Abstract
Purpose: Oxidative stress plays a central role in heat stress-induced gastrointestinal injury. Punicalagin (PUN), a major polyphenol abundant in pomegranate fruit, husk and juice, exhibits antioxidative effects. In this study we used a heat stress model to investigate the intestinal protection effect of PUN and the underlying mechanisms. Materials and methods: IEC-6 cells were pretreated with PUN for 6 h and exposed to 42 °C for 6 h. Intracellular reactive oxygen species levels, malondialdehyde, nitrogen oxide, and superoxide dismutase activity were measured. IEC-6 cells were treated with PUN at different times and doses, the protein levels of haeme oxygenase-1 (HO-1) were evaluated. The nuclear translocation of the transcription factor NF-erythroid 2-related factor (Nrf2) and the phosphorylation level of PI3K/Akt were also investigated. Results: PUN significantly decreased the heat stress-induced cell death and apoptosis. Heat stress increased reactive oxygen species, malondialdehyde and nitrogen oxide production, while it decreased superoxide dismutase activity. These effects were markedly inversed when the cells were pretreated with PUN. Furthermore, PUN treatment induced the expression of HO-1 and increased Nrf2 nuclear translocation in a time- and dose-dependent manner. The Nrf2-related cytoprotective effects of PUN were via the PI3K/Akt signalling pathway, as LY294002, a specific PI3K/Akt inhibitor, suppressed the PUN-induced nuclear translocation of Nrf2, the HO-1 up-regulation and the protective effect of PUN against oxidative stress. Conclusions: PUN protects IEC-6 cells against oxidative stress by up-regulating the expression of HO-1 via a mechanism that involves PI3K/Akt activation and Nrf2 translocation.
Introduction
The intestinal epithelium is in direct contact with a variety of nutrients, microbes and exogenous toxins, offering both a barrier and absorptive functions in the intestine [Citation1]. The integrity of the small intestinal epithelium determines its normal physiological function [Citation2,Citation3]. However, the integrity of the intestinal epithelium is easily destroyed by many stressors such as radiation, lipopolysaccharides (LPS), endotoxins and heat stress [Citation4,Citation5]. Concerning heat stress, reactive oxygen species (ROS) play a crucial role in heat stress-induced injury in the rat small intestine [Citation6]. Overproduction of ROS disrupts the balance between oxidation and antioxidant defence systems, as it can cause intracellular protein and DNA damage, lipid peroxidation, and induce apoptosis of intestinal epithelial cells [Citation7,Citation8]. Therefore, it is essential for cells to up-regulate antioxidants by activating their defence mechanisms.
Nuclear factor-erythroid 2-related factor 2 (Nrf2) is a transcription factor that binds to antioxidant response element (ARE) and regulates ARE-mediated gene expression [Citation9]. Under physiological conditions, Nrf2 is sequestered to the cytoplasm by binding to Kelch-like ECH-associated protein-1 (Keap1) [Citation10]. In response to intracellular oxidative stress, Nrf2 separates from Keap1 and translocates into the nucleus to activate the expression of antioxidant genes [Citation11]. Studies have shown that antioxidants are crucial for cells to prevent damage caused by ROS-mediated oxidative stress [Citation12–14]. Haemeoxygenase-1 (HO-1) is downstream of Nrf2, which is a vital antioxidant [Citation15]. HO-1 is expressed at a low level in normal cells, and is rapidly up-regulated when stimulated by a variety of oxidative-inducing agents, such as heat stress [Citation16], hydrogen peroxide [Citation17] and LPS [Citation18]. Apoptosis is known as programmed cell death, in which cells die under strict control [Citation19]. Many studies have revealed that phosphatidylinositol 3-kinase (PI3K)/Akt is an upstream signalling molecule of Nrf2 and plays a crucial role in regulating cell proliferation, survival and apoptosis [Citation20–22]. Thus, the PI3K/Akt pathway is a potential target of drug therapy.
Punicalagin, or 2,3-(S)-Hexahydroxydiphenoyl-4,6-(S,S)-gallagyl-D-glucose) (PUN), is a major polyphenol, abundant in pomegranate fruit, husk and juice [Citation23]. Previous studies have reported that PUN has antioxidant and anti-inflammation effects [Citation24,Citation25]. However, so far, there is no research focused on the effect of PUN on heat stress-induced oxidative stress in rat intestinal epithelial cell line (IEC-6). IEC-6 has been reported as a homogenous population of epithelial-like cells that are often used in pharmacological and pathological physiology research [Citation26,Citation27]. In this study we show for the first time that PUN is able to attenuate oxidative damage induced by heat stress in IEC-6 cells and up-regulate the activity and expression of HO-1 via activation of the PI3K/Akt signalling pathway and Nrf2 translocation.
Materials and methods
Cell culture
IEC-6 cells (CRL21592, purchased from Peking Union Medical College) were cultured in Dulbecco’s modified Eagle medium (DMEM) high glucose supplemented with 10% fetal bovine serum (FBS) (HyClone, Logun, UT, USA), 2 mg/L insulin, 50 IU/mL penicillin and 50 mg/mL streptomycin. IEC-6 cells were grown in a humid incubator at 37 °C in 5% CO2.
