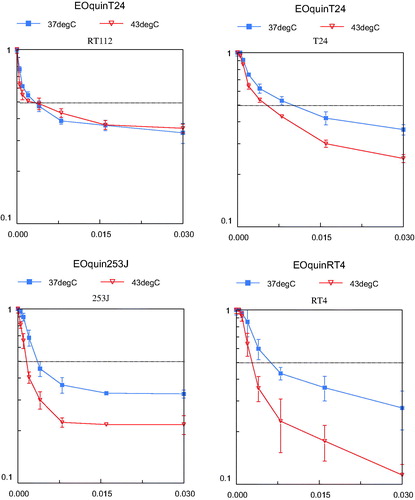
Figure 4. Cell survival curves using increasing concentrations MMC, the most often used intravesical chemotherapeutic drug. Effect of 1 h hyperthermia (43 °C) and MMC treatment (red line, triangle) on the growth of four human bladder cancer cell lines compared to cells treated 1 h with MMC at 37 °C (blue line, square). The dotted line indicates the LD50 axis. The vertical axis represents the cell survival (scale 0–1) and the horizontal axis represents the concentration MMC in μg/mL.
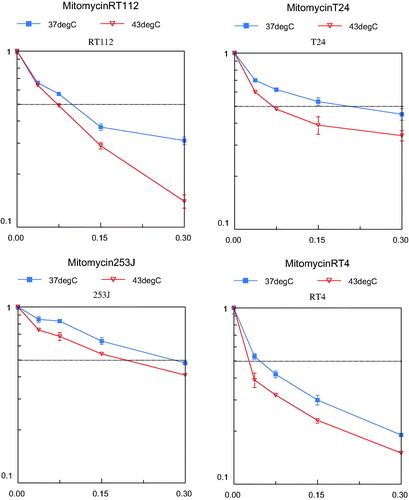
Summary and conclusions
The remarkable success of intravesical MMC with hyperthermia in the treatment of NMIBC lends strong rationale for moving toward making this therapy a standard of care. However, the mechanisms underlying the success of this combination therapy are only partially understood. We have provided some clues as to what may be in play. More work needs to be done to investigate mechanisms that lead to enhanced antitumour effect when combining hyperthermia with agents that can be used for intravesical treatment.
As a final point, there have been several clinical reports using MMC and hyperthermia to treat patients whose tumours have recurred after BCG therapy.[Citation2]. It is curious, however, that we were unable to identify any reports testing whether hyperthermia augments the efficacy of BCG. Since the mechanism underlying efficacy of BCG is thought to be immunological, one might believe hyperthermia with BCG would prove beneficial. There is strong evidence that heat shock proteins play an important role in the efficacy of BCG [Citation83]. Hyperthermia increases the expression of heat shock proteins and enhances antigen presentation by dendritic cells [Citation84,Citation85]. Via a range of effects, hyperthermia boosts the immune system to increase antitumour efficacy [Citation86–91]. These effects may extend to tumour-bearing areas that are not effectively heated, via abscopal immune mediated effects [Citation92].
Acknowledgements
The authors thank Fan Yuan for his helpful discussions of the effects of tissue oedema on drug diffusion.
Disclosure statement
The authors report no conflicts of interest. The authors alone are responsible for the content and writing of the paper.
References
- Colombo R, Salonia A, Leib Z, Pavone-Macaluso M, Engelstein D. Long-term outcomes of a randomized controlled trial comparing thermochemotherapy with mitomycin-C alone as adjuvant treatment for non-muscle-invasive bladder cancer (NMIBC). BJU Int 2011;107:912–18.
- Nativ O, Witjes JA, Hendricksen K, Cohen M, Kedar D, Sidi A, et al. Combined thermo-chemotherapy for recurrent bladder cancer after bacillus Calmette-Guerin. J Urol 2009;182:1313–17.
- Minchinton AI, Tannock IF. Drug penetration in solid tumours. Nat Rev Cancer 2006;6:583–92.
- Jain RK, Stylianopoulos T. Delivering nanomedicine to solid tumors. Nat Rev Clin Oncol 2010;7:653–64.