Cell viability assay
IEC-6 cells were seeded in 96-well plates at 5 × 104 cells per well and allowed to grow to 90% confluence in complete medium. Cells were washed twice with phosphate-buffered saline (PBS) and incubated with serum-free medium for 1 h at 37 °C in 5% CO2, then cells were subjected to 42 °C for 1, 3, 6, 9 or 12 h in the incubator. Cell viability was measured by the 3-[4,5-dimethylthiazol-2-yl]-2,5 diphenyl tetrazolium bromide assay (MTT) (Sigma-Aldrich, St Louis, MO, USA). To examine the cytotoxicity of PUN, cells were treated with or without PUN (0–40 μM; Shanghai Tauto Biotech, Shanghai, China) for 48 h. Then the medium was removed and DMEM containing 10% MTT was added to each well. The formazan complex was dissolved in 100 μL dimethyl sulphoxide (DMSO) after 4 h of incubation. The optical density was measured using a microplate reader (Bio-Rad, Hercules, CA) at 490 nm.
Determination of ROS generation
The intracellular generation of ROS was analysed with the probe, 2',7'-dichlorodihydrofluorescein diacetate (DCFH2-DA; Invitrogen, Carlsbad, CA, USA). For this assay, cells were cultured in 24-well plates for 24 h and treated with different conditions. Then the cells were washed twice with PBS, and 20 μM DCFH2-DA was added to the wells for 15 min at 37 °C. Fluorescence was visualised using fluorescence microscopy (Olympus, IX71, Tokyo, Japan) and ROS generation was evaluated with a fluorescence microplate reader (Tecan, Sunrise, Männedorf, Switzerland) at an excitation wavelength of 495 nm and an emission wavelength of 525 nm.
NO assay
Nitric oxide (NO) release from IEC-6 cells was measured using an NO assay kit (Beyotime Company, Beijing, China) according to the manufacturer’s protocol.
SOD activity and MDA content determination
Superoxide dismutase (SOD) activity and malondialdehyde (MDA) content in IEC-6 cells were assessed using commercial kits (Nanjing Jiancheng Bioengineering Institute, Nanjing, China), following the manufacturer’s instructions. SOD activity is expressed as U/mg of total protein and MDA content is expressed as nmol/mg of protein. The total protein concentration was determined using a BCA protein assay kit (Beyotime Biotech, Shanghai, China).
Nuclear protein extraction
IEC-6 cells were harvested following treatment, and then nuclear protein was extracted using the nuclear protein extraction kit (KeyGEN Biotech, Ningjing, China) according to the manufacturer’s instructions. Nuclear protein concentration was determined using a BCA protein assay kit (Beyotime Biotech).
Immunofluorescence staining
IEC-6 cells were grown on coverslips to 100% confluence then treated with various experimental conditions. At the end of the experimental period, IEC-6 cells were washed twice in PBS and fixed with 4% paraformaldehyde for 10 min at room temperature. Then cells were permeabilised with 0.2% Triton X-100 in PBS at 4 °C for 10 min, followed by blocking in 3% BSA for 1 h at room temperature. Cells were incubated overnight at 4 °C with primary antibodies against Nrf2, at a 1:50 dilution. After washing with PBS, coverslips were incubated with Alexa Fluor 488 goat anti-rabbit IgG secondary antibody (Life Technologies, Molecular Probes, Carlsbad, CA) at a dilution of 1:400 for 40 min at room temperature in the dark. Cells were also incubated with 4',6-diamidino-2-phenylindole (DAPI) (Beyotime, Jiangsu, China) for 5 min to stain nuclei. After washing three times with PBS, cells were mounted with Prolong Antifade medium (Solarbio, Beijing, China). Stained cells were determined with a fluorescence microscope (Olympus IX71, Tokyo, Japan).
Assessment of apoptosis by flow cytometry
Cell apoptosis rate was measured using the Annexin V-FITC/PI apoptosis detection kit (KeyGEN Biotech) according to the manufacturer’s protocol. Then the cells were incubated in the dark for 10 min according to the manufacturer’s protocol. The degree of apoptosis was analysed by flow cytometry.
Western blot analysis
IEC-6 cells were treated with the indicated agents, and then protein was extracted using a total protein extraction kit (KeyGEN Biotech). The protein concentration was determined using a BCA protein assay kit (Beyotime Biotech). Proteins were separated by SDS-polyacrylamide and electrotransferred onto nitrocellulose membranes (Pierce, Waltham, MA). Next, the membranes were incubated with specific antibodies. Antibodies against β-actin, AKT and P-AKT, and the inhibitor LY294002 were purchased from Cell Signaling Technology (Danvers, MA). Anti-Nrf2 antibody was obtained from Abcam (Cambridge, UK). Antibodies against HO-1 and PCNA were purchased from Bioworld Technology (Nanjing). Goat anti-rabbit antibody was purchased from LI-COR Odyssey (Lincoln, NE). β-actin or PCNA content was used as an internal control. Densitometric values of immunoblot signals were obtained from three separate experiments using Image J (National Institutes of Health, Bethesda, MD).