- Babjuk M, Burger M, Zigeuner R, Shariat SF, van Rhijn BWG, Comperat E, et al. EAU guidelines on non-muscle-invasive urothelial carcinoma of the bladder: Update 2013. Eur Urol 2013;64:639–53.
- Porter MP, Gore JL, Wright JL. Hospital volume and 90-day mortality risk after radical cystectomy: A population-based cohort study. World J Urol 2011;29:73–7.
- Malmstrom P-U, Sylvester RJ, Crawford DE, Friedrich M, Krege S, Rintala E, et al. An individual patient data meta-analysis of the long-term outcome of randomised studies comparing intravesical mitomycin C versus bacillus Calmette-Guerin for non-muscle-invasive bladder cancer. Eur Urol 2009;56:247–56.
- Breau RH, Karnes RJ, Farmer SA, Thapa P, Cagiannos I, Morash C, et al. Progression to detrusor muscle invasion during urothelial carcinoma surveillance is associated with poor prognosis. BJU Int 2014;113:900–6.
- See WA, Xia Q. Regional chemotherapy for bladder neoplasms using continuous intravesical infusion of doxorubicin – impact of concomitant administration of dimethyl-sulfoxide on drug absorption and antitumor activity. J Natl Cancer Inst 1992;84:510–15.
- Parsons CL, Boychuk D, Jones S, Hurst R, Callahan H. Bladder surface glycosaminoglycans – an epithelial permeability barrier. J Urol 1990;143:139–42.
- Kohli AG, Kivimaee S, Tiffany MR, Szoka FC. Improving the distribution of Doxil (R) in the tumor matrix by depletion of tumor hyaluronan. J Control Release 2014;191:105–14.
- Ranney D, Antich P, Dadey E, Mason R, Kulkarni P, Singh O, et al. Dermatan carriers for neovascular transport targeting, deep tumor penetration and improved therapy. J Control Release 2005;109:222–35.
- Mugabe C, Matsui Y, So AI, Gleave ME, Baker JHE, Minchinton AI, et al. In vivo evaluation of mucoadhesive nanoparticulate docetaxel for intravesical treatment of non-muscle-invasive bladder cancer. Clin Cancer Res 2011;17:2788–98.
- Topazio L, Miano R, Maurelli V, Gaziev G, Gacci M, Iacovelli V, et al. Could hyaluronic acid (HA) reduce bacillus Calmette–Guerin (BCG) local side effects? Results of a pilot study. BMC Urol 2014;14:64.
- Apodaca G. The uroepithelium: Not just a passive barrier. Traffic 2004;5:117–28.
- Khandelwal P, Abraham SN, Apodaca G. Cell biology and physiology of the uroepithelium. Am J Physiol Renal Physiol 2009;297:F1477–501.
- Wientjes MG, Badalament RA, Wang RC, Hassan F, Au JLS. Penetration of mitomycin-C in human bladder. Cancer Res 1993;53:3314–20.
- Rath-Wolfson L, Moskovitz B, Dekel Y, Kugel V, Koren R. Combined intravesical hyperthermia and mitomycin chemotherapy: A preliminary in vivo study. Int J Exper Pathol 2003;84:145–52.
- Paroni R, Salonia A, Lev A, Da Pozzo LF, Cighetti G, Montorsi F, et al. Effect of local hyperthermia of the bladder on mitomycin C pharmacokinetics during intravesical chemotherapy for the treatment of superficial transitional cell carcinoma. Br J Clin Pharmacol 2001;52:273–8.
- Coss RA, Linnemans WAM. The effects of hyperthermia on the cytoskeleton: A review. Int J Hyperthermia 1996;12:173–96.
- Kong G, Braun RD, Dewhirst MW. Hyperthermia enables tumor-specific nanoparticle delivery: Effect of particle size. Cancer Res 2000;60:4440–5.
- Au JL, Wientjes MG. Combination intravesical hyperthermia and chemotherapy for bladder cancer. Oncology (Williston Park). 2010;26:1155–60.
- Inman BA, Etienne W, Rubin R, Owusu RA, Oliveira TR, Rodriques DB, et al. The impact of temperature and urinary constituents on urine viscosity and its relevance to bladder hyperthermia treatment. Int J Hyperthermia 2013;29:206–10.