Statistical analysis
Data are expressed as mean ± standard error of mean (SEM). Data were analysed for statistical significance by one-way analysis of variance (ANOVA) using SPSS version 16.0 (IBM, Armonk, NY). A p < 0.05 was considered statistically significant. Graphs were created using Graphpad Prism version 5.0.
Results
Effect of PUN on cell viability
IEC-6 cells were incubated with 0, 2.5, 5, 10, 20 or 40 μM PUN for 48 h and cell viability was analysed by MTT assay. The results showed that compared with the control cells PUN had no cytotoxic effect on IEC-6 cells ().
Figure 1. The effect of PUN on IEC-6 cell viability. Cells were incubated with 0, 2.5, 5, 10, 20 or 40 μM PUN for 48 h, and cell viability was analysed by the MTT assay. Data are expressed as mean ± SEM of three independent experiments. Differences between mean values were assessed by one-way ANOVA.
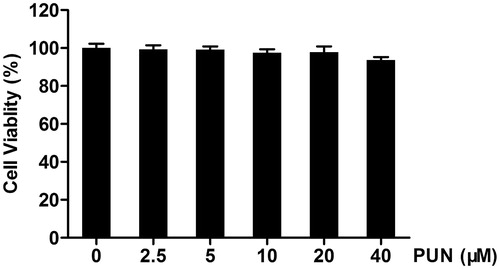
Effect of PUN on heat stress-induced cell death
To examine the cytotoxic effect of heat stress-induced cell injury, cells were exposed to 42 °C for 1, 3, 6, 9 or 12 h and cell viability was measured by MTT assay. We found that the cell viability significantly decreased after exposure to 42 °C for 6 h (). Therefore, cells were exposed to 42 °C for 6 h in the following experiments.
Figure 2. Protective effect of PUN against cell death induced by heat stress. (A) Cells were exposed to 42 °C for 1, 3, 6, 9 or 12 h, and cell viability was analysed by the MTT assay. (B) Cells were pretreated with 2.5, 5 or 10 μM PUN for 6 h and exposed to 42 °C for 6 h, followed by cell viability analysis by the MTT assay. Data are expressed as mean ± SEM of three independent experiments. Differences between mean values were assessed by one-way ANOVA. *p < 0.05 and **p < 0.01 compared with the control group or heat stress-treated group.
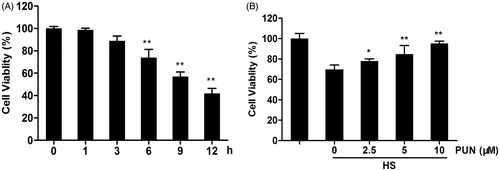
To investigate the effect of PUN on heat stress-induced cell death, cells were pretreated with PUN (2.5, 5 or 10 μM) for 6 h and exposed to 42 °C for another 6 h, followed by cell viability analysis by MTT assay. The results revealed that PUN clearly decreased heat stress-induced cell death in a concentration-dependent manner ().
PUN significantly inhibits heat stress-induced oxidative stress
To investigate the potential antioxidant effect of PUN, cells were pretreated with PUN (2.5, 5 or 10 μM) for 6 h and exposed to 42 °C for another 6 h. ROS production, SOD activity, MDA content and NO release from IEC-6 cells were determined. The results demonstrated that the heat stress challenge significantly increased the level of ROS, MDA and NO, and significantly decreased SOD activity. In contrast, pretreatment with PUN significantly suppressed the increase in ROS, MDA and NO () in a dose-dependent manner. However, SOD activity did not significantly increase until the concentration of PUN was higher than 5 μM ().
Figure 3. Effects of PUN on heat stress-induced ROS, SOD, MDA and NO expression. Cells were pretreated with 2.5, 5 or 10 μM PUN for 6 h and exposed to 42 °C for 6 h. (A) Cells were incubated with DCFH2-DA for 15 min. ROS production was observed using fluorescence microscopy. (B) IEC-6 cells were incubated with DCFH2-DA for 15 min. ROS production was measured by a fluorescence microplate reader. (C and D) SOD and MDA levels were determined by commercial kits according to the manufacturer’s protocol. (E) NO concentration in the supernatant was determined using the Griess reaction. Data are expressed as mean ± SEM of three independent experiments. Differences between mean values were assessed by one-way ANOVA. *p < 0.05 and **p < 0.01 compared with the heat stress-treated group.
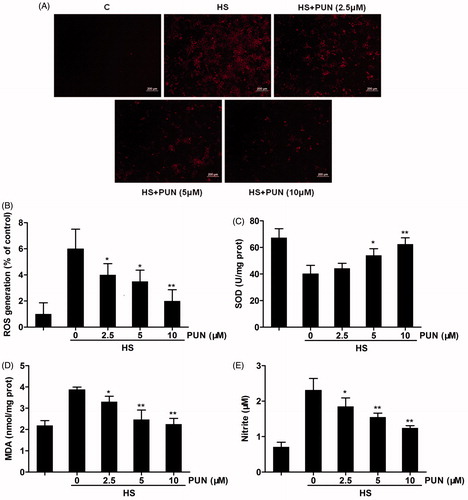
Effect of PUN on HO-1 expression and Nrf2 nuclear translocation in IEC-6 cells
HO-1 is considered as one of the vital antioxidants that protect cells against oxidative damage. To explore whether PUN induces the expression of HO-1 in IEC-6 cells, we examined the protein levels of HO-1. IEC-6 cells were cultured with 10 μM PUN for 1, 3, 6, 9 or 12 h to conduct time-course experiments, and cells were incubated with PUN (2.5, 5 or 10 μM) to carry out concentration-course experiments. HO-1 expression was analysed by Wwestern blotting. The results indicated that PUN induced HO-1 expression in a dose- and time-dependent manner ().