- Hildebrandt B, Wust P, Ahlers O, Dieing A, Sreenivasa G, Kerner T, et al. The cellular and molecular basis of hyperthermia. Crit Rev Oncol Hematol 2002;43:33–56.
- Ware MJ, Tinger S, Colbert KL, Corr SJ, Rees P, Koshkina N, et al. Radiofrequency treatment alters cancer cell phenotype. Sci Rep 2015;5:12083.
- Ajili F, Kacem M, Tounsi H, Darouiche A, Enayfer E, Chebi M, et al. Prognostic impact of angiogenesis in nonmuscle invasive bladder cancer as defined by microvessel density after immunohistochemical staining for CD34. Ultrastruct Pathol 2012;36:336–42.
- Song CW. Effect of local hyperthermia on blood-flow and microenvironment – a review. Cancer Res 1984;44:4721–30.
- Chen C, Roemer RB. A thermo-pharmacokinetic model of tissue temperature oscillations during localized heating. Int J Hyperthermia 2005;21:107–24.
- Kim E-C, Choi S-K, Lim M, Yeon S-I, Lee Y-H. Role of endogenous ENaC and TRP channels in the myogenic response of rat posterior cerebral arteries. PLoS one 2013;8:e84194.
- Caterina MJ, Rosen TA, Tominaga M, Brake AJ, Julius D. A capsaicin-receptor homologue with a high threshold for noxious heat. Nature 1999;398(6726):436–41.
- Vujaskovic Z, Poulson JM, Gaskin AA, Thrall DE, Page RL, Charles HC, et al. Temperature-dependent changes in physiologic parameters of spontaneous canine soft tissue sarcomas after combined radiotherapy and hyperthermia treatment. Int J Radiat Oncol Biol Phys 2000;46:179–85.
- Jones EL, Prosnitz LR, Dewhirst MW, Marcom PK, Hardenbergh PH, Marks LB, et al. Thermochemoradiotherapy improves oxygenation in locally advanced breast cancer. Clin Cancer Res 2004;10:4287–93.
- Colombo R, Do Pozzo LF, Salonia A, Rigatti P, Leib Z, Baniel J, et al. Multicentric study comparing intravesical chemotherapy alone and with local microwave hyperthermia for prophylaxis of recurrence of superficial transitional cell carcinoma. J Clin Oncol 2003;21:4270–6.
- Inman BA, Stauffer PR, Craciunescu OA, Maccarini PF, Dewhirst MW, Vujaskovic Z. A pilot clinical trial of intravesical mitomycin-C and external deep pelvic hyperthermia for non-muscle-invasive bladder cancer. Int J Hyperthermia 2014;30:171–5.
- Huang C, Li Y, Cao P, Xie Z, Qin Z. Synergistic effect of hyperthermia and neferine on reverse multidrug resistance in adriamycin-resistant SGC7901/ADM gastric cancer cells. J Huazhong Univ Sci Technol Med Sci 2011;31:488–96.
- Alvarez-Berrios MP, Castillo A, Mendez J, Soto O, Rinaldi C, Torres-Lugo M. Hyperthermic potentiation of cisplatin by magnetic nanoparticle heaters is correlated with an increase in cell membrane fluidity. Int J Nanomed 2013;8:1003–13.
- Iliakis G, Wu W, Wang M. DNA double strand break repair inhibition as a cause of heat radiosensitization: Re-evaluation considering backup pathways of NHEJ. Int J Hyperthermia. 2008;24:17–29.
- Krawczyk PM, Eppink B, Essers J, Stap J, Rodermond H, Odijk H, et al. Mild hyperthermia inhibits homologous recombination, induces BRCA2 degradation, and sensitizes cancer cells to poly (ADP-ribose) polymerase-1 inhibition. Proc Natl Acad Sci USA 2011;108:9851–6.
- Mivechi NF, Dewey WC. DNA polymerase-alpha and DNA polymerase-beta activities during the cell-cycle and their role in heat radiosensitization in Chinese-hamster ovary cells. Radiat Res 1985;103:337–50.