Figure 4. Effect of PUN on HO-1 expression and Nrf2 nuclear translocation in IEC-6 cells. Cells were treated with 10 μM PUN for 1, 3, 6, 9 or 12 h, or with 2.5, 5 or 10 μM PUN for 12 h. (A) and (C) Cells were treated with PUN (10 μM) for the indicated time periods. (B and D) Cells were treated with PUN (0, 2.5, 5 or 10 μM) for 12 h. (E) IEC-6 cells were pretreated with PUN (10 μM) for 12 h and then Nrf2 nuclear translocation was determined by immunofluorescence staining. The green fluorescent staining of Nrf2 overlapped with blue DAPI staining of nucleus, suggesting the nuclear localization. HO-1 and nuclear Nrf2 levels were determined by Western blotting. PCNA was used as nuclear loading control. Data are expressed as mean ± S.E.M. of three independent experiments. Differences between mean values were assessed by one-way ANOVA. *p < 0.05 and **p < 0.01 compared with the control group.
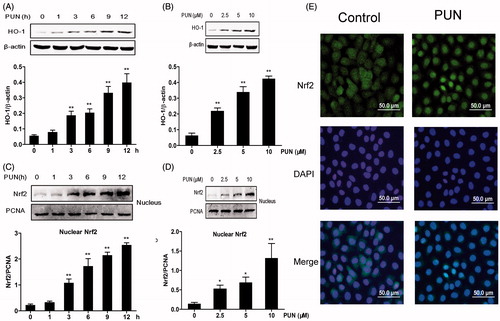
Nrf2, a major transcription factor, induces the expression of HO-1. Thus, we examined Nrf2 nuclear translocation by Western blotting. The results showed that the nuclear expression of Nrf2 increased at 3 h, and continued to rise up to 12 h compared with the control group (). Namely, Nrf2 translocated into the nucleus in a concentration-dependent manner (). We further characterised the Nrf2 nuclear translocation by immunofluorescence staining after treatment of IEC-6 cells with 10 μM PUN for 12 h. Nrf2 stained with FITC presented mostly in the cytoplasm of control group, whereas cells treated with PUN led to the appearance of FITC signal mostly in the nucleus ().
Effect of PUN on the activation of the PI3K/Akt pathway
PI3K/Akt is upstream of Nrf2, and plays a crucial role in the induction of Nrf2/ARE-driven gene expression. Cells were treated with PUN, and the phosphorylation of Akt was analysed by Western blotting. The results indicated that PUN up-regulated Akt phosphorylation in a dose- and time-dependent manner ().
Figure 5. Effect of PUN on PI3K/Akt phosphorylation in IEC-6 cells. (A) Cells were treated with 10 μM PUN for 1, 3, 6, 9 or 12 h. (B) Cells were treated with 2.5, 5 or 10 μM PUN for 12 h. P-Akt and Akt levels were determined by Western blotting, and β-actin was used as a loading control. Data are expressed as mean ± SEM of three independent experiments. Differences between mean values were assessed by one-way ANOVA. *p < 0.05 and **p < 0.01 compared with the control group.
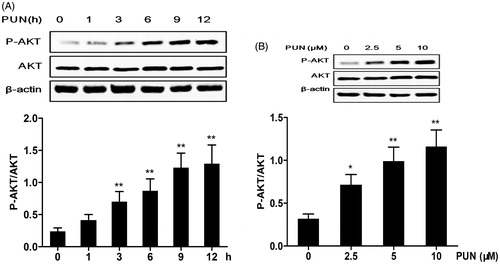
Role of the PI3K/Akt pathway in PUN-induced Nrf2 translocation and HO-1 expression
To elucidate whether activation of the PI3K/Akt signalling pathway is essential for the PUN-induced nuclear translocation of Nrf2 and HO-1 expression, LY294002, a specific PI3K/Akt inhibitor, was used to inhibit Akt phosphorylation. We found that inhibition of the PI3K/Akt pathway significantly suppressed Akt phosphorylation and the nuclear translocation of Nrf2 induced by PUN (). Similarly, treatment of IEC-6 cells with the PI3K/Akt inhibitor completely suppressed the expression of HO-1 induced by PUN ().
Figure 6. Role of the PI3K/Akt pathway in PUN-induced Nrf2 transcription and HO-1 expression. (A–C) IEC-6 cells were pretreated with 10 μM LY294002 for 1 h, and then treated with 10 μM PUN for 12 h. Nrf2, P-Akt and HO-1 levels were determined by Western blotting. PCNA was used as nuclear loading control. Data are expressed as mean ± SEM of three independent experiments. Differences between mean values were assessed by one-way ANOVA. *p < 0.05 and **p < 0.01 compared with the PUN-treated group.