- Raoof M, Zhu C, Cisneros BT, Liu H, Corr SJ, Wilson LJ, et al. Hyperthermia inhibits recombination repair of gemcitabine-stalled replication forks. J Natl Cancer Inst 2014;106. doi: 10.1093/jnci/dju183.
- Alberts DS, Peng YM, Chen HSG, Moon TE, Cetas TC, Hoeschele JD. Therapeutic synergism of hyperthermia-cis-platinum in a mouse-tumor model. J Natl Cancer Inst 1980;65:455–61.
- Meyn RE, Corry PM, Fletcher SE, Demetriades M. Thermal enhancement of DNA damage in mammalian cells treated with cis-diamminedichloroplatinum(II). Cancer Res 1980;40:1136–9.
- Los G, Vanvugt MJH, Pinedo HM. Response of peritoneal solid tumors after intraperitoneal chemohyperthermia treatment with cisplatin or carboplatin. Br J Cancer. 1994;69:235–41.
- Los G, Vanvugt MJH, Denengelse L, Pinedo HM. Effects of temperature on the interaction of cisplatin and carboplatin with cellular DNA. Biochem Pharmacol 1993;46:1229–37.
- Landon CD, Benjamin SE, Ashcraft KA, Dewhirst MW. A role for the copper transporter Ctr1 in the synergistic interaction between hyperthermia and cisplatin treatment. Int J Hyperthermia 2013;29:528–38.
- Wallner KE, Li GC. Effect of drug exposure duration and sequencing on hyperthermic potentiation of mitomycin-C and cisplatin. Cancer Res 1987;47:493–5.
- Mizuno S, Amagai M, Ishida A. Synergistic cell killing by anti-tumor agents and hyperthermia in cultured cells. Gann 1980;71:471–8.
- Nakajima K, Hisazumi H. Enhanced radioinduced cytotoxicity of cultured human bladder-cancer cells using 43-degrees-C hyperthermia or anticancer drugs. Urol Res 1987;15:255–60.
- Teicher BA, Kowal CD, Kennedy KA, Sartorelli AC. Enhancement by hyperthermia of the in vitro cyto-toxicity of mitomycin-C toward hypoxic tumor-cells. Cancer Res 1981;41:1096–9.
- Herman TS, Teicher BA, Holden SA. Trimodality therapy (drug/hyperthermia radiation) with BCNU or mitomycin-C. Int J Radiat Oncol Biol Phys 1990;18:375–82.
- Herman TS, Teicher BA, Holden SA. Addition of mitomycin-C to cis-diamminedichloroplatinum(II)/hyperthermia radiation-therapy in the FSaIIC fibrosarcoma. Int J Hyperthermia 1991;7:893–903.
- Sakaguchi Y, Kohnoe S, Emi Y, Maehara Y, Kusumoto T, Sugimachi K. Cytotoxicity of mitomycin-C and carboquone combined with hyperthermia against hypoxic tumor cells in vitro. Oncology 1992;49:227–32.
- Wallner KE, Banda M, Li GC. Hyperthermic enhancement of cell killing by mitomycin-C in mitomycin-C-resistant Chinese hamster ovary cells. Cancer Res 1987;47(5):1308–12.
- Hoskin PJ, Sibtain A, Daley FM, Saunders MI, Wilson GD. The immunohistochemical assessment of hypoxia, vascularity and proliferation in bladder carcinoma. Radiother Oncol 2004;72:159–68.
- Kirui DK, Celia C, Molinaro R, Bansal SS, Cosco D, Fresta M, et al. Mild hyperthermia enhances transport of liposomal gemcitabine and improves in vivo therapeutic response. Adv Healthc Mater 2015;4:1092–103.
- Adachi S, Kokura S, Okayama T, Ishikawa T, Takagi T, Handa O, et al. Effect of hyperthermia combined with gemcitabine on apoptotic cell death in cultured human pancreatic cancer cell lines. Int J Hyperthermia 2009;25:210–19.
- Vertrees RA, Das GC, Popov VL, Coscio AM, Goodwin TJ, Logrono R, et al. Synergistic interaction of hyperthermia and gemcitabine in lung cancer. Cancer Biol Therapy 2005;4:1144–53.