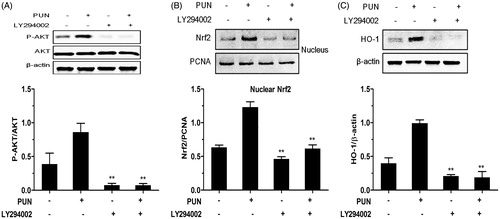
Role of the PI3K/Akt pathway in cytoprotection induced by PUN in IEC-6 cells
To investigate whether PUN has a cytoprotection effect by activating PI3K/Akt, IEC-6 cells were cultured with LY294002 for 1 h, and then incubated with or without PUN (10 μM) for 12 h. Then cells were exposed to 42 °C for 6 h, and cell viability was measured by the MTT assay. To reveal the effect of PUN on cell apoptosis induced by heat stress, apoptotic cells were measured by Annexin V-FITC/PI double-staining. Inhibition of the Akt pathway abolished the protective effect of PUN against cell vitality and apoptosis induced by heat stress ().
Figure 7. Effect of PUN and selective inhibitor LY294002 on heat stress-induced cell death and apoptosis in IEC-6 cells. Cells were pretreated with 10 μM LY294002 for 1 h, incubated with PUN (10 μM) for another 12 h, and then exposed to 42 °C for 6 h. (A) Cell viability was measured by the MTT assay. (B) and (C) Cell apoptosis was determined by the annexin V-FITC/PI staining assay. Data are expressed as mean ± S E M of three independent experiments. Differences between mean values were assessed by one-way ANOVA. ##p < 0.01 compared with the heat-stressed group; **p < 0.01 compared with the PUN treatment group; &&p < 0.01 compared with the control group.
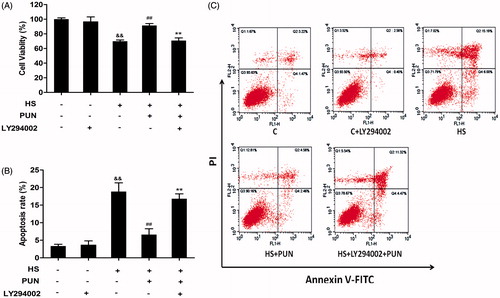
Discussion
Polyphenols have been reported to have many beneficial health effects. Pomegranate is an excellent source of polyphenols, which have been shown to have antioxidant, anti-inflammation and anti-diarrhoea effects [Citation28–30]. PUN, one of the most abundant polyphenols, extracted from the pomegranate husk, is a strong antioxidant [Citation31]. A previous study has shown that PUN is non-toxic to mouse macrophages [Citation25]. In the current study, the MTT assay indicated that PUN did not inhibit cell viability when cells were treated with PUN in concentrations from 0 to 40 μM (). Previous studies have revealed the antioxidant effect of PUN, but its effect on heat stress-induced oxidative stress and apoptosis in IEC-6 cells has never been studied.
Intestinal epithelium can be destroyed by many stressors, such as LPS, pharmacological drugs, endotoxins and extreme heat. Focusing on heat stress, IEC-6 cells were chosen as an in vitro model to analyse cell viability and the mechanism of PUN against oxidative stress induced by heat stress. It has been shown that IEC-6 cell vitality decreases depending on temperature and duration of heat exposure [Citation32]. In our study, cell vitality significantly decreased when cells were exposed to 42 °C for 6 h, thus these conditions were chosen for the current in vitro study (). Our results showed that PUN significantly attenuated heat stress-induced cell damage in a concentration-dependent manner ().
Previous studies have shown that oxidative stress is the key factor in heat stress-induced rat small intestine injury [Citation33,Citation34]. Overproduction of ROS causes oxidative stress and contributes to small intestinal mucosal damage [Citation35]. MDA content is frequently used to measure the degree of lipid peroxidation, which is closely related to cell damage [Citation36]. Preventive antioxidants such as SOD correlate with the elimination of free radicals [Citation37]. When excessively produced, NO may react with superoxide anion radicals, leading to the strong oxidant, peroxynitrite, and destroying functional tissues [Citation38]. In the current study, PUN significantly decreased ROS and NO production, and the level of MDA (), while increasing SOD activity in IEC-6 cells (). These findings indicate that PUN could increase the antioxidant capacity and prevention of oxidative stress induced by heat stress in IEC-6 cells.
HO-1, is one of the pivotal cytoprotective enzymes in cellular defence which have been shown to be involved in cellular responses to oxidative stress, heat shock, cytokines and LPS [Citation39]. Many investigations have confirmed the cytoprotective effects of HO-1 against oxidative stress in a wide variety of cells, including IEC-6 cells [Citation40–42]. Although the mechanisms underlying the HO-1 effects on gene expression are not well known, the results obtained in recent years have demonstrated its importance in the modulation of oxidative stress. In this study we found that pretreatment with PUN significantly up-regulated HO-1 expression in a time- and concentration-dependent manner ().