- Haveman J, Rietbroek RC, Geerdink A, Vanrijn J, Bakker PJM. Effect of hyperthermia on the cytotoxicity of 2',2'-difluorodeoxycytidine (gemcitabine) in cultured SW1573 cells. Int J Cancer 1995;62:627–30.
- Mohamed F, Marchettini P, Stuart OA, Urano M, Sugarbaker PH. Thermal enhancement of new chemotherapeutic agents at moderate hyperthermia. Ann Surg Oncol 2003;10:463–8.
- Hahn GM, Braun J, Harkedar I. Thermochemotherapy – synergism between hyperthermia (42–43 degrees) and adriamycin (or bleomycin) in mammalian-cell inactivation. Proc Natl Acad Sci US A 1975;72:937–40.
- Bates DA, Mackillop WJ. Hyperthermia, adriamycin transport, and cytotoxicity in drug-sensitive and drug-resistant Chinese hamster ovary cells. Cancer Res1986;46:5477–81.
- Kawai H, Minamiya Y, Kitamura M, Matsuzaki I, Hashimoto M, Suzuki H, et al. Direct measurement of doxorubicin concentration in the intact, living single cancer cell during hyperthermia. Cancer 1997;79:214–19.
- Dean M, Fojo T, Bates S. Tumour stem cells and drug resistance. Nat Rev Cancer 2005;5:275–84.
- Batist G, Tulpule A, Sinha BK, Katki AG, Myers CE, Cowan KH. Overexpression of a novel anionic glutathione transferase in multidrug-resistant human-breast cancer cells. J Biol Chem 1986;261:5544–9.
- Trachootham D, Alexandre J, Huang P. Targeting cancer cells by ROS-mediated mechanisms: A radical therapeutic approach? Nat Rev Drug Discov 2009;8:579–91.
- Franke K, Kettering M, Lange K, Kaiser WA, Hilger I. The exposure of cancer cells to hyperthermia, iron oxide nanoparticles, and mitomycin C influences membrane multidrug resistance protein expression levels. Int J Nanomed 2013;8:351–63.
- Hicks KO, Pruijn FB, Secomb TW, Hay MP, Hsu R, Brown JM, et al. Use of three-dimensional tissue cultures to model extravascular transport and predict in vivo activity of hypoxia-targeted anticancer drugs. J Natl Cancer Inst 2006;98:1118–28.
- Arrick BA, Nathan CF. Glutathione metabolism as a determinant of therapeutic efficacy – a review. Cancer Res 1984;44:4224–32.
- Laskowitz DT, Elion GB, Dewhirst MW, Griffith OW, Savina PM, Blum MR, et al. Hyperthermia-induced enhancement of melphalan activity against a melphalan-resistant human rhabdomyosarcoma xenograft. Radiat Res 1992;129:218–23.
- Moon EJ, Sonveaux P, Porporato PE, Danhier P, Gallez B, Batinic-Haberle I, et al. NADPH oxidase-mediated reactive oxygen species production activates hypoxia-inducible factor-1 (HIF-1) via the ERK pathway after hyperthermia treatment. Proc Natl Acad Sci USA 2010;107:20477–82.
- Mitchell JB, Russo A. Thiols, thiol depletion, and thermosensitivity. Radiat Res 1983;95:471–85.
- McDaniel JR, MacEwan SR, Li XH, Radford DC, Landon CD, Dewhirst M, et al. Rational design of ‘heat seeking’ drug loaded polypeptide nanoparticles that thermally target solid tumors. Nano Lett 2014;14:2890–5.
- Landon C, Park JY, Needham D, Dewhirst M. Nanoscale drug delivery and hyperthermia: The materials design and preclinical and clinical testing of low temperature sensitive liposomes used in combination with mild hyperthermia in the treatment of local cancer. Open Nanomed J 2011;3:38–64.
- Zagar TM, Vujaskovic Z, Formenti S, Rugo H, Muggia F, O’Connor B, et al. Two phase I dose-escalation/pharmacokinetics studies of low temperature liposomal doxorubicin (LTLD) and mild local hyperthermia in heavily pretreated patients with local regionally recurrent breast cancer. Int J Hyperthermia 2014;30:285–94.