The transcription factor, Nrf2, plays a crucial role in the up-regulation of cytoprotective genes and proteins, principally in response to oxidative stress [Citation43]. Under basal conditions, Nrf2 is anchored in the inactive form to the cytoplasm through binding to Keap1. In response to oxidative stress, Nrf2 is released from Keap1, leading to nuclear translocation, which induces the expression of ARE-related genes, including HO-1 [Citation44]. In our study, the nuclear translocation of Nrf2 increased significantly in a time- and dose-dependent manner when cells were pretreated with PUN (). Furthermore, the immunofluorescence staining indicated Nrf2 translocated from cytoplasm into nucleus ().
PI3K/Akt, which is activated through phosphorylation of its threonine 308 residue, plays vital roles in the regulation of proliferation, differentiation and survival [Citation45]. It has been reported that PUN prevents LPS-induced oxidative stress via PI3K/Akt activation [Citation21]. Our findings show that the phosphorylation of Akt in IEC-6 cells significantly increased in a time- and concentration-dependent manner after treatment with PUN, while there was no significant change in the total Akt protein level (). Researchers have shown that Nrf2 is a target of the PI3K/Akt pathway and the nuclear translocation of Nrf2 requires the activation of the PI3K/Akt signalling pathway [Citation46,Citation47]. To further demonstrate the role of the PI3K/Akt pathway, IEC-6 cells were treated with LY294002 (a specific PI3K/Akt inhibitor) and PUN. The results indicated that inhibition of the PI3K/Akt pathway significantly inhibited the phosphorylation of PI3K/Akt, attenuated the nuclear localisation of Nrf2 and abolished HO-1 protein expression induced by PUN (). These results revealed that the PI3K/Akt pathway was involved in Nrf2 activation induced by PUN. Furthermore, LY294002 attenuated the cytoprotective effect of PUN against heat stress-induced cell vitality and apoptosis (). Thus, these results confirmed that the PI3K/Akt pathway plays a crucial role in cellular antioxidant defence.
Although we revealed that PUN had the ability to reduce heat stress-induced decrease in cell vitality in IEC-6 cells via the PI3K/Akt pathway and the Nrf2 pathway, it has to be emphasised whether there is the same effect in vivo or in other cell lines. In addition, besides Nrf2, other oxidant-sensitive transcription factors, such as nuclear factor-κB (NF-κB), activator protein-1 (AP-1) and signal transducer and activator of transcription 3 (STAT3), may regulate the expression of antioxidant enzymes [Citation48], and PUN may promote the nuclear translocation of Nrf2 via other signalling pathways, such as mitogen-activated protein kinases [Citation49]. Further investigations will be carried out in the future to reveal these mechanisms.
Conclusions
In summary, our results showed that PUN protected IEC-6 cells against heat stress-induced cell death by decreasing ROS, MDA and NO production, while increasing SOD activity. Our data also demonstrated that the up-regulation of HO-1 was due to the nuclear translocation of Nrf2. Furthermore, the upstream signalling pathway PI3K/Akt plays a central role in PUN-induced Nrf2 translocation. These findings provide new perspectives for protecting intestinal injury induced by heat stress.
Acknowledgements
We are thankful for the help from the members of CAU-BUA TCVM teaching and research team.
Disclosure statement
This work was supported by grants from the National Natural Science Foundation of China (No. 31272478), National Twelve-Five Technological Supported Plan of China (No. 2013BAD10B04), Ministry of Agriculture, public service sectors agriculture research projects (No. 201403051-07), Importation and Development of High-Caliber Talents Project of Beijing Municipal Institutions (CIT&TCD20130324). The authors declare that there is no conflict of interests regarding the publication of this article. The authors alone are responsible for the content and writing of the paper.
References
- Turner JR. Intestinal mucosal barrier function in health and disease. Nat Rev Immunol 2009;9:799–809.
- Malago JJ, Koninkx JF, van Dijk JE. The heat shock response and cytoprotection of the intestinal epithelium. Cell Stress Chaperones 2002;7:191–9.
- Leon LR, DuBose DA, Mason CW. Heat stress induces a biphasic thermoregulatory response in mice. Am J Physiol Regul Integ Compar Physiol 2005;288:R197–204.
- Shen L, Su L, Turner JR. Mechanisms and functional implications of intestinal barrier defects. Dig Dis 2009;27:443–9.
- Yu J, Liu F, Yin P, Zhu X, Cheng G, Wang N, et al. Integrating miRNA and mRNA expression profiles in response to heat stress-induced injury in rat small intestine. FunctI Integr Genomics 2011;11:203–13.
- Yu J, Liu F, Yin P, Zhao H, Luan W, Hou X, et al. Involvement of oxidative stress and mitogen-activated protein kinase signaling pathways in heat stress-induced injury in the rat small intestine. Stress 2013;16:99–113.
- Pisoschi AM, Pop A. The role of antioxidants in the chemistry of oxidative stress: A review. Eur J Med Chem 2015;97:55–74.
- Chang CJ, Lin JF, Chang HH, Lee GA, Hung CF. Lutein protects against methotrexate-induced and reactive oxygen species-mediated apoptotic cell injury of IEC-6 cells. PloS ONE 2013;8:e72553.