- Poon RTP, Borys N. Lyso-thermosensitive liposomal doxorubicin: An adjuvant to increase the cure rate of radiofrequency ablation in liver cancer. Future Oncol 2011;7:937–45.
- Kneidl B, Peller M, Winter G, Lindner LH, Hossann M. Thermosensitive liposomal drug delivery systems: State of the art review. Int J Nanomed 2014;9:4387–98.
- Koning GA, Eggermont AMM, Lindner LH, ten Hagen TLM. Hyperthermia and Thermosensitive Liposomes for Improved Delivery of Chemotherapeutic Drugs to Solid Tumors. Pharmaceut Res 2010;27:1750–4.
- Manzoor AA, Lindner LH, Landon CD, Park JY, Simnick AJ, Dreher MR, et al. Overcoming limitations in nanoparticle drug delivery: Triggered, intravascular release to improve drug penetration into tumors. Cancer Res 2012;72:5566–75.
- Dou YN, Zheng JZ, Foltz WD, Weersink R, Chaudary N, Jaffray DA, et al. Heat-activated thermosensitive liposomal cisplatin (HTLC) results in effective growth delay of cervical carcinoma in mice. J Control Release 2014;178:69–78.
- Lim SK, Shin DH, Choi MH, Kim JS. Enhanced antitumor efficacy of gemcitabine-loaded temperature-sensitive liposome by hyperthermia in tumor-bearing mice. Drug Devel Ind Pharm 2014;40:470–6.
- van der Heijden AG, Verhaegh G, Jansen CFJ, Schalken JA, Witjes JA. Effect of hyperthermia on the cytotoxicity of 4 chemotherapeutic agents currently used for the treatment of transitional cell carcinoma of the bladder: An in vitro study. J Urol 2005;173:1375–80.
- Loadman PM, Bibby MC, Phillips RM. Pharmacological approach towards the development of indolequinone bioreductive drugs based on the clinically inactive agent EO9. Br J Pharmacol 2002;137:701–9.
- Ischia J, So AI. The role of heat shock proteins in bladder cancer. Nat Rev Urol 2013;10:386–95.
- Dayanc BE, Bansal S, Gure AO, Gollnick SO, Repasky EA. Enhanced sensitivity of colon tumour cells to natural killer cell cytotoxicity after mild thermal stress is regulated through HSF1-mediated expression of MICA. Int J Hyperthermia 2013;29:480–90.
- Torigoe T, Hirohashi Y, Yasuda K, Sato N. Constitutive expression and activation of stress response genes in cancer stem-like cells/tumour initiating cells: Potent targets for cancer stem cell therapy. Int J Hyperthermia 2013;29:436–41.
- Toraya-Brown S, Fiering S. Local tumour hyperthermia as immunotherapy for metastatic cancer. Int J Hyperthermia 2014;30:531–9.
- Calderwood SK. From stress protein biochemistry to novel immunotherapeutics. Int J Hyperthermia 2013;29:362–3.
- Graner MW, Romanoski A, Katsanis E. The ‘peptidome’ of tumour-derived chaperone-rich cell lysate anti-cancer vaccines reveals potential tumour antigens that stimulate tumour immunity. Int J Hyperthermia 2013;29:380–9.
- Mayer-Sonnenfeld T, Har-Noy M, Lillehei KO, Graner MW. Proteomic analyses of different human tumour-derived chaperone-rich cell lysate (CRCL) anti-cancer vaccines reveal antigen content and strong similarities amongst the vaccines along with a basis for CRCL’s unique structure: CRCL vaccine proteome leads to unique structure. Int J Hyperthermia 2013;29:520–7.
- Repasky EA. Progress in development of biomedical applications of heat shock proteins and thermal stress. Int J Hyperthermia 2013;29:359–61.
- Van Herwijnen MJC, Van der Zee R, Van Eden W, Broere F. Heat shock proteins can be targets of regulatory T cells for therapeutic intervention in rheumatoid arthritis. Int J Hyperthermia 2013;29:448–54.
- Viglianti BL, Dewhirst MW, Boruta RJ, Park JY, Landon C, Fontanella AN, et al. Systemic anti-tumour effects of local thermally sensitive liposome therapy. Int J Hyperthermia 2014;30:385–92.