- Jaiswal AK. Nrf2 signaling in coordinated activation of antioxidant gene expression. Free Rad Biol Med 2004;36:1199–207.
- Deng Y, Zhu J, Mi C, Xu B, Jiao C, Li Y, et al. Melatonin antagonizes Mn-induced oxidative injury through the activation of Keap1-Nrf2-ARE signaling pathway in the striatum of mice. Neurotox Res 2015;27:156–71.
- Chowdhury SR, Sengupta S, Biswas S, Sen R, Sinha TK, Basak RK, et al. Low fucose containing bacterial polysaccharide facilitate mitochondria-dependent ROS-induced apoptosis of human lung epithelial carcinoma via controlled regulation of MAPKs-mediated Nrf2/Keap1 homeostasis signaling. Mol Carcinog2015;54:1636–55.
- Chapple SJ, Siow RC, Mann GE. Crosstalk between Nrf2 and the proteasome: therapeutic potential of Nrf2 inducers in vascular disease and aging. Int J Biochem Cell Biol 2012;44:1315–20.
- Chen XL, Kunsch C. Induction of cytoprotective genes through Nrf2/antioxidant response element pathway: A new therapeutic approach for the treatment of inflammatory diseases. Curr Pharm Des 2004;10:879–91.
- Jeong WS, Jun M, Kong AN. Nrf2: A potential molecular target for cancer chemoprevention by natural compounds. Antiox Redox Signaling 2006;8:99–106.
- Liu W, Xu Z, Yang T, Deng Y, Xu B, Feng S. Tea polyphenols protect against methylmercury-induced cell injury in rat primary cultured astrocytes, involvement of oxidative stress and glutamate uptake/metabolism disorders. Mol Neurobiol 2015. PMID 25952541. 1–15. http://link.springer.com/article/10.1007/s12035-015-9161-y
- Bloomer SA, Zhang HJ, Brown KE, Kregel KC. Differential regulation of hepatic heme oxygenase-1 protein with aging and heat stress. J Gerontol A, 2009;64:419–25.
- Chen YC, Chow JM, Lin CW, Wu CY, Shen SC. Baicalein inhibition of oxidative-stress-induced apoptosis via modulation of ERKs activation and induction of HO-1 gene expression in rat glioma cells C6. Toxicol Appl Pharmacol 2006;216:263–73.
- Chung SW, Liu X, Macias AA, Baron RM, Perrella MA. Heme oxygenase-1-derived carbon monoxide enhances the host defense response to microbial sepsis in mice. J ClinI Invest 2008;118:239–47.
- Cho SG, Choi EJ. Apoptotic signaling pathways: caspases and stress-activated protein kinases. J Biochem Mol Biol 2002;35:24–7.
- Jayasooriya RG, Park SR, Choi YH, Hyun JW, Chang WY, Kim GY. Camptothecin suppresses expression of matrix metalloproteinase-9 and vascular endothelial growth factor in DU145 cells through PI3K/Akt-mediated inhibition of NF-kappaB activity and Nrf2-dependent induction of HO-1 expression. Environ Toxicol Pharmacol 2015;39:1189–98.
- Xu X, Li H, Hou X, Li D, He S, Wan C, et al. Punicalagin induces Nrf2/HO-1 expression via upregulation of PI3K/AKT pathway and inhibits LPS-induced oxidative stress in RAW264.7 macrophages. Mediators Inflamm 2015;2015:380218.
- Wang P, Peng X, Wei ZF, Wei FY, Wang W, Ma WD, et al. Geraniin exerts cytoprotective effect against cellular oxidative stress by upregulation of Nrf2-mediated antioxidant enzyme expression via PI3K/AKT and ERK1/2 pathway. Biochim Biophys Acta 2015;1850:1751–61.
- Erkan-Koc B, Turkyilmaz M, Yemis O, Ozkan M. Effects of various protein- and polysaccharide-based clarification agents on antioxidative compounds and colour of pomegranate juice. Food Chem 2015;184:37–45.
- Aqil F, Munagala R, Vadhanam MV, Kausar H, Jeyabalan J, Schultz DJ, et al. Anti-proliferative activity and protection against oxidative DNA damage by punicalagin isolated from pomegranate husk. Food Res Int 2012;49:345–53.
- Xu X, Yin P, Wan C, Chong X, Liu M, Cheng P, et al. Punicalagin inhibits inflammation in LPS-induced RAW264.7 macrophages via the suppression of TLR4-mediated MAPKs and NF-kappaB activation. Inflammation 2014;37:956–65.
- Deng W, Viar MJ, Johnson LR. Polyamine depletion inhibits irradiation-induced apoptosis in intestinal epithelia. Am J Physiol Gastrointest Liver Physiol 2005;289:G599–606.
- Lee ES, Lee HJ, Lee YJ, Jeong JH, Kang S, Lim YB. Chemical chaperones reduce ionizing radiation-induced endoplasmic reticulum stress and cell death in IEC-6 cells. Biochemical and b Biophys Res Commun 2014;450:1005–9.
- Aharoni S, Lati Y, Aviram M, Fuhrman B. Pomegranate juice polyphenols induce a phenotypic switch in macrophage polarization favoring a M2 anti-inflammatory state. BioFactors 2015;41:44–51.
- Kilic E, Turkoglu A, Keles A, Ekinci A, Kesgin S, Gumus M. The antioxidant effects of pomegranate extract on local and remote organs in a mesenteric ischemia and reperfusion model. Redox Rep 2015. PMID 26010809.
- Souli A, Sebai H, Rtibi K, Chehimi L, Sakly M, Amri M, et al. Inhibitory effects of two varieties of Tunisian pomegranate (Punica granatum L.) Extracts on gastrointestinal transit in rat. JMed Food 2015;18:1007–12.
- Wang Y, Zhang H, Liang H, Yuan Q. Purification, antioxidant activity and protein-precipitating capacity of punicalin from pomegranate husk. Food Chem 2013;138:437–43.
- Yu J, Yin P, Yin J, Liu F, Zhu X, Cheng G, et al. Involvement of ERK1/2 signalling and growth-related molecules' expression in response to heat stress-induced damage in rat jejunum and IEC-6 cells. Int J Hyperthermia 2010;26:538–55.
- Lu A, Wang H, Hou X, Li H, Cheng G, Wang N, et al. Microarray analysis of gene expression profiles of rat small intestine in response to heat stress. J Biomol Screen 2011;16:655–67.
- Yu J, Yin P, Liu F, Cheng G, Guo K, Lu A, et al. Effect of heat stress on the porcine small intestine: a morphological and gene expression study. Compar Biochem Physiol A MolI Integr Physiol 2010;156:119–28.
- Omatsu T, Naito Y, Handa O, Mizushima K, Hayashi N, Qin Y, et al. Reactive oxygen species-quenching and anti-apoptotic effect of polaprezinc on indomethacin-induced small intestinal epithelial cell injury. J Gastroenterol 2010;45:692–702.
- Yin H, Pan X, Song Z, Wang S, Yang L, Sun G. Protective effect of wheat peptides against indomethacin-induced oxidative stress in IEC-6 cells. Nutrients 2014;6:564–74.
- Chen LH, Lin ZB, Li WD. Ganoderma lucidum polysaccharides reduce methotrexate-induced small intestinal damage in mice via induction of epithelial cell proliferation and migration. Acta Pharmacol Sinica 2011;32:1505–12.
- Mikulec CD, Rundhaug JE, Simper MS, Lubet RA, Fischer SM. The chemopreventive efficacies of nonsteroidal anti-inflammatory drugs: the relationship of short-term biomarkers to long-term skin tumor outcome. Cancer Prevention Res (Phila) 2013;6:675–85.
- Shibahara S. Regulation of heme oxygenase gene expression. SeminHematol 1988;25:370–6.
- Liu C, Zhu C, Wang G, Xu R, Zhu Y. Higenamine regulates Nrf2-HO-1-Hmgb1 axis and attenuates intestinal ischemia-reperfusion injury in mice. Inflamm Res 2015;64:395–403.
- Peng S, Zhang B, Yao J, Duan D, Fang J. Dual protection of hydroxytyrosol, an olive oil polyphenol, against oxidative damage in PC12 cells. Food Funct 2015;6:2091–100.
- Chen N, Wu L, Yuan H, Wang J. ROS/autophagy/Nrf2 pathway mediated low-dose radiation induced radio-resistance in human lung adenocarcinoma A549 cell. Int J Biol Sci 2015;11:833–44.
- Zhang Q, Pi J, Woods CG, Andersen ME. A systems biology perspective on Nrf2-mediated antioxidant response. Toxicol Appl Pharmacol 2010;244:84–97.
- Wu J, Cheng M, Liu Q, Yang J, Wu S, Lu X, et al. Protective role of tert-butylhydroquinone against sodium fluoride-induced oxidative stress and apoptosis in PC12 cells. Cell Mol Neurobiol 2015;35:1017–25.
- Shanware NP, Bray K, Abraham RT. The PI3K, metabolic, and autophagy networks: interactive partners in cellular health and disease. Annu Rev Pharmacol Toxicol 2013;53:89–106.
- Ma W, Hu J, Cheng Y, Wang J, Zhang X, Xu M. Ginkgolide B protects against cisplatin-induced ototoxicity: enhancement of Akt-Nrf2-HO-1 signaling and reduction of NADPH oxidase. Cancer Chemother Pharmacol 2015;75:949–59.
- Yin X, Wang X, Fan Z, Peng C, Ren Z, Huang L, et al. Hyperbaric oxygen preconditioning attenuates myocardium ischemia-reperfusion injury through upregulation of heme oxygenase 1 expression: PI3K/Akt/Nrf2 pathway involved. J Cardiovasc Pharmacol Therapeut 2015;20:428–38.
- Yu JH, Kim H. Oxidative stress and inflammatory signaling in cerulein pancreatitis. World J Gastroenterol 2014;20:17324–9.
- Rodriguez-Ramiro I, Ramos S, Bravo L, Goya L, Martin MA. Procyanidin B2 induces Nrf2 translocation and glutathione S-transferase P1 expression via ERKs and p38-MAPK pathways and protect human colonic cells against oxidative stress. Eur J Nutrition 2012;51:881–92